-
PDF
- Split View
-
Views
-
Cite
Cite
Jun-Young Kim, Jong-Hyung Park, Sun-Min Seo, Jin-Il Park, Hee-Yeon Jeon, Han-Kyul Lee, Ran-Ji Yoo, Yong-Jin Lee, Sang-Keun Woo, Woo-Jong Lee, Chi-Min Choi, Yang-Kyu Choi, Radioprotective effect of newly synthesized toll-like receptor 5 agonist, KMRC011, in mice exposed to total-body irradiation, Journal of Radiation Research, Volume 60, Issue 4, July 2019, Pages 432–441, https://doi.org/10.1093/jrr/rrz024
- Share Icon Share
Abstract
Exposure to ionizing radiation leads to severe damages in radiosensitive organs and induces acute radiation syndrome, including effects on the hematopoietic system and gastrointestinal system. In this study, the radioprotective ability of KMRC011, a novel toll-like receptor 5 (TLR5) agonist, was investigated in C57BL6/N mice exposed to lethal total-body gamma-irradiation. In a 30-day survival study, KMRC011-treated mice had a significantly improved survival rate compared with control after 11 Gy total-body irradiation (TBI), and it was found that the radioprotective activity of KMRC011 depended on its dosage and repeated treatment. In a 5-day short-term study, we demonstrated that KMRC011 treatment stimulated cell proliferation and had an anti-apoptotic effect. Furthermore, KMRC011 increased the expressions of genes related to DNA repair, such as Rad21, Gadd45b, Sod2 and Irg1, in the small intestine of lethally irradiated mice. Interestingly, downregulation of NF-κB p65 in the mouse intestine by KMRC011 treatment was observed. This data indicated that KMRC011 exerted a radioprotective activity partially by regulating NF-κB signaling. Finally, peak expression levels of G-CSF, IL-6, IFN-γ, TNF-α and IP-10 induced by KMRC011 treatment were different depending on the route of administration and type of cytokine. These cytokines could be used as candidate biomarkers for the evaluation of KMRC011 clinical efficacy. Our data indicated that KMRC011 has radioprotective activity in lethally irradiated mice and may be developed as a therapeutic agent for radioprotection.
INTRODUCTION
Radiation is a risk element that can damage and disrupt the function of normal tissue and cells in living organisms. Ionizing radiation is composed of X-rays, γ-radiation, and particle radiation such as alpha particles, beta particles and neutrons [1]. The radiation can be created artificially or naturally. Soil, water and air are sources of natural radiation [2, 3]. Artificial radiation originates from man-made products such as X-rays used to diagnose injury, radiotherapy sources, or even radioactive materials used as a weapon for intentional radiation exposure [4]. Acute radiation syndrome, including effects experienced in the hematopoietic system and the gastrointestinal system, can be induced when the subject is exposed to a high dose of radiation within a relatively short period of time [5]; the intensity of the damage is correlated with the total amount of radiation to which the organism has been exposed. Factors affecting the amount of damage are the total time of the radiation exposure, how the radiation has been delivered throughout the body, and whether only a part of the body or the whole body has been exposed. Damage due to the ionizing radiation is increased by increasing the percentage of the body that has been exposed to it, by increasing the strength of the irradiation, or by increasing the time of exposure [1].
The hematopoietic, cardiovascular, gastrointestinal, and central nervous systems, as well as the gonads and skin are affected significantly by high levels of ionizing radiation. Among these, the hematopoietic and gastrointestinal cells are the most vulnerable to ionizing radiation. Cryptic cells in the small intestine consist of highly proliferative cells and rarely develop into cancer, suggesting the existence of an efficient protective mechanism [6]. Intestinal stem cells do not undergo cell cycle arrest and regeneration, but rather undergo apoptosis [7]. Therefore, radioprotection or radiomitigation of the small intestine is crucial before damages to the cells become irreversible. Many radiation therapies focus on reducing genotoxic adverse side effects on the hematopoietic and gastrointestinal systems by enhancing antioxidants that aid in the protection of tissues [8], as well as cytokines related to tissue regeneration [9].
Entolimod (CBLB502), an agonist of toll-like receptor 5 (TLR5), was shown to have radioprotective effects in mice and rhesus monkeys [10, 11]. Recent experiments have shown that activation of TLRs by intestinal microflora in mice decreased radiation-induced DNA damage by activating the expression of growth arrest and DNA damage–inducible 45 beta (Gadd45b), manganese superoxide dismutase (Sod2), and Rad21 [12]. Immune responsive gene 1 (Irg1) is used as an activation marker of TLR agonists [12]. Sod2 was also found to play an important role in delayed radioprotective effect [13].
Entolimod had an additional heterologous region containing hexa-histidine tag, an enterokinase recognition site, and 34 amino acid residues (MRGSHHHHHHGMASMTGGQQMGRDLYDDDDKDPM) that were helpful in purification of protein. KMRC011 is a novel TLR5 agonist manufactured by the Korea Institute of Industrial Technology. KMRC011 is a partially modified version of entolimod [10] derived from flagellin protein from Salmonella enterica. To make the KMRC011, 34 amino acid residues in entolimod were selectively cut out by ubiquitin using ubiquitin-specific protease (UBP1), because these amino acid residues could cause an immune response [14]. In this study, we evaluated the radioprotective effect of KMRC011 in mice exposed to total-body gamma irradiation (TBI).
MATERIALS AND METHODS
Animals
Seven-week-old male C57BL/6N mice (20–25 g) and ICR mice (33–38 g) were purchased from Orient Bio Inc. (Seongnam, Korea). Mice were then acclimatized at the animal facility at the College of Veterinary Medicine, Konkuk University for 1 week. All mice were caged in a sterilized polycarbonate cage with a stainless steel lid and sterilized wooden bedding. Five mice per cage were fed with autoclaved water and food. All cages were placed in a pathogenically free environment at a temperature of 22 ± 2°C, 50 ± 10% humidity, and a 12-h light/dark cycle.
KMRC011
TLR 5 agonist, KMRC011 was manufactured and provided by the Korea Institute of Industrial Technology (Yeongcheon, Korea). The purity of the KMRC011 was determined as 100% using size-exclusion high-performance liquid chromatography. The quality control was assured by Advanced Protein Technologies Corp. (Suwon, Korea). Thawing of KMRC011 was done at room temperature, and it was then diluted in phosphate-buffered saline (PBS) to a working dilution.
Radiation exposure
TBI was carried out with 60Cobalt γ radiation (GammaBeam 100–80, Best Theratronics, Ottawa, Canada) at the Korea Institute of Radiological and Medical Science. Mice were intraperitoneally anesthetized with a mixture of Zoletil (Virbac Latoratories, Carros, France) and Rumpun (Bayer Korea Ltd, Seoul, Korea) prior to the TBI with 11 Gy at a dose rate of 0.5 Gy/min. Nano-Dot (Landauer Inc, Glenwood, IL); a glass dosimeter was placed alongside the mice during radiation exposure to ensure its radiation dosimetry. All procedures were approved by the Institutional Animal Care and Use Committee (KU15101).
Thirty-day survival study
To investigate whether KMRC011 has radioprotective activity to achieve LD70/30, C57BL/6N mice were intramuscularly treated with KMRC011 (0.01 or 0.05 mg/kg) or PBS at different time points (15 min, 24 h, 48 h or 72 h) after 11 Gy TBI at a dose rate of 0.5 Gy/min. Mice were divided into five groups as follows: mice treated with PBS (PBS treatment at 15 min after TBI, control), mice treated with a high dose of KMRC011 (0.05 mg/kg KMRC011 treatment at 15 min after TBI), mice treated with a single dose of KMRC011 (0.01 mg/kg KMRC011 treatment at 15 min after TBI), mice treated with two doses of KMRC011 (0.01 mg/kg KMRC011 at 15 min and 24 h after TBI) and mice treated with four doses of KMRC011 (0.01 mg/kg KMRC011 at 15 min, 24 h, 48 h and 72 h after TBI). To determine the survival, clinical signs, and death of mice, all mice were visually checked twice daily for 30 days. After 30 days, surviving mice were euthanized in a CO2 gas chamber.
Five-day short-term study
To investigate the radioprotective mechanism of KMRC011, C57BL/6N mice were intramuscularly injected with KMRC011 (0.05 mg/kg) or PBS 15 min after the 11 Gy TBI. The mice were euthanized 1, 3 or 5 days after the TBI. Mice were divided into five groups as follows: control (non-irradiated control mice, PBS treatment), KMRC 1 day (mice were sacrificed on the first day after the TBI and KMRC011 treatment), KMRC 3 days (mice were sacrificed on the third day after the TBI and KMRC011 treatment), KMPC 5 days (mice were sacrificed on the fifth day after the TBI and KMRC011 treatment), PBS 5 days (mice were sacrificed on the fifth day after TBI and PBS treatment). All mice were anesthetized with a mixture of Zoletil and Rumpun, then weighed prior to necropsy. The blood was collected via caudal vena cava and stored in heparinized tubes immediately after the collection at room temperature. The hematological data was measured by an Advia 2120i hematology analyzer (Siemens Healthineers, Erlangen, Germany). The liver and spleen were removed, weighed in sterile conditions, and fixed in 10% neutral-buffered formalin. One part of the jejunum was immersed into liquid nitrogen immediately and stored at –70°C for later use, and the other part of the jejunum was fixed with 10% neutral-buffered formalin. The paraffin blocks were sectioned at a thickness of 5 μm and then subjected to hematoxylin and eosin (H&E) staining, immunohistochemical analysis (IHC) and the TUNEL assay.
Pharmacokinetics of KMRC011
To examine changes in the cytokines in the blood after KMRC011 injection, non-irradiated ICR mice were administered KMRC011 at a dose of 0.2 mg/kg by intramuscular (IM), intraperitoneal (IP) or subcutaneous (SC) injection. Blood was collected via the retro-orbital plexus at 0 (non-injected mice), 1, 2, 4, 8 and 24 h after the KMRC011 injection. The collected blood was stored at 4°C overnight, and the blood serum was separated, aliquoted, and stored at –70°C until analysis.
Immunohistochemical analysis
Numbers of Sod2 and BrdU-positive cells were assessed by immunohistochemistry. To detect BrdU incorporation, mice were intraperitoneally administered with BrdU (Merck, Kenilworth, NJ) at a dose of 2 mg per mouse at 2 h prior to necropsy. Sectioned slides were deparaffinized and rehydrated, and the antigen was retrieved with sodium-citrate buffer (0.01 M, PH 6.0) by heating in a microwave for 10 min and cooling for 30 min at room temperature. The sections were then stained with a rabbit polyclonal antibody against Sod2 (ab13533, Abcam, Cambridge, MA), or a rat polyclonal antibody against BrdU (ab6326, Abcam), and incubated at 4°C overnight. A secondary antibody corresponding to each primary antibody was added to each slide, and the slides were incubated for an hour at room temperature. The slides were then incubated with ABC reagent for 30 min at room temperature. TUNEL staining was performed on the intestine using an In Situ Cell Death Detection Kit (11684795910, Roche, Basel, Switzerland), and numbers of TUNEL-positive cells were assessed according to the manufacturer’s instruction. The sections were stained with DAB, counterstained with hematoxylin, and examined using a ×200 objective on a BX51 light microscope (Olympus, Tokyo, Japan); the digital images were captured with a DP71 digital camera (Olympus, Tokyo, Japan). Positive cells were counted on ImageJ, and the counting was performed by two independent pathologists under blinded conditions.
cDNA synthesis and quantitative real-time polymerase chain reaction
Total cellular RNA from the jejunum was extracted using TRIzol reagent (Ambion, Austin, TX). The cDNA was synthesized from total RNA (5 μg) in a 20 μl mixture containing oligo-dT, dNTP, RNase OUTtm, 5X First Strand Buffer, 0.1 M DTT and M-MLV reverse transcriptase. Quantitative real-time polymerase chain reaction (qRT-PCR)was carried out with a CFX96tm real-time system (Bio-Rad, Hercules, CA) using an iQtm SYBR Green Supermix kit (Bio-Rad, Hercules, CA), following the manufacturer’s instruction. All of the PCR primers (Chen et al. 2014) were synthesized by the Bioneer corporation (Daejeon, Korea). The annealing temperature was 58°C, and there were 39 cycles of amplification. The primers used were for Gadd45b (F: 5′-ATT GAC ATC GTC CGG GTA TC-3′; R: 5′-TGA CAG TTC GTG ACC AGG AG-3′), SOD2 (F: 5′-CAC ATT AAC GCG CAG ATC ATG-3′; R: 5′-CCA GAG CCT CGT GGT ACT TCT C-3′), Rad21 (F: 5′-TCC GCA TCT ATC ACA G-3′; R: 5′-TAA ATC TGG CAG TGG C-3′) and Irg1 (F: 5′-GAC AGG CAC AGA AGT G-3′; R: 5′-AGG GTG CCA TGT GTC ATC-3′), and the expressions were normalized to GAPDH (F: 5′-CTG GAG AAA CCT GCC AAG TA-3′; R: 5′-GTC CTC ACT GTA GCC CAA GA-3′). The threshold cycle (Ct value), which inversely correlated with target mRNA levels, was measured as the cycle number at which the reporter fluorescent emission increased above a threshold level. All samples were analyzed in triplicate and plotted with mean values.
Western blot analysis
Proteins were extracted from the jejunum (20–25 μg) using tissue-protein extraction reagent (Thermo Scientific, Waltham, MA). Protein concentration was calculated and standardized using a BCAtm Protein Assay Kit (Thermo Scientific, Waltham, MA) and boiled at 95°C for 5 min with ×4 sample buffer. Equal amounts of proteins (20 μg per lane) were separated on an 8% SDS-polyacrylamide gel, transferred onto Immobilon-P nitrocellulose membranes (Merck, Kenilworth, NJ), blocked with 5% non-fat dry skim milk in TBS with 1% Trixon X-100 (TBST) for an hour at room temperature, and incubated overnight at 4°C with the monoclonal antibodies against β-actin (sc-47778, 1:1000; Santa Cruz Biotechnology, Santa Cruz, CA) and NF-κB p65 (sc-8008, 1:1000; Santa Cruz Biotechnology, Santa Cruz, CA). The membranes were then washed with TBST and incubated with rabbit anti-mouse IgG-HRP secondary antibody (sc-358914, 1:2000; Santa Cruz Biotechnology, Santa Cruz, CA). Each targeted antibody was detected with Claritytm Western ECL substrate (Bio-Rad, Hercules, CA). The intensities of the bands were visualized by using ImageQuant LAS 4000 (GE Healthcare, little Chalfont, England) and ImageQuant software (Multiguage V 3.0, Fuji-Film, Tokyo, Japan) and normalized to β-actin expression
Enzyme-linked immunosorbent assay
The levels of various cytokines were measured using the Duoset Enzyme Linked ImmunoSorbent Assay (ELISA) kit (R&D systems, Minneapolis, MN). Levels of tumor necrosis factor-α (TNF-α), granulocyte colony stimulating factor (G-CSF), interleukin 6 (IL-6) and interferon gamma inducible protein 10 (IP-10) were measured as per the manufacturer’s instruction. The serum was diluted 1:5 in diluent buffer to adjust overwhelming cytokine levels. Each cytokine level was analyzed with a reference to the standard curve according to its recombinant cytokine. Absorbance was measured at 450 nm and 570 nm using the Sunrise micro plate reader (Tecan, Männedorf, Switzerland). Detection limits for TNF-α, G-CSF, Il-6 and IP-10 were 31.3, 31.3, 15.6 and 62.5 pg/ml, respectively.
Statistical analysis
Quantitative data were reported as mean values with standard deviation or standard errors. For the survival data, the difference in the 30-day survival between groups was determined by the log-rank test. The differences between experimental groups were analyzed by the two-tailed Student’s t-test. The value of P < 0.05 was determined to be statistically significant.
RESULTS
KMRC011 enhanced the survival rate in lethally irradiated mice depending on its dosage and repeated treatment
To analyze whether KMRC011 could increase the survival rate of C57BL/6N mice after γ irradiation exposure, various doses and repetitions of KMRC011 injections were performed and assessed for up to 30 days. We found that KMRC011-treated mice had a significantly improved survival rate after 11 Gy TBI, whereas only 10% of the mice in the PBS-treated group survived until the end of the experiment. The survival rate of mice receiving a high dose of KMRC011 (0.05 mg/kg) was 1.7 times higher compared with those receiving a low dose (0.01 mg/kg), but this was not statistically significant (P = 0.18).
To accelerate the radioprotective activity of KMRC011, we performed one, two or four administrations (15 min, 24 h, 48 h and 72 h after the radiation exposure) of KMRC011. We observed that additional injections resulted in better radioprotective activity, leading to an increased survival rate. The survival rates of mice injected one, two or four times were 40, 50 and 90%, respectively. Interestingly, 90% of the mice that were injected four times with KMRC011 survived until the end of the experiment. In addition, there was a highly significant difference in the survival rates of the mice treated with four-times injection with KMRC011 (P = 0.0002), one-time injection with KMRC011 (P = 0.07), and two-times injection with KMRC011 (P = 0.009) compared with control) (Fig. 1).
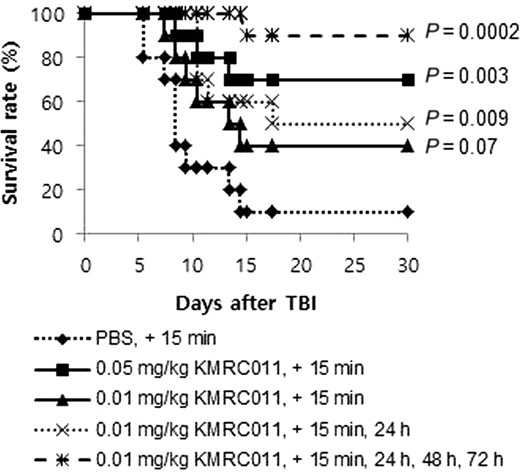
Survival rate of mice injected with KMRC011 after 11 Gy total-body irradiation (TBI). Ten C57BL/6N mice per group were treated with a single or multiple intramuscular injection(s) of KMRC011 or PBS at different time points (15 min, 24 h, 48 h, or 72 h) after TBI. P values were calculated by the log-rank test versus the PBS-treated group.
KMRC011 stimulated cell proliferation and inhibited apoptosis in the small intestine of lethally irradiated mice
In an endeavour to understand the radioprotective mechanism of KMRC011, C57BL/6N mice were sacrificed at 1, 3 or 5 days after 11 Gy TBI. Then, we compared the KMRC011-treated group with the PBS 5 days group and the non-irradiated control group. To detect cell proliferation within the small intestinal crypts, BrdU-positive cells were examined. BrdU-labeled cells were significantly (P < 0.01) decreased in mice 1 day after the 11 Gy TBI compared with non-irradiated mice or those sacrificed at Day 3. After 5 days, the KMRC011-treated irradiated mice showed significantly (P < 0.05) greater numbers of BrdU-positive cells than the non-irradiated control group and the PBS 5 days group (Fig. 2D). These data suggest that KMRC011 treatment increased the proliferation of crypt cells in the small intestine.
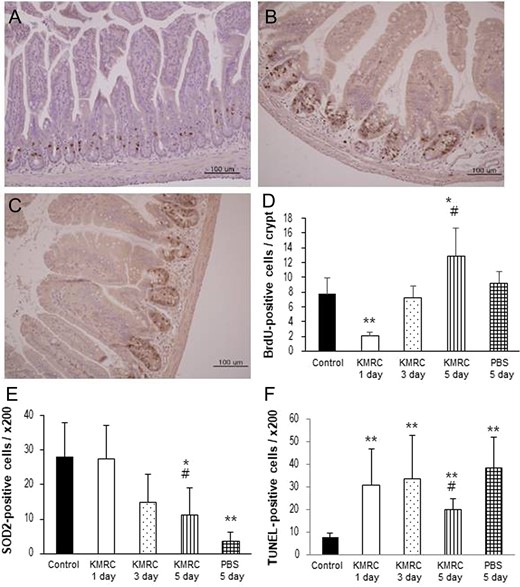
Representative image of BrdU immunoreactivity in the small intestine in KMRC011-treated irradiated C57BL/6N mice after 1 day (Panel A) or 5 days (Panel B) compared with the PBS 5 days group (Panel C). Panel D: Quantification of BrdU-positive cells in the crypt cells of the small intestine. Panel E: Quantification of SOD2-positive cells in the small intestine. Panel F: Quantification of TUNEL-positive cells in the small intestine. KMRC011 was intramuscularly injected 15 min after 11 Gy total-body irradiation (TBI), except for the control group. Data are expressed as mean ± SD (n = 6). *P < 0.05 and **P < 0.01 versus control group; #P < 0.05 versus PBS 5 days group, using two-tailed t-tests.
To determine the Sod2-mediated radioprotective property achieved by KMRC011, Sod2-labeled cells were counted in the mucosa of the small intestine. A continuous decrease in Sod2-positive cells was observed in the treatment groups until 5 days after the TBI. After 5 days, Sod2-labeled cells in irradiated mice were decreased significantly (P < 0.05) compared with in non-irradiated mice. However, after 5 days, KMRC011-treated irradiated mice showed a significant (P < 0.05) increase in Sod2-positive cells compared with the PBS 5 days mice (Fig. 2E). These data demonstrate that KMRC011 treatment was effective for protecting the intestinal mucosa against injury from TBI.
To determine the anti-apoptotic effects of KMRC011 in the small intestine, we used the In Situ Cell Death Detection Kit. The number of TUNEL-labeled cells was significantly (P < 0.01) increased in all groups that received 11 Gy TBI, compared with the non-irradiated control group. The KMRC011-treated irradiated mice after 5 days showed a significantly (P < 0.05) lower number of apoptotic cells than the PBS 5 days mice (Fig. 2F). These results indicated that KMRC011 treatment is effective for protecting against apoptosis in the intestinal mucosa injured by TBI.
KMRC011 increased the expression of genes related to DNA repair
To determine whether KMRC011 could protect against radiation-induced DNA damage in C57BL/6N mice, relative mRNA levels of Rad21, Gadd45b and Sod2 were measured using real-time PCR analysis. The relative mRNA expression levels of Rad21 decreased 1 day after the radiation exposure, but then increased greatly after 3 days; the increased expression levels persisted for at least 5 days after the TBI. KMRC011-treated irradiated mice showed significantly (P < 0.05) higher Rad21 expression after 5 days than the control group (Fig. 3A). The levels of Gadd45b mRNA were decreased 1 day after the radiation, then showed a significant (P < 0.05) increase at Day 3 compared with the control group. A significant (P < 0.05) increase in Gadd45b mRNA expression was also detected in KMRC011-treated irradiated mice after 5 days compared with the control (Fig. 3B). The Sod2 mRNA expression levels were decreased in KMRC011-treated irradiated mice until at least 5 days after the radiation exposure. KMRC011-treated irradiated mice showed significantly (P < 0.05) higher Sod2 expression levels after 5 days compared with the control group (Fig. 3C). These findings suggest that KMRC011 treatment after TBI increased the expression of DNA repair–related genes, such as Rad21, Gadd45b and Sod2.
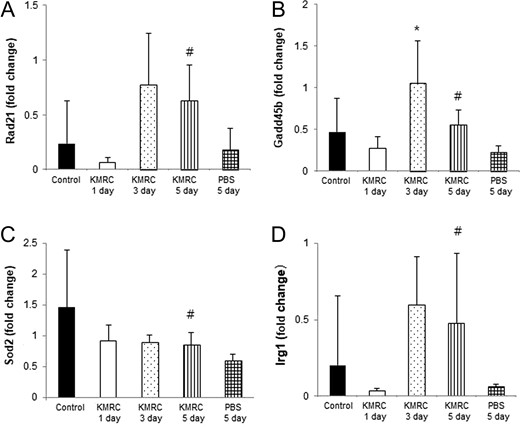
Real-time PCR analysis of Rad21 (Panel A), Gadd45b (Panel B), Sod2 (Panel C) and Irg1 (Panel D) in the small intestine of C57BL/6N mice. KMRC011 was intramuscularly injected 15 min after 11 Gy total-body irradiation (TBI), except for the control group. Data are expressed as mean ± SD (n = 6). *P < 0.05 and *P < 0.05 versus the control group; #P < 0.05 versus the PBS 5 days group, using two-tailed t-tests.
To test whether KMRC011 treatment could increase Irg1 gene expression in the intestine damaged by TBI, Irg1 mRNA expression was assessed using real-time PCR analysis. The relative mRNA expression levels of Irg1 were decreased 1 day after the radiation exposure, but then greatly increased at Day 3; the increase persisted for at least 5 days after the TBI. KMRC011-treated irradiated mice at 5 days after the TBI had significantly (P < 0.05) higher Irg1 expression levels than the control group (Fig. 3D). This finding indicated that KMRC011 triggered Irg1 gene expression, thus modulating immune responses occurring after the irradiated small intestine injury.
We also compared the hematology data in C57BL/6N mice after the 11 Gy TBI. White blood cells were significantly (P < 0.05) decreased in all irradiated groups, compared with the non-irradiated control group (Fig. 4A). Also, the counts of neutrophils, red blood cells, and hemoglobin were significantly (P < 0.05) decreased in the KMRC 3 days, KMRC 5 days and PBS 3 days groups, compared with the non-irradiated control group (Fig. 4B, C and D). However, there was no statistically significant difference between the KMRC 5 days group and the PBS 5 days group.
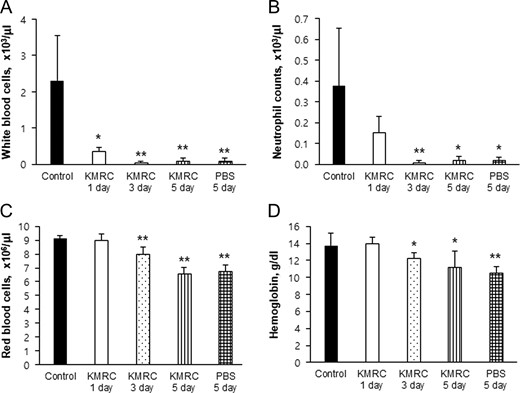
Comparison of peripheral blood white blood cells (Panel A), neutrophils (Panel B), red blood cells (Panel C) and hemoglobin concentration (Panel D) in C57BL/6N mice. KMRC011 was intramuscularly injected 15 min after 11 Gy total body irradiation (TBI), except for the control group. Data are expressed as mean ± SD (n = 6). *P < 0.05 and *P < 0.05 versus the control group, using two-tailed t-tests.
KMRC011 reduced the expression of NF-κB in the small intestine injured by TBI
To assess whether the expression levels of NF-κB were changed in the small intestine of C57BL/6N mice by the KMRC011 treatment, NF-κB p65 levels were measured by western blotting. NF-κB p65 expression was significantly (P < 0.05) lower in KMRC011-treated irradiated mice after 5 days compared with in the control group (Fig. 5). This data indicated that KMRC011 reduced the levels of p65 expression in the small intestine injured by TBI.
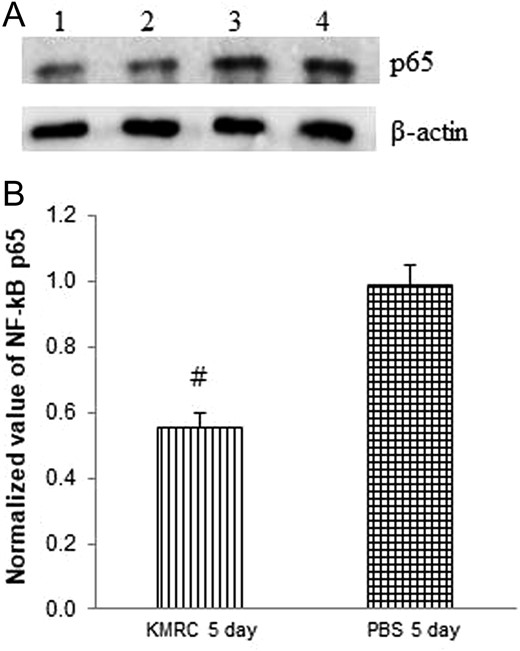
The expression of NF-κB p65 (Panel A; Lane 1 and 2: KMRC 5 days, Lane 3 and 4: PBS 5 days) in the small intestine of C57BL/6N mice by western blot analysis. Panel B: relative density of NF-κB p65. Intensities of the bands were measured against β-actin expression. KMRC011 was intramuscularly injected 15 min after 11 Gy total body irradiation (TBI), except for the control group. Data are expressed as mean ± SD (n = 6). #P < 0.05 versus the PBS 5 days group, using two-ailed t-tests.
Cytokine levels induced by KMRC011 differed depending on the route of administration and the type of cytokine
We assessed serum levels of G-CSF, IL-6, IFN-γ, TNF-α and IP-10 in ICR mice at multiple time points following KMRC011 treatment. Time-dependent changes in serum cytokine levels were similar regardless of the route of administration. The cytokine levels of G-CSF reached a peak level at 2 h, which persisted until 8 h, then normalized over the period 8–24 h after the KMRC011 treatment. The IP group showed the highest G-CSF level, followed by the SC group. Maximally observed serum G-CSF levels in the IP group were ~23 500 pg/ml. The IP and SC groups showed significantly higher IL-6 levels (~2-fold increases), compared with the IM group (Fig. 6A).
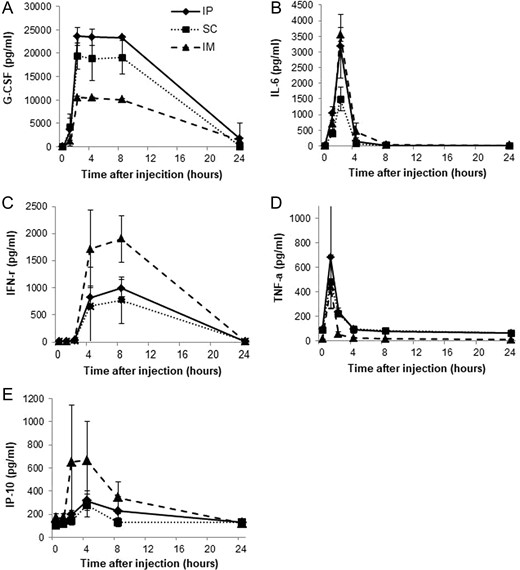
Time-dependent changes in cytokine levels in the serum of non-irradiated ICR mice injected intramuscularly, intraperitoneally or subcutaneously with KMRC011 (0.2 mg/kg). Serum levels of G-CSF (Panel A), IL-6 (Panel B), IFN-γ (Panel C), TNF-α (Panel D) and IP-10 (Panel E) after KMRC011 injection were measured. Data are expressed as mean ± SD (n = 5).
IL-6 levels reached the highest peak at 2 h and returned to baseline levels at 8 h after the KMRC011 injection. The IM group showed the highest IL-6 level, followed by the IP group. Maximally induced IL-6 levels in the IM group were ~3500 pg/ml. The IM and IP groups showed significantly higher IL-6 levels (about a 2-fold increase) compared with the SC group (Fig. 6B).
The cytokine levels of IFN-γ were dramatically increased at 4 h, reached a peak level at 8 h, and returned to baseline levels over the period 8–24 h after the KMRC011 injection. The IM group showed the highest IFN-γ level, followed by the IP group. Maximal IFN-γ levels in the IM group were ~1700 pg/ml. The IM group showed significantly higher levels of IFN-γ (about a 2-fold increase) compared with the IP and SC groups (Fig. 6C).
TNF-α levels increased rapidly, reached a peak at 1 h, and normalized by 4 h after the KMRC011 treatment. The IP group showed the highest TNF-α level, followed by the SC group. The maximally observed serum TNF-α levels were ~680 pg/ml. The IP group showed significantly higher TNF-α levels (about a 1.4-fold increase) compared with the SC and IM groups (Fig. 6D).
IP-10 levels dramatically increased at 2 h, reached a peak at 4 h, and returned to baseline levels over the period 8–24 h after the KMRC011 injection. The IM group showed the highest IP-10 level, followed by the IP group. The maximal IP-10 level in the IM group was ~650 pg/ml. The IM group showed a significantly higher IP-10 level (about 3.3-fold higher) than the SC and IP groups (Fig. 6E).
DISCUSSION
Radiation can be used therapeutically or can be potentially damaging to living organisms. Almost a half of the current cancer therapies rely on radiotherapy, despite its risks of side effects. [15]. It is crucial to discover how to prevent or reduce the side effects of radiation therapy [16]. In the current study, the novel TLR5 agonist KMRC011 was investigated to analyze its potential as a pharmaceutical drug to reduce death caused by TBI.
CBLB502 has been reported as a radioprotective therapeutic in mice and rhesus monkeys exposed to lethal TBI. NIH Swiss mice were injected with CBLB502 (0.2 mg/kg) 30 min before 13 Gy TBI, and 87% of the mice survived for at least 30 days [11]. Flagellin-elicited radioprotection was effective if given until 4 h after exposure of mice to irradiation [17]. Also, administration of entolimod 1 h after fractionated head-and-neck irradiation in mice reduced its damage more than treatment administered 30 min prior to irradiation did [18]. In a 30-day survival study, 90% of C57BL/6N mice exposed to 11 Gy TBI died after 5–15 days due to acute gastrointestinal syndromes (Fig. 1). Also, 71% of ICR mice exposed to 12 Gy TBI died after 6–20 days in a 30-day survival study (Fig. S1). Our data show that KMRC011 has a radioprotective activity in mice irradiated with 11 Gy or 12 Gy. There was no significant difference between KMRC011 and CBLB502 in a 30-day survival study using ICR mice (Fig. S1). Interestingly, the most efficient radioprotection (90% survival) was observed in C57BL/6N mice injected with KMRC011 intramuscularly at 0.01 mg/kg for 4 repeated days; the next most efficient radioprotection was observed in mice treated with a high dose (0.05 mg/kg) for 1 day (70% survival). When considering repeated injection, adverse health effects should be considered. As a result of 28‐day repeated‐dose toxicity of KMRC011, the no‐observed‐adverse‐effect level of KMRC011 was <0.25 mg/kg/day in rats [19] and <0.04 mg/kg/day in cynomolgus monkeys [20].
Although the mechanism of intestine damage due to ionizing radiation is not fully understood, protection of intestinal cells may be due to the inhibition of apoptosis and an increase in cell proliferation [21]. Our immunohistochemical study revealed that the number of proliferative crypt cells in the small intestine were significantly higher in KMRC011-treated irradiated mice after 5 days compared with in control mice, as shown by the BrdU assay. The number of apoptotic cells in the small intestine was significantly decreased in KMRC011-treated irradiated mice after 5 days, compared with control mice, as shown by the TUNEL assay (Fig. 2). These results indicate that the radioprotective effect of KMRC011 may be due to the protection of intestinal stem cells and to an increase in proliferating crypt cells. In the previous study, the TLR5 agonist also decreased pulmonary cell apoptosis after irradiation [22].
Several genes involved in DNA repair have been reported to be associated with irradiation. Rad21 is a gene involved in repairing double-strand breaks and cell cycle regulation [23, 24]. Gadd45b is a gene involved in cell cycle arrest, inducing apoptosis, and DNA damage repair [25]. Sod2 is a gene involved in decreasing superoxide production and inducing production of hydrogen peroxide [26]. In this study, DNA repair–related genes such as Rad21, Gadd45b, Sod2 and Irg1 were significantly upregulated in the small intestine of KMRC011-treated irradiated mice after 5 days, compared with control mice, as revealed by real-time PCR (Fig. 3). The number of Sod2-positive cells in the lamina propria of the small intestine was significantly higher in KMRC011-treated irradiated mice after 5 days, compared with in the control mice, as revealed by immunohistochemical staining (Fig. 2E) and the CBLB502 experiment [11]. This result was similar to that in another report showing that DNA repair–related genes were upregulated by TLR agonists [12]. Our results indicated that irradiation-induced DNA damage in the intestinal epithelium could be repaired by KMRC011 treatment through a process associated with TLR5 activation.
The TLR5 agonist induces the innate immune response and cell survival via the NF-κB pathway upon binding of bacterial flagella [27, 28]. The western blot analysis of NF-κB expression in this study showed that p65 (RelA) expression levels were significantly downregulated in the KMRC 5 days group, compared with the PBS 5 days group (Fig. 5). Recently, melatonin gel reduced apoptosis in the small intestine of Wistar rats that had received 7.5 Gy, by inhibition of the NF-κB and NLRP3 inflammasome pathways [29]. Likewise, KMRC011 administration reduced small intestinal mucosal damage by downregulating the NF-kB and NLRP3 pathways, thus aiding recovery. In a previous study, CBLB502 reduced NF-κB p65 expression levels in the mouse colon, but NF-kB expression was not significantly lower than in the ulcerative colitis model group [30]. Recently, via the NF-kB signaling pathway, CBLB502 was found to decrease chromosomal aberration and testicular injuries [31]. Also, in the earlier studies, ionizing irradiation increased NF-κB binding to DNA in a dose-dependent manner [32, 33]. This suggests that our observation that KMRC011 had a radioprotective effect might be explained by its inhibiting the NF-kB and NLRP3 pathways to minimize the intestinal damage.
The pharmacokinetic results for KMRC011 differed depending on the route of administration in the non-irradiated mice. The IP group showed the highest levels of G-CSF and TNF-α, and the IM group showed the highest levels of IL-6, IFN-γ and IP-10. Interestingly, the amount of time required to reach peak levels in the serum after KMRC011 treatment differed depending on the type of cytokine. Of the five cytokines that were analyzed, serum G-CSF cytokine reached the highest expression level and persisted at this level for the longest period of time. TNF-α reached its peak level the quickest at 1 h after the KMRC011 injection. IFN-γ was the slowest to reach its peak level (at 8 h after the injection) (Fig. 6). These experiments showed that G-CSF, IL-6, IFN-γ, TNF-α and IP-10 cytokines could be used as candidate biomarkers for evaluating the clinical efficacy of KMRC011, and that these cytokines played important roles in the radioprotective mechanisms of KMRC011. G-CSF and IL-6 were reported as biomarkers for evaluating the protective efficacy of CBLB502, a TLR5 agonist [11, 34]. G-CSF and granulocyte macrophage colony-stimulating factor are known to play important roles in hematopoiesis and could be used for treatment of patients with radiological/nuclear injuries [35]. In summary, our results indicate that KMRC011, a novel TLR5 agonist, has radioprotective activity in lethally irradiated mice, and that KMRC011 can be developed as a therapeutic agent for radioprotection through further studies.
CONFLICT OF INTEREST
The Korea Institute of Industrial Technology owned patent right of KR101744297B1 on the method for production of TLR5 agonist (KMRC011, https://patents.google.com/patent/KR101634380B1/en). The authors alone are responsible for the content and writing of the paper.
FUNDING
This research was supported by a grant funded by the Civil-Military Technology Cooperation Program (14-CM-EB-09; NTIS 1695004819).