-
PDF
- Split View
-
Views
-
Cite
Cite
Kiyonao Nakamura, Itaru Ikeda, Haruo Inokuchi, Kenji Takayama, Takahiro Inoue, Tomomi Kamba, Osamu Ogawa, Masahiro Hiraoka, Takashi Mizowaki, A pilot study of highly hypofractionated intensity-modulated radiation therapy over 3 weeks for localized prostate cancer, Journal of Radiation Research, Volume 59, Issue 5, September 2018, Pages 656–663, https://doi.org/10.1093/jrr/rry060
- Share Icon Share
Abstract
The purpose of this pilot study was to evaluate the feasibility of highly hypofractionated intensity-modulated radiation therapy (IMRT) in 15 fractions over 3 weeks for treating localized prostate cancer based on prostate position-based image-guided radiation therapy. Twenty-five patients with National Comprehensive Cancer Network (NCCN) very low- to unfavorable intermediate-risk prostate cancer were enrolled in this study from April 2014 to September 2015 to receive highly hypofractionated IMRT (without intraprostatic fiducial markers) delivering 54 Gy in 15 fractions over 3 weeks. Patients with intermediate-risk disease underwent neoadjuvant androgen suppression for 4–8 months. Twenty-four patients were treated with highly hypofractionated IMRT, and one was treated with conventionally fractionated IMRT because the dose constraint of the small bowel seemed difficult to achieve during the simulation. Seventeen percent had very low- or low-risk, 42% had favorable intermediate-risk, and 42% had unfavorable intermediate-risk disease according to NCCN guidelines. The median follow-up period was 31 months (range, 24–42 months). No Grade ≥3 acute toxicity was observed, and the incidence rates of Grade 2 acute genitourinary and gastrointestinal toxicities were 21% and 4%, respectively. No Grade ≥2 late toxicity was observed. Biochemical relapse was observed in one patient at 15 months, and the biochemical relapse-free survival rate was 95.8% at 2 years. A prostate-specific antigen bounce of ≥0.4 ng/ml was observed in 11 patients (46%). The highly hypofractionated IMRT regimen is feasible in patients with localized prostate cancer and is more convenient than conventionally fractionated schedules for patients and health-care providers.
INTRODUCTION
Prostate cancer has been projected to be the third most common male cancer diagnosed in Japan in 2017 [1]. Radical treatment options include prostatectomy, brachytherapy, and external-beam radiation therapy (EBRT). Some EBRT studies have shown that increasing the radiation dose significantly improves biochemical relapse-free survival (BRFS) [2]. However, the risk of toxicity to normal tissues around the prostate increases as the dose increases. In addition, the prostate is a mobile organ, so larger margins around the target should be added during EBRT than the margins for other non-mobile organs [3]. Therefore, increasing the dose to the prostate using 3D conformal radiation therapy (3DCRT) is difficult. In recent years, image-guided radiation therapy (IGRT) and intensity-modulated radiation therapy (IMRT) have been developed to provide precise irradiation and dose escalation to the prostate without increasing the dose to normal tissues; several reports have shown good oncological outcomes for prostate cancer with IMRT [4]. One of the disadvantages of conventionally fractionated IMRT is the long treatment course: It usually takes 8–9 weeks to complete treatment, which is much longer than prostatectomy and brachytherapy and is inconvenient for patients.
The α/β value in prostate cancer is lower than the values for other tumors and normal tissues [5–7]. Hence, increasing the fraction size enhances the antitumor effect more than for other tumors. In other words, the large fraction size of hypofractionated radiotherapy has a tremendous advantage over conventionally fractionated radiotherapy based on the linear-quadratic (LQ) model. In addition, a shorter course of treatment is more convenient for the patient and reduces health-care costs. Therefore, hypofractionated radiotherapy is a promising approach.
We designed a pilot study of highly hypofractionated IMRT for treating localized prostate cancer by delivering 54 Gy in 15 fractions (3.6 Gy per fraction) over 3 weeks. The equivalent total dose in 2 Gy fractions (EQD2) of this regimen to prostate cancer (α/β value = 1.5 Gy) was estimated to be ~78.7 Gy, and the EQD2 to normal tissues (α/β value = 3 Gy) was estimated to be 71.3 Gy. Here we report the outcomes of this prospective pilot study.
MATERIALS AND METHODS
This single-institution, prospective pilot clinical trial was approved by the institutional review board of our institution (Approval no. C751).
Eligibility
Eligible patients were men aged 50–79 years who had histologically confirmed adenocarcinoma of the prostate with National Comprehensive Cancer Network (NCCN) very low- to unfavorable intermediate-risk disease [8] and Eastern Cooperative Oncology Group performance status of 0–1. Written informed consent was required for participation.
The pretreatment evaluation consisted of digital rectal examinations, basic blood examinations [including serum prostate-specific antigen (PSA) level], abdominal computed tomography (CT), pelvic magnetic resonance imaging (MRI), and a prostate biopsy. Bone scintigraphy was not mandatory.
Exclusion criteria included (i) history of transurethral resection of the prostate or high-intensity focused ultrasound of the prostate, (ii) presence of hip prosthesis, (iii) severe diabetes mellitus, (iv) history of inflammatory bowel disease, (v) previous radiotherapy to the pelvis, (vi) active double cancer (i.e. overlapping cancer or asynchronous cancer within 5 years, except carcinoma in situ, intramucosal cancer, and other equivalent lesions), (vii) severe psychosis, (viii) difficulty withdrawing from an anticoagulant and (ix) previous abdominal surgery other than appendectomy.
Androgen deprivation therapy
Patients with intermediate-risk disease underwent neoadjuvant androgen deprivation therapy (NA-ADT) for 4–8 months. NA-ADT consisted of either luteinizing hormone-releasing hormone agonists alone or gonadotropin-releasing hormone antagonists alone or combined with an androgen blockade. NA-ADT was not mandatory in patients with low-risk disease. Adjuvant ADT was not provided for any patient. The serum testosterone levels of the patients in this trial were not routinely measured because measurement of them for prostate cancer are not covered by health insurance in Japan.
Radiation therapy
The details of our CT simulation procedures have been reported previously [9]. Patients were immobilized in the prone or supine position using a vacuum pillow (Vac-Lok system; Med-Tec, Orange City, IA, USA) with an original leg support and instructed to void their urinary bladder and rectum 1–1.5 h before the CT simulation. Axial CT images were collected through the pelvis at 2.5 mm intervals.
The clinical target volume included the prostate and base of the seminal vesicles. We generated the planning target volume (PTV) by adding 7.5 mm margins in the craniocaudal directions, 6 mm margins in the anterior and lateral directions, and a 5 mm margin at the prostatorectal interface. The rectal outer wall, bladder outer wall, small bowel, and large bowel were contoured and defined as organs at risk (OARs).
A nominal dose of 54 Gy in 15 fractions (3.6 Gy per fraction) was delivered over 3 weeks using image-guided IMRT. The prescribed dose was defined as the dose delivered to 50% of the volume (D50) of the PTV. The PTV was covered by the 95% isodose, except for the prostatorectal interface. The maximum dose could not be within the OARs. The treatment planning goals are listed in Table 1. The dose constraints in this trial were set based on our clinically validated IMRT protocol delivering 78 Gy in 39 fractions [9, 10], so that each constraint did not exceed the corresponding constraint of the 78 Gy protocol, when converted to EQD2. Minor violations were acceptable. All treatment plans were carried out using Vero4DRT (MHI-TM2000; Mitsubishi Heavy Industries, Hiroshima, Japan, and BrainLAB, Munich, Germany) with the 6 MV 7-field static multileaf collimator technique for IMRT beam delivery.
Structure . | No violation . | Minor violation . | Major violation . |
---|---|---|---|
CTV | |||
D2 | ≤56.7 Gy | ≤57.78 Gy | >57.78 Gy |
D98 | ≥51.3 Gy | ≥50.22 Gy | <50.22 Gy |
PTV | |||
D2 | ≤56.7 Gy | ≤57.87 Gy | >57.78 Gy |
D50 | 53.46 Gy < D50 < 54.54 Gy | ||
D95 | ≥51.3 Gy | ≥50.22 Gy | <50.22 Gy |
Rectal wall | |||
V30 Gy | ≤60% | ≤65% | |
V45 Gy | ≤30% | ≤35% | |
V50 Gy | ≤20% | ≤25% | |
V54 Gy | <1% | ||
Bladder wall | |||
V30 Gy | ≤60% | ≤65% | |
V50 Gy | ≤0% | ≤35% | |
Small bowel | |||
V45 Gy | <0.5 ml | ||
Large bowel | |||
V48 Gy | <0.5 ml |
Structure . | No violation . | Minor violation . | Major violation . |
---|---|---|---|
CTV | |||
D2 | ≤56.7 Gy | ≤57.78 Gy | >57.78 Gy |
D98 | ≥51.3 Gy | ≥50.22 Gy | <50.22 Gy |
PTV | |||
D2 | ≤56.7 Gy | ≤57.87 Gy | >57.78 Gy |
D50 | 53.46 Gy < D50 < 54.54 Gy | ||
D95 | ≥51.3 Gy | ≥50.22 Gy | <50.22 Gy |
Rectal wall | |||
V30 Gy | ≤60% | ≤65% | |
V45 Gy | ≤30% | ≤35% | |
V50 Gy | ≤20% | ≤25% | |
V54 Gy | <1% | ||
Bladder wall | |||
V30 Gy | ≤60% | ≤65% | |
V50 Gy | ≤0% | ≤35% | |
Small bowel | |||
V45 Gy | <0.5 ml | ||
Large bowel | |||
V48 Gy | <0.5 ml |
CTV = clinical target volume, PTV = planning target volume, Dx = dose delivered to x% of volume, Vx Gy = percentage of volume receiving x Gy or the volume receiving x Gy.
Structure . | No violation . | Minor violation . | Major violation . |
---|---|---|---|
CTV | |||
D2 | ≤56.7 Gy | ≤57.78 Gy | >57.78 Gy |
D98 | ≥51.3 Gy | ≥50.22 Gy | <50.22 Gy |
PTV | |||
D2 | ≤56.7 Gy | ≤57.87 Gy | >57.78 Gy |
D50 | 53.46 Gy < D50 < 54.54 Gy | ||
D95 | ≥51.3 Gy | ≥50.22 Gy | <50.22 Gy |
Rectal wall | |||
V30 Gy | ≤60% | ≤65% | |
V45 Gy | ≤30% | ≤35% | |
V50 Gy | ≤20% | ≤25% | |
V54 Gy | <1% | ||
Bladder wall | |||
V30 Gy | ≤60% | ≤65% | |
V50 Gy | ≤0% | ≤35% | |
Small bowel | |||
V45 Gy | <0.5 ml | ||
Large bowel | |||
V48 Gy | <0.5 ml |
Structure . | No violation . | Minor violation . | Major violation . |
---|---|---|---|
CTV | |||
D2 | ≤56.7 Gy | ≤57.78 Gy | >57.78 Gy |
D98 | ≥51.3 Gy | ≥50.22 Gy | <50.22 Gy |
PTV | |||
D2 | ≤56.7 Gy | ≤57.87 Gy | >57.78 Gy |
D50 | 53.46 Gy < D50 < 54.54 Gy | ||
D95 | ≥51.3 Gy | ≥50.22 Gy | <50.22 Gy |
Rectal wall | |||
V30 Gy | ≤60% | ≤65% | |
V45 Gy | ≤30% | ≤35% | |
V50 Gy | ≤20% | ≤25% | |
V54 Gy | <1% | ||
Bladder wall | |||
V30 Gy | ≤60% | ≤65% | |
V50 Gy | ≤0% | ≤35% | |
Small bowel | |||
V45 Gy | <0.5 ml | ||
Large bowel | |||
V48 Gy | <0.5 ml |
CTV = clinical target volume, PTV = planning target volume, Dx = dose delivered to x% of volume, Vx Gy = percentage of volume receiving x Gy or the volume receiving x Gy.
Prostate position–based IGRT was performed in each treatment session. If patients accepted implantation of intraprostatic fiducial markers, fiducial markers were implanted 1–2 weeks before the CT simulation, and orthogonal kilovoltage radiographs were obtained immediately before each treatment session using the ExacTrac System (BrainLAB) and automatically registered to the reference image of digitally reconstructed radiographs. If a patient refused implantation, cone-beam computed tomography (CBCT) was taken before each session, and manual soft-tissue registration for prostate alignment between CBCT and the simulation CT was performed.
Study end-points and follow-up
A sample size was set at 25. The primary end-point was the incidence rate of acute toxicities, and the secondary end-points were incidence rates of late toxicities at 2 years and BRFS at 2 years. Acute toxicities were evaluated in the first 90 days after the beginning of radiation therapy, based on the Common Terminology Criteria for Adverse Events version 4.0. Late toxicities were evaluated based on the Radiation Therapy Oncology Group/European Organization for the Research and Treatment of Cancer (RTOG/EORTC) Late Radiation Morbidity Scoring Schema. Biochemical relapse was defined according to the Phoenix definition of PSA nadir +2 ng/ml [11], and clinical failure was defined as either radiological signs of relapse, a positive prostate biopsy after biochemical failure, or initiation of salvage ADT. Acute toxicities were scored weekly during radiation therapy and at 3 months after the initiation of radiation therapy. A PSA bounce was defined as a transient increase in PSA of ≥0.4 ng/ml above the nadir followed by a subsequent decrease in PSA. The follow-up interval was 3 months for a minimum of 2 years, and every 6 months thereafter.
Because this study was a pilot study, we accepted a two-fold higher confidence limit for toxicities from previously reported data [12] as the allowable upper confidence limit of this trial. That is, if three or fewer patients developed acute Grade ≥3 toxicities (90% CI; 1.3–22.7%), we supposed that it would be acceptable to step forward.
Statistical analysis
We estimated the BRFS rate and the late toxicity incidence rate using Kaplan–Meier analysis from the date of initiation of radiotherapy. All statistical analyses were conducted with EZR (Saitama Medical Center, Jichi Medical University, Saitama, Japan), a graphical user interface for R (R Foundation for Statistical Computing, Vienna, Austria).
RESULTS
Twenty-five patients were enrolled in the trial from April 2014 to September 2015. No patient had used an alpha blocker or an anticholinergic agent before the initiation of treatment. All patients refused implantation of fiducial markers, so IGRT was performed using CBCT without fiducial markers in any patient. All patients completed radiotherapy without interruption. Twenty-four patients were treated with highly hypofractionated IMRT, and one was treated with conventionally fractionated IMRT because his small bowel was close to the prostate and the dose constraint of the small bowel seemed difficult to achieve. Table 2 summarizes the characteristics of the 24 patients. The median age was 71 years; four patients (17%) had very low- or low-risk, 10 (42%) had favorable intermediate-risk, and 10 (42%) had unfavorable intermediate-risk disease according to NCCN guidelines.
Number of patients | 24 | |
Age (years) | ||
median (range) | 71 (62–79) | |
Clinical T stage | ||
T1c | 13 (54%) | |
T2a | 11 (46%) | |
T2b | 0 (0%) | |
Pretreatment PSA (ng/ml) | ||
<10 | 16 (67%) | |
10–20 | 8 (33%) | |
median (range) | 6.43 (4.19–16.12) | |
Gleason score | ||
3 + 3 | 5 (21%) | |
3 + 4 | 11 (46%) | |
4 + 3 | 8 (33%) | |
D’Amico risk | ||
low | 4 (17%) | |
intermediate | 20 (83%) | |
NCCN risk group | ||
very low | 1 (4%) | |
low | 3 (13%) | |
favorable intermediate | 10 (42%) | |
unfavorable intermediate | 10 (42%) | |
Neoadjuvant hormonal therapy | ||
CAB | 14 (58%) | |
LHRH antagonist | 8 (33%) | |
LHRH agonist | 1 (4%) | |
None | 1 (4%) | |
median duration (month) | 5.2 | |
Pretreatment prostate volume (ml) | ||
median (range) | 29.80 (8.15–46.00) |
Number of patients | 24 | |
Age (years) | ||
median (range) | 71 (62–79) | |
Clinical T stage | ||
T1c | 13 (54%) | |
T2a | 11 (46%) | |
T2b | 0 (0%) | |
Pretreatment PSA (ng/ml) | ||
<10 | 16 (67%) | |
10–20 | 8 (33%) | |
median (range) | 6.43 (4.19–16.12) | |
Gleason score | ||
3 + 3 | 5 (21%) | |
3 + 4 | 11 (46%) | |
4 + 3 | 8 (33%) | |
D’Amico risk | ||
low | 4 (17%) | |
intermediate | 20 (83%) | |
NCCN risk group | ||
very low | 1 (4%) | |
low | 3 (13%) | |
favorable intermediate | 10 (42%) | |
unfavorable intermediate | 10 (42%) | |
Neoadjuvant hormonal therapy | ||
CAB | 14 (58%) | |
LHRH antagonist | 8 (33%) | |
LHRH agonist | 1 (4%) | |
None | 1 (4%) | |
median duration (month) | 5.2 | |
Pretreatment prostate volume (ml) | ||
median (range) | 29.80 (8.15–46.00) |
PSA = prostate-specific antigen, CAB = combined androgen blockade, LHRH = luteinizing hormone–releasing hormone, NCCN = National Comprehensive Cancer Network.
Number of patients | 24 | |
Age (years) | ||
median (range) | 71 (62–79) | |
Clinical T stage | ||
T1c | 13 (54%) | |
T2a | 11 (46%) | |
T2b | 0 (0%) | |
Pretreatment PSA (ng/ml) | ||
<10 | 16 (67%) | |
10–20 | 8 (33%) | |
median (range) | 6.43 (4.19–16.12) | |
Gleason score | ||
3 + 3 | 5 (21%) | |
3 + 4 | 11 (46%) | |
4 + 3 | 8 (33%) | |
D’Amico risk | ||
low | 4 (17%) | |
intermediate | 20 (83%) | |
NCCN risk group | ||
very low | 1 (4%) | |
low | 3 (13%) | |
favorable intermediate | 10 (42%) | |
unfavorable intermediate | 10 (42%) | |
Neoadjuvant hormonal therapy | ||
CAB | 14 (58%) | |
LHRH antagonist | 8 (33%) | |
LHRH agonist | 1 (4%) | |
None | 1 (4%) | |
median duration (month) | 5.2 | |
Pretreatment prostate volume (ml) | ||
median (range) | 29.80 (8.15–46.00) |
Number of patients | 24 | |
Age (years) | ||
median (range) | 71 (62–79) | |
Clinical T stage | ||
T1c | 13 (54%) | |
T2a | 11 (46%) | |
T2b | 0 (0%) | |
Pretreatment PSA (ng/ml) | ||
<10 | 16 (67%) | |
10–20 | 8 (33%) | |
median (range) | 6.43 (4.19–16.12) | |
Gleason score | ||
3 + 3 | 5 (21%) | |
3 + 4 | 11 (46%) | |
4 + 3 | 8 (33%) | |
D’Amico risk | ||
low | 4 (17%) | |
intermediate | 20 (83%) | |
NCCN risk group | ||
very low | 1 (4%) | |
low | 3 (13%) | |
favorable intermediate | 10 (42%) | |
unfavorable intermediate | 10 (42%) | |
Neoadjuvant hormonal therapy | ||
CAB | 14 (58%) | |
LHRH antagonist | 8 (33%) | |
LHRH agonist | 1 (4%) | |
None | 1 (4%) | |
median duration (month) | 5.2 | |
Pretreatment prostate volume (ml) | ||
median (range) | 29.80 (8.15–46.00) |
PSA = prostate-specific antigen, CAB = combined androgen blockade, LHRH = luteinizing hormone–releasing hormone, NCCN = National Comprehensive Cancer Network.
Table 3 details the dose parameter of the targets and OARs and the dose constraint violations. No major dose constraint violation was observed in any patient. Minor dose constraint violations of PTV D95 were observed in eight patients (33%). No other minor violation was observed.
Structure . | Mean ± SD . | Minor violation . | Major violation . |
---|---|---|---|
CTV | |||
D2 | 56.05 ± 0.72 Gy | 0 (0%) | 0 (0%) |
D98 | 52.82 ± 0.44 Gy | 0 (0%) | 0 (0%) |
PTV | |||
D2 | 56.04 ± 0.38 Gy | 0 (0%) | 0 (0%) |
D50 | 54.35 ± 0.23 Gy | 0 (0%) | 0 (0%) |
D95 | 51.44 ± 0.48 Gy | 8 (33%) | 0 (0%) |
Rectal wall | |||
V30 Gy | 30.77 ± 6.50% | 0 (0%) | 0 (0%) |
V45 Gy | 15.48 ± 3.69% | 0 (0%) | 0 (0%) |
V50 Gy | 6.60 ± 2.51% | 0 (0%) | 0 (0%) |
V54 Gy | 0 ± 0% | 0 (0%) | 0 (0%) |
Bladder wall | |||
V30 Gy | 25.04 ± 7.65% | 0 (0%) | 0 (0%) |
V50 Gy | 13.93 ± 4.13% | 0 (0%) | 0 (0%) |
Small bowel | |||
V45 Gy | 0 ± 0 ml | 0 (0%) | 0 (0%) |
Large bowel | |||
V48 Gy | 0 ± 0 ml | 0 (0%) | 0 (0%) |
Structure . | Mean ± SD . | Minor violation . | Major violation . |
---|---|---|---|
CTV | |||
D2 | 56.05 ± 0.72 Gy | 0 (0%) | 0 (0%) |
D98 | 52.82 ± 0.44 Gy | 0 (0%) | 0 (0%) |
PTV | |||
D2 | 56.04 ± 0.38 Gy | 0 (0%) | 0 (0%) |
D50 | 54.35 ± 0.23 Gy | 0 (0%) | 0 (0%) |
D95 | 51.44 ± 0.48 Gy | 8 (33%) | 0 (0%) |
Rectal wall | |||
V30 Gy | 30.77 ± 6.50% | 0 (0%) | 0 (0%) |
V45 Gy | 15.48 ± 3.69% | 0 (0%) | 0 (0%) |
V50 Gy | 6.60 ± 2.51% | 0 (0%) | 0 (0%) |
V54 Gy | 0 ± 0% | 0 (0%) | 0 (0%) |
Bladder wall | |||
V30 Gy | 25.04 ± 7.65% | 0 (0%) | 0 (0%) |
V50 Gy | 13.93 ± 4.13% | 0 (0%) | 0 (0%) |
Small bowel | |||
V45 Gy | 0 ± 0 ml | 0 (0%) | 0 (0%) |
Large bowel | |||
V48 Gy | 0 ± 0 ml | 0 (0%) | 0 (0%) |
SD = standard deviation, CTV = clinical target volume, PTV = planning target volume, Dx = dose delivered to x% of volume, VxGy = percentage of volume receiving x Gy, or the volume receiving x Gy.
Structure . | Mean ± SD . | Minor violation . | Major violation . |
---|---|---|---|
CTV | |||
D2 | 56.05 ± 0.72 Gy | 0 (0%) | 0 (0%) |
D98 | 52.82 ± 0.44 Gy | 0 (0%) | 0 (0%) |
PTV | |||
D2 | 56.04 ± 0.38 Gy | 0 (0%) | 0 (0%) |
D50 | 54.35 ± 0.23 Gy | 0 (0%) | 0 (0%) |
D95 | 51.44 ± 0.48 Gy | 8 (33%) | 0 (0%) |
Rectal wall | |||
V30 Gy | 30.77 ± 6.50% | 0 (0%) | 0 (0%) |
V45 Gy | 15.48 ± 3.69% | 0 (0%) | 0 (0%) |
V50 Gy | 6.60 ± 2.51% | 0 (0%) | 0 (0%) |
V54 Gy | 0 ± 0% | 0 (0%) | 0 (0%) |
Bladder wall | |||
V30 Gy | 25.04 ± 7.65% | 0 (0%) | 0 (0%) |
V50 Gy | 13.93 ± 4.13% | 0 (0%) | 0 (0%) |
Small bowel | |||
V45 Gy | 0 ± 0 ml | 0 (0%) | 0 (0%) |
Large bowel | |||
V48 Gy | 0 ± 0 ml | 0 (0%) | 0 (0%) |
Structure . | Mean ± SD . | Minor violation . | Major violation . |
---|---|---|---|
CTV | |||
D2 | 56.05 ± 0.72 Gy | 0 (0%) | 0 (0%) |
D98 | 52.82 ± 0.44 Gy | 0 (0%) | 0 (0%) |
PTV | |||
D2 | 56.04 ± 0.38 Gy | 0 (0%) | 0 (0%) |
D50 | 54.35 ± 0.23 Gy | 0 (0%) | 0 (0%) |
D95 | 51.44 ± 0.48 Gy | 8 (33%) | 0 (0%) |
Rectal wall | |||
V30 Gy | 30.77 ± 6.50% | 0 (0%) | 0 (0%) |
V45 Gy | 15.48 ± 3.69% | 0 (0%) | 0 (0%) |
V50 Gy | 6.60 ± 2.51% | 0 (0%) | 0 (0%) |
V54 Gy | 0 ± 0% | 0 (0%) | 0 (0%) |
Bladder wall | |||
V30 Gy | 25.04 ± 7.65% | 0 (0%) | 0 (0%) |
V50 Gy | 13.93 ± 4.13% | 0 (0%) | 0 (0%) |
Small bowel | |||
V45 Gy | 0 ± 0 ml | 0 (0%) | 0 (0%) |
Large bowel | |||
V48 Gy | 0 ± 0 ml | 0 (0%) | 0 (0%) |
SD = standard deviation, CTV = clinical target volume, PTV = planning target volume, Dx = dose delivered to x% of volume, VxGy = percentage of volume receiving x Gy, or the volume receiving x Gy.
The median follow-up was 31 months (range, 24–42 months). No Grade ≥3 acute toxicity was observed, and the incidence rates of Grade 2 acute genitourinary (GU) and gastrointestinal (GI) toxicities were 21% (five patients) and 4% (one patient), respectively. Details of the acute toxicities are shown in Table 4. In most cases, acute toxicities were noted 2–3 weeks after the start of radiotherapy. To date, no Grade ≥2 late toxicity has been observed. The BRFS rate was 95.8% at 2 years (Fig. 1), and no clinical failure has been detected. One patient failed biochemically at 15 months. The nadir PSA level of this patient was 0.302 ng/ml at 4 months, and the serum PSA level increased to 5.96 ng/ml at 18 months. This increase in serum PSA level was counted as a biochemical failure. However, the PSA level fell to 1.12 ng/ml after 12 months without any treatment, so this transient PSA elevation was considered a benign PSA bounce, not a tumor recurrence. PSA bounce >0.4 ng/ml was observed in 11 patients (46%; including the previous patient).
Overall GU Grade ≥ 2 | 5 (21%) |
Urinary frequency | 2 (8%) |
Urinary retention without catheterization | 2 (8%) |
Urinary tract pain | 1 (4%) |
Overall GI Grade ≥ 2 | 1 (4%) |
Proctitis | 1 (4%) |
Diarrhea | 1 (4%) |
Overall GU Grade ≥ 2 | 5 (21%) |
Urinary frequency | 2 (8%) |
Urinary retention without catheterization | 2 (8%) |
Urinary tract pain | 1 (4%) |
Overall GI Grade ≥ 2 | 1 (4%) |
Proctitis | 1 (4%) |
Diarrhea | 1 (4%) |
GU = genitourinary toxicities, GI = gastrointestinal toxicities.
Overall GU Grade ≥ 2 | 5 (21%) |
Urinary frequency | 2 (8%) |
Urinary retention without catheterization | 2 (8%) |
Urinary tract pain | 1 (4%) |
Overall GI Grade ≥ 2 | 1 (4%) |
Proctitis | 1 (4%) |
Diarrhea | 1 (4%) |
Overall GU Grade ≥ 2 | 5 (21%) |
Urinary frequency | 2 (8%) |
Urinary retention without catheterization | 2 (8%) |
Urinary tract pain | 1 (4%) |
Overall GI Grade ≥ 2 | 1 (4%) |
Proctitis | 1 (4%) |
Diarrhea | 1 (4%) |
GU = genitourinary toxicities, GI = gastrointestinal toxicities.
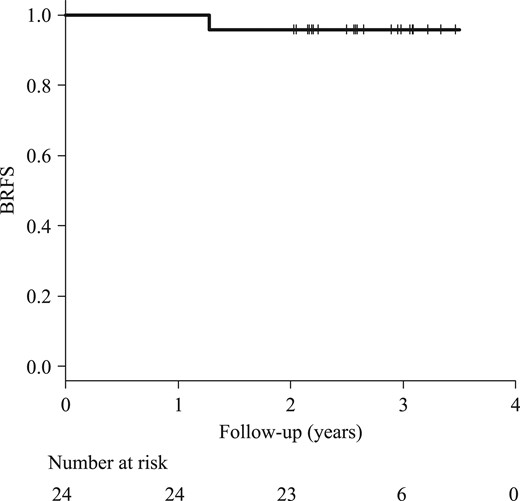
Kaplan–Meier curve of the BRFS rate. The BRFS rate at 2 years was 95.8%. One patient failed biochemically at 15 months, but his PSA level dropped naturally to 1.12 ng/ml. This increase in PSA was considered a benign PSA bounce. BRFS = biochemical relapse-free survival, PSA = prostate-specific antigen.
DISCUSSION
Patients with prostate cancer are not likely to die of the prostate cancer, but likely to die of other cancers or non-cancer cause [13]; therefore, reduction of treatment-related toxicities is a key in the prostate cancer treatment. In this trial, no Grade ≥3 acute toxicity was observed, and the incidence rates of Grade 2 acute GU and GI toxicities were 21% and 4%, respectively. To date, no Grade ≥2 late toxicity has been identified. To the best of our knowledge, only two published trials used close to the number of fractionations as in our trial [12, 14]. In one trial, which was a Phase 1/2 trial, 111 and 135 patients were treated with IMRT, delivering 58.06 Gy in 16 fractions (EQD2 = 85.1 Gy) and 64.7 Gy in 12 fractions (EQD2 = 85.5 Gy), respectively. The Grade ≥2 acute GU and GI toxicities were 20–30% and 4–9%, almost the same as in our trial. However, late rectal bleeding was reported in 8% of cases at 2 years, which was not observed in our trial. An excessive dose increase was considered the cause of the higher late toxicity rate. The other trial was a Phase 2 trial using 3DCRT delivering 55 Gy in 16 fractions (EQD2 = 77.6 Gy). The Grade ≥2 acute GU and GI toxicities were 44% and 38%, respectively, and Grade ≥2 late GU and GI toxicities were 12% and 6% at 2 years, respectively. We believe that the higher toxicity rates resulted from the use of 3DCRT without intensity modulation.
Two major hypofractionated radiotherapy approaches for prostate cancer—moderate hypofractionation (2.4–3.4 Gy per fraction) and extreme hypofractionation (also called ultrahypofractionation or stereotactic body radiation therapy; ≥5 Gy per fraction)—should be considered separately. A published Phase 3 trial showed that acute Grade ≥2 GU and GI toxicity rates were 27–60.5% and 10.5–42%, and Grade 3 GU and GI toxicity rates were 3.3–20.3% and 0.6–5.7%, respectively, after moderately hypofractionated IMRT [15, 16]. Based on the trials, IMRT delivering 60 Gy in 20 fractions or 70 Gy in 28 fractions (EQD2 = 77.1–80.2 Gy) was not inferior to conventionally fractionated IMRT in terms of efficacy or toxicity. The results of our trial are consistent with these other trials. However, no published Phase 3 trial data on extremely hypofractionated IMRT with a long follow-up are available to date. The largest available dataset is a pooled analysis from the USA [17], and it shows 5-year BRFS rates of 95% and 83% in low- and intermediate-risk patients, respectively. Another systematic review reported that acute and late toxicities after extreme hypofractionation are relatively more common than in a historical cohort of conventional fractionation [18].
Both types of hypofractionation have advantages and disadvantages. The advantages of moderate hypofractionation include the substantial evidence from many Phase 3 trials. A disadvantage is the overall treatment time, as it takes 4–6 weeks to complete the treatment. This is less than conventional fractionation, but is much longer than other treatment modalities, such as surgical resection and brachytherapy.
Extreme hypofractionation has a short treatment duration comparable with the other treatment modalities. However, a disadvantage of extreme hypofractionation is the tendency for random errors (including an interplay effect) due to the small fraction number. Extreme hypofractionation requires the implantation of intraprostatic fiducial markers for image guidance or target tracking to compensate for errors, which is an invasive procedure with a risk of infection and bleeding. All patients enrolled in our trial refused implantation of the fiducials, and we assume they expected EBRT to be non-invasive. If clinicians want to treat patients with low- or intermediate-risk prostate cancer invasively, they should perform brachytherapy, not EBRT. Furthermore, implantation should be done 1–2 weeks before the simulation, so it takes 3–5 weeks between implantation and the completion of treatment.
Another disadvantage of extreme hypofractionation is the uncertain applicability of high-dose radiation to the LQ model. Hypofractionated radiotherapy for prostate cancer is based on the hypothesis that the LQ model is applicable to radiotherapy with a high dose per fraction. However, Puck et al. demonstrated the inapplicability of the LQ model for high-dose regions 60 years ago [19], and no conclusion has been reached concerning whether the LQ model fits cases with a large dose per fraction. The linear-quadratic-linear (LQL) model by Guerrero and Li [20, 21] fits the cell survival curve at high doses better than the LQ model. We plotted relationship curves for fraction size and biological effective dose (BED) to normal tissue based on the LQL and LQ models when the BED to the prostate cancer was constant (α/β value in prostate cancer = 1.5 Gy, α/β value in late responding tissue = 3 Gy; Fig. 2). Although the two curves almost overlapped each other by ≤4 Gy, the BED based on the LQL model increased by >4 Gy. In other words, late toxicities might increase in extreme hypofractionation compared with conventional fractionation.
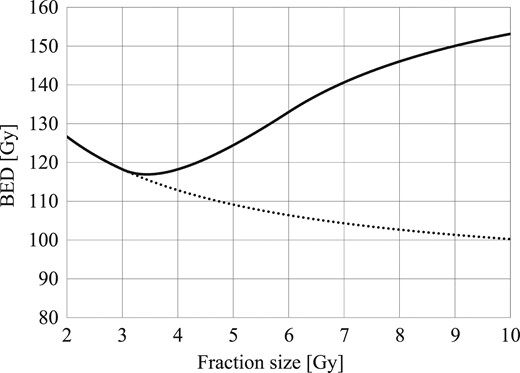
The BED to normal tissue based on the LQ model (plotted as a dotted line) and the LQL model (plotted as a solid line) if the BED to the prostate cancer was constant (based on 76 Gy in 2 Gy per fraction). The two curves almost overlapped each other by ≤4 Gy, but the discrepancy increased above 4 Gy. BED = biological effective dose, LQ = linear-quadratic, LQL = linear-quadratic-linear.
There is a large gap between the two hypofractionation approaches, and our highly hypofractionated IMRT approach falls within this gap. First, it delivered 3.6 Gy × 15 fractions over 3 weeks; this treatment duration was shorter than that of moderate hypofractionation and comparable with that of extreme hypofractionation, which requires 3–5 weeks from implantation of the fiducial markers to completion of the treatment. Second, the number of fractions was large enough to compensate for random errors; therefore, it did not need a fiducial marker. Image guidance was performed using CBCT, which is less invasive than extreme hypofractionation. Finally, the BED discrepancy between the LQ and LQL models was small for 15 fractions, and the BED to normal tissue was less than that of conventional fractionation based on the LQ and LQL models. Zaorsky showed a plateau of the dose–response sigmoidal curve in a meta-analysis [2], and the BED of our highly hypofractionated IMRT approach was likely at the plateau.
In our trial, the 2-year BRFS was 95.8%. One patient failed biochemically, but the higher PSA level decreased without any treatment, so the transient elevation was considered to be a PSA bounce. A transient increase in serum PSA, known as a PSA bounce, is common not only after brachytherapy but also after EBRT for prostate cancer [22–24]. The mechanism of the PSA bounce is unclear, but possibilities include bacterial and/or radiation proctitis, recent ejaculation, instrumentation, and bicycle riding [25]. The definition of PSA bounce is not fixed, with different thresholds within the serum PSA range of 0.1–0.5 ng/ml [26, 27]. In a multi-institutional pooled analysis, Horwitz et al. showed that the rate of PSA bounce >0.4 ng/ml was 20% in 4839 patients who received conventionally fractionated EBRT for prostate cancer [22]. Other reports have shown that PSA bounce rates are 16–51% in SBRT [17, 28–30] and 23.9–46% in brachytherapy [31]. In this trial, PSA bounce >0.4 ng/ml was observed in 11 patients (46%); this rate was more similar to that for SBRT and brachytherapy than that for EBRT. Anwar et al. reported that the incidence of PSA bounce is higher in patients treated with SBRT than in those treated with conventionally fractionated IMRT [32]. Therefore, PSA bounce may occur more frequently in patients undergoing highly hypofractionated IMRT than conventionally fractionated IMRT.
A limitation of our study is that it was a pilot study. In this report, the median follow-up period was 31 months, which is too short to draw conclusions about late toxicities and tumor control outcomes; therefore, a longer follow-up is needed. Furthermore, men with high-risk disease were not included in this study; thus, the results should not be applied to men with high-risk disease. The efficacy of hypofractionated IMRT for treating high-risk disease has not been adequately shown. Therefore, extreme caution should be taken when delivering highly hypofractionated IMRT to high-risk disease patients.
In conclusion, highly hypofractionated IMRT delivering 54 Gy in 15 fractions over 3 weeks, with a daily correction of the prostate position (without any intraprostatic fiducial markers) is feasible in patients with localized prostate cancer. This approach should be evaluated in a Phase 2 trial. A benign PSA bounce >0.4 ng/ml may occur more frequently than with conventionally fractionated IMRT.
ACKNOWLEDGEMENTS
The authors wish to thank Ms Keiko Furukawa for her assistance in data management. This work was presented at the 2018 Genitourinary Cancers Symposium of the American Society of Clinical Oncology, 8–10 February 2018, at Moscone West Building, San Francisco.
Trial Registration: UMIN000012057, registered 17 October 2013, https://upload.umin.ac.jp/cgi-open-bin/ctr_e/ctr_view.cgi?recptno=R000014072.
CONFLICT OF INTEREST
Takashi Mizowaki received a research donation from Mitsubishi Heavy Industries. The other authors report no conflicts of interest with regard to this manuscript.
FUNDING
This work was supported by Grants-in-Aid for Scientific Research from the Ministry of Education, Culture, Sports, Science, and Technology, Japan [Grant nos. 24591838 and 16K10390].