-
PDF
- Split View
-
Views
-
Cite
Cite
Changxi Yin, Lijun Gan, Denny Ng, Xie Zhou, Kai Xia, Decreased panicle-derived indole-3-acetic acid reduces gibberellin A1 level in the uppermost internode, causing panicle enclosure in male sterile rice Zhenshan 97A, Journal of Experimental Botany, Volume 58, Issue 10, July 2007, Pages 2441–2449, https://doi.org/10.1093/jxb/erm077
- Share Icon Share
Abstract
Cytoplasmic male sterile (CMS) rice Zhenshan 97A (ZS97A) has been widely used in hybrid rice production in China. However, ZS97A suffers from serious panicle enclosure, which blocks normal pollination and greatly reduces seed production of hybrid rice. Little is known about the cause of panicle closure in ZS97A. In this study, it was found that the occurrence of cytoplasmic male sterility caused a deficiency of indole-3-acetic acid (IAA) in ZS97A panicles, and less IAA was provided to the uppermost internode (UI). Further, it was found that the decreased panicle-derived IAA caused a gibberellin A1 (GA1) deficiency in the UI by the down-regulation of OsGA3ox2 transcript level. Reduced GA1 level in the UI led to decreases of both cell number and cell elongation, resulting in a shortened UI. The shortened UI was unable to push the panicle out of the flag leaf sheath that remained normal, which resulted in panicle enclosure in ZS97A. These findings suggest that decreased panicle-derived IAA reduces the GA1 level in the UI, causing panicle enclosure in CMS rice ZS97A.
Introduction
Hybrid rice normally has more than 15–20% yield advantage over inbred varieties (Sabar and Akhter, 2003), it is widely applied in China and other countries, and it covers about 50% of China's rice acreage and accounts for 60% of production (Normile, 2000). Hybrid rice is produced by crossing two genetically different rice plants with superior qualities by cross-pollination. Because rice is a strictly self-pollinating crop, one of the parents must have a sterile male part in order to facilitate such a cross. In 1970, Chinese researchers discovered a wild abortive (WA) rice plant. Thereafter, a number of cytoplasmic male sterile (CMS) lines were developed from the WA plant for hybrid rice production through a three-line breeding system. The three-line breeding system requires a CMS line, a maintainer line, and a restorer line. The maintainer line is used to pollinate the CMS line to maintain a supply of CMS line seeds, and the restorer line is used as male parent to produce F1 hybrid rice seeds via the cross between CMS and restorer line.
However, panicle enclosure is associated with almost all CMS lines in hybrid rice seed production (Shen et al., 1987; Yang et al., 2000, 2002; Gangashetti et al., 2004). Panicle enclosure means that the panicle is partly or fully enclosed within the flag leaf sheath, which blocks normal pollination and greatly reduces seed production of hybrid rice. However, panicle enclosure in CMS rice can be eliminated by exogenous bioactive gibberellin (GA). This suggests that CMS rice might lack enough bioactive GA.
Zhenshan 97A (ZS97A), an elite CMS line, is the female parent of a number of widely used hybrids in China. ZS97A and its maintainer line Zhenshan 97B (ZS97B) have an identical nuclear background but different cytoplasms. They normally show no significant phenotypic difference at the vegetative stage, but distinct differences at the reproductive stage. ZS97A pollen grains are completely abortive, while ZS97B develops normal fertile pollen grains. ZS97A suffers from serious panicle enclosure, and panicle enclosure in ZS97A can be eliminated by exogenous GA which promotes elongation of the UI. However, ZS97B develops a fertile panicle, and ZS97B UI is long enough to push its panicle out of the flag leaf sheath completely. So it is suggested that panicle enclosure may be a phenotype associated with CMS rice, and that the UI of CMS rice may lack enough bioactive GA for normal elongation of the UI. In this study, CMS line rice ZS97A, associated with serious panicle enclosure, was used to investigate the cause of panicle enclosure in CMS rice.
Materials and methods
Plant materials and growth conditions
ZS97A (Oryza sativa L.) and its isonuclear maintainer line ZS97B were used in this study. A WA rice plant (Oryza sativa f. spontanea) was crossed with ZS97B, and the F1 plant was repeatedly backcrossed to the recurrent male parent ZS97B, up to the sixth backcross generation to get a WA-type CMS rice named ZS97A. Rice seeds were immersed in distilled water for 2 d, grown for 1 month in a greenhouse, and then transplanted to an experimental paddy field in Nanjing, China.
Pollen viability test
Mature pollen grains of ZS97A and ZS97B were stained by 1% I2–KI, and then examined with a microscope (Zeiss Stemi 2000-C).
Observation of panicle development and internode elongation
Panicle development was divided into eight stages as described previously (Hoshikawa, 1989a). The examination of the young panicle after removing the leaves was performed with a magnifying glass to determine the developmental stage of the panicle. Internode length was measured with a ruler.
Light microscopy
After the heading stage, mature UIs of ZS97A and ZS97B were collected for light microscopy. The UIs were equally divided into three sections (top, middle, and bottom), and each section was then sampled and fixed with 70% (v/v) ethanol:acetic acid:10% (w/v) formaldehyde (90:5:5, by vol.), dehydrated with an n-butanol series, and embedded in paraffin. Longitudinal sections were then stained with haematoxylin solution and observed under a light microscope (OLYMPUS BH-2).
Treatments with exogenous plant hormones and their inhibitors
At the early heading stage, the plants of ZS97A and ZS97B were sprayed with 150 μM GA1 and 100 μM uniconazole solution, respectively. Control plants were treated with distilled water. GA1 was a gift from Professor N Murofushi (Tokyo University, Japan), and uniconazole was purchased from Sichuan Academy of Chemical Industry Research and Design (Chengdu, China).
Plants with elongating UIs about 7.5 cm long were selected for TIBA treatment at the early heading stage. Exogenous 100 μM TIBA aqueous solution was supplied to the uppermost node of the rice stem in a ring of absorbent cotton. Control plants received absorbent cottons containing the same volume of distilled water. The solutions were refreshed every 12 h until 48 h after the first application. TIBA was purchased from Sigma Chemical Company (St Louis, MO, USA).
At the early heading stage, plants with elongating UIs about 7.5 cm long were selected for decapitation. Decapitation was performed by excising the panicle 1 mm above the uppermost node of the stem with a razor blade at a right angle to the vertical axis of the stem. The decapitated plants were treated immediately on the excised stumps with absorbent cottons separately containing solutions of 50 μM IAA, 150 μM GA1, or distilled water. The solutions were refreshed every 12 h until 48 h after the first application.
Plant hormone analysis by enzyme-linked immunosorbent assay (ELISA)
Samples for the assay of plant hormones were harvested separately and washed three times with distilled water then dried with tissue paper. Samples (0.5 g) were weighted and placed into liquid nitrogen and then kept at –28 °C until extraction of hormones.
Extraction and purification of plant hormones prior to immunoassay have been described previously (Cohen et al., 1987; Chen et al., 1996; Yang et al., 2001; Cui et al., 2005). The main steps are: extraction of homogenized samples in cold 80% (v/v) aqueous methanol at a rate of 5 ml g−1 fresh weight overnight at 4 °C with butylated hydroxytoluene (10 mg l−1) to prevent oxidation. The supernatant was collected after centrifugation at 10 000 g (4 °C) for 20 min. Then, the crude extract was passed through a C18 Sep-Pak cartridge (Waters, Milford. MA). The filtrate was collected and 400 μl removed and dried in N2. The residue was dissolved in 200 μl phosphate-buffered saline (PBS) (0.01 M, pH 9.2) and adjusted to pH 8.5, before partitioning three times with equal volume of ethyl acetate. The remaining aqueous phase was adjusted to pH 2.5 and extracted three times with equal volumes of ethyl acetate. The extracts (ethyl acetate phase) were pooled and dried in N2, the residue was either redissolved in 200 μl PBS (0.01 M, pH 7.4) to analyse GA1, or redissolved in 200 μl 100% methanol for methylation with freshly synthesized ethereal diazomethane and taken up with 200 μl PBS for analysis of IAA.
The levels of IAA and GA1 were then determined by ELISA based on monoclonal antibodies (provided by Nanjing Agricultural University, China), as described previously (Cui et al., 2005).
RT-PCR assay
Total RNA was isolated from the UI using the guanidinium isocyanate/acidic phenol method described by Chomczynski and Sacchi (1987). The cDNA was synthesized using the first-strand cDNA synthesis kit (Fermentas) including Oligo(dT), Ribolock RNase Inhibitor and M_MuLV, dNTP mix. 0.5 μl of cDNA was used as template in a 25 μl PCR reaction. The OsGA20ox2 fragment (268 bp; Genbank accession number AP003561) was amplified with primers: (5′-TAC TAC AGG GAG TTC TTC GCG GAC AGC A-3’) and (5′-TGT GCA GGC AGC TCT TAT ACC TCC CGT T-3’). The OsGA3ox2 fragment (346 bp; Genbank accession number AP002523) was amplified with primers: (5′-TCT CCA AGC TCA TGT GGT CCG AGG GCT A-3’) and (5′-TGG AGC ACG AAG GTG AAG AAG CCC GAG T-3’). The OsGA2ox1 fragment (332 bp; Genbank accession number AC119288) was amplified with primers: (5′-CGA GCA AAC GAT GTG GAA GGG CTA CAG G-3’) and (5′-TGG CTC AGG CGG AGT GAG TAC ATT GTC G-3’). OsGA20ox2, OsGA3ox2, and OsGA2ox1 fragments were amplified at 94 °C for 3 min; followed by 30 cycles at 94 °C for 45 s, 55 °C for 45 s, 72 °C for 45 s, and finished by an extension at 72 °C for 10 min. The rice actin (ACTIN) gene fragment was used as an internal control in the RT-PCR analysis with the primer pair (5′-TCC ATC TTG GCA TCT CTC AG-3’) and (5′-GTA CCC GCA TCA GGC ATC TG-3’). The ACTIN fragment (335 bp; Genbank accession number AB047313) was amplified at 94 °C for 3 min, followed by 20 cycles at 94 °C for 45 s, 55 °C for 45 s, 72 °C for 45 s, and finished by an extension at 72 °C for 10 min.
IAA oxidase activity assay
The panicles were collected at the pollen development stage and the heading stage. IAA oxidase activity was assayed as described previously (Mills and Todd, 1973).
Results
ZS97A is sterile with abortive pollen grains
Pollen grains of CMS rice ZS97A are sterile, while those of its isonuclear maintainer ZS97B are fertile. As shown in Fig. 1, there was nothing but empty cell wall in abortive pollen grains of ZS97A, while fertile pollen grains of ZS97B were fully filled with starch granules and could be stained by 1% I2–KI. A statistical result showed that ZS97B pollen viability was very high (99.2±0.6%), while all ZS97A pollen grains were abortive and could not be stained by 1% I2–KI.
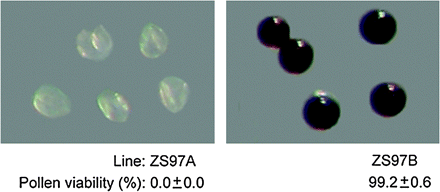
Pollen viability test. Abortive pollen grains of ZS97A could not be stained by 1% I2-KI, while fertile pollen grains of ZS97B could be stained. At least 3000 pollen grains collected from 30 anthers were examined and counted to get a statistical result of pollen viability.
Cytoplasmic male sterility causes panicle enclosure by shortening the UI length in ZS97A
The elongation of the internode along with the development of the panicle was compared between ZS97A and ZS97B. Both ZS97A and ZS97B had four elongated internodes from the uppermost one (UI) to the lower ones (internodes II, III, and IV) after the internode elongation stage (Fig. 2B). Internode IV was the lowest one in the elongated stem portion. As shown in Fig. 2A, internode IV began to elongate at the first bract differentiation stage (stage 1), followed by the elongation of internode III at the secondary rachis–branch differentiation stage (stage 3). Internodes IV and III ceased their elongation at the stage of meiosis in anthers (stage 7) and pollen level enrichment (stage 8), respectively. The elongation of internode II started from stage 7. After stage 7, the pollen grains of ZS97A became abortive, thereafter, internode II of ZS97A had a lower elongation rate than that of ZS97B. Finally, the UI began to elongate at stage 8, and the panicle emerged from the flag leaf sheath (this is called heading). At the heading stage, ZS97A had a much lower elongation rate of the UI than ZS97B (Fig. 2C), leading to a decrease in final UI length (Fig. 2B). This result suggested that the decrease of the internode elongation rate of ZS97A was due to the occurrence of the cytoplasmic male sterility, because ZS97A and ZS97B had the same internode elongation rate before the abortion of ZS97A pollen grains, while after the abortion, ZS97A had a lower internode elongation rate than ZS97B.
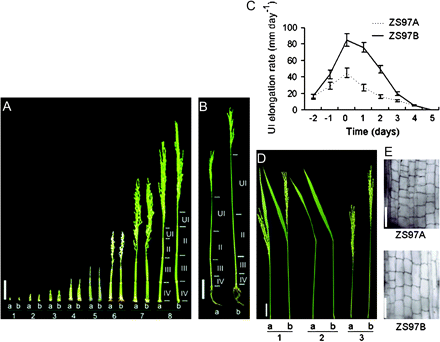
Panicle enclosure in CMS rice ZS97A was caused by shortening of the UI. (A) Internode elongation process of ZS97A and ZS97B throughout the panicle development. (1) Stage 1, first bract differentiation; (2) Stage 2, primary rachis–branch differentiation; (3) Stage 3, secondary rachis–branch differentiation; (4) Stage 4, glumous flower differentiation; (5) Stage 5, stamen and pistil differentiation; (6) Stage 6, pollen mother cell differentiation; (7) Stage 7, meiosis in anthers; (8) Stage 8, pollen level enrichment. Scale bar=50 mm. (B) Fully elongated internodes of ZS97A and ZS97B. Scale bar=100 mm. (C) Comparison of UI elongation rate between ZS97A and ZS97B at the heading stage. Days before and after the beginning of the heading stage are shown as negative and positive numbers, respectively. Values are means ±SE of 20 UIs. (D) Phenotypes of ZS97A and ZS97B after the heading stage. (1) ZS97A panicle was enclosed within the flag leaf sheath, while ZS97B panicle extended fully out of the flag leaf sheath. (2) ZS97A and ZS97B had flag leaf sheaths of almost the same length. (3) The UI of ZS97A was much shorter than that of ZS97B. Scale bar=50 mm. (E) Microscopic longitudinal section in the middle section of the UI. Scale bar=100 μm. UI, uppermost internode; II, internode II; III, internode III; IV, internode IV; a, ZS97A; b, ZS97B.
After the heading stage, the final length of the UI in ZS97A was only 185.2±16.3 mm, which was much less than that of 321.4±27.6 mm in ZS97B (Table 1). The shortened UI was unable to push the panicle out of the flag leaf sheath that remained normal, which resulted in panicle enclosure in ZS97A (Fig. 2D).
Lengths of the UI, the flag leaf sheath, and the cell in the top, middle, and bottom sections of the UIs in ZS97A and ZS97B
Trait | ZS97A | ZS97B |
UI (mm) | 185.2±16.3 | 321.4±27.6 |
Flag leaf sheath (mm) | 302.1±27.8 | 301.7±26.9 |
Top section cell (μm) | 44.8±5.1 | 54.2±6.2 |
Middle section cell (μm) | 65.9±6.6 | 89.7±9.1 |
Bottom section cell (μm) | 48.4±5.8 | 60.3±6.7 |
Trait | ZS97A | ZS97B |
UI (mm) | 185.2±16.3 | 321.4±27.6 |
Flag leaf sheath (mm) | 302.1±27.8 | 301.7±26.9 |
Top section cell (μm) | 44.8±5.1 | 54.2±6.2 |
Middle section cell (μm) | 65.9±6.6 | 89.7±9.1 |
Bottom section cell (μm) | 48.4±5.8 | 60.3±6.7 |
The data show means ±SE, n=20 for lengths of the UI and the flag leaf sheath, and n=100 for the cell length.
Lengths of the UI, the flag leaf sheath, and the cell in the top, middle, and bottom sections of the UIs in ZS97A and ZS97B
Trait | ZS97A | ZS97B |
UI (mm) | 185.2±16.3 | 321.4±27.6 |
Flag leaf sheath (mm) | 302.1±27.8 | 301.7±26.9 |
Top section cell (μm) | 44.8±5.1 | 54.2±6.2 |
Middle section cell (μm) | 65.9±6.6 | 89.7±9.1 |
Bottom section cell (μm) | 48.4±5.8 | 60.3±6.7 |
Trait | ZS97A | ZS97B |
UI (mm) | 185.2±16.3 | 321.4±27.6 |
Flag leaf sheath (mm) | 302.1±27.8 | 301.7±26.9 |
Top section cell (μm) | 44.8±5.1 | 54.2±6.2 |
Middle section cell (μm) | 65.9±6.6 | 89.7±9.1 |
Bottom section cell (μm) | 48.4±5.8 | 60.3±6.7 |
The data show means ±SE, n=20 for lengths of the UI and the flag leaf sheath, and n=100 for the cell length.
To understand further whether the shortening of the UI in ZS97A was caused by the decrease of cell number and/or cell length, longitudinal sections of mature UIs were compared after the heading stage. Three equally divided sections were subjected to microscopic observation. In each section, the cell length of ZS97A was shorter than that of ZS97B, and the cell length decreased ratios were 1.21-, 1.36-, and 1.25-fold from the top to the bottom section, respectively (Table 1; Fig. 2E). However, the UI length of ZS97A was much shorter than that of ZS97B, and the UI length decreased ratio was 1.74-fold (Table 1). Therefore, the shortening of the UI length in ZS97A should be attributed to the decreases of both cell number and cell length.
Shortening of the UI length is caused by GA1-deficiency in the UI of ZS97A
Growth of rice internodes is under strict spatiotemporal control. Rapid internode elongation usually happens at the reproductive stage. At the heading stage, the UI of ZS97B elongated rapidly (Fig. 2C), and the UI pushed the panicle out of the flag leaf sheath completely (Fig. 3A, c). However, the elongation rate of the UI in ZS97A was much lower than that in ZS97B (Fig. 2C), and the shortened UI was unable to push the panicle out of the flag leaf sheath completely in ZS97A (Fig. 3A, a).
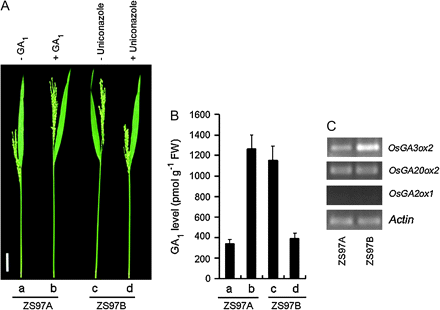
Shortening of the UI in ZS97A was caused by GA1-deficiency. (A) Application of exogenous GA1 to ZS97A and uniconazole to ZS97B. (a) ZS97A exhibited a serious panicle enclosure. (b) Application of 150 μM GA1 eliminated panicle enclosure in ZS97A. (c) ZS97B exhibited no panicle enclosure. (d) Application of 100 μM uniconazole caused panicle enclosure in ZS97B. All the treatments were performed at the early heading stage once a day for four days, and photographs were taken when the UIs had reached their final lengths. Scale bar=50 mm. (B) Endogenous GA1 level in the UI. (a) to (d) Samples for the assay of plant hormone were collected 48 h after the first treatment of (A, a) to (A, d), respectively. Each column represents the mean ±SE of three replicates of independently harvested plant material. FW, fresh weight. (C) Transcript levels of selected genes involved in the GA metabolic pathway in elongating UIs of ZS97A and ZS97B. RT-PCR was performed as described in the Materials and methods section with Actin as the control.
Previous studies indicated that GA-deficient and GA-insensitive rice mutants show alterations in plant height (Ashikari et al., 1999; Spielmeyer et al., 2002), and that rice vegetative growth is primarily regulated by GA1 (Kobayashi et al., 1988, 1989, 1994). The content of GA1 is at the highest level during internodal elongation, and GA1 is proposed to play a major role in rice internode elongation (Kobayashi et al., 1988). Therefore, in order to investigate the cause of the shortening of the UI length in ZS97A, the endogenous bioactive GA1 level was compared between ZS97A and ZS97B UI. At the heading stage, endogenous GA1 level in the UI in ZS97A was only about one-third of that in ZS97B (Fig. 3B, a, c). The application of exogenous 150 μM GA1 increased the GA1 level in ZS97A UI (Fig. 3B, b), and promoted ZS97A UI elongation to the same length as ZS97B UI (Fig. 3A, b, c), thus pushing ZS97A panicle out of the flag leaf sheath (Fig. 3A, b). The application of 100 μM uniconazole (a GA biosynthesis inhibitor) markedly decreased GA1 level in ZS97B UI (Fig. 3B, d), and caused panicle enclosure in ZS97B by shortening the UI length (Fig. 3A, d). These data indicated that GA1-deficiency in the UI decreases the UI length, resulting in panicle enclosure in ZS97A.
GA1-deficiency in the UI of ZS97A is caused by reduced GA biosynthesis
In plants, GA levels are modulated by both GA biosynthetic enzymes and GA catabolism enzymes. The genes encoding GA biosynthetic enzymes have been identified in numerous species. Among these enzymes, GA 20-oxidase (GA20ox) and GA 3-oxidase (GA3ox), corresponding to the last steps of GA biosynthesis, are particularly important for the control of bioactive GA levels (Fleet and Sun, 2005). In addition to biosynthesis, GA levels are also modulated by catabolism via GA-2-oxidase (GA2ox), which converts bioactive GA1 to inactive GA8 (Sakamoto et al., 2004).
To investigate whether the GA1-deficiency in UI was caused by reduced GA biosynthesis and/or by high GA catabolism, the transcript levels of OsGA20ox, OsGA3ox, and OsGA2ox in elongating UI were compared between ZS97A and ZS97B. As shown in Fig. 3C, ZS97A had a much lower OsGA3ox2 transcript level in its UI than ZS97B, while no difference in the transcript level of OsGA20ox2 was detected in the UI between ZS97A and ZS97B. OsGA2ox1 transcript was not detected in UIs of both ZS97A and ZS97B. This result indicated that GA1-deficiency in UI of ZS97A is most likely caused by reduced GA biosynthesis.
CMS panicle of ZS97A provides less IAA to the UI, which contributes to reduced GA biosynthesis
Because apically-derived IAA is necessary for GA biosynthesis in stem (Ross, 1998; Wolbang and Ross, 2001; Wolbang et al., 2004), there was a need to test whether reduced GA biosynthesis in UI of ZS97A was caused by a deficiency in IAA. Therefore, the IAA levels in UI and in the panicles of ZS97A and ZS97B were measured directly. As shown in Fig. 4A, IAA levels in ZS97A panicles were not more than 60% of that in ZS97B panicles throughout pollen development. At the uninucleate stage (stage IV) the pollen grains became abortive, thereafter the IAA level in ZS97A panicles was not more than 45% of that in ZS97B panicles. After the pollen development stage, the rice entered the heading stage. At the heading stage, the IAA level in the panicle or UI in ZS97A was about one-third of that in ZS97B (Fig. 4B). This result demonstrated that the occurrence of cytoplasmic male sterility in ZS97A was accompanied by IAA-deficiency in the panicle.
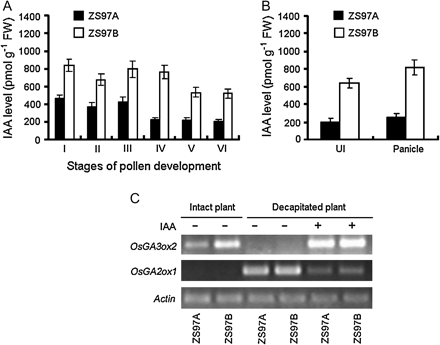
GA1-deficiency in the UI was caused by IAA-deficiency via the down-regulation of the OsGA3ox2 transcript level. (A) Endogenous IAA levels in the panicles of ZS97A and ZS97B throughout the pollen development process. (I) Stamen and pistil formation stage; (II) Pollen mother cell formation stage; (III) Pollen mother cell meiosis stage; (IV) Uninucleate pollen stage; (V) Binucleate pollen stage; (VI) Trinucleate pollen stage. (B) Endogenous IAA levels in the panicle and in the UI of ZS97A and ZS97B at the heading stage. In (A) and (B), each column represents the mean ±SE of three replicates of independently harvested plant material. FW, fresh weight. (C) Impact of exogenous 50 μM IAA on OsGA3ox2 and OsGA2ox1 transcript levels in the UIs of ZS97A and ZS97B at the early heading stage. For RT-PCR analysis, the UI was harvested 24 h after the first treatment. Actin was used as an internal control.
Taken together with above results, it was hypothesized that GA1-deficiency in the UI of ZS97A was caused by decreased IAA derived from the panicle. To test this hypothesis, TIBA (an inhibitor of auxin polar transport) was applied to the uppermost node of the rice stem. Application of 100 μM TIBA elicited decreases in IAA level by 2.35-fold and in GA1 level by 3.04-fold in ZS97B UI, and correspondingly, TIBA treatment decreased the UI elongation rate of ZS97B almost to the same level as the control plant of ZS97A (Table 2). This result could be explained by the fact that IAA in the elongating UI was mainly derived from the panicle, so TIBA could strongly inhibit IAA polar transport from the panicle to the UI, leading to IAA-deficiency in the UI, which was accompanied by GA1-deficiency in the UI and a decreased UI elongation rate. Decapitation also caused a huge decrease of panicle-derived IAA in the UI (Table 2). Thus for ZS97A, the CMS panicle had less IAA and provided less IAA to the UI, which, in turn, caused a GA1-deficiency in the UI and a decrease in UI length.
Effects of TIBA (100 μM) and decapitation on endogenous IAA and GA1 level and on UI elongation rate
Line | Treatment | IAA level (pmol g−1 FW) | GA1 level (pmol g−1 FW) | UI elongation rate (mm 48 h−1) |
ZS97A | H2O | 238.1±26.1 | 337.4±30.1 | 53.7±4.4 |
ZS97B | H2O | 658.4±63.3 | 1142.3±108.9 | 154.6±13.2 |
TIBA | 279.8±27.5 | 376.3±33.6 | 57.6±5.1 | |
Decapitation+H2O | 37.9±4.4 | 61.2±5.7 | 7.1±0.5 |
Line | Treatment | IAA level (pmol g−1 FW) | GA1 level (pmol g−1 FW) | UI elongation rate (mm 48 h−1) |
ZS97A | H2O | 238.1±26.1 | 337.4±30.1 | 53.7±4.4 |
ZS97B | H2O | 658.4±63.3 | 1142.3±108.9 | 154.6±13.2 |
TIBA | 279.8±27.5 | 376.3±33.6 | 57.6±5.1 | |
Decapitation+H2O | 37.9±4.4 | 61.2±5.7 | 7.1±0.5 |
TIBA was applied to the uppermost node of the rice stem, and H2O was used for controls. The UI was harvested 48 h after the first application. The data show means ±SE, n=3 for IAA and GA1 level, and n=20 for UI elongation rate. FW, fresh weight.
Effects of TIBA (100 μM) and decapitation on endogenous IAA and GA1 level and on UI elongation rate
Line | Treatment | IAA level (pmol g−1 FW) | GA1 level (pmol g−1 FW) | UI elongation rate (mm 48 h−1) |
ZS97A | H2O | 238.1±26.1 | 337.4±30.1 | 53.7±4.4 |
ZS97B | H2O | 658.4±63.3 | 1142.3±108.9 | 154.6±13.2 |
TIBA | 279.8±27.5 | 376.3±33.6 | 57.6±5.1 | |
Decapitation+H2O | 37.9±4.4 | 61.2±5.7 | 7.1±0.5 |
Line | Treatment | IAA level (pmol g−1 FW) | GA1 level (pmol g−1 FW) | UI elongation rate (mm 48 h−1) |
ZS97A | H2O | 238.1±26.1 | 337.4±30.1 | 53.7±4.4 |
ZS97B | H2O | 658.4±63.3 | 1142.3±108.9 | 154.6±13.2 |
TIBA | 279.8±27.5 | 376.3±33.6 | 57.6±5.1 | |
Decapitation+H2O | 37.9±4.4 | 61.2±5.7 | 7.1±0.5 |
TIBA was applied to the uppermost node of the rice stem, and H2O was used for controls. The UI was harvested 48 h after the first application. The data show means ±SE, n=3 for IAA and GA1 level, and n=20 for UI elongation rate. FW, fresh weight.
In order to clarify the specific reason why decreased panicle-derived IAA was accompanied by GA1-deficiency in the UI of ZS97A, exogenous IAA and GA1 were supplied to the decapitated plants of ZS97A and ZS97B. GA1 level, transcript levels of certain genes involved in GA metabolism in the UIs were then compared between ZS97A and ZS97B. As shown in Fig. 4C, decapitation significantly decreased the transcript level of OsGA3ox2 in UIs of both ZS97A and ZS97B, which could be completely rescued by the application of 50 μM IAA. On the other hand, the transcript level of OsGA2ox1 was very high in the UIs of decapitated plants, and the OsGA2ox1 transcript level could be strongly down-regulated by exogenous IAA application. Correspondingly, compared with intact control plant (Table 2), decapitation significantly decreased the GA1 level in the UI, as well as the elongation rate, while application of exogenous 50 μM IAA increased GA1 level by more than 4-fold and increased the elongation rate by more than 6-fold in both ZS97A and ZS97B decapitated plants (Table 3). However, in decapitated plants, even though the transcript level of OsGA3ox2 could be completely rescued by exogenous 50 μM IAA, OsGA2ox1 transcript could not be completely eliminated by exogenous 50 μM IAA (Fig. 4C). Therefore, in decapitated plants treated with 50 μM IAA, the GA1 level and the UI elongation rate were still lower than that in intact control plants (Tables 2, 3). In addition, exogenous 150 μM GA1 application caused significant increases in GA1 level and in UI elongation rate in both ZS97A and ZS97B decapitated plants (Table 3).
Effects of exogenous applied IAA, GA1 on endogenous GA1 level and on UI elongation rate in the decapitated plants of ZS97A and ZS97B
Line | Treatment | GA1 level (pmol g−1 FW) | UI elongation rate (mm 48 h−1) |
ZS97A | Decapitation+H2O | 55.2±5.3 | 6.8±0.5 |
Decapitation+IAA | 237.3±21.1 | 43.4±3.7 | |
Decapitation+GA1 | 1227±116.5 | 158.1±12.7 | |
ZS97B | Decapitation+H2O | 61.2±5.7 | 7.1±0.5 |
Decapitation+IAA | 253.0±24.8 | 45.4±3.9 | |
Decapitation+GA1 | 1298.2±101.5 | 164.2±15.2 |
Line | Treatment | GA1 level (pmol g−1 FW) | UI elongation rate (mm 48 h−1) |
ZS97A | Decapitation+H2O | 55.2±5.3 | 6.8±0.5 |
Decapitation+IAA | 237.3±21.1 | 43.4±3.7 | |
Decapitation+GA1 | 1227±116.5 | 158.1±12.7 | |
ZS97B | Decapitation+H2O | 61.2±5.7 | 7.1±0.5 |
Decapitation+IAA | 253.0±24.8 | 45.4±3.9 | |
Decapitation+GA1 | 1298.2±101.5 | 164.2±15.2 |
Exogenous 50 μM IAA, 150 μM GA1, respectively, were applied to the excised stumps for 48 h. Control decapitated plants were treated with distilled water. The UI was harvested 48 h after the first application. The data show means ±SE, n=3 for GA1 level, and n=20 for UI elongation rate. FW, fresh weight.
Effects of exogenous applied IAA, GA1 on endogenous GA1 level and on UI elongation rate in the decapitated plants of ZS97A and ZS97B
Line | Treatment | GA1 level (pmol g−1 FW) | UI elongation rate (mm 48 h−1) |
ZS97A | Decapitation+H2O | 55.2±5.3 | 6.8±0.5 |
Decapitation+IAA | 237.3±21.1 | 43.4±3.7 | |
Decapitation+GA1 | 1227±116.5 | 158.1±12.7 | |
ZS97B | Decapitation+H2O | 61.2±5.7 | 7.1±0.5 |
Decapitation+IAA | 253.0±24.8 | 45.4±3.9 | |
Decapitation+GA1 | 1298.2±101.5 | 164.2±15.2 |
Line | Treatment | GA1 level (pmol g−1 FW) | UI elongation rate (mm 48 h−1) |
ZS97A | Decapitation+H2O | 55.2±5.3 | 6.8±0.5 |
Decapitation+IAA | 237.3±21.1 | 43.4±3.7 | |
Decapitation+GA1 | 1227±116.5 | 158.1±12.7 | |
ZS97B | Decapitation+H2O | 61.2±5.7 | 7.1±0.5 |
Decapitation+IAA | 253.0±24.8 | 45.4±3.9 | |
Decapitation+GA1 | 1298.2±101.5 | 164.2±15.2 |
Exogenous 50 μM IAA, 150 μM GA1, respectively, were applied to the excised stumps for 48 h. Control decapitated plants were treated with distilled water. The UI was harvested 48 h after the first application. The data show means ±SE, n=3 for GA1 level, and n=20 for UI elongation rate. FW, fresh weight.
Decapitation eliminated the difference in the level of panicle-derived IAA between ZS97A and ZS97B. In decapitated plants, notable differences in the transcript levels of OsGA3ox2 and OsGA2ox1 were not detected in the UIs between ZS97A and ZS97B (Fig. 4C), and ZS97A and ZS97B had almost the same GA1 level in the UI and the same UI elongation rate (Table 3). When the same amount of exogenous IAA was applied to the decapitated plants, GA1 levels in the UIs of ZS97A and ZS97B were enhanced to the same levels (Table 3), and the UIs of ZS97A and ZS97B also had the same elongation rates (Table 3). This result suggested that the UIs of ZS97A and ZS97B had the same capacity to synthesize GA1, provided that the same level of IAA was present. The UIs of ZS97A and ZS97B exhibited the same sensitivity to GA1, because the same amount of exogenous GA1 led them to have the same elongation rate (Table 3).
Taken together, in intact rice plants, normal IAA levels derived from the panicle is necessary for GA1 biosynthesis by modulating OsGA3ox2 transcript level. As for intact ZS97A plants, the decreased GA1 biosynthesis resulted from the decreased OsGA3ox2 transcript level in ZS97A UI, which is a consequence of the decreased IAA derived from the CMS panicle.
High IAA oxidase activity confers IAA-deficiency in CMS panicle of ZS97A
The IAA oxidase system is able to influence plant growth by regulating the endogenous IAA level (Bryant and Lane, 1979; Pandey and Pathak, 1981; Kochhar et al., 2005). To investigate the cause of IAA-deficiency in CMS panicles of ZS97A, IAA oxidase activity was assayed in this study. This result showed that the level of IAA oxidase activity in CMS panicles of ZS97A was three to four times higher than that in fertile panicles of ZS97B throughout the pollen development process (Fig. 5A), and the same result was found at the heading stage (Fig. 5B). This result suggested that IAA-deficiency in CMS panicles of ZS97A might be caused by high levels of IAA oxidase activity.
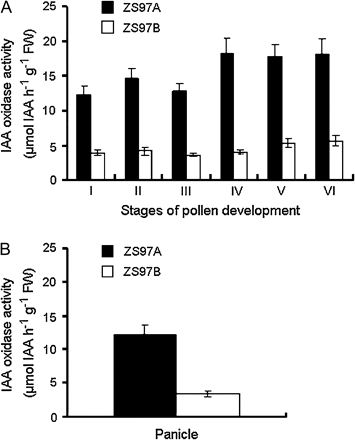
Comparison of IAA oxidase activity in the panicle between ZS97A and ZS97B. (A) IAA oxidase activity in the panicle throughout pollen development process. (I) Stamen and pistil formation stage; (II) Pollen mother cell formation stage; (III) Pollen mother cell meiosis stage; (IV) Uninucleate pollen stage; (V) Binucleate pollen stage; (VI) Trinucleate pollen stage. (B) IAA oxidase activity in the panicle at the heading stage. In (A) and (B), each column represents the mean ±SE of three replicates of independently harvested plant material. FW, fresh weight.
Discussion
CMS line rice shows shortened UI
Since the discovery of the first wild abortive (WA) rice plant in China in 1970, more than 200 WA-type CMS lines, such as ZS97A, Erjiunan 1A, and V20A, have been developed by using WA lines as donors of male sterile genes by successive backcross (Li et al., 1982). After analysing the traits of all the reported WA-type CMS lines, it was found that their pollen grains abort at the uninucleate stage, an early stage during pollen development, and they all show shortened UIs and suffer from serious panicle enclosure (data not shown). Besides WA-type lines, HongLian (HL) and BaoTai (BT) type lines have been developed as CMS lines in China (Huang et al., 2003). Compared with WA-type lines, HL-type lines have a later abortion of pollen grains at the binucleate stage and show slighter panicle enclosure, and BT-type lines have an even later abortion of pollen grains at the trinucleate stage and show little panicle enclosure. However, all UIs of HL- and BT-type lines are shorter than those of their corresponding maintainer lines. Taken together, it is suggested that the final lengths of UIs could be affected by the stage of pollen grains abortion in CMS rice. The earlier the pollen grains abort, the less the UIs elongate, which leads to more serious panicle enclosure.
Role of GA in rice UI elongation
GAs are a large family of tetracyclic, diterpenoid plant hormones that promote various growth and developmental processes in higher plants (Hooley, 1994; Olszewski et al., 2002; Cheng et al., 2004). Previous studies suggested that rice internode elongation is modulated not only by GA level but also GA sensitivity (Ashikari et al., 1999; Spielmeyer et al., 2002; Zhu et al., 2006). In this study, it was found that ZS97A UI suffered from a serious GA1-deficiency, while UIs of ZS97A and ZS97B exhibited the same sensitivity to GA1. These results suggested that shortening of the UI length was caused by GA1-deficiency in the UI.
Rice internode elongation is attributed to cell division in the intercalary meristem followed by cell elongation in the elongation zone (Hoshikawa, 1989b). GA plays important roles in plant cell division and cell elongation (Sauter and Kende, 1992; Yang et al., 1996; Asahina et al., 2002; Ška et al., 1993). Bioactive GA has been demonstrated to promote cell division by regulating cell division cycle (Sauter and Kende, 1992; Knaap et al., 2000), and promote cell elongation by causing transverse orientation of microfibrils and microtubules (Mita and Katsumi, 1986; Sauter et al., 1993; Ška et al., 1993). In this study, these results indicate that reduced GA1 level leads to decreases of both cell number and cell length, resulting in a shortened UI.
Role of IAA in rice UI elongation
Previous evidence indicated that IAA is able to regulate stem elongation (Yang et al., 1993, 1996). Subsequently, IAA was proposed to promote GA biosynthesis (Ross, 1998; Wolbang and Ross, 2001; Wolbang et al., 2004) at the final activation step, from GA20 to GA1 via GA3ox in pea, or the step from GA19 to GA20 via GA20ox in tobacco (Ross et al., 2002).
In this study, it was found that, in intact rice, IAA derived from the developing panicle was necessary for UI elongation by promoting GA1 biosynthesis via the up-regulation of the OsGA3ox2 transcript level. It also showed that the UIs of ZS97A and ZS97B had the same capacity to synthesize GA1 in the UI strongly, provided that the same level of IAA was present. These results led to the suggestion that GA1 in the elongating UI is synthesized mainly by UI itself in intact rice plant, and that GA1-deficiency in the UI is caused mainly by IAA-deficiency via the down-regulation of OsGA3ox2 transcript level in intact ZS97A plant. This suggestion is consistent with previous reports that the divisional zone (basal region of the elongating internode) is able strongly to synthesize bioactive GA in rice internodes (Kaneko et al., 2003), and that the transported hormone is not a GA but rather IAA (Wolbang et al., 2004). Therefore, it is proposed that IAA promotes the UI elongation by promoting GA1 biosynthesis in intact rice plant.
IAA-deficiency in ZS97A panicle
Plant tissues capable of rapid growth have been found to exhibit low IAA oxidase activity, whereas higher levels of activity were observed in slower growing tissues (Galston and Dalberg, 1954; Bolduc et al., 1970; Darbyshire, 1971). Evidence for an inverse relationship between IAA oxidase activity and endogenous IAA level has been reported (Khan, 2003; Alagarswamy et al., 2004; Parmar and Chanda, 2005). Increased IAA oxidase activity was found in both photosensitive genic and thermosensitive genic male sterile lines in rice, and the increased IAA oxidase activity was accompanied by a decreased IAA level (Shen and Gao, 1992; Alagarswamy et al., 2004).
In this study, it was found that the IAA level in ZS97A panicles was sustained much lower than that of ZS97B throughout both the panicle development and heading stages. Simultaneously, the much higher activity of IAA oxidase was found in ZS97A panicle during the same period. So it is suggested that high IAA oxidase activity might contribute to IAA-deficiency in ZS97A panicle. However, pathways of IAA biosynthesis and conjugation in ZS97A panicle are awaiting further investigation.
Abbreviations
- CMS
cytoplasmic male sterile
- GA1
gibberellin A1
- IAA
indole-3-acetic acid
- TIBA
2,3,5-triiodobenzoic acid
- UI
uppermost internode
- ZS97A
Zhenshan 97A
- ZS97B
Zhenshan 97B
We thank Dr Da Luo (Institute of Plant Physiology and Ecology, Shanghai, China) for technical assistance, and Dr Qingsheng Cai (Nanjing Agricultural University, China) for valuable advice. This work was supported by The National Natural Science Foundation of China (No. 30300216).
Comments