-
PDF
- Split View
-
Views
-
Cite
Cite
Cristobal Uauy, Juan Carlos Brevis, Jorge Dubcovsky, The high grain protein content gene Gpc-B1 accelerates senescence and has pleiotropic effects on protein content in wheat, Journal of Experimental Botany, Volume 57, Issue 11, August 2006, Pages 2785–2794, https://doi.org/10.1093/jxb/erl047
- Share Icon Share
Abstract
High grain protein content (GPC) is a frequent target of wheat breeding programmes because of its positive effect on bread and pasta quality. A wild wheat allele at the Gpc-B1 locus with a significant impact on this trait was identified previously. The precise mapping of several senescence-related traits in a set of tetraploid recombinant substitution lines (RSLs) segregating for Gpc-B1 is reported here. Flag leaf chlorophyll degradation, change in peduncle colour, and spike water content were completely linked to the Gpc-B1 locus and to the differences in GPC within a 0.3 cM interval corresponding to a physical distance of only 250 kb. The effect of Gpc-B1 was also examined in different environments and genetic backgrounds using a set of tetraploid and hexaploid pairs of isogenic lines. The results were consistent with those observed in the RSLs. The high GPC allele conferred a shorter duration of grain fill due to earlier flag leaf senescence and increased GPC in all four genetic backgrounds. The effect on grain size was more variable, depending on the genotype–environment combinations. These results are consistent with a model in which the wild-type allele of Gpc-B1 accelerates senescence in flag leaves producing pleiotropic effects on nitrogen remobilization, total GPC, and grain size.
Abbreviations
- BAC
bacterial artificial chromosome
- DAA
days after anthesis
- DIC
wild emmer wheat (Triticum turgidum ssp. dicoccoides) accession FA15-3
- GPC
grain protein content
- LDN
tetraploid wheat cultivar Langdon
- N
nitrogen
- QTL
quantitative trait locus
- RSL
recombinant substitution line
- SEM
standard error of the mean
- TKW
thousand kernel weight
- UCD
University of California at Davis
Introduction
Grain protein content (GPC) is an important determinant of bread and pasta quality and is a frequent target of hard common and durum wheat breeding programmes. Despite its significance, efforts to increase GPC have been hindered by a high environmental effect and a complex genetic system governing this trait (Simmonds, 1995). More recently, quantitative trait loci (QTLs) studies have been used to start dissecting the different loci governing this complex trait and to provide selection tools for breeders (Blanco et al., 1996; Joppa et al., 1997; Prasad et al., 1999; Börner et al., 2002). Of particular interest is the Gpc-B1 gene mapped on chromosome arm 6BS (Joppa et al., 1997; Olmos et al., 2003) which has shown consistent GPC increases (on average 14 g kg−1) in both tetraploid and hexaploid wheat (Mesfin et al., 1999; Chee et al., 2001) as well as across diverse environments (Joppa et al., 1997; Olmos et al., 2003).
The Gpc-B1 allele for high GPC was originally identified in wild emmer wheat Triticum turgidum ssp. dicoccoides accession FA15-3 (referred to hereafter as DIC; Avivi, 1978). Joppa and Cantrell (1990) developed substitution lines of the DIC chromosomes in the cultivar ‘Langdon’ (LDN) and showed that a locus for high GPC was present on chromosome 6B. Using a population of recombinant substitution lines (RSLs) from a LDN(DIC 6B)×LDN cross, Joppa et al. (1997) mapped a QTL for GPC on the proximal region of chromosome arm 6BS. Using isogenic recombinant lines and a large number of replications, Olmos et al. (2003) mapped this QTL as a single Mendelian locus (designated Gpc-B1) within a 2.7 cM region. A more precise map was later produced by Distelfeld et al. (2004) using wheat–rice micro-colinearity, which narrowed the Gpc-B1 region to a 0.3 cM interval. A complete physical map spanning a 250 kb region encompassing the Gpc-B1 gene has recently been completed (Distelfeld et al., 2006).
During field experiments aimed to map the Gpc-B1 gene, differences in senescence in the tetraploid RSLs segregating for the Gpc-B1 locus were observed. This was an important observation because senescence, the programmed degradation of cell constituents, makes nutrients available for remobilization to developing seeds (Mae, 2004) and therefore can have a significant impact on GPC. Previous work by Kade et al. (2005) showed increased levels of soluble protein and amino acids in flag leaves at anthesis and increased efficiency in N remobilization in lines carrying the DIC Gpc-B1 allele. Our preliminary observation, together with the results from Kade et al. (2005), suggested that the effect of this locus on GPC could be a pleiotropic effect of the observed differences in senescence.
The first objective of the present study was to validate the observed differences in senescence and to characterize better the effects of these differences in other senescence-related parameters such as chlorophyll degradation and grain water content, as well as on GPC. The second objective was to map these traits precisely, relative to Gpc-B1 in selected tetraploid RSLs carrying critical recombination events flanking the Gpc-B1 region. The third and final objective was to validate these observations in different genetic backgrounds using a set of tetraploid and hexaploid isogenic lines.
Materials and methods
Plant material
The tetraploid RSLs included in this study came from several sources but are all in the same genetic background. RSL 28 and 65 are part of the original mapping population developed by Joppa et al. (1997) and were provided by LR Joppa. RSLs 121, 129, and 135 were generated by Olmos et al. (2003) from the RSL65×LDN cross, while RSL 209 was generated by Distelfeld et al. (2004) from the same cross. A new RSL (RSL 300) was generated by screening 132 additional F2 plants from the original cross by Olmos et al. (2003) and selecting for plants with recombination events between polymerase chain reaction (PCR) markers Xucw79 (completely linked to Xuhw89) and Xucw71 (Distelfeld et al., 2004). The selected F2 RSL was self-pollinated and F3 homozygous recombinant plants were selected and seed increased for replicated field trials. Twelve hundred gametes were screened with flanking molecular markers to select all the recombinant lines used in this study.
Isogenic lines for Gpc-B1 in different tetraploid and hexaploid genetic backgrounds were developed by six backcrosses to their respective recurrent parents followed by two generations of self-pollination to produce BC6F3 homozygous lines (Chicaiza et al., 2006). The Gpc-B1 DIC allele was selected with molecular markers during the different steps of the introgression process (Khan et al., 2000). RSL 65 was the source of Gpc-B1 for the tetraploid cultivars ‘Kofa’ and ‘Kronos’ and the breeding line UC1113 (Chicaiza et al., 2006). Common wheat cultivar ‘Glupro’ (Khan et al., 2000) was the source of Gpc-B1 for the hexaploid hard red spring cultivars ‘Anza’ and ‘RSI5’ and the breeding line UC1041.
Field experiments
Experiments 1 and 2:
Field trials for the tetraploid RSLs were conducted in the experimental field of the University of California, Davis, USA (UCD, Sacramento Valley, 38°32′ N, 121°46′ W). Planting dates for the experiments were 6 November 2003 (experiment 1) and 9 November 2004 (experiment 2). The field conditions and agronomic practices have been described before (Olmos et al., 2003). Both experiments were fertilized with 100 kg ha−1 of N prior to planting. The two experiments were organized in a randomized complete block design with 10 blocks. Experimental units consisted of 1 m row plots, spaced 0.3 m apart, and with adjacent RSL rows separated by a row of dwarf common wheat to avoid mixing. Both experiments consisted of four RSLs (RSL 28, 121, 135, and 209 for experiment 1; RSL 121, 129, 209, and 300 for experiment 2) along with the two parental lines (LDN and RSL 65) included as controls.
Experiments 3 and 4:
Field trials with the isogenic lines were conducted only at UCD in experiment 3, and at three locations (UCD, Kings, and Imperial Valley) in experiment 4. Both experiments were harvested in 2004 and were organized in a split-plot design in five (Anza, Kofa, Kronos, UC1041, and UC1113) or 10 (RSI5) randomized complete blocks. The pairs of isogenic lines were sown such that cultivar or breeding lines were assigned to the main plots, whereas the presence or absence of Gpc-B1 was assigned to the subplots. This design was adopted to maximize the sensitivity of the comparison within the isogenic pairs. For experiment 3 (isogenic pairs of Anza, UC1041, and Kofa) and experiment 4 (isogenic pairs of Kofa, Kronos, and UC1113), the experimental units consisted of 7 m2 plots. Due to seed limitations for the isogenic lines of RSI5 (experiment 3), the large plots were replaced by 1 m rows. This difference in the experimental units invalidates comparisons between varieties but, since only comparisons within each variety were of interest, RSI5 was included as part of experiment 3. These plots were fertilized with 112 kg ha−1 of N prior to planting, while agronomic practices for the three sites for experiment 4 can be found in Table 1 of Jackson et al. (2005).
Days after anthesis | |||||||
13 | 19 | 24 | 26 | 28 | 32 | 35 | |
LDN | 42.09 | 43.17 | 32.74 | 22.70 | 13.75 | 8.23 | 4.22 |
129 | 41.07 | 42.67 | 28.66 | 20.63 | 13.03 | 8.93 | 4.09 |
209 | 43.28 | 43.78 | 32.21 | 22.72 | 13.72 | 7.17 | 3.55 |
65 | 42.35 | 40.75 | 25.01 | 12.60 | 8.41 | 5.25 | 3.95 |
300 | 43.73 | 45.52 | 27.22 | 14.52 | 9.62 | 5.04 | 4.17 |
121 | 41.60 | 42.21 | 24.92 | 11.65 | 7.33 | 4.03 | 3.78 |
Contrast | 0.23 | 0.79 | 0.0056 | 0.0012 | 0.0008 | <0.0001 | 0.82 |
Days after anthesis | |||||||
13 | 19 | 24 | 26 | 28 | 32 | 35 | |
LDN | 42.09 | 43.17 | 32.74 | 22.70 | 13.75 | 8.23 | 4.22 |
129 | 41.07 | 42.67 | 28.66 | 20.63 | 13.03 | 8.93 | 4.09 |
209 | 43.28 | 43.78 | 32.21 | 22.72 | 13.72 | 7.17 | 3.55 |
65 | 42.35 | 40.75 | 25.01 | 12.60 | 8.41 | 5.25 | 3.95 |
300 | 43.73 | 45.52 | 27.22 | 14.52 | 9.62 | 5.04 | 4.17 |
121 | 41.60 | 42.21 | 24.92 | 11.65 | 7.33 | 4.03 | 3.78 |
Contrast | 0.23 | 0.79 | 0.0056 | 0.0012 | 0.0008 | <0.0001 | 0.82 |
Contrasts compared the three lines with the LDN allele at the Gpc-B1 locus (LDN, 129, and 209) with the three lines with the DIC allele at the Gpc-B1 locus (65, 300, and 121). Significant contrasts are presented in bold.
Days after anthesis | |||||||
13 | 19 | 24 | 26 | 28 | 32 | 35 | |
LDN | 42.09 | 43.17 | 32.74 | 22.70 | 13.75 | 8.23 | 4.22 |
129 | 41.07 | 42.67 | 28.66 | 20.63 | 13.03 | 8.93 | 4.09 |
209 | 43.28 | 43.78 | 32.21 | 22.72 | 13.72 | 7.17 | 3.55 |
65 | 42.35 | 40.75 | 25.01 | 12.60 | 8.41 | 5.25 | 3.95 |
300 | 43.73 | 45.52 | 27.22 | 14.52 | 9.62 | 5.04 | 4.17 |
121 | 41.60 | 42.21 | 24.92 | 11.65 | 7.33 | 4.03 | 3.78 |
Contrast | 0.23 | 0.79 | 0.0056 | 0.0012 | 0.0008 | <0.0001 | 0.82 |
Days after anthesis | |||||||
13 | 19 | 24 | 26 | 28 | 32 | 35 | |
LDN | 42.09 | 43.17 | 32.74 | 22.70 | 13.75 | 8.23 | 4.22 |
129 | 41.07 | 42.67 | 28.66 | 20.63 | 13.03 | 8.93 | 4.09 |
209 | 43.28 | 43.78 | 32.21 | 22.72 | 13.72 | 7.17 | 3.55 |
65 | 42.35 | 40.75 | 25.01 | 12.60 | 8.41 | 5.25 | 3.95 |
300 | 43.73 | 45.52 | 27.22 | 14.52 | 9.62 | 5.04 | 4.17 |
121 | 41.60 | 42.21 | 24.92 | 11.65 | 7.33 | 4.03 | 3.78 |
Contrast | 0.23 | 0.79 | 0.0056 | 0.0012 | 0.0008 | <0.0001 | 0.82 |
Contrasts compared the three lines with the LDN allele at the Gpc-B1 locus (LDN, 129, and 209) with the three lines with the DIC allele at the Gpc-B1 locus (65, 300, and 121). Significant contrasts are presented in bold.
In experiments 1, 2, and 3, stripe rust (Puccinia striiformis) was controlled by applying propiconazole [120 g ha−1 active ingredient (a.i.) at Feekes stage 10 (Large, 1954)] and azoxystrobin (180 g ha−1 a.i. at Feekes stage 10.5) using a a CO2 backpack sprayer model D (R & D Sprayers, Opelousas, LA, USA) with 2.8 bar of pressure. Plots in experiment 4 were not sprayed, but weekly visual examinations recorded no significant presence of stripe rust at the three sites.
Determination of relative chlorophyll content
Experiment 2:
Anthesis occurred on 29 April 2005 for all six lines, and 15 main spikes were tagged per plot on this date. The corresponding flag leaf of each spike was later used to measure chlorophyll content in a non-destructive manner using a hand-held chlorophyll meter (SPAD-502, Minolta, UK). For each sampling date [13, 19, 24, 26, 28, 32, and 35 d after anthesis (DAA)], the value of each replication consisted of the average readings of each of the 15 flag leaves, which in turn consisted of the average of 10 sampling positions per flag leaf. This large number of subsamples allowed us to reduce experimental variation.
Experiment 3:
Anthesis was recorded and was similar for each of the two lines within each isogenic pair (Anza, 11 April; RSI5, 3 April; UC1041, 31 March; and Kofa, 2 April). Fifteen individual spikes were tagged per plot at anthesis and the average chlorophyll content was determined in a manner similar to experiment 2. All chlorophyll content measurements are given in relative SPAD units.
Determination of peduncle colour and spike or grain water content
In experiment 1, anthesis occurred simultaneously for all six lines included in the trial (28 April 2004). At 30 DAA, six main spikes per plot were collected and sealed in plastic bags. The fresh weight was determined and then the samples were dried to constant weight at 60 °C. The percentage water content was determined as the difference between the fresh and dry weight (total water content) divided by the fresh weight. The percentage of yellow peduncles was determined at 30 DAA by counting the number of peduncles that were completely yellow from a random sample of 10 peduncles per plot.
In experiment 3, 50 spikes per plot were tagged and numbered at anthesis. Sampling was performed on a 2–5 d basis according to the maturity of the plot and the temperature conditions. For each sampling date, five random spikes per plot were collected, sealed in plastic bags, and kept under constant temperature inside a cooler. From each spike, the fresh weight of 10 grains from the central spikelets was determined. The 50 grains from each plot were then dried to constant weight at 60 °C and grain moisture content was calculated.
Nitrogen determinations
For experiments 1 and 2, nitrogen determinations were performed according to Olmos et al. (2003). For experiments 3 and 4, a sample of 600 g of grain was used to determine protein using an Infratec 1241 near infra-red grain analyser (Foss Tecator AB, Högänas, Sweden).
Determination of thousand kernel weight (TKW)
For experiments 3 and 4, 1000 grains from each plot were counted using an electronic seed counter Model 2500 (The Old Mill Company, Savage, MD, USA). These samples were manually cleaned to discard broken grains and impurities in order to ensure that only whole kernels were in the sample. The 1000 grains were dried to constant weight and this value is reported throughout the text.
Statistical analysis
Analyses of variance were performed using the SAS Version 9.1 program (SAS Institute, 2003). The general linear model (PROC GLM) was used to assess the effect of the DIC Gpc-B1 allele in the tetraploid RSLs and the DIC segment in the isogenic lines. In order to meet the assumptions of the model, data were transformed when necessary using logarithmic and power transformations. For protein content, lines were classified as high and low protein at the end of the experiment by comparison with low and high GPC controls as described before (Olmos et al., 2003). The same analysis was performed for spike water content, peduncle colour, and chlorophyll degradation to classify the RSLs with recombination events flanking Gpc-B1 into early and late senescing lines. Briefly, a line was classified only if it was significantly different from one control line and not significantly different from the other. For the time courses of chlorophyll degradation and grain maturity in the isogenic lines, each time point was analysed separately.
Results
Effect of Gpc-B1 on senescence in tetraploid RSLs
Experiment 1:
In this experiment, RSLs 28 and 121 had significantly higher GPC than the low GPC control LDN (P <0.001) and were not significantly different from high GPC control RSL 65 (P >0.9) (Fig. 1a). Based on these results, these lines were classified as high GPC (DIC allele) for the Gpc-B1 locus, a similar result to that obtained by Olmos et al. (2003). The other two lines, RSL 135 and RSL 209, showed the opposite profile. They had significantly lower GPC than the high GPC control RSL 65 (P <0.01) and were not significantly different from low GPC control LDN (P >0.65). These lines were classified as low GPC (LDN allele) for the Gpc-B1 locus. The average GPC increase in the lines with the DIC allele at the Gpc-B1 locus relative to the lines with the LDN allele was 19 g kg−1 (P <0.001). These results confirmed that the experimental conditions of experiment 1 were appropriate to express the differences between the Gpc-B1 alleles (Fig. 1a).
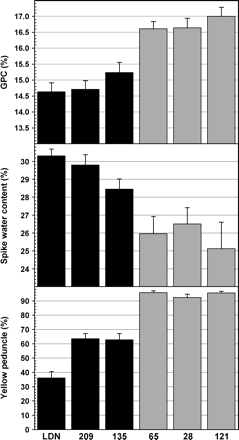
Experiment 1. (a) Grain protein content in RSLs (209, 135, 28, and 121) and control lines (LDN and RSL 65). RSLs 209 and 135 were similar to the low protein control LDN and were assigned the Gpc-B1 low protein allele (black bars), whereas RSLs 28 and 121 were similar to the high protein control RSL 65 and were assigned the Gpc-B1 high protein allele (grey bars). (b) Percentage of water content in 10 spikes of RSLs and controls at 30 DAA. (c) Percentage of yellow peduncles of RSLs at 30 DAA. Error bars are SEMs.
A few days after the first visual symptoms of senescence (flag leaf yellowing), 10 rows (replications) from each RSL were evaluated for the percentage of yellow peduncles and water content in the spikes (Fig. 1b, c). The analysis of variance for these two traits showed that RSLs with the DIC allele at Gpc-B1 had a significantly higher (P <0.001) percentage of yellow peduncles (95%) and lower water content in the spikes (26%) than RSLs with the Gpc-B1 LDN allele (54% yellow peduncles, 30% water content). No significant differences were detected in ear emergence or time of anthesis between the two alleles, indicating that the observed differences were the results of differences in senescence or maturity rates.
RSLs 28 and 121 are of particular interest since the closest flanking crossovers in these lines delimit a region of 250 kb (0.3 cM), between markers Xuhw89 and Xucw71, including the Gpc-B1 gene (Fig. 2; Distelfeld et al., 2006). These two lines were not significantly different from the high protein control RSL 65 for protein content (P >0.05), percentage of yellow peduncles (P >0.05), and water content in the spikes (P >0.05), but differ significantly in these three parameters from the low protein control LDN (P <0.001 for both RSLs). Based on these results, it was concluded that the locus controlling differences in peduncle colour and spike water content was completely linked to the 250 kb region including the Gpc-B1 gene (Fig. 2).
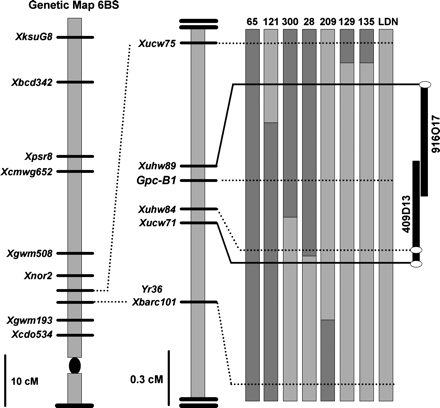
Genetic and physical maps of the Gpc-B1 region and graphical genotypes of RSLs used in experiments 1 and 2. The genetic map on the left shows the position of Gpc-B1 and Yr36 on chromosome arm 6BS, with a detailed high-density map of the targeted region to the right. The central figure represents the graphical genotypes of the RSLs used in this study. Bars represent chromosome arms, with dark grey bars representing DIC markers and light grey bars representing LDN markers. Recombination events are represented by a change between dark and light grey bars. The figure on the right represents the 250 kb physical map of the Gpc-B1 region (Distelfeld et al., 2006).
Experiment 2:
The second experiment included the new recombination event discovered in RSL 300, which further delimited the Gpc-B1 region to the Xuhw89–Xuhw84 interval (0.2 cM, Fig. 2). RSLs 121 and 300 showed significantly higher GPC than the low GPC control LDN (P <0.05) and were not significantly different from the high GPC control RSL 65 (P >0.70). On the other hand, RSLs 129 and 209 showed significantly lower GPC than the high GPC control RSL 65 (P <0.01) but were not significantly different from the low GPC control LDN (P >0.99). Based on these results, RSLs 121 and 300 were assigned the Gpc-B1 DIC allele and RSLs 129 and 209 were assigned the LDN allele for this locus. The average GPC increase in the lines with the DIC allele relative to the lines with the LDN allele at the Gpc-B1 locus was 12 g kg−1 (P <0.001, Fig. 3a), confirming that the conditions of experiment 2 were appropriate for the expression of the differences attributable to the Gpc-B1 locus.
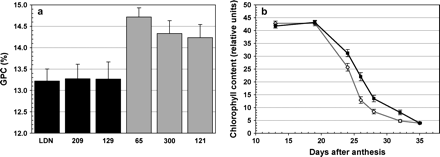
Experiment 2. (a) Grain protein content of RSLs (209, 129, 300, and 121) and control lines (LDN and 65). RSLs 209 and 129 were similar to the low protein control LDN and were assigned the Gpc-B1 low protein allele (black bars), whereas RSLs 300 and 121 were similar to the high protein control RSL 65 and were assigned the Gpc-B1 high protein allele (grey bars). (b) Chlorophyll content profile (SPAD units) from 13 to 35 DAA. The grey line represents lines with the DIC Gpc-B1 allele (control line 65 and RSLs 300 and 121) and the black line represents lines with the LDN Gpc-B1 allele (LDN and RSLs 209 and 129). Error bars are SEMs.
To characterize better the senescence process in these lines, a time-course of chlorophyll content in the flag leaves of plants grown under field conditions was established (Fig. 3b). Plants with the DIC allele at Gpc-B1 underwent earlier chlorophyll degradation than plants with the LDN allele, starting 19 DAA. Significant differences in flag leaf chlorophyll content were detected in the measurements performed at 24 DAA (P <0.006), 26 DAA (P <0.002), 28 DAA (P <0.001), and 32 DAA (P <0.001) (Table 1). Measurements for both alleles converged when the flag leaves were completely yellow (35 DAA). As in the previous experiment, no differences were detected between RSLs and controls for time of anthesis.
The recombination events in RSLs 121 and 300 used in experiment 2 further delimited the candidate region for the Gpc-B1 locus to a region within the 250 kb of the physical map and excluded Xuhw84 as a candidate gene (Fig. 2). Contrasts were used to classify the flag leaf chlorophyll degradation profile of these two lines as similar to either LDN (late senescence) or RSL 65 (early senescence) (Table 1). In RSL 121, significant differences were detected with respect to LDN for 24 (P=0.02), 26 (P=0.03), 28 (P=0.003) and 32 DAA (P=0.003), while no significant differences were detected between RSL 121 and early senescing parent RSL 65 at these sampling dates (P >0.45 for all four dates). In RSL 300, significant differences were detected 24 (P=0.04), 26 (P=0.047), and 32 DAA (P=0.01) with respect to LDN, while no significant differences were found with RSL65 at any time point (P >0.65 for all dates). Therefore, these two RSLs were classified as early senescing lines (similar to RSL 65), and it was concluded that both the locus controlling the differences in senescence and the locus controlling GPC were completely linked within the region delimited by flanking markers Xuhw84 and Xuhw89. Based on the sizes of the bacterial artificial chromosomes (BACs) 409D13 and 916O17, this region is less than 250 kb long.
The two other RSLs used in this experiment (129 and 209) were classified as late senescing lines similar to LDN (Table 1). These lines share the LDN genotype within the complete 250 kb region (Fig. 2), thereby supporting the proposed map position of the locus responsible for the differences in chlorophyll degradation.
Effect of Gpc-B1 on senescence in tetraploid and hexaploid isogenic lines
Experiment 3:
The effect of Gpc-B1 and the senescence locus in different genetic backgrounds was tested in three hexaploid (Anza, RSI5, and UC1041) and one tetraploid (Kofa) pairs of isogenic lines (BC6F3) (Table 2). The presence of the Gpc-B1 DIC allele significantly increased GPC (P <0.01) in the three hexaploid isogenic lines (Anza, RSI5, and UC1041) with respect to the recurrent parent by an average of 12.1 g kg−1. The tetraploid line Kofa carrying the DIC allele showed a smaller increase of GPC (3.3 g kg−1, P=0.26) relative to the recurrent parent (a high protein content variety), and the differences were not significant in this experiment (Table 2).
Effect of Gpc-B1 on GPC, TKW, and total protein in isogenic lines of Anza, RSI5, UC1041, and Kofa (experiment 3)
Line | Allele at Gpc-B1 | GPC (%) | TKW (g) | Total protein (g/1000 seeds) | ||||||
Mean | Δ (%) | P-value | Mean | Δ (%) | P-value | Mean | Δ (%) | P-value | ||
Anza | Anza | 11.74 | 4.98 | <0.01 | 40.85 | −0.06 | 0.98 | 4.79 | 4.92 | 0.02 |
DIC | 12.32 | 40.82 | 5.03 | |||||||
RSI 5 | RSI 5 | 13.44 | 15.77 | <0.01 | 57.02 | −4.29 | 0.04 | 7.66 | 10.81 | <0.01 |
DIC | 15.56 | 54.58 | 8.49 | |||||||
UC1041 | UC1041 | 13.30 | 6.93 | <0.01 | 44.14 | −13.02 | <0.01 | 5.87 | −7.01 | 0.03 |
DIC | 14.22 | 38.40 | 5.46 | |||||||
Kofa | Kofa | 13.96 | 2.39 | 0.26 | 58.01 | −3.59 | 0.10 | 8.10 | −1.25 | 0.47 |
DIC | 14.30 | 55.93 | 8.00 |
Line | Allele at Gpc-B1 | GPC (%) | TKW (g) | Total protein (g/1000 seeds) | ||||||
Mean | Δ (%) | P-value | Mean | Δ (%) | P-value | Mean | Δ (%) | P-value | ||
Anza | Anza | 11.74 | 4.98 | <0.01 | 40.85 | −0.06 | 0.98 | 4.79 | 4.92 | 0.02 |
DIC | 12.32 | 40.82 | 5.03 | |||||||
RSI 5 | RSI 5 | 13.44 | 15.77 | <0.01 | 57.02 | −4.29 | 0.04 | 7.66 | 10.81 | <0.01 |
DIC | 15.56 | 54.58 | 8.49 | |||||||
UC1041 | UC1041 | 13.30 | 6.93 | <0.01 | 44.14 | −13.02 | <0.01 | 5.87 | −7.01 | 0.03 |
DIC | 14.22 | 38.40 | 5.46 | |||||||
Kofa | Kofa | 13.96 | 2.39 | 0.26 | 58.01 | −3.59 | 0.10 | 8.10 | −1.25 | 0.47 |
DIC | 14.30 | 55.93 | 8.00 |
Differences between the means of the isogenic lines (Δ%) are reported in terms of change with respect to the isogenic line carrying the DIC Gpc-B1 allele. Significant values are presented in bold.
Effect of Gpc-B1 on GPC, TKW, and total protein in isogenic lines of Anza, RSI5, UC1041, and Kofa (experiment 3)
Line | Allele at Gpc-B1 | GPC (%) | TKW (g) | Total protein (g/1000 seeds) | ||||||
Mean | Δ (%) | P-value | Mean | Δ (%) | P-value | Mean | Δ (%) | P-value | ||
Anza | Anza | 11.74 | 4.98 | <0.01 | 40.85 | −0.06 | 0.98 | 4.79 | 4.92 | 0.02 |
DIC | 12.32 | 40.82 | 5.03 | |||||||
RSI 5 | RSI 5 | 13.44 | 15.77 | <0.01 | 57.02 | −4.29 | 0.04 | 7.66 | 10.81 | <0.01 |
DIC | 15.56 | 54.58 | 8.49 | |||||||
UC1041 | UC1041 | 13.30 | 6.93 | <0.01 | 44.14 | −13.02 | <0.01 | 5.87 | −7.01 | 0.03 |
DIC | 14.22 | 38.40 | 5.46 | |||||||
Kofa | Kofa | 13.96 | 2.39 | 0.26 | 58.01 | −3.59 | 0.10 | 8.10 | −1.25 | 0.47 |
DIC | 14.30 | 55.93 | 8.00 |
Line | Allele at Gpc-B1 | GPC (%) | TKW (g) | Total protein (g/1000 seeds) | ||||||
Mean | Δ (%) | P-value | Mean | Δ (%) | P-value | Mean | Δ (%) | P-value | ||
Anza | Anza | 11.74 | 4.98 | <0.01 | 40.85 | −0.06 | 0.98 | 4.79 | 4.92 | 0.02 |
DIC | 12.32 | 40.82 | 5.03 | |||||||
RSI 5 | RSI 5 | 13.44 | 15.77 | <0.01 | 57.02 | −4.29 | 0.04 | 7.66 | 10.81 | <0.01 |
DIC | 15.56 | 54.58 | 8.49 | |||||||
UC1041 | UC1041 | 13.30 | 6.93 | <0.01 | 44.14 | −13.02 | <0.01 | 5.87 | −7.01 | 0.03 |
DIC | 14.22 | 38.40 | 5.46 | |||||||
Kofa | Kofa | 13.96 | 2.39 | 0.26 | 58.01 | −3.59 | 0.10 | 8.10 | −1.25 | 0.47 |
DIC | 14.30 | 55.93 | 8.00 |
Differences between the means of the isogenic lines (Δ%) are reported in terms of change with respect to the isogenic line carrying the DIC Gpc-B1 allele. Significant values are presented in bold.
In all four genetic backgrounds, the chlorophyll degradation time-course (Fig. 4) showed more rapid chlorophyll degradation in the lines with the DIC allele than in the isogenic lines with the recurrent parental allele. These differences did not originate in flowering time variability since the lines for each isogenic pair reached anthesis at the same time. During the first 20–30 DAA, no significant differences in chlorophyll content were detected in the flag leaves, but after that, chlorophyll degradation was faster in the isogenic lines carrying the DIC chromosome segment (Fig. 4, grey lines) compared with the original recurrent parent (Fig. 4, black lines). The differences were similar across genotypes, with the isogenic lines carrying the DIC segment being on average 3–4 d more advanced in their senescence process than the corresponding recurrent parent. The significant differences in chlorophyll content (P <0.05, indicated by arrows in Fig. 4) were maintained until the flag leaves of the isogenic pairs approached complete yellowing.
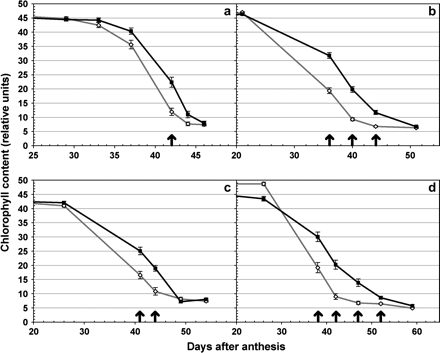
Experiment 3: chlorophyll content. Effect of the DIC Gpc-B1 allele on the chlorophyll content profile of isogenic lines of hexaploid wheat Anza (a), RSI5 (b), and UC1041 (c), and tetraploid wheat Kofa (d). Chlorophyll content is measured in relative SPAD units from 20–25 DAA until complete yellowing (50–60 DAA). Black lines with filled squares represent the original recurrent parent, and grey lines with open diamonds represent the corresponding isogenic line with the DIC Gpc-B1 allele. Significant differences (P <0.05) between isogenic pairs are represented by arrows on the corresponding date. Error bars are SEMs.
In addition to chlorophyll degradation, the progression of grain moisture content was also measured, and it was observed that the isogenic lines with the DIC chromosome segment presented significantly lower grain moisture content than the isogenic lines without the DIC segment (P <0.05, Fig. 5). Although isogenic lines of UC1041 were not included in Fig. 5 because they were sampled at only one time point (54 DAA), they also showed significant differences in grain moisture content (P=0.02) between the recurrent UC1041 line (20.2% moisture content) and the UC1041 isogenic line carrying the DIC allele (8.9% moisture content). These results indicate that the more rapid chlorophyll degradation in the isogenic lines carrying the DIC Gpc-B1 allele was associated with a shorter grain-filling period in all four genotypes. This result is consistent with those observed from the tetraploid recombinant substitution lines (experiments 1 and 2).
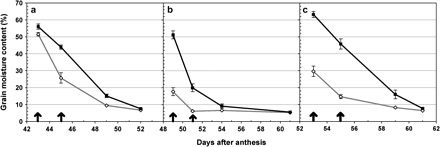
Experiment 3: grain moisture content. Effect of DIC Gpc-B1 on grain moisture content in isogenic lines of hexaploid wheat Anza (a) and RSI5 (b), and tetraploid wheat Kofa (c). Moisture content was measured from 42 to 60 DAA depending on the variety. Black lines with filled squares represent the original recurrent parent, and grey lines with open diamonds represent the corresponding isogenic line with the DIC Gpc-B1 allele. Significant differences (P <0.05) between isogenic pairs are represented by arrows on the corresponding date. Error bars are SEMs.
To test if the differences in the length of the grain-filling period were associated with differences in grain size, thousand kernel weight (TKW) was evaluated in the different pairs of isogenic lines (Table 2). The effect of the DIC segment on TKW and total protein was less consistent and was dependent on the variety. TKW decreased significantly in the RSI5 and UC1041 isogenic lines carrying the DIC Gpc-B1 allele compared with the recurrent parents (P <0.05). The Kofa isogenic line with Gpc-B1 presented a small decrease in TKW, although not significant (P=0.10), while the isogenic lines of Anza had a similar TKW (Table 2).
To analyse the combined effects of the changes in GPC and grain size, the differences in total protein (TKW×GPC) were also tested (Table 2). The Anza and RSI5 isogenic lines with the DIC Gpc-B1 allele showed a significant increase in total protein in 1000 grains relative to their recurrent parents. By contrast, the introgression of Gpc-B1 in UC1041 resulted in a significantly lower amount of total protein (P=0.03) due to the large negative effect that the gene had on grain size. Isogenic lines of Kofa presented no significant differences in total protein (P=0.47).
Experiment 4:
This last experiment was aimed to test the effect of different environments on GPC, TKW, and total protein (Table 3). The Gpc-B1 DIC allele increased GPC by an average of 10.8 g kg−1 (7.75%) with respect to the recurrent parent alleles. Although the presence of the DIC allele was associated with an increase in GPC in all the genotype–environment combinations, the results varied in magnitude and were not significant in some of them (Table 3).
Effect of Gpc-B1 on GPC, TKW, and total protein in isogenic lines of Kofa, Kronos, and UC1113 at Davis, Imperial Valley, and Kings (experiment 4)
Line | Location | Allele at Gpc-B1 | GPC (%) | TKW (g) | Total protein (g/1000 seeds) | ||||||
Mean | Δ (%) | P-value | Mean | Δ (%) | P-value | Mean | Δ (%) | P-value | |||
Kofa | Davis | Kofa | 13.99 | 9.72 | 0.02 | 46.7 | 14.51 | <0.01 | 6.53 | 25.6 | <0.01 |
DIC | 15.35 | 53.5 | 8.21 | ||||||||
Imperial | Kofa | 14.76 | 8.64 | <0.01 | 50.6 | −3.46 | 0.35 | 7.46 | 4.90 | 0.31 | |
DIC | 16.04 | 48.8 | 7.83 | ||||||||
Kings | Kofa | 14.72 | 5.03 | 0.05 | 43.1 | 11.14 | <0.01 | 6.34 | 16.8 | <0.01 | |
DIC | 15.46 | 47.9 | 7.41 | ||||||||
Kronos | Davis | Kronos | 15.34 | 5.08 | 0.08 | 57.1 | −2.02 | 0.55 | 8.76 | 2.91 | 0.60 |
DIC | 16.12 | 55.9 | 9.01 | ||||||||
Imperial | Kronos | 15.08 | 5.67 | 0.02 | 50.6 | −9.14 | 0.04 | 7.63 | −4.00 | 0.16 | |
DIC | 15.94 | 46.0 | 7.33 | ||||||||
Kings | Kronos | 15.28 | 3.11 | 0.15 | 43.0 | 5.00 | 0.24 | 6.57 | 8.19 | 0.03 | |
DIC | 15.75 | 45.2 | 7.11 | ||||||||
UC1113 | Davis | UC1113 | 13.59 | 5.45 | 0.07 | 49.0 | −0.05 | 0.99 | 6.65 | 5.40 | 0.28 |
DIC | 14.33 | 48.9 | 7.01 | ||||||||
Imperial | UC1113 | 13.21 | 11.79 | 0.04 | 48.6 | −9.77 | 0.07 | 6.43 | 0.92 | 0.91 | |
DIC | 14.77 | 43.9 | 6.48 | ||||||||
Kings | UC1113 | 12.85 | 15.27 | 0.02 | 41.7 | −3.78 | 0.60 | 5.36 | 10.85 | 0.25 | |
DIC | 14.82 | 40.1 | 5.94 |
Line | Location | Allele at Gpc-B1 | GPC (%) | TKW (g) | Total protein (g/1000 seeds) | ||||||
Mean | Δ (%) | P-value | Mean | Δ (%) | P-value | Mean | Δ (%) | P-value | |||
Kofa | Davis | Kofa | 13.99 | 9.72 | 0.02 | 46.7 | 14.51 | <0.01 | 6.53 | 25.6 | <0.01 |
DIC | 15.35 | 53.5 | 8.21 | ||||||||
Imperial | Kofa | 14.76 | 8.64 | <0.01 | 50.6 | −3.46 | 0.35 | 7.46 | 4.90 | 0.31 | |
DIC | 16.04 | 48.8 | 7.83 | ||||||||
Kings | Kofa | 14.72 | 5.03 | 0.05 | 43.1 | 11.14 | <0.01 | 6.34 | 16.8 | <0.01 | |
DIC | 15.46 | 47.9 | 7.41 | ||||||||
Kronos | Davis | Kronos | 15.34 | 5.08 | 0.08 | 57.1 | −2.02 | 0.55 | 8.76 | 2.91 | 0.60 |
DIC | 16.12 | 55.9 | 9.01 | ||||||||
Imperial | Kronos | 15.08 | 5.67 | 0.02 | 50.6 | −9.14 | 0.04 | 7.63 | −4.00 | 0.16 | |
DIC | 15.94 | 46.0 | 7.33 | ||||||||
Kings | Kronos | 15.28 | 3.11 | 0.15 | 43.0 | 5.00 | 0.24 | 6.57 | 8.19 | 0.03 | |
DIC | 15.75 | 45.2 | 7.11 | ||||||||
UC1113 | Davis | UC1113 | 13.59 | 5.45 | 0.07 | 49.0 | −0.05 | 0.99 | 6.65 | 5.40 | 0.28 |
DIC | 14.33 | 48.9 | 7.01 | ||||||||
Imperial | UC1113 | 13.21 | 11.79 | 0.04 | 48.6 | −9.77 | 0.07 | 6.43 | 0.92 | 0.91 | |
DIC | 14.77 | 43.9 | 6.48 | ||||||||
Kings | UC1113 | 12.85 | 15.27 | 0.02 | 41.7 | −3.78 | 0.60 | 5.36 | 10.85 | 0.25 | |
DIC | 14.82 | 40.1 | 5.94 |
Differences between the means of the isogenic lines (Δ%) are reported in terms of change with respect to the isogenic line carrying the DIC Gpc-B1 allele. Significant values are presented in bold.
Effect of Gpc-B1 on GPC, TKW, and total protein in isogenic lines of Kofa, Kronos, and UC1113 at Davis, Imperial Valley, and Kings (experiment 4)
Line | Location | Allele at Gpc-B1 | GPC (%) | TKW (g) | Total protein (g/1000 seeds) | ||||||
Mean | Δ (%) | P-value | Mean | Δ (%) | P-value | Mean | Δ (%) | P-value | |||
Kofa | Davis | Kofa | 13.99 | 9.72 | 0.02 | 46.7 | 14.51 | <0.01 | 6.53 | 25.6 | <0.01 |
DIC | 15.35 | 53.5 | 8.21 | ||||||||
Imperial | Kofa | 14.76 | 8.64 | <0.01 | 50.6 | −3.46 | 0.35 | 7.46 | 4.90 | 0.31 | |
DIC | 16.04 | 48.8 | 7.83 | ||||||||
Kings | Kofa | 14.72 | 5.03 | 0.05 | 43.1 | 11.14 | <0.01 | 6.34 | 16.8 | <0.01 | |
DIC | 15.46 | 47.9 | 7.41 | ||||||||
Kronos | Davis | Kronos | 15.34 | 5.08 | 0.08 | 57.1 | −2.02 | 0.55 | 8.76 | 2.91 | 0.60 |
DIC | 16.12 | 55.9 | 9.01 | ||||||||
Imperial | Kronos | 15.08 | 5.67 | 0.02 | 50.6 | −9.14 | 0.04 | 7.63 | −4.00 | 0.16 | |
DIC | 15.94 | 46.0 | 7.33 | ||||||||
Kings | Kronos | 15.28 | 3.11 | 0.15 | 43.0 | 5.00 | 0.24 | 6.57 | 8.19 | 0.03 | |
DIC | 15.75 | 45.2 | 7.11 | ||||||||
UC1113 | Davis | UC1113 | 13.59 | 5.45 | 0.07 | 49.0 | −0.05 | 0.99 | 6.65 | 5.40 | 0.28 |
DIC | 14.33 | 48.9 | 7.01 | ||||||||
Imperial | UC1113 | 13.21 | 11.79 | 0.04 | 48.6 | −9.77 | 0.07 | 6.43 | 0.92 | 0.91 | |
DIC | 14.77 | 43.9 | 6.48 | ||||||||
Kings | UC1113 | 12.85 | 15.27 | 0.02 | 41.7 | −3.78 | 0.60 | 5.36 | 10.85 | 0.25 | |
DIC | 14.82 | 40.1 | 5.94 |
Line | Location | Allele at Gpc-B1 | GPC (%) | TKW (g) | Total protein (g/1000 seeds) | ||||||
Mean | Δ (%) | P-value | Mean | Δ (%) | P-value | Mean | Δ (%) | P-value | |||
Kofa | Davis | Kofa | 13.99 | 9.72 | 0.02 | 46.7 | 14.51 | <0.01 | 6.53 | 25.6 | <0.01 |
DIC | 15.35 | 53.5 | 8.21 | ||||||||
Imperial | Kofa | 14.76 | 8.64 | <0.01 | 50.6 | −3.46 | 0.35 | 7.46 | 4.90 | 0.31 | |
DIC | 16.04 | 48.8 | 7.83 | ||||||||
Kings | Kofa | 14.72 | 5.03 | 0.05 | 43.1 | 11.14 | <0.01 | 6.34 | 16.8 | <0.01 | |
DIC | 15.46 | 47.9 | 7.41 | ||||||||
Kronos | Davis | Kronos | 15.34 | 5.08 | 0.08 | 57.1 | −2.02 | 0.55 | 8.76 | 2.91 | 0.60 |
DIC | 16.12 | 55.9 | 9.01 | ||||||||
Imperial | Kronos | 15.08 | 5.67 | 0.02 | 50.6 | −9.14 | 0.04 | 7.63 | −4.00 | 0.16 | |
DIC | 15.94 | 46.0 | 7.33 | ||||||||
Kings | Kronos | 15.28 | 3.11 | 0.15 | 43.0 | 5.00 | 0.24 | 6.57 | 8.19 | 0.03 | |
DIC | 15.75 | 45.2 | 7.11 | ||||||||
UC1113 | Davis | UC1113 | 13.59 | 5.45 | 0.07 | 49.0 | −0.05 | 0.99 | 6.65 | 5.40 | 0.28 |
DIC | 14.33 | 48.9 | 7.01 | ||||||||
Imperial | UC1113 | 13.21 | 11.79 | 0.04 | 48.6 | −9.77 | 0.07 | 6.43 | 0.92 | 0.91 | |
DIC | 14.77 | 43.9 | 6.48 | ||||||||
Kings | UC1113 | 12.85 | 15.27 | 0.02 | 41.7 | −3.78 | 0.60 | 5.36 | 10.85 | 0.25 | |
DIC | 14.82 | 40.1 | 5.94 |
Differences between the means of the isogenic lines (Δ%) are reported in terms of change with respect to the isogenic line carrying the DIC Gpc-B1 allele. Significant values are presented in bold.
The effect of the DIC Gpc-B1 allele on TKW was less consistent than its effect on GPC, and varied across genotypes and environments (Table 3). The DIC Gpc-B1 allele had a tendency to decrease grain size in UC1113 across environments (although non-significantly), while it significantly increased TKW at two locations (P <0.01) when present in the Kofa background. Kronos, on the other hand, experienced a significant decrease in TKW in the isogenic line carrying Gpc-B1 at Imperial, while the gene had no significant effect on grain size at Davis and Kings.
Despite the variable effect of Gpc-B1 on TKW, a general tendency for the DIC allele to increase total protein in 1000 grains with respect to the recurrent parent was observed due to the positive effect that this allele has on GPC. The magnitude of the increase in total protein depended on the relative importance of the increase in GPC and the effect of that allele on TKW for each genotype–environment combination. For example, significant increases in GPC and TKW in isogenic lines of Kofa carrying Gpc-B1 at Davis and Kings led to a significant increase in total protein in 1000 grains compared with the recurrent parent at both locations. In contrast, the significant increase in GPC in UC1113 at Imperial (11.79%) was offset by the decrease in TKW (−9.77%) yielding a non-significant effect of the DIC Gpc-B1 allele on total protein (P=0.91). Only three genotype–environment combinations presented significant increases in total protein content.
Discussion
Mapping of senescence-related parameters to Gpc-B1
Gpc-B1 has been shown previously to affect GPC in tetraploid and hexaploid wheat, and recently to influence the remobilization of nitrogen from flag leaves to ears during grain filling (Kade et al., 2005). It was demonstrated here that additional traits, including chlorophyll degradation in the flag leaf, changes in peduncle colour, and grain moisture content, were also associated with the presence of the same DIC segment including the Gpc-B1 locus.
Using RSLs carrying critical recombination events within the Gpc-B1 region, we were able to demonstrate that all these traits map to a region delimited by flanking markers Xuhw89 and Xuhw84, which are 0.2 cM apart within a chromosome region that is less than 250 kb long (Distelfeld et al., 2004, 2006). The same RSLs can be used to demonstrate that the observed differences are not the result of the presence of Yr36, an adult-plant stripe rust resistance gene present in the DIC chromosome segment proximal to Xucw71 (Fig. 2; Uauy et al., 2005). Since it is well known that stripe rust can affect GPC and green flag leaf area (Dimmock and Gooding, 2002), the field experiments were sprayed with a systemic fungicide to minimize the confounding effects of this pathogen. In addition, RSLs 28, 209, and 300 included in experiments 1 and 2 carry recombination events between Gpc-B1 and Yr36, allowing the separation of the effects of these two genes (Fig. 2). In both experiments, the allele present at the Gpc-B1 locus determined the phenotype, whereas Yr36 had no significant effect on chlorophyll degradation (Table 1), peduncle colour, or spike water content (Fig. 1).
Other studies have shown that a major gene affecting GPC in the Triticeae is located in a similar region of the short arms of group 6 homoeologous chromosomes (reviewed in Distelfed et al., 2006). A major QTL for TKW and GPC was identified in this region by Elouafi and Nachit (2004) in a cross between a cultivated durum variety Omrabi 5 (high TKW, low GPC) and a wild accession of T. turgidum ssp. dicoccoides (low TKW, high GPC). The peak of these QTLs was associated with Xgwm518, which was mapped between Xgwm508 and Xgwm193, two markers that flank the Gpc-B1 locus (Olmos et al., 2003). To our knowledge, no QTLs for flag leaf senescence have been identified previously in the Gpc-B1 region. A study published by Verma et al. (2004) identified two QTLs for flag leaf senescence, but both were in different chromosomes (2B and 2D).
Effect of Gpc-B1 on grain size and total grain protein
GPC is a function of the total amount of N remobilized to the grain and the total amount of dry matter accumulated in the seed. Therefore, the increase in GPC in lines carrying the DIC allele could be determined by a decrease in grain size (thereby concentrating a similar amount of protein in a smaller grain) or, alternatively, by a net increase in the total amount of protein in the grain through more efficient N remobilization. The association of the DIC segment with differences in senescence can explain both mechanisms. A shorter duration of the grain-filling period can reduce TKW under favourable environmental conditions for grain filling, but also might result in a more efficient N translocation by initiating the remobilization process earlier (Kade et al., 2005).
Based on the results of experiments 3 and 4, it is proposed that a combination of both mechanisms is responsible for the observed differences. The different genotype by environment combinations would determine the relative importance of the modifications in TKW and N remobilization processes to the final total N content. For example, in environments where the grain is affected by severe environmental stresses by the end of the grain-filling period, a shorter maturity time can help the plant escape from those stressful conditions, resulting in an increase in both TKW and GPC (e.g. Kofa, Table 3). From the results presented for the isogenic lines in different environments (Tables 2, 3), it is fair to conclude that the DIC allele at the Gpc-B1 locus is associated with a consistent increase in GPC across genotypes and environments, but that the final effect on total protein content would depend on the more variable effect of the accelerated senescence on TKW. For the 13 genotype–environment combinations tested in experiments 3 and 4, the total protein was significantly higher in the isogenic lines carrying the DIC allele in five cases and lower in one (Tables 2, 3).
Gpc-B1 plays a role in flag leaf senescence and nitrogen remobilization
In contrast to the progress in the genetic and physical mapping of this gene, there is limited knowledge regarding the physiological basis of the effect of the Gpc-B1 locus on GPC. Higher GPC contents can be accomplished by an increase in total N uptake by the plant and/or a more efficient remobilization of N to the grain. Deckard et al. (1996) identified significant contributions of the complete 6B chromosome from DIC to both effects, but the differences in N uptake were significant in only one of the three LDN(DIC6B) lines analysed, and were the result of a lower total dry weight rather than a higher total N. Kade et al. (2005) defined the Gpc-B1 effect on N uptake and remobilization more precisely by using RSL 68 (similar to RSL 65 used in this study), rather than the complete substitution line as in Deckard et al. (1996). Their work showed that the main effect of Gpc-B1 is a more efficient translocation of N from leaves to ears during grain filling. More importantly, they revealed that the differences between the high and low GPC lines were manifested in the form of higher soluble protein concentration in the flag leaves of RSL 68 compared with LDN as early as anthesis (Kade et al., 2005).
The discovery that the 250 kb region including Gpc-B1 has significant effects on senescence-related parameters provides a possible explanation for the multiple effects associated with this small chromosome region. An effect on the regulation of senescence can explain not only the observed differences in parameters usually associated with this process, such as chlorophyll degradation, spike and grain water content, and changes in peduncle colour, but also those in the amount of soluble protein concentration, nitrogen remobilization, GPC, and TKW.
The accumulation of nitrogen in the grain is likely to be the result of events happening at both the sink (developing grains) and at the source (leaves). However, several studies suggest that source regulation plays a significant role in grain protein accumulation (Barneix and Guitman, 1993; Martre et al., 2003). The increased level of total soluble amino acids in flag leaves at anthesis in the lines carrying the DIC allele provides evidence that Gpc-B1 is active in leaves before grain formation (Kade et al., 2005) and suggests a role for Gpc-B1 at the N source level, rather than at the sink level. The co-localization of several nitrogen metabolism QTLs in barley to the Gpc-B1 homologous locus (data by Yang et al., 2004, re-analysed by Distelfeld et al., 2005) supports this model of Gpc-B1 affecting senescence at the source level and having pleiotropic effects on parameters in the grain, such as GPC and grain size.
Recent molecular studies in Arabidopsis have shown that senescence is driven by transcription factor networks that regulate the timely expression of hundreds of genes (Guo et al., 2004; Lin and Wu, 2004). Members of this regulatory network would be expected to affect several senescence-related parameters simultaneously, similar to the pleiotropic effects documented here for the Gpc-B1 locus. It would be interesting to determine if Gpc-B1 is part of the network involved in the regulation of the senescence cascade. This would not be an unusual result, since half of the cloned and validated plant QTLs have been found to be transcription factors (reviewed in Salvi and Tuberosa, 2005).
At this point, the possibility of multiple genes present within the 250 kb region governing different aspects of the responses observed here cannot be ruled out. However, due to the interrelatedness of the mapped phenotypes, we speculate that it is more likely that these multiple traits are pleiotropic effects of a single gene rather than the result of multiple independent genes. The final isolation and characterization of Gpc-B1 will be essential to test these alternative hypotheses and to understand the genetic mechanisms responsible for the complex quantitative processes involved in senescence, nitrogen remobilization, and grain size in polyploid wheat.
This research was supported by Research Grant no. US-3573-04C from BARD, the United States–Israel Binational Agricultural Research and Development Fund, and equipment grants from Israel Science Foundation (nos 048/99 and 1478/04).
Comments