-
PDF
- Split View
-
Views
-
Cite
Cite
Miao Liu, Helena Korpelainen, Chunyang Li, Sexual differences and sex ratios of dioecious plants under stressful environments, Journal of Plant Ecology, Volume 14, Issue 5, October 2021, Pages 920–933, https://doi.org/10.1093/jpe/rtab038
- Share Icon Share
Abstract
Dioecious plants exhibit sexual dimorphism in both sexual features (reproductive organs) and secondary sex characteristics (vegetative traits). Sexual differences in secondary traits, including morphological, physiological and ecological characters, have been commonly associated with trade-offs between the cost of reproduction and other plant functions. Such trade-offs may be modified by environmental stressors, although there is evidence that sexually dimorphic responses to stress do not always exist in all plant species. When sexual dimorphism exists, sexually different responses appear to depend on the species and stress types. Yet, further studies on dioecious plant species are needed to allow the generalization of stress effects on males and females. Additionally, sexual dimorphism may influence the frequency and distribution of the sexes along environmental gradients, likely causing niche differentiation and spatial segregation of sexes. At the present, the causes and mechanisms governing sex ratio biases are poorly understood. This review aims to discuss sex-specific responses and sex ratio biases occurring under adverse conditions, which will advance our knowledge of sexually dimorphic responses to environmental stressors.
摘要
雌雄异株植物在性特征(繁殖器官)和次级性特征(植物的特征)均表现出性二态。形态、生理与生态特征等次级性特征的性别差异,通常在繁殖成本和其他功能性状之间存在着权衡。尽管有证据表明性二态对环境胁迫的响应不一定存在于所有植物中,但次级性特征的权衡可能受到环境胁迫的影响。当植物表现出性二态时,不同的物种与胁迫因子可以导致性别特异性的响应。因此,胁迫作用对雌雄异株植物影响的概括性研究是必须的。另外,性二态可能会影响雌雄异株植物沿着环境梯度的频率和分布,引起生态位分化与性别空间分异。目前,控制性别比例偏差的原因和机制还知之甚少。本综述旨在讨论不利环境下的性别特异性响应与性别比例偏差,有利于深入的理解性二态对环境胁迫的响应。
INTRODUCTION
The majority of flowering plants show a hermaphroditic sex expression, while dioecy, i.e. the presence of two separate sexes, is relatively rare. It has been reported that dioecious plants represent 5%–6% of angiosperm species and they occur in 43% of angiosperm families, with 15 600 dioecious angiosperm species in 987 genera and 175 families (Renner 2014). Sex determination systems of flowering plants involving separate sexes have evolved independently many times from hermaphroditism in a lineage-specific manner (Charlesworth 2002; Juvany and Munné-Bosch 2015). Such transitions are often related to the evolution of sexual dimorphism, probably due to maximizing respective female and male fitness (Tonnabel et al. 2017). The incidence of dioecy in flowering plants may represent an evolutionary and ecological advantage.
Dioecious plants exhibit sexual dimorphism, not only in terms of their primary sexual traits but also of their vegetative traits (secondary sexual dimorphism) (Barrett and Hough 2013). Secondary sexual traits involve morphological, physiological and life-history differences between sexes, and they reflect sex-specific adaptations to different resource demands. Sexual dimorphism in secondary sexual traits can be attributable to resource trade-offs between reproduction and other plant functions (e.g. defense and growth) (Galen 2000; Lei et al. 2017). Such resource trade-offs between females and males are probably modified by abiotic and biotic environmental factors during their life cycles, for instance, by heavy metal stress, drought, nutrient deficiency and the presence of pathogens and herbivores (Barrett and Hough 2013; Liu et al. 2020a; Ribeiro-Mendes et al. 2002; Xia et al. 2020).
The theory of resource allocation suggests that reproduction can directly compete with vegetative growth and defense responses when resources are limited (Doust 1989). Therefore, a higher reproductive cost may be detrimental to plant growth and defense in dioecious plants. Generally, females are associated with a greater resource demand to produce flowers and seeds, and they commonly perform worse than males under abiotic stresses (Bañuelos et al. 2004; Leigh and Nicotra 2003), but this pattern is not universal (Juvany and Munné-Bosch 2015). In fact, some studies have shown that the reproductive costs of males may exceed or match those of females due to their high investment in nitrogen-rich pollen in some wind-pollinated plants (Delph 1999; Harris and Pannell 2008; Leigh 2006). Overall, based on previous studies, there is no consistent pattern in the responses of the sexes to environmental stresses, but the responses seem to vary depending on the plant species and the type of stress, but a greater range of studies is needed (Retuerto et al. 2018).
Environmental changes influence secondary sex characteristics of dioecious plants, probably causing biased sex ratios as well (Graff et al. 2013; Petry et al. 2016; Simancas et al. 2018). The sex ratios of dioecious plants are expected to be close to 1:1 when the parental investments producing females and males are equal (Fisher 1930). However, biased sex ratios are commonly found (e.g. Barrett et al. 2010; Bisang and Hedenäs 2005; Pucholt et al. 2017; Sinclair et al. 2012). Male-biased sex ratios are often associated with a long growth period, biotic seed dispersal and fleshy fruits that are prevalent in trees and vines (Field et al. 2013; Sinclair et al. 2012; Wheelwright and Logan 2004). In contrast, female-biased sex ratios are commonly related to clonality and heteromorphic sex chromosomes found in many herbs, annuals and shrubs (Field et al. 2013; Lloyd and Webb 1977).
The evolutionary and ecological mechanisms underlying the biased sex ratios may be attributable to a biased primary sex ratio (Stehlik et al. 2008), mortality differences (Simancas et al. 2018), germination requirements (Stark et al. 2001), sex lability (Mesgaran et al. 2019) and spatial segregation of sexes (Alvarenga et al. 2013). However, no general conclusion is drawn on the relative importance of these mechanisms, depending largely on the plant species and specific environmental factors. Environmental stresses probably impose a threat to plant species and communities, especially in plants with sexually dimorphic breeding systems (Hultine et al. 2016; Varga and Soulsbury 2020). Importantly, highly skewed sex ratios may cause population declines and even mass extinctions, with potential effects on the structure and stability of populations (Petry et al. 2016). Therefore, it is urgent to reveal the effects of environmental changes on dimorphic species.
In this review, we comprehensively analyze how males and females respond to abiotic and biotic stressors, and provide possible causes for sex-related differences in stress responses and tolerance. Firstly, we develop a comprehensive synthesis of sexual differences and adaptive responses under environmental stress conditions (abiotic and biotic stresses). Secondly, we evaluate and discuss the potential causes, processes and consequences of biased sex ratios under changing environmental conditions. Finally, we introduce the role of sexual interactions in responses to environmental factors. Thus, this review will expand our knowledge of the biology of dioecious plant species.
SEXUAL DIFFERENCES IN RESPONSES TO ENVIRONMENTAL STRESSORS
Abiotic stresses
In dioecious plants, differences in reproductive costs and demands for resources between sexes may lead to sex-specific adaptations and responses to environmental constraints or stressors (Fig. 1). Dimorphic investments in reproduction may be reflected in different physiological responses to abiotic stresses in males and females (Fig. 2). Generally, females have higher reproductive costs for the production of flowers, fruits and seeds, i.e. a higher reproductive investment when compared with males. As discussed in the study of Juvany and Munné-Bosch (2015), higher reproductive investment in females may cause a decrease in the energy invested into growth and defense, thus reducing stress tolerance. Indeed, female plants have been reported to perform worse than males under environmental stresses (Bañuelos et al. 2004; Liu et al. 2021a; Pickering et al. 2003; Yu et al. 2020).
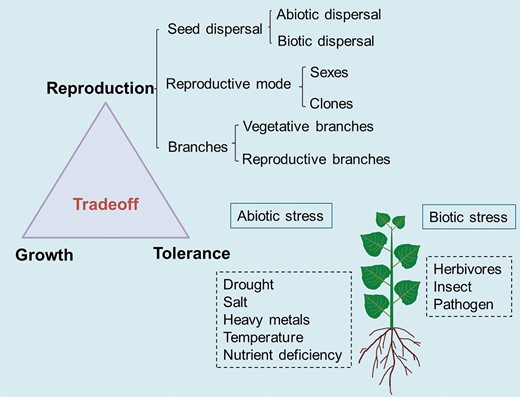
Determining factors of secondary sexual dimorphism in dioecious plants (adapted from Juvany and Munné-Bosch 2015). Secondary sexual dimorphism can be modified by trade-offs among vegetative growth, reproduction and stress tolerance. Sexual differences in reproductive costs determine reproductive fitness, growth and defense. Reproductive costs are affected by the type of seed dispersal, reproduction and branches.
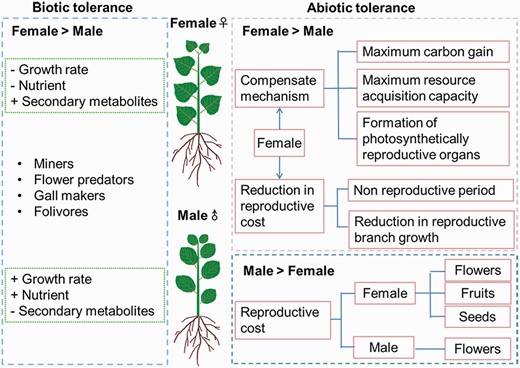
Sexually different tolerances to abiotic stress and biotic stress. Sexual difference in response to biotic stress was adapted from Núñez-Farfán and Valverde (2020). Female > male means that the stress tolerance (abiotic stress and biotic stress) is usually higher in females than in males. A higher biotic stress tolerance in females is often associated with lower rate of growth, lower content of nutrients and higher secondary metabolites. A lower abiotic stress tolerance in females is often associated with higher reproductive costs for producing flowers, fruits and seeds. Male > female means that the stress tolerance (abiotic stress) is usually higher in males than in females. A higher tolerance to abiotic stress in females is usually associated with reduced reproductive costs and compensation mechanisms.
The differential plasticity hypothesis suggests that females and males exhibit sex-specific plasticity in responses to environmental factors, probably associated with the different demands of resource ‘currencies’ (such as carbon and nitrogen) for their reproduction (Delph and Bell 2008; Obeso 2002). Owing to the demands for carbon and water during fruiting and seed setting, females tend to increase water acquisition and photosynthetic carbon gain, which is probably achieved by the high stomatal conductance and hydraulic conductivity (Olano et al. 2017). Such resource acquisition in females easily suffers from hydraulic failure and carbon starvation, potentially as a consequence of higher stomatal sensitivity and more vulnerable xylem cavitation, especially under xeric conditions (Chen et al. 2010; Han et al. 2013; Olano et al. 2017). In contrast, the superior performance of males observed in some studies is associated with the more conservative strategy to maximize abiotic stress tolerance, especially in drier sites. Interestingly, under well-watered conditions, secondary sexual dimorphisms in physiological characters may disappear (Chen et al. 2014).
The ‘functional equilibrium’ of biomass allocation assumes that biomass allocation is associated with the growth-limiting factors. That is, plants would allocate relatively more biomass to roots, if the growth-limiting factors are below ground. However, relatively more biomass would be allocated to shoots, if the growth-limiting factors are above ground (Brouwer 1963; Poorter et al. 2012; Thornley 1972). The great demand for carbon in females may be met by higher photosynthetic rates. Generally, females of dioecious poplars tend to have a higher resource uptake and carbon costs for the root system and shoot growth under sufficient phosphorus and nitrogen supplies (Song et al. 2018; Xia et al. 2020). Conversely, males usually have a higher resource use efficiency and more conservative nutrient demand to maximize stress tolerance and gain opportunities to survive in low-resource habitats (Xia et al. 2020; Zhang et al. 2019).
In Populus cathayana, females have a higher specific root length and root length density to maximize resource uptake, while males have a lower root biomass allocation, as well as a higher antioxidation, acid phosphatase exudation and colonization and hyphal biomass of arbuscular mycorrhizal fungi in response to a heterogeneous phosphorus distribution (Xia et al. 2020; Zhang et al. 2019). Interestingly, the resource consumption traits of females in some species appear not to distinguish ions, which are toxic or necessary for plants. For example, when compared with male poplars, females have a better capacity to acquire nitrogen (Zhang et al. 2014), phosphorus (Xia et al. 2020) and potassium (Yang et al. 2015) under a sufficient nutrient supply, but they also accumulate more sodium, chloride, cadmium and zinc under salinity and heavy metal stress in the shoots (Li et al. 2016; Liu et al. 2020b, 2021b; Song et al. 2018). The excessive accumulation of salt and heavy metal ions and the inability of detoxification certainly cause more adverse effects in females (Li et al. 2016; Liu et al. 2020b).
However, it has been suggested that males have higher reproductive costs than females, particularly in some wind-pollinated herbaceous species (Delph 1999; Harris and Pannell 2008). Herbs tend to have a greater demand for nitrogen than woody plants, as pollen is richer in nitrogen than fruit and seed (Ishida et al. 2005; Kerkhoff and Enquist 2006). Thus, when nitrogen is limited, the allocation of nitrogen to pollen production in males could compromise the growth of males more than that of females with more carbon allocation to fruits and seeds. It is worth noting that reproductive costs are probably linked to the timing of the resource allocation (Barrett and Hough 2013; Sánchez-Vilas and Pannell 2010). For example, males have higher reproductive costs during flowering and those are associated with flowering times, pollen production and amounts of nectar per flower. Actually, final reproductive costs are higher in females producing fruits and seeds, at least if we consider the whole reproduction season (Cepeda-Cornejo and Dirzo 2010; Núñez-Farfán and Valverde 2020). However, the costs of reproduction and perennial organs in the males of wind-pollinated species cause reduced defense responses (Harris and Pannell 2008; Morales et al. 2013; Oñate et al. 2012).
Under drought conditions, Humulus scandens and Silene otitis males maintain lower photosynthesis and show greater photosynthetic damage, thus rendering them higher drought sensitivity and mortality than females during the vegetative growth stage (Liu and Duan 2013; Soldaat et al. 2000). Comparably in Phillyrea angustifolia, females exhibit higher photosynthesis and intrinsic water use efficiency, and better growth performance than males under salt stress and desiccation (Medina-Gavilán et al. 2008). On the other hand, no sexual differences under drought stress have been found in Acer negundo and Populus angustifolia (Juvany and Munné-Bosch 2015; Ward et al. 2002). Thus, there are no general patterns about the responses of the sexes to environmental stresses. As discussed by Retuerto et al. (2018), sex-specific responses are highly dependent on species and stress types. Further research is needed to be made to elucidate the physiological, morphological and molecular mechanisms underlying sexually different responses to individual and combined environmental stresses during the whole growing season or even during the whole life cycle of perennial plants (Retuerto et al. 2018).
In addition, sex-specific responses to rising CO2 and temperature have been investigated in plant species to predict the potential mechanisms underlying plants’ responses to the climate change (Chen et al. 2021). These studies have suggested that males benefit more from elevated CO2 levels by increasing their leaf expansion and carbon assimilation throughout the whole growing season (Xu et al. 2008; Zhao et al. 2012). Elevated temperature leads to higher biomass in males through an increasing leaf expansion, resulting in higher carbon assimilation with higher sink activities (Tognetti 2012). The combination of CO2 and temperature increases root growth and biomass in males to meet the enhanced photosynthetic capacities (Zhao et al. 2012). In contrast, females have a larger leaf expansion, higher reproductive mass and more investment in density growth than males under elevated CO2 and temperature (Huang et al. 2014). These results suggest that females and males of dioecious plants possess sex-specific strategies to cope with elevated CO2 and/or temperature.
Biotic stresses
Sexual dimorphism is commonly associated with sexual differences in the nutritional status, growth rates and/or chemical defenses, which in turn may affect herbivory rates. In this section, we discuss sex-biased herbivory by focusing mainly on the resource allocation into growth, reproduction and defense, as well as on the effects of environmental stressors on sex-biased herbivory. Several studies have shown the presence of male-biased herbivory i.e. mostly derived from the cost of reproduction (Cornelissen and Stiling 2005). The resource allocation principle assumes that growth, reproduction and maintenance functions compete directly with each other, so that an increase in resource allocation to one function will result in a decrease in others (Charnov 2020; Juvany and Munné-Bosch 2015). Some studies reported that male plants have greater vegetative growth rates, more leaves and lower resource investment in physiological/chemical defenses, while females invest more nutrients into defense or reproduction (Avila-Sakar and Romanow 2012; Cornelissen and Stiling 2005). Usually, females have larger sizes and superior herbivory tolerance when compared with males in most herbaceous species (Bertiller et al. 2002; Vasiliauskas and Aarssen 1992). However, as reviewed by Avila-Sakar and Romanow (2012), it remains controversial, whether high or low growth rates favor herbivory tolerance.
Plants’ nutritional quality may also affect sex-biased herbivory since herbivores are usually limited by nitrogen compounds, such as amino acids (Mattson 1980). Rivkin et al. (2018) have suggested that the lower nitrogen content in Sagittaria latifolia males reduces the survival of Listronotus appendiculatus larvae. Moreover, nectar and pollen are important food resources for herbivores and their natural enemies (Stenberg et al. 2015). Males have increased nitrogen demands for pollen production, and they often exhibit great growth rates and nutritional qualities, as well as higher amounts of nectar per flower compared with females (Barrett and Hough 2013; Renner 2014). A quantitative analysis performed by Cornelissen and Stiling (2005) indicated that the herbivory induced by gall makers and folivores is more significant in male plants, and the herbivore damage affected by plant sex is more significant for flower predators than for browsers and folivores.
For chemical defense, plants can produce toxic chemical substances, such as secondary metabolites, to kill herbivores or elongate their development time (Fürstenberg-Hägg et al. 2013). Females have increased carbon demands for fruits and seeds. It appears that the large carbon storage in females during the nonproductive period may increase the synthetic potential of carbon-containing secondary metabolites, facilitating the defense against herbivores. For example, female plants have higher levels of tannin, phenolic and quercetin-glucoside-related compounds, and better chemical defense than males in dioecious Chloroclystis excise, Chamaedorea palms, Betula pendula Roth and B. pubesce (Cepeda-Cornejo and Dirzo 2010; Palo et al. 1992; Tsuji and Sota 2010). This is consistent with the hypothesis of ‘sex-biased herbivory’, which assumes that females have a better defense against insect herbivores due to their increased carbon investment into reproduction and carbon-based secondary metabolisms, whereas males are mostly attracted by herbivores because of the increased energy investment in growth (biomass) (Cepeda-Cornejo and Dirzo 2010; Coley 1988; Fincher 2009).
Most studies have reported that male-biased herbivory is common in dioecious plants studies so far (Ashman 2002; Retuerto et al. 2006; Yule and Burns 2019). However, some studies have found female-biased herbivory or similar herbivory in both sexes (Buckley and Avila-Sakar 2013; Espírito-Santo et al. 2012; McCall 2008). For example, in the Mexican ciruela, females have higher levels of herbivory associated with their nutritional content (Maldonado-López et al. 2014). In Ilex glabra, female-biased herbivory is found only after flowering (Buckley and Avila-Sakar 2013). The herbivory of marula trees is induced by African elephants, as the female branches are less ramified and shorter than those of male trees (Hemborg and Bond 2007). Thus, male-biased herbivory is not universal. As emphasized by Avila-Sakar and Romanow (2012), the assumption of male-biased herbivory in dioecious plants may be due to the taxonomic bias of species studied and the lack of empirical studies. Therefore, there is a need for more precise quantitative measurements of herbivore levels, growth rates and reproductive allocation in male and female plants.
Environmental conditions affect resource allocation and the content of defensive compounds in both sexes (Randriamanana et al. 2014; Yang et al. 2020). Females synthesize more condensed tannins than males under limited nutrient conditions, but less under sufficient nutrient conditions (Jiang et al. 2016). Additionally, plants’ low nutritional quality can provide potential defense against herbivores (Fritz and Simms 1992; Haukioja et al. 1991; Yang et al. 2020). Overall, the sex-biased herbivory appears to be associated with trade-offs between growth, production and maintenance, although there are still uncertainties of the mechanism (Juvany and Munné-Bosch 2015; Núñez-Farfán and Valverde 2020). The lack of a general pattern emerging from previous studies is assumed to be associated with the insufficient scope of investigations, too few plant families covered and the involvement of a single time point only (Núñez-Farfán and Valverde 2020; Retuerto et al. 2018). Therefore, further studies should be performed to have comprehensive coverage of plant species and life histories.
Overall, there is no clear pattern in the responses of different sexes to abiotic and biotic stresses. Larger scale studies encompassing multiple stress factors are needed to allow generalizations (Núñez-Farfán and Valverde 2020). These studies about sex-specific responses must consider the species in question, the nature and intensity of stresses and life cycle stages, including also responses to combined abiotic and biotic stresses.
BIASED SEX RATIOS
According to the hypothesis by Fisher (1930), the sex ratios of offspring should be close to 1:1. Despite this expectation, biased sex ratios commonly exist in dioecious plants (e.g. Field et al. 2013; Hardy 2002; Sinclair et al. 2012). Based on published studies, only 29% of plant populations exhibit 1:1 sex ratios, while 59% show deviations from equality, of which almost two-thirds being male-biased (Delph 1999; Sinclair et al. 2012). It has been suggested that male-biased sex ratios are dominant in dioecious vines and trees, whereas female-biased sex ratios are generally prevalent in shrubs, herbs and annuals (Field et al. 2012). Male-biased sex ratios are associated with long-lived growth, fleshy fruits and biotic seed dispersal, whereas female-biased sex ratios are associated with short-lived growth forms (e.g. herbs), clonality and abiotic pollen dispersal (Field et al. 2012; Sinclair et al. 2012) (Fig. 3). Highly skewed sex ratios probably cause plant population declines and even extinction, potentially affecting the structure and stability of communities (Retuerto et al. 2018). Understanding the mechanism underlying sex ratio biases is necessary for predicting sex-specific responses and effects caused by environmental stresses on dioecious species.
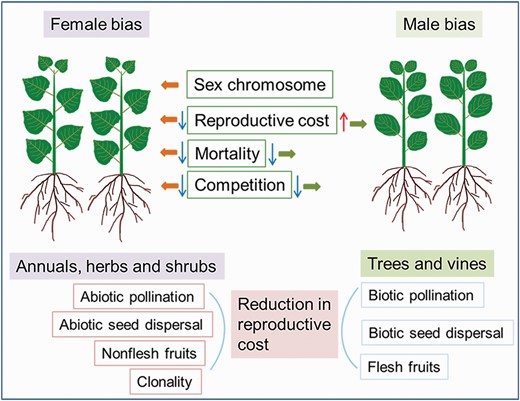
Factors causing biased sex ratios in plants. Biased sex ratios are usually determined by sex chromosomes, reproductive costs, mortality and sexual competition. Female-biased sex ratios are common in annuals, herbs and shrubs, being associated with sex chromosomes, reduced reproductive costs (such as abiotic pollination, abiotic seed dispersal and nonfleshy fruits), clonality and low mortality and competition. Male-biased sex ratios are common in trees and vines, being associated with lower mortality and sexual competition.
Mechanisms driving sex ratios are often associated with unknown genetic and ecological factors, including resource allocation, vegetative vigor, sex choice/switching, pollination competition and offspring mortality (Field et al. 2013; Harris and Pannell 2008; Stehlik and Barrett 2006). The effects of environmental factors on sex ratio biases may be attributable to the manner of sexual expression. Sex ratio biases may relate to sex chromosome meiotic drive (Jaenike 2001), pollen competition (certation) (Stehlik and Barrett 2005), genotype-specific mortality (Billiard et al. 2015) or allelic/genotypic incompatibilities (Pucholt et al. 2017). Among those, the certation hypothesis assumes that competition between male- and female-determinating microgametophytes (certation) would result in variation in pollination intensity, and large pollen loads would cause female-biased offspring sex ratios, as proposed for Rumex nivalis (Conn and Blum 1981). The offspring sex ratios depend on the maternal pollination environment (Stehlik and Barrett 2005). Stehlik et al. (2008) have suggested that R. nivalis females positioned close to males have higher stigmatic pollen loads and produce more strongly female-biased sex ratios than females further away from males.
Meiotic drive in Silene latifolia assumes that the X chromosome is associated with the female-biased ratio (Taylor 1994, 1999). Allelic or genotypic incompatibilities at key loci probably cause the genotype-specific mortality of seeds, zygotes and seedlings and, thus, sex ratio biases, such as single-locus self-incompatibility observed in the dioecious shrub species P. angustifolia (Billiard et al. 2015). Furthermore, female-biased sex ratios often occur in species with heteromorphic sex chromosomes, although sex chromosomes are rare in angiosperms (Charlesworth 2002; Vyskot and Hobza 2004). The ‘unguarded sex chromosome hypothesis’ predicts that species with heterogametic sex may have higher mortalities than species with homogametic sex due to the recombination between sex-determining loci is absent, thus leading to the consequent accumulation of deleterious mutations (Pipoly et al. 2015; Stehlik and Barrett 2006).
The secondary sex ratio is usually reported to be affected by secondary sexual traits (Meagher 1992). Biased secondary sex ratios may be associated with sex-specific resource allocation, the cost of reproduction (Ortiz et al. 2002), susceptibility to environmental stressors (Varga and Soulsbury 2020), mortality and competition between sexes (Hroneš et al. 2019). Environmentally sexual determination is expected to bias sex ratios toward the sex facilitating survival and fitness in poor environments (Charnov and Bull 1977; Wade et al. 2003). The correlation of sex ratios and environmental stressors will ultimately be the consequence of the sexes experiencing environmental pressures in a sex-specific manner (Retuerto et al. 2018). Environmental factors, including light, water, temperature, CO2 level and nutrient availability, are known to influence sex ratios in dioecious plant species (Mesgaran et al. 2019; Petry et al. 2016; Retuerto et al. 2018; Sinclair et al. 2012; Varga and Soulsbury 2020).
The sex allocation theory predicts that the environmental facilitation effect on plant growth induces female sex expression, while resource-poor conditions should favor maleness (Charnov 2020). Commonly, females exhibit greater reproductive costs than males, possibly experiencing higher mortality as well, resulting in male-biased sex ratios, especially in less favorable environments. Mesgaran et al. (2019) have suggested that the overrepresentation of male plants under stressful environments may be attributable to sex-differential mortality rather than to an actual sex change. Other studies have proposed that males produce massive amounts of pollen to successfully reproduce, and such great reproductive investment would cause female-biased sex ratios (Harris and Pannell 2008; Sinclair et al. 2012). Yet, the genetic and ecological mechanisms governing sex ratio patterns in dioecious plant species are still unclear. Future work should attempt to elucidate in depth the effects of environmental stressors, including multiple interacting factors, on the sex-biased ratios.
SEXUAL INTERACTIONS
Sex recognition and adaptation
Competition plays a fundamental role in determining the composition and function of biological communities (Chen et al. 2014; Goldberg and Barton 1992). Plants have evolved the ability to recognize their neighbors’ identity and plastically adjust their phenotypes in response to competition (Dong et al. 2017; Jaafry et al. 2019; Mercer and Eppley 2014). Recognition of neighboring individuals includes light quality, the presence of chemical and nutrient substances and mechanosensing of neighbors (Ballare et al. 1990; Gagliano et al. 2012; Poorter et al. 2012).
Dioecious plant species exhibit sexual dimorphism in morphology, physiology, life histories, resource allocation and defense mechanisms (Bullock and Bawa 1981; Cepeda-Cornejo and Dirzo 2010; Sánchez-Vilas et al. 2012). Some studies have suggested that females and males can recognize their neighbors’ identity and exhibit sex-specific responses as a result of above- and belowground competition, visible, for instance, as shade avoidance and root system architecture (Dong et al. 2017; Jaafry et al. 2019; Mercer and Eppley 2014). According to the concept of a ‘functional equilibrium’ of biomass allocation, plants would allocate more biomass to roots, if the growth-limiting factor is below ground (e.g. water and nutrients), whereas more biomass would be allocated to shoots, if the growth-limiting factor is above ground (e.g. light and CO2) (Brouwer 1963; Poorter et al. 2012; Thornley 1972).
Some studies have suggested that males show more resource allocation to growth than do females in response to aboveground competition (Sánchez-Vilas and Pannell 2010). The study by Tonnabel et al. (2017) reported that males of Mercurialis annua tend to reduce aboveground biomass and canopy growth more significantly compared with females under increasing light competition. Belowground competition is mainly driven by the availability of soil resources, such as nutrients and water (Schenk 2006). Consistently, female poplars have more root biomass than males under intersexual competition in a resource-rich environment (Chen et al. 2015). Contrarily, male poplars have higher root/shoot ratios than females when under competition with limited resources (Chen et al. 2015). As far as we know, only a few studies have investigated adaptive responses related to sex-specific belowground competition. More studies are needed to reveal morphological, physiological and molecular differences between the sexes under competition in response to environmental stressors.
Sexual competition
Females and males exhibit many kinds of morphological and physiological responses to optimize their performance and competitive ability (Chen et al. 2017). Consistently, many studies have suggested that female-biased sex ratios are favored under a better availability of resources to meet the greater reproductive cost of females, while male-biased sex ratios are favored in lower-resource habitats with a better stress tolerance of males (Chen et al. 2017; Correia and Barradas 2000; Dawson and Geber 1999). According to the maximum growth theory, plants with a maximum vegetative growth rate would be superior in competition, whereas the minimum resource requirement theory suggests that plant species with lower resource requirements are more competitive (Grace and Tilman 1990). Consistently, females with larger sizes exhibit a greater competitive ability than males in most herbaceous species (Bertiller et al. 2002; Vasiliauskas and Aarssen 1992; Zhang et al. 2009). In some woody dioecious perennials, males with larger sizes are expected to be competitively superior in growth (Obeso 2002). Hawkins et al. (2009) have shown that females and males exhibited similar competitive abilities in Lindera melissifolia (Lauraceae). Thus, it is evident that the competitive ability and intensity between the sexes vary among species and are probably related to life-history stages, reproductive costs, resource demands and environmental factors.
The two-phase resource dynamics hypothesis of plant interactions assumes that the resources are temporally variable. Consequently, most growth occurs under high-resource conditions, while most mortality takes place under poor resources (Deborah and Ariel 1997). In P. cathayana, females show a greater competitive ability than do males under well-watered, high phosphorus and nitrogen conditions, but the opposite occurs under drought and nutrient deficiency conditions (Wu et al. 2021; Xia et al. 2020). Therefore, the sex-specific utilization of resources may be decisive for the intensity and direction of competition in dioecious plants. Niche complementarity predicts that species differing in their use of resources have reduced competition and they are more likely to coexist, even in highly variable environments (Pacala and Tilman 1994). Many different kinds of coexistence mechanisms are reported, such as differential responses to spatial and temporal environmental variation (Chesson 1994, 2000), resource partitioning (Tilman 1982) and species-specific natural enemies (Connell 1971; Janzen 1970). These all lead to niche differences (Adler et al. 2010).
Dioecious plant species exhibit sexual differences in reproductive morphology, which may cause sexual specialization to different habitats leading to niche differentiation (Mizuki et al. 2018; Rogers and Eppley 2012). Further analyses have suggested that competition for limited resources would be the main results of the evolution of the sexual dimorphism in the utilization of resources and niche occupancy (Eppley 2005; Slatkin and Anderson 1984). The proposed hypothesis of the evolution of sexual niche partitioning assumes that niche differences are derived from natural selection for decreasing intersexual competition (Cox 1981). Such a hypothesis is expected to be valid only if the resulting distances in time and space are not so great that the chances for reproduction would reduce greatly (Cox 1981). Some studies have suggested that intersexual competition is greater than intrasexual one in dioecious plants, such as in Antennaria dioica and Osyris quadripartite (Herrera 1988; Varga and Kytöviita 2011), thus supporting the niche partitioning hypothesis. In turn, as a result of niche partitioning, intersexual competition between males and females may be expected to be alleviated compared with that between plants of the same sex (e.g. Zhang et al. 2009).
As we discussed above, the space segregation in patchy habitats may be attributable to the sexual specialization and competition in habitat and/or resource utilization, thus allowing the coexistence of sexes in dioecious species. The stress gradient hypothesis assumes that facilitative interactions are predominant under the most severe environmental conditions, whereas competition is common when the stress level is lower (Brooker et al. 2008; Maestre et al. 2009). Intrasexual competition is stronger in response to drought and heavy metals in P. cathayana (Chen et al. 2014, 2017; Li et al. 2016). However, the intersexual competition of poplars is slightly alleviated by nitrogen deficiency and drought (Chen et al. 2014, 2015) but increased by salt stress (Li et al. 2016). Therefore, environmental factors, such as nutrient availability, can significantly modify the strength and direction of competition. However, more research is needed to explore the intensity and direction of competition (including aboveground and underground competition) based on reproductive allocation and spatial sex differentiation.
CONCLUSIONS AND PERSPECTIVES
Sexual differences and sex ratio biases of dioecious plant species have been explored for many decades, given the prevalence of sex ratio biases in dioecious flowering plants. Generally, females have higher reproductive costs and, consequently, reduced abiotic stresses tolerance when compared with males in most trees and vines. Conversely, males show better tolerance to biotic stresses in some studies. However, the general patterns are still lack related to sex-specific responses to stresses (abiotic and biotic stresses). Sexually different tolerances largely depend on the trade-off between growth, reproduction and defense, requiring taking consideration of plant reproductive frequency and intensity, life history and developmental stages. Further studies are needed to reveal the strategies and mechanisms adopted by different sexes to cope with environmental stresses (abiotic stress, biotic stress and combination of them).
Patterns of sex ratios largely depend on sex choice/switch, mortality, reproductive costs and sexual competition, which may cause spatial segregation of the sexes along environmental gradients. More studies are needed on the impact of environmental changes on population structures, especially under changing climate conditions. Moreover, the exploration of physiological and molecular mechanisms of sexual recognition and adaptation under sexual competition (including shoot and root competitions) could help to integrate the key factors determining sexual dimorphism and sex ratios under environmental changes.
Funding
This work was supported by the Natural Science Foundation of China (U1803231) and the Talent Program of the Hangzhou Normal University (2016QDL020).
Conflict of interest statement. The authors declare that they have no conflict of interest.