-
PDF
- Split View
-
Views
-
Cite
Cite
Xi Luo, Loralee Larios, Carla D’Antonio, Xiaohong Xu, Hui Guo, An invading annual plant benefits less from soil biota and has reduced competitive power with a resident grass, Journal of Plant Ecology, Volume 14, Issue 5, October 2021, Pages 945–958, https://doi.org/10.1093/jpe/rtab050
- Share Icon Share
Abstract
Interactions between plants and their soil biota, arbuscular mycorrhizal fungi (AMF) in particular, may play a vital role in the establishment and the range expansion of exotic plants in new environments. However, whether there are post-introduction shifts in dependence on AMF and how dependency interacts with competition remains poorly understood.
We conducted a common garden greenhouse experiment to examine how native (USA) and invasive (China) populations of the plant species Plantago virginica, respond to soil biota, and whether these responses change in the presence of a competitor.
We found that while native populations consistently had a higher AMF colonization rate and benefited from AMF in both biomass and seed production, invasive populations received less benefit from AMF, and even showed reduced biomass with AMF in the presence of a competitor. This low mycorrhizal dependency in invasive populations correlated with greater suppression by an indigenous competitor for the invader. The different responses of the invasive and native populations to AMF suggest that alteration of mycorrhizal dependency has occurred during the invasion of P. virginica into China. Our findings suggest that this reduced dependency incurs a cost during interspecific competition.
摘要
植物与土壤生物,特别是与丛枝菌根真菌(AMF)的关系,可能对外来植物在新环境中的建立和扩张发挥着至关重要的作用。但是,植物对AMF的依赖是否会在入侵后发生变化,及其如何影响与本 地物种的竞争仍然知之甚少。通过同质园实验,我们研究了入侵物种北美车前(Plantago virginica)的原产地(美国)和入侵地(中国)种群对AMF的响应,以及在有无竞争者的情况下这些响应是否发生变化。研究结果显示,原产地种群始终具有较高的AMF侵染率,并且其生物量和种子产量都受益于AMF。不同的是, 入侵地种群从AMF中获得的收益较少,甚至在存在竞争者的情况下,AMF的侵染使得入侵种群的生物量有所降低。入侵种群的这种低菌根依赖度可能与受到本地竞争者的更大抑制作用有关。北美车前入侵地和原产地种群对AMF的不同响应表明,其对菌根真菌的依赖性在入侵中国的过程中发生了改变。我们的发现表明,这种减少的依赖性会使入侵植物在种间竞争中付出一定的代价。
INTRODUCTION
Plants can change soil abiotic and biotic properties (e.g. soil-borne pathogens, beneficial symbionts) that in turn impact plant’s subsequent performances either negatively or positively arising plant–soil feedbacks (PSFs). PSFs have widely been used for explaining vegetation dynamics, community succession as well as plant invasion (Bever 1994; Callaway et al. 2004; van der Putten et al. 2013, 2016). A large body of studies has shown that soil biota exerted profound effects on the success of introduced exotic species in new habitats (Bever 1994; Levine et al. 2004; Pringle et al. 2009; Revillini et al. 2016; Suding et al. 2013). Specifically, positive PSFs are expected to increase invasive plants’ competitive ability to natives, fitness and relative abundance in introduced communities (Aldorfová et al. 2020; Inderjit and van der Putte 2010; Klironomos 2002; Pringle et al. 2009; Shelby et al. 2016).
As one of the most ubiquitous and important groups of mutualists (Davison et al. 2015; Smith and Read 2008), arbuscular mycorrhizal fungi (AMF) may play a decisive role in the establishment and range expansion of plants in new environments (Callaway et al. 2004; Inderjit and van der Putten 2010; Mitchell et al. 2006; Wardle et al. 2004). Invading plants are likely to form new mycorrhizal associations in the invasive range due to the low host-specificity of most AMF. These new relationships may facilitate establishment and range expansion of invasive species if they benefit from the AMF more than the native species (i.e. enhanced mutualisms, Reinhart and Callaway 2006). Conversely, if the invading plants are less dependent on AMF, they may reduce the abundance or efficacy of AMF for the resident native species (i.e. degraded mutualisms, Hartnett et al. 1999, Klironomos 2003; Vogelsang et al. 2009). These relationships with AMF may influence the outcome of competitive interactions between invader and resident species (Waller et al. 2016). However, we lack empirical tests of how AMF influences competition between invaders and resident native species.
Despite the growing research focus on AM associations and invasions, there is mixed evidence for shifts in mycorrhizal dependency (the growth or seed production benefit gained by associating with mycorrhizal fungi) during species invasions (Bunn et al. 2015; Mitchell et al. 2006; Pringle et al. 2009). Biogeographic studies comparing invading populations to native populations or evaluating among population variation in AMF dependency in the invaded range, are a powerful approach for understanding how the biotic and abiotic environment affect the role of AMF in invasions. Seifert et al. (2009) first reported an evolutionary change of mycorrhizal dependency during plant invasion by comparing native and invasive populations of Hypericum perforatum. They found that introduced North American populations responded less to inoculation with AMF than European populations, suggesting a genetically based reduction of mycorrhizal dependence. Reductions in mycorrhizal dependency during invasion may be an important advantage for species entering habitats where rapid growth or aboveground investment is critical, but the prevalence of this phenomenon is unknown.
Mycorrhizal dependency can have implications for competitive interactions between invasive and native plants. Waller et al. (2016) found that an individual’s biomass of native populations of Centaurea solstitialis was suppressed more by inter-specific competitors than those from invasive populations, when AMF were present. This suggests that a shift toward less mycorrhizal dependence by invading populations can increase their competitiveness, potentially by investing relatively more photosynthate into aboveground plant parts (Bunn et al. 2015; Pringle et al. 2009; Richardson et al. 2000). This might occur when nutrients are abundant, and rapid growth is important. By contrast, invasive species entering stressful environments (e.g. nutrient or water-limited), could benefit from enhanced association with AMF fungi via increased rates of resource acquisition or enhancement of survival during drought (Liu et al. 2013). Annual plants invading water stressed environments, however, may be selected to grow very rapidly when water is available selecting against mycorrhizal association (Haubensak et al. 2014). Thus, the strength and direction of plant–soil interactions may be altered by soil conditions and competition among plant species (Crawford et al. 2017; Sun et al. 2014). Despite the potential importance of AMF and their potential influence on growth and competitive interactions, AMF dependency and influence on competitive interactions and allocation during invasion needs further investigation.
In this study, we evaluated whether the invasion of Plantago virginica in China has resulted in a shift in mycorrhizal dependency from its native North American origin and how this mycorrhizal dependency may influence competitive interactions with a native species from China. We asked the following questions: (i) Do P. virginica plants from the introduced versus native origins differ in their mycorrhizal dependency? and (ii) Does dependency change when a competitor is present and is this response origin specific? We hypothesized two possible scenarios of AMF dependence strategies of the invasive plant P. virginica in competition with resident competitors when invading new regions (Fig. 1). Scenario ①: Under fertile environments in an invasive region, the invasive populations of P. virginica allocate more carbon (i.e. photosynthate) to aboveground parts than to belowground (root and AMF) parts. In this case, the AMF dependence would decrease, and the aboveground biased carbon allocation may help plants take advantage of light resource more efficiently and then outcompete with resident competitors. Scenario ②: Under unfertile environments, the invasive populations of P. virginica would be associated with AMF more closely to compete nutrients with resident competitors. In this case, more carbon is expected to allocate into belowground parts (root and AMF) leading to a relatively weak competition ability. In a competition experiment in which plants were grown with and without AMF, we measured growth and reproduction of plants from the native and invaded origins, in order to further understand the mechanisms by which AMF and competition mediate the invasion process. We also measured plant nutrient status across all treatments.
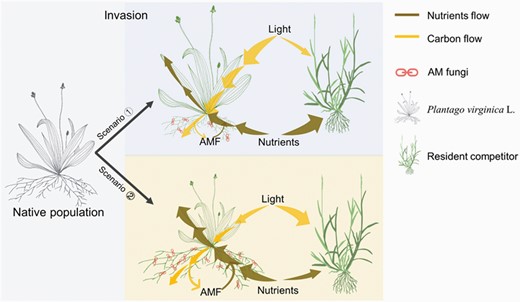
Conceptual model showing two possible scenarios of AMF dependence strategies of the invasive plant Plantago virginica in competition with resident competitor when invading new regions. Scenario ①: Under fertile environments in an invasive region, the invasive populations of P. virginica allocate more carbon (i.e. photosynthate) to aboveground parts than to belowground (root and AMF) parts. In this case, the AMF dependence would decrease, and the aboveground biased carbon allocation may help plants take advantage of light resource more efficiently and then outcompete with resident competitors. Scenario ②: Under unfertile environments, the invasive populations of P. virginica would be associated with AMF more closely to compete nutrients with resident competitors. In this case, more carbon is expected to allocate into belowground parts (root and AMF) leading to a relatively weak light competition ability.
MATERIALS AND METHODS
Study species
Plantago virginica is a winter annual herb native to North America where it has a wide geographic range. It was first reported in southeastern China in 1951 and has since rapidly spread throughout abandoned farmland, roadsides and residential areas with high densities (Guo et al. 1996). Over time, it has invaded the lawns of cities in southeast China where it is considered a weed. Controlling it is expensive and labor intensive (Wang et al. 2015). Festuca elata Keng ex E. Alexeev, a native (to China) cool-season perennial C3 grass, is a common lawn species and a competitor of P. virginica in the introduced origin.
Seed collection and soil preparation
Seeds of P. virginica were collected in 2012 from three populations each within its native (USA) and invasive origins (China) distributed along a latitudinal gradient (Supplementary Table S1). For each population, we collected seeds from a minimum of 30 individuals that were chosen to represent different maternal families along 10 linear transects (100 m apart). The seeds of F. elata were collected from College of Agro-Grassland Science, Nanjing Agriculture University. In October 2016, seeds of six populations of P. virginica and F. elata were pre-germinated under 20 oC and 12 h daylight for 2 weeks. We sterilized the surface of the seeds with 75% ethyl alcohol before germination.
We collected soils from the same invaded sites where P. virginica seeds were collected in China. At each site, soil from three separate locations was collected to a depth of approximately 15 cm after removing surface litter. We sieved the soil through a 5-mm sieve to remove rocks, roots and visible soil faunas. After sieving, 20 g of soil from each site was used set aside for soil properties measurements (Supplementary Table S2). A quarter of the remaining soil was stored at 4 °C for the live soil microbe inoculum and isolation of filtrate in the greenhouse experiment. The remaining soil was sterilized by γ-irradiation (60Co-γ, 40 kGy). This procedure can kill the initial soil biota and allow keeping soil structure and nutrient levels similar among treatments (Zuppinger-Dingley et al. 2011).
Experimental design
To evaluate changes in AMF dependency in the invasive and native populations of P. virginica, we applied factorial treatments of AMF presence/absence and competition (grown with one F. elata or partitioned by a solid barrier, Supplementary Fig. S1). This yielded a total of 24 treatment combinations (2 origins × 3 populations × 2 AMF × 2 competition) in a randomized block design. Each treatment had 30 replicates, which were randomly arranged in six blocks. We used the same size pots for all treatments. In each pot, one P. virginica and one F. elata were planted. For the treatment without competition, a piece of plastic film was used to separate the roots of the two individuals. In total we planted 720 pots. Each plastic pot (top diameter: 11.3 cm × bottom diameter: 8 cm × height: 9.5 cm) was filled with 1 kg of a 1:1 mix (by volume) of sterilized soil from the three sites and sand.
For the +AMF treatment, to maximize the role of the AMF community and simulate the field conditions, 20 g of live inoculum (i.e. unsterilized field soil) was added directly to each pot during transplantation. Because soils were only collected in the invasive origin, the only inoculum available was from the invasive origin. All three soils were used as inoculum for all six seed populations so of the 30 pots per treatment, there were 10 each from each of the three inoculum sources. For the −AMF treatment, the live soil inoculum was replaced by 20 g sterilized soil. To correct for possible differences in microbial communities, we added 40 mL of soil filtrate to the sterile treatments, corresponding to the soil collection sites. The 40 mL was split 20/20 in the pots with the barriers. The soil filtrates were prepared by blending live soil and water in a 1:2 ratio and passing the soil slurry through a 25-μm sieve. The relatively large AM fungal spores were trapped by the sieve, whereas smaller organisms could pass through, allowing us to add the majority of soil microbes (Johnson et al. 2010). The limitation of this method is that the large size microbes and soil fauna such as pathogens or nematodes cannot be included in soil filtrate. However, we considered the indirect effect of decomposition and nutrients release of soil fauna could be ignored in our short-term and non-nutrient limited microcosms. As for the pathogen effects, the strong effect of this treatment (see results) suggests that this does not affect our main results.
We conducted the experiment in a greenhouse at Pailou Teaching Research Base, Nanjing Agricultural University (32°1′25.7ʺ N, 118°51′48.1″ E). Pots in each block were rotated weekly to eliminate the potential effect of spatial heterogeneity in light and temperature in the greenhouse. Plants were watered every 2 days or as needed. After 2 weeks, we fertilized each pot with 10 mL nutrient solution (5 mL nutrient solution to each cell in the pots that were separated by the barrier) at a 2-week interval to ensure that nutrients other than phosphorus (P) were not limited. The nutrient solution was a diluted Hoagland’s solution (25% strength of the full Hoagland’s solution, 52.5 ppm of NO3-) without P. We applied 10 mL nutrient solution every 2 weeks.
Measurements
The experiment began on 1 December 2016 and lasted 5 months. We measured both vegetative and reproductive traits at two different time points during the experiment, each time by harvesting 15 randomly selected individuals per treatment. The first sampling was carried out 12 weeks after transplanting when the plants were still in a vegetative stage. For this sampling, we measured each plant’s above-ground biomass and AMF colonization of roots. The second sampling was at the end of April when most seeds of P. virginica were mature. For the second sampling, we also recorded the number and length of inflorescences. At the second sampling, the infructescences with mature seeds of the P. virginica individuals were cut and packed separately into paper envelopes.
The harvested plants were dried to a constant mass at 65 °C for 48 h before weighing to the nearest 0.0001 g. To determine AMF colonization, we carefully washed the roots, stained one hundred 1 cm root segments from each root sample (from five replicates for each treatment) with trypan blue and quantified colonization using a standard grid-line intersect method (Mcgonigle et al. 1990).
We determined the nitrogen (N) and phosphorus (P) of the above-ground tissue using wet digestion with sulfuric acid and hydrogen peroxide (a modified Kjeldahl procedure, 1 h at 200 °C and 2 h at 340 °C). N concentrations were determined with a continuous-flow autoanalyzer (Skalar San++, Holland), while P concentrations were determined on the digest using the molybdate-blue method. We calculated the total N and P (g) in biomass by multiplying the %N and %P concentrations by the corresponding biomass (g).
Statistical analyses
Nested three-way ANOVAs were used to detect the effects of origin, AMF treatment, competition treatment and their interactions on root colonization, plant growth and reproductive traits. Population was nested within origin. Inoculum source was not a significant contributor to variation, so it is not considered further. Simple linear regressions were used to assess how aboveground biomass of P. virginica related to AMF colonization rate. Aboveground biomass was natural log-transformed to meet normality.
We calculated a proportional growth response to AMF for native and invasive origin populations and then compared them. To do this we calculated a Relative Interaction Intensity (RII) Index (Waller et al. 2016) of aboveground biomass response to AMF as:
Means were calculated from 15 individuals of each population under each competition treatment at the first sampling. We similarly calculated the RII of reproductive output after the second sampling. RII ranges from −1 (negative effect) to +1 (positive effect). The significance was assessed using Nested two-way ANOVAs to compare the responsiveness of P. virginica to AMF between origins, competition treatments and their interactions. Population was nested within origin. All analyses were performed in JMP version 7.0 (SAS Institute Inc., Cary, NC, USA).
RESULTS
AMF colonization
For all populations, roots had higher AMF colonization rates in +AMF soil than in −AMF soils (Table 1; Fig. 2). Under +AMF treatment, AMF colonization rates were positively related to plant aboveground biomass when competitors were either absent or present (P = 0.02 and 0.08, Supplementary Fig. S5). For the −AMF treatment, the aboveground biomass was not related to AMF colonization rate independently of the presence of competitor (P = 0.93 and 0.19, Supplementary Fig. S5). Some differences were found among populations in colonization rates within each origin (Table 1; Supplementary Fig. S3). In the absence of competitors, the variation in the colonization rates among native populations was greater than that of the invasive populations (Supplementary Fig. S3). By contrast, there was a greater difference among the invasive populations when grown with competitors (Supplementary Fig. S3). A significant interaction between origin and AMF treatment was also detected (Table 1), as native populations had 32% higher colonization in +AMF treatments compare to invasive populations but had similar colonization in −AMF treatments. Populations from both origins responded similarly to competition in terms of colonization rate with slightly higher colonization rates in the −AMF treatment plants in the +competition pots compared with −competition, hence there were weakly significant two or three-way interactions involving competition.
Summary of nested three-way ANOVAs results (F-ratios and significance levels) testing the effects of origin, AMF, competition and their interactions on plant traits of P. virginica
Factor . | df . | Colonization rate (%) . | Above ground biomass (g) . | Seed biomass (g) . |
---|---|---|---|---|
Origin | 1 | 51.32* | 63.13* | 0.21 |
Population [Origin] | 4 | 3.54** | 48.83* | 2.53*** |
AMF | 1 | 534.36* | 13.92* | 4.67*** |
Competition | 1 | 29.83** | 9.71** | 14.66** |
Origin × AMF | 1 | 41.75* | 10.05** | 0.71 |
Origin × Competition | 1 | 0.18 | 2.67 | 1.05 |
AMF × Competition | 1 | 2.53 | 7.19** | 1.69 |
Origin × AMF × Competition | 1 | 2.40 | 3.26+ | 1.86 |
Factor . | df . | Colonization rate (%) . | Above ground biomass (g) . | Seed biomass (g) . |
---|---|---|---|---|
Origin | 1 | 51.32* | 63.13* | 0.21 |
Population [Origin] | 4 | 3.54** | 48.83* | 2.53*** |
AMF | 1 | 534.36* | 13.92* | 4.67*** |
Competition | 1 | 29.83** | 9.71** | 14.66** |
Origin × AMF | 1 | 41.75* | 10.05** | 0.71 |
Origin × Competition | 1 | 0.18 | 2.67 | 1.05 |
AMF × Competition | 1 | 2.53 | 7.19** | 1.69 |
Origin × AMF × Competition | 1 | 2.40 | 3.26+ | 1.86 |
Population was nested within origin. Significance levels: *P < 0.05, **P < 0.01, ***P < 0.001, +: marginal significant; no symbol: P > 0.05.
Summary of nested three-way ANOVAs results (F-ratios and significance levels) testing the effects of origin, AMF, competition and their interactions on plant traits of P. virginica
Factor . | df . | Colonization rate (%) . | Above ground biomass (g) . | Seed biomass (g) . |
---|---|---|---|---|
Origin | 1 | 51.32* | 63.13* | 0.21 |
Population [Origin] | 4 | 3.54** | 48.83* | 2.53*** |
AMF | 1 | 534.36* | 13.92* | 4.67*** |
Competition | 1 | 29.83** | 9.71** | 14.66** |
Origin × AMF | 1 | 41.75* | 10.05** | 0.71 |
Origin × Competition | 1 | 0.18 | 2.67 | 1.05 |
AMF × Competition | 1 | 2.53 | 7.19** | 1.69 |
Origin × AMF × Competition | 1 | 2.40 | 3.26+ | 1.86 |
Factor . | df . | Colonization rate (%) . | Above ground biomass (g) . | Seed biomass (g) . |
---|---|---|---|---|
Origin | 1 | 51.32* | 63.13* | 0.21 |
Population [Origin] | 4 | 3.54** | 48.83* | 2.53*** |
AMF | 1 | 534.36* | 13.92* | 4.67*** |
Competition | 1 | 29.83** | 9.71** | 14.66** |
Origin × AMF | 1 | 41.75* | 10.05** | 0.71 |
Origin × Competition | 1 | 0.18 | 2.67 | 1.05 |
AMF × Competition | 1 | 2.53 | 7.19** | 1.69 |
Origin × AMF × Competition | 1 | 2.40 | 3.26+ | 1.86 |
Population was nested within origin. Significance levels: *P < 0.05, **P < 0.01, ***P < 0.001, +: marginal significant; no symbol: P > 0.05.
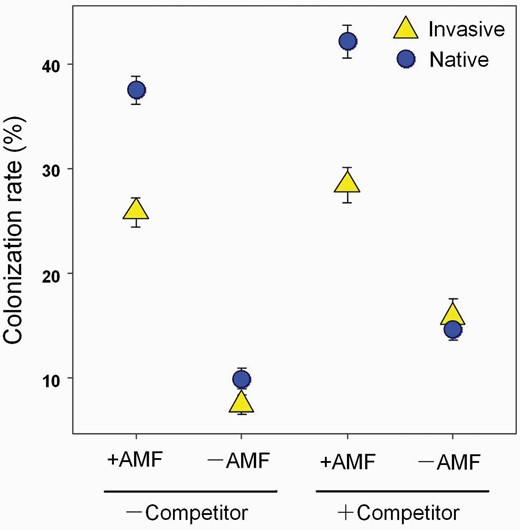
Arbuscular mycorrhizal (AM) colonization rate of P. virginica (Invasive populations, yellow triangles; Native populations, blue dots) under AMF treatment (presence: +AMF or absence: −AMF) and competition treatment (grown with competitor: +competitor or without competitor: −competitor). Values are means ± 1 SE.
Plant responses to AMF and competition
AMF and competition treatments significantly affected the aboveground biomass of P. virginica but the influence varied with origin (Table 1; Fig. 3a). Interactive effects were found between origin and AMF treatment, and between AMF and competition treatments (Table 1). Three-way interaction of origin, AMF and competition was marginally significant (P = 0.07). In the absence of a competitor, the biomass of P. virginica populations from both native and invasive origins increased with +AMF, with the native population benefitting more (Fig. 3a). The invasive population had a larger overall biomass and plants in −AMF were slightly bigger than the native origin plants + AMF (Fig. 3a). Similarly, without competitors, a positive biomass RII was found in both native and invasive populations with native origin plants having a higher RII (Fig. 4a).
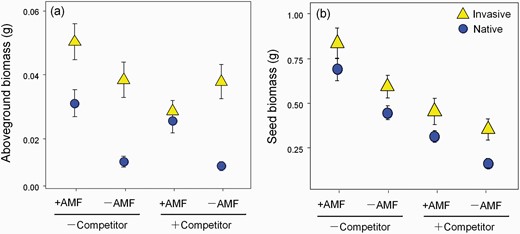
Aboveground biomass (a) and seed biomass (b) of P. virginica (invasive populations, yellow triangles; native populations, blue dots) under AMF treatment (presence: +AMF or absence: −AMF) and competition treatment (grown with competitor: +competitor or without competitor: −competitor). Values are mean ± 1 SE.
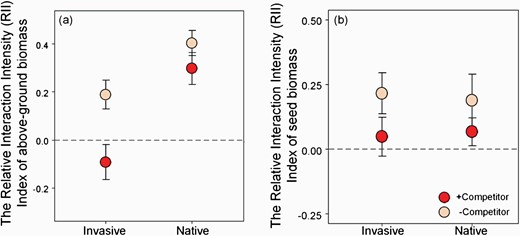
The Relative Interaction Intensity (RII) Index (mean ± SE) of (a) aboveground biomass; (b) reproductive output of invasive (China) and native (USA) populations of P. virginica. Positive values demonstrate that plants received a net benefit from AMF when grown with competitor or without competitor. Values are mean ± 1 SE.
The aboveground biomass of native populations of P. virginica behaved similarly with versus without a competitor (Fig. 3a). However, the increased growth that invasive populations experienced from AMF when grown alone was eliminated when grown with a competitor (Fig. 3a). This differential effect of competition on mycorrhizal responsiveness resulted in a negative RII for invasive populations with competitors but no change for the native populations (Fig. 4a).
Significant differences in aboveground biomass were found among populations within origins (Table 1). Two of the three invasive origin populations (CN and CF, see Supplementary Fig. S4) showed a negative effect of AMF on aboveground biomass when they grew with the competitors (Supplementary Fig. S4b). Two of three native populations (i.e. UG and UF) had a significantly positive influence of AMF on aboveground biomass both with and without competitors (Supplementary Fig. S4a and b).
AMF increased the reproductive output of both native and invasive populations of P. virginica regardless of competitive environment (Fig. 3b). Competition, however, decreased overall reproductive output for both the native and invasive populations, but the invasive populations maintained greater seed biomass in almost all treatments (Fig. 3b). The net reduction in seed biomass in the presence of a competitor translated to a decrease in RII to almost 0 for the invasive populations. There was a decreasing trend in RII for native populations with competition (Fig. 4b). At the population level, all native populations had similar seed production to invasive populations with the exception of the CN population (Supplementary Fig. S6a and b). Independent of competition, seed production of CN responded positively to AMF. Two of three native populations (UN and UF) also showed enhanced seed production with AMF (Supplementary Fig. S6a and b).
AMF and competition effects on plant nutrient acquisition
Both concentration and total amount of N in biomass of P. virginica were significantly affected by origin and competition (Table 2; Fig. 5a and c). Having a competitor reduced the N concentration and N biomass in both native and invasive origin populations (Table 2; Fig. 5a). The invasive populations tended to have a higher average N concentration and the total amount of N than the native populations particularly with competitors (Fig. 5a and c), although origin was not directly tested in our model. AMF treatment did not affect biomass N, but reduced N concentration, which may be indirectly caused by a dilution effect with increasing biomass (Table 2; Fig. 3). The influence of AMF and competition on P concentration varied by origin. In native origin populations, P concentration increased with competition but was not affected by AMF. In invasive origin populations, P concentration was generally low and affected by competition only in the presence of AMF (Fig. 5b). Biomass P of both invasive and native populations benefited from AMF in the absence of competitors (Fig. 5d). This likely reflects increased biomass with AMF without competitors (Fig. 3). Within origins, population showed significant effects on the amount and concentration of N and P (Table 2).
Summary of nested three-way ANOVAs results (F-ratios and significance levels) testing the effects of origin, AMF, competition and their interactions on nitrogen and phosphorus concentration and content in biomass
Factor . | df . | N (%) . | P (%) . | N (g) . | P (g) . |
---|---|---|---|---|---|
Origin | 1 | 6.22* | 52.03** | 24.74** | 3.27+ |
Population [Origin] | 4 | 3.69* | 2.52* | 7.67** | 8.30** |
AMF | 1 | 5.04* | 0.83 | 0.79 | 9.79*** |
Competition | 1 | 12.72** | 27.92** | 56.84** | 32.65** |
Origin × AMF | 1 | 0.67 | 0.70 | 0.71 | 0.032 |
Origin × Competition | 1 | 1.04 | 18.82** | 0.07 | 0.80 |
AMF × Competition | 1 | 1.14 | 2.60 | 0.01 | 4.31* |
Origin × AMF × Competition | 1 | 0.27 | 0.82 | 0.30 | 0.55 |
Factor . | df . | N (%) . | P (%) . | N (g) . | P (g) . |
---|---|---|---|---|---|
Origin | 1 | 6.22* | 52.03** | 24.74** | 3.27+ |
Population [Origin] | 4 | 3.69* | 2.52* | 7.67** | 8.30** |
AMF | 1 | 5.04* | 0.83 | 0.79 | 9.79*** |
Competition | 1 | 12.72** | 27.92** | 56.84** | 32.65** |
Origin × AMF | 1 | 0.67 | 0.70 | 0.71 | 0.032 |
Origin × Competition | 1 | 1.04 | 18.82** | 0.07 | 0.80 |
AMF × Competition | 1 | 1.14 | 2.60 | 0.01 | 4.31* |
Origin × AMF × Competition | 1 | 0.27 | 0.82 | 0.30 | 0.55 |
Population was nested within origin. Significance levels: *P < 0.05, **P < 0.01, ***P < 0.001, +: marginal significant; no symbol: P > 0.05
Summary of nested three-way ANOVAs results (F-ratios and significance levels) testing the effects of origin, AMF, competition and their interactions on nitrogen and phosphorus concentration and content in biomass
Factor . | df . | N (%) . | P (%) . | N (g) . | P (g) . |
---|---|---|---|---|---|
Origin | 1 | 6.22* | 52.03** | 24.74** | 3.27+ |
Population [Origin] | 4 | 3.69* | 2.52* | 7.67** | 8.30** |
AMF | 1 | 5.04* | 0.83 | 0.79 | 9.79*** |
Competition | 1 | 12.72** | 27.92** | 56.84** | 32.65** |
Origin × AMF | 1 | 0.67 | 0.70 | 0.71 | 0.032 |
Origin × Competition | 1 | 1.04 | 18.82** | 0.07 | 0.80 |
AMF × Competition | 1 | 1.14 | 2.60 | 0.01 | 4.31* |
Origin × AMF × Competition | 1 | 0.27 | 0.82 | 0.30 | 0.55 |
Factor . | df . | N (%) . | P (%) . | N (g) . | P (g) . |
---|---|---|---|---|---|
Origin | 1 | 6.22* | 52.03** | 24.74** | 3.27+ |
Population [Origin] | 4 | 3.69* | 2.52* | 7.67** | 8.30** |
AMF | 1 | 5.04* | 0.83 | 0.79 | 9.79*** |
Competition | 1 | 12.72** | 27.92** | 56.84** | 32.65** |
Origin × AMF | 1 | 0.67 | 0.70 | 0.71 | 0.032 |
Origin × Competition | 1 | 1.04 | 18.82** | 0.07 | 0.80 |
AMF × Competition | 1 | 1.14 | 2.60 | 0.01 | 4.31* |
Origin × AMF × Competition | 1 | 0.27 | 0.82 | 0.30 | 0.55 |
Population was nested within origin. Significance levels: *P < 0.05, **P < 0.01, ***P < 0.001, +: marginal significant; no symbol: P > 0.05
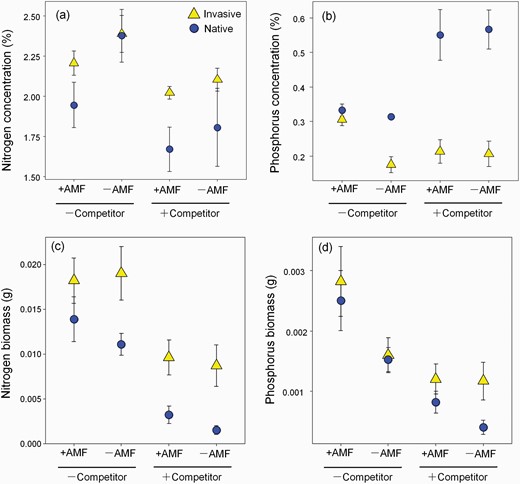
Nitrogen (a) and phosphorus (b) concentration and nitrogen (c) and phosphorus (d) biomass (mean ± SE) of P. virginica (invasive populations, yellow triangles; native populations, blue dots) under competition treatment (grown with competitor: +competitor or without competitor: −competitor). Values are mean ± 1 SE.
When grown without the competitor, the impact of AMF on N and P concentration was inconsistent in the three native populations. Positive, neutral and negative effects were found (Supplementary Fig. S7b). AMF had positive effects on P concentration in invasive populations when grown alone (Supplementary Fig. S7c). Under all treatments, the total biomass N and P of CN population were higher than the biomass N and P of the other two invasive populations respectively (Supplementary Fig. S8). In native populations, UN showed increased total N and P under AMF treatment in the absence of competitors (Supplementary Fig. S8c and d). UF population showed decreased total N and P amount under AMF treatment when grown with the competitors (Supplementary Fig. S8a and b).
DISCUSSION
We observed differential responses of native and invasive P. virginica to AMF and competition in an experimental greenhouse setting (Fig. 3; Table 1): AMF facilitated the growth and reproduction of native populations, with or without competition, but invasive populations only benefited from AMF in the absence of competition (Fig. 3). In the presence of competition, individuals were harmed by AMF (i.e. had lower biomass) (Fig. 3a). Our data suggest that the invasive populations are less dependent on AMF than native populations and are able to attain greater biomass compared with the native regardless of AMF presence as long as competitors are not present. They are however, negatively affected by competition when AMF are present suggesting that somehow the competitors cause AMF to act parasitically on the invaders. The native populations, which are smaller to begin with, appear to be immune to this effect. We also found significant among-population variation in AMF responsiveness within both invaded and native origin plants (Supplementary Figs S4 and S6), suggesting that selective pressures vary among populations. Our results highlight the complex interactions between competition and soil mutualists that could mediate plant invasions and suggest that relatively rapid differentiation can be found among populations of an invasive species. Beyond AMF, we cannot completely rule out the effects of other soil biota such as nematodes or microbial pathogens due to the limitation of our AMF inoculum method (mentioned above). However, AMF root colonization was significantly linked to biomass responses (Supplementary Fig. S5), suggesting that AMF should be a main factor driving observed patterns. The discussion below focus on the AMF effects.
Different responses of native and invasive populations to AMF
Intraspecific comparisons between native and invasive populations allow us to better examine the processes by which a species is able to successfully proliferate in a new origin. Here, we found that both invasive and native populations benefited from AMF when they grew alone, but the plants in invasive populations had lower mycorrhizal colonization (Fig. 2). Yet they grew larger overall supporting the idea that they are more independent of AMF than plants from native populations (Figs 2 and 3). This is consistent with the degraded mutualism hypothesis. Other studies, such as Seifert et al. (2009), similarly demonstrated that introduced populations of an invader exhibited reduced mycorrhizal dependence, and were accompanied by altered biomass allocation patterns. As shown by a previous greenhouse study, invasive populations had a significantly greater shoot and root biomass and lower root: shoot ratios than native populations (Luo et al. 2019). These results suggest that the invasive populations may have been selected for rapid resource acquisition investing relatively more resources into aboveground growth rather than roots and mycorrhiza. As previously reported, invasive species may benefit from the reduced investment in symbiosis when they invade disturbed or interspecific competition-free environments (Cortois et al. 2016; Koziol et al. 2015).
The AMF communities in invaded sites may play a critical role in driving the changes in AMF dependency, because AMF communities are likely to be different from ones in the native origin due to dispersal-limitation of fungi (Andonian et al. 2012; Tedersoo et al. 2014). Also, AMF spore densities may be low at invaded sites since many sites with high invader abundance have experienced frequent disturbance (Vogelsang and Bever 2009) or may be affected by allelochemicals released by other invasive species (Dieng et al. 2015; Mummey et al. 2006). In fact, P. virginica has been reported to have allelopathic potential (Wang et al. 2015). Additionally, increases in available N can cause significant changes in AMF community composition and species richness (Williams et al. 2017). Because the areas where P. virginica has become invasive in China have experienced significant increases in N deposition since the 1980s due to rapid urbanization (Liu et al. 2013; Xu et al. 2015), the increased available N may have reduced the AMF dependence of this invader (Johnson et al. 2003, 2008).
Effects of AMF and competition on N and P acquisition
The +AMF treatment reduced N concentration in plants, but did not affect biomass N, which may be indirectly caused by a dilution effect with increasing biomass (Table 2; Fig. 3). For P concentration, AMF increased the P concentration in invasive populations more than native populations when grown alone, even though plants from invasive populations were less colonized (Fig. 5b). The distinct responses of plant P concentrations to AMF between invasive and native populations may be attributed to their different carbon investments into AMF. Because plants of the native populations are smaller than those of the invasive populations, they were more easily shaded by the competitor F. elata (Supplementary Fig. S2). Moreover, the invasive populations had significantly higher photosynthetic rates than native populations (Luo et al. 2019). Thus, native populations may have less photosynthate to provide to the AMF in exchange for P. Meanwhile, the biomass P of native populations was less sensitive to AMF than invasive populations and was higher with the presence of grass competitor.
Reproductive outputs in both native and invasive populations showed a positive response to the presence of AMF (Figs 3b and 4b). Because seed production is usually a P limited process in many plant species, AMF was likely to increase seed production via increased access to P. In contrast to our results, however, other studies have reported that AMF colonization has no or a negative effect on the reproductive output of hosts (Aguilar-Chama et al. 2012; Nuortila et al. 2004).
AMF did not affect the N and P concentrations when grown with the competitor. This result also indicated that the growth and reproduction of P. virginica facilitated by AMF were not directly reflected by an increased absorption of nutrients (i.e. N, P). The mutualism relationships might enhance the fitness of plants through other non-nutritional functions of mycorrhiza, such as to help plant tolerate with drought (Ruiz-Lozano et al. 2006) or protect them from soil pathogens in introduced region (Borowicz 2001). When plant photosynthates were insufficient, relatively low AMF colonization could benefit plants due to the lesser sources allocation into AMF.
Plant responses to AMF and competition
AMF had positive effects on the vegetative growth of all plants in native populations, whereas they suppressed growth of invasive populations in competition with F. elata (Fig. 3a). Similarly, Waller et al. (2016) found that AMF increased the suppressive effects of the native grass Stipa pulchra on invasive Centaurea solstitialis. In their study, the suppressing effect of the competitor occurred because C. solstitialis was shaded by S. pulchra. Chen et al. (2020) found that higher AMF colonization favored an invasive plant competing with a native plant, but P enrichment changed the association of invasive plants with AMF from mutualism to parasitism. In our study, the negative effects of AMF were only found on the invasive populations of P. virginica. Greater positive effects of AMF on native populations may be partly attributed to the higher AMF colonization rate of native populations than invasive populations. Moreover, species composition of AMF community can also drive the distinct effects. AMF communities consist typically of many species, so it is likely that the combination of F. elata and invasive P. virginica selected for AMF species that were able to parasitize the P. virginica while being beneficial to the grass indirectly (Supplementary Fig. S9), or the grass in the presence of AMF, promoted pathogens that negatively affected P. virginica growth. For native populations, these negative effects may be offset by the positive effect of AMF (Fig. 6).
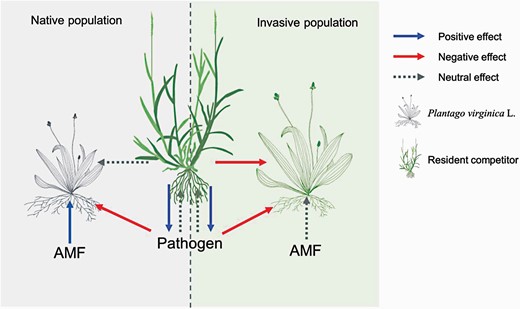
Speculated competition feedback model for invasive and native populations of Plantago virginica. Both invasive and native populations may be adversely affected by pathogens caused by root growth of grass competitors. The positive impact of the native populations from the AMF can offset this pathogens impact, while net neutrality feedback can reduce the suppression of competitors. In contrast, invasive populations were less affected or even negatively affected by AMF, which results in net negative feedback i.e. not conducive to competition.
The association with AMF in P. virginica may have diminished during its invasion into China. Haubensak et al. (2014) demonstrated significant differentiation among populations in ability to form AMF associations in the invasive-origin of Bromus tectorum in North America. In their study, populations from stressful (saline desert) soils had decreased AMF colonization, even when grown in AMF rich soils. These same populations flowered much earlier than plants from less stressful environments suggesting selection for rapid growth to reproduction. In our study, the strategy of resource allocation biased to growth rather symbiosis may help invasive population of P. virginica take advantage of openings/disturbances in otherwise grass dominated sites. As in the Haubensak et al. (2014) study, altered phenology maybe important to take advantage of short lived resources. In our previous experiment (Xu et al. 2019), we found that the invasive populations of P. virginica had earlier germination than native populations. This may contribute to the higher biomass and seed biomass produced by invasive populations (Fig. 3b).
Between population variation in role of AMF
Population as a random factor within origins had significant effects on all responses to AMF and competition treatments (Tables 1 and 2) suggesting that populations in both native and invasive origins are responding to local environmental factors. These include the AMF community, resident competitors, soil properties and climate. Especially, soil conditions may be a key driver of local adaptation in AM symbioses (Haubensak et al. 2014; Johnson et al. 2010). AM associations can origin from strong mutualism to parasitism depending on soil characteristics (Johnson et al. 2010). Here, we found that the soil from CN site had higher P than the other two invaded sites, and aboveground biomass of CN plants did not clearly benefit from AMF. In fact, in this population, AMF reduced growth in the presence of a competitor suggesting that for CN individuals, AMF may be parasitic.
CONCLUSIONS
Understanding the feedback of soil biota to invasive plants, especially the dependence on mycorrhizal fungi in their introduced origins is important for identifying invasion mechanisms and predicting invasion success. Flexibility in AMF association can help a plant population persist in a stressful or highly competitive environment or help plants take advantage of resource pulses where rapid growth is important. Here, we found distinct responses of native and invasive populations to AMF in P. virginica. The invasive populations were less colonized and received less of a benefit from AMF. This low mycorrhizal dependency in invasive populations correlated with greater suppression by an indigenous competitor for the invader. While we do not understand the mechanism through which this suppression occurred, the different patterns between invasive and native populations in response to AMF and competition suggest that genetically based alteration of mycorrhizal dependency is occurring in the introduced origin of P. virginica. Furthermore, other soil biota taxa including pathogens, nematodes or protozoa should be paid more attention in future studies.
Supplementary Material
Supplementary material is available at Journal of Plant Ecology online.
Table S1: Summarize information of the populations of P. virginica focused in the common garden experiment.
Table S2: Soil properties from sampling sties dominated by P. virginica in China (invaded soil, n = 3).
Figure S1: Schematic diagram of experimental design.
Figure S2: Photos of our experimental pots.
Figure S3: Arbuscular mycorrhizal (AM) colonization of P. virginica populations with (a) the absence of competitor and (b) the presence of competitor at +AMF and −AMF treatments.
Figure S4: Aboveground biomass of P. virginica populations with (a) the absence of competitor and (b) the presence of competitor at +AMF and −AMF treatments.
Figure S5: Relationship between aboveground biomass of P. virginica and AMF colocalization rate under (a) the presence of AMF and without competition treatment; (b) the presence of AMF and with competition treatment; (c) the absence of AMF and without competition treatment; (d) the absence of AMF and with competition treatment.
Figure S6: Seed biomass of P. virginica populations with (a) the absence of competitor and (b) the presence of competitor at +AMF and −AMF treatments.
Figure S7: Nitrogen and phosphorus concentration of P. virginica populations with (a, b) the absence of competitor and (c, d) the present of competitor at +AMF and −AMF treatments.
Figure S8: Nitrogen and phosphorus biomass of P. virginica populations with (a, b) the absence of competitor and (c, d) the presence of competitor at +AMF and −AMF treatments.
Figure S9: Aboveground biomass of Festuca elata Keng ex E. Alexeev (grow with invasive populations, yellow triangles; with native populations, blue dots) under AMF treatment (presence: +AMF or absence: −AMF) and competition treatment (root grown with Plantago virginica: +competitor or without Plantago virginica: −competitor).
Funding
This work was supported by the Project of National Natural Science Foundation of China (31971435) and National Key R&D Program of China (2017YFC1200105).
Acknowledgements
We thank Xiaoyi Wang, Qi Wang, Shuang Wang, Zhaoyuan Wang and Kailing Huang for support in the laboratory and greenhouse. Our manuscript was improved by suggestions and comments of Dr Jeffery Diez and Dr Shuijin Hu.
Conflict of interest statement. The authors declare that they have no conflict of interest.
REFERENCES
Inderjit,