-
PDF
- Split View
-
Views
-
Cite
Cite
Yanting Hu, Jianguo Gao, Ping Zhao, Weijun Shen, Peiqiang Zhao, Liwei Zhu, Guangyan Ni, Junfeng Niu, Lei Ouyang, Water transport of native and exotic tree species in relation to xylem anatomical characteristics in low subtropical China, Journal of Plant Ecology, Volume 11, Issue 3, June 2018, Pages 423–433, https://doi.org/10.1093/jpe/rtx010
- Share Icon Share
Abstract
Exotic fast-growing tree species have been commonly planted as pioneer species to facilitate ecological restoration in South China. Their growth and resource utilization behavior related to intrinsic physiology and structural properties have profound influences on forest ecosystem. However, the contrastive research focusing on water utilization features along with xylem anatomical properties between native and exotic species is scarce in South China. The objective of this study is to investigate the sapwood anatomical characteristics and water utilization conditions of native and exotic fast-growing species, and to elucidate the relationship between sap-flux density and conduit features.
We measured sap-flux density, conduit length, diameter and density of four native species (Schima superba, Michelia macclurei, Castanopsis hystrix and Castanopsis fissa) and four exotic species (Eucalyptus citriodora, Eucalyptus urophylla × grandis, Acacia auriculaeformis and Acacia mangium). Sap flux density was measured based on the Granier’s thermal dissipation probe method. The whole-tree water transport was quantified by multiplying sap-flux density by sapwood area. The measurements of conduit characteristics were conducted by using segregation and slice method.
Sapwood area increased with the growing diameter at breast height (DBH) as a power function. Native species had a larger water-conducting tissue area than exotic species at the same DBH value when trees grew to a size with a certain value of DBH. The conduit diameter of exotic species was significantly larger than that of native species. Conversely, native species, such as S. superba and M. macclurei, had longer conduit length and higher conduit density than other tree species. Based on a physiological interpretation of the measured conduit characteristics, native tree species developed a safe water transport system while exotic fast-growing tree species come into being an efficient system instead. Water transport increased with the growing DBH as a power function, and the exponent for native species (1.60) was higher than that for exotic species (1.22). Under the combined impact of sap-flux density and sapwood area, native species presented a larger water transport at a larger DBH value, indicating that growth advantage of exotic fast-growing species might weaken as DBH increased.
INTRODUCTION
Deforestation is the second largest anthropogenic source of carbon dioxide to the atmosphere after fossil fuel combustion (van der Werf et al. 2009). In recent years, large scale reforestation as an effective approach to mitigate anthropogenic greenhouse-gas emissions has attracted more and more interest (Solomon 2007). In many regions, exotic fast-growing tree species were introduced as a means of achieving wood production and promoting conservation of forests in consideration of their high biomass and productivity, and thus increasing carbon sequestration (Calvo-Alvarado et al. 2007; Messier et al. 2003; Siregar et al. 2007). The growth and resource utilization behaviour of exotic fast-growing tree species related to intrinsic physiology and structural properties has profound influences on carbon storage (Kaul et al. 2010; Pejchar and Mooney 2009; Zhang et al. 2012), nutrient cycling (Ares and Fownes 2000), water budget (Dye 1996; Scott et al. 2000) and other related ecological process and function. Thus, the characteristics of these introduced tree species and the underlining mechanism between structure and function should be investigated specifically and comprehensively in order to elucidate the intrinsic mechanisms of the above effects.
Many studies addressing characteristics of the introduced tree species have been conducted; some of which emphasized their ecological influences (Nampakdee et al. 2010; Peterken 2001; Simberloff et al. 2010; Wu et al. 2013), while others focused on the vegetation growth and physiological process mechanism per se (DeWalt et al. 2004; Lee et al. 2004; Meunpong et al. 2010; Tian et al. 2002). Due to diverse physiological features and structural characteristics, e.g., photosynthetic efficiency, water-conducting tissue properties and hydraulic conductivity, the pattern of water use for exotic tree species may present a disparate way compared to that for indigenous ones. Water utilization characteristics for diverse exotic tree species has been reported separately (Cienciala et al. 2000; Morris et al. 2004; Otieno et al. 2005). However, the contrastive research focusing on water utilization features along with xylem anatomical properties between native and exotic tree species is scarce.
National scale afforestation has significantly increased forest area in China since 1950 with a government policy (Kauppi et al. 2006; Zhang and Song 2006; Zhao and Zhou 2005). Due to the high productivity and adaptability of exotic fast-growing species, their cultivation was propelled instead of using native tree species (Zhang et al. 2000). In South China, since the launch of the campaign of ‘Afforesting the Barren Hilly Lands’ in the mid-1980s, exotic fast-growing species have been commonly planted as pioneer species to facilitate ecological restoration, among them Eucalyptus and Acacia species are frequently used for afforesting and greening degraded hilly lands in South China (Chen et al. 2006; Peng et al. 2005; Wu et al. 2013; Yang et al. 2009). Researches on the water utilization properties of the introduced species will help comprehend advantages and disadvantages of water use strategy for exotic species compared to native tree species in the process of restoration, and increase our ability to formulate realistic management approach (D’Antonio and Meyerson 2002).
In South China, researches concerning about the transpiration characteristics of native tree species have been conducted (Ma et al. 2008; Wang et al. 2012; Zhao et al. 2006). Meanwhile, a number of researches focusing on water utilization of exotic species were also reported (Lane et al. 2004; Morris et al. 2004; Zhu et al. 2015). Most of these researches mainly lay emphasis on transpiration and its response to environmental factors of native or exotic species separately. However, the differences in water transport between them were much less documented. Moreover, intrinsic structural features such as conduit anatomy as well have influence on water transport apart from external environmental factors. Conduit anatomy can explain how plants are coping with the trade-off between safety and efficiency of water transport (Zach et al. 2010). There is seldom research on analyzing conduit anatomy features of tree species and their relationship to water transport in low subtropical China.
In this research, we investigated sapwood anatomical characteristics and water transport features of four native species (Schima superba, Michelia macclurei, Castanopsis hystrix and Castanopsis fissa) and four exotic species (Eucalyptus citriodora, Eucalyptus urophylla × grandis, Acacia auriculaeformis and Acacia mangium) that are very common in low subtropical China. The main objectives are: (i) to document and compare the sapwood anatomical characteristics of native and exotic species; (ii) to investigate the water transport quantity for indigenous and exotic fast-growing species; (iii) to elucidate the relationship between sap flux density and sapwood conduit features.
MATERIALS AND METHODS
Site description and studied tree species
We chose eight representative and commonly planted tree species from three different sites in South China for research. The first site (112°54′E, 22°41′N) is located in Heshan National Field Research Station of Forest Ecosystem, Guangdong Province, China. This region is dominated by a subtropical monsoon climate, with mean annual evaporation of 1600 mm, precipitation of 1700 mm, and average temperature of 21.7°C. The highest temperature is 37.5°C in July, and the lowest temperature is 0°C in January. In this region, the climax community is subtropical monsoon evergreen broad-leaved forest. As human disturbance had brought about vegetation degradation, an ecological restoration campaign which was intended to reforest this degraded hilly land was launched in the early 1980. At this site, four native species including S. superba, M. macclurei, C. hystrix, C. fissa and an exotic tree species A. mangium were selected for this investigation. The soil has a pH of 4.3, the organic matter content of 24.2 g·kg−1 and total nitrogen content of 1.2 g·kg−1 (Gao et al. 2015).
The second site (113°22′E, 23°11′N) is located in the South China Botanical Garden, Guangzhou city, China. This region is typically dominated by a subtropical monsoon climate, nearly the same as that of the first site. It experiences an annual precipitation of 1697 mm and an annual average temperature of 21.9°C. The soil is a loam with a pH of 4.0, the organic matter content of 23.0 g·kg−1 and total nitrogen content of 0.7 g·kg−1 (Zhu et al. 2012). In this site, exotic tree species, E. citriodora and A. auriculaeformis, were selected for measurements. The plantations have been planted since the mid-1980s.
The third site (109°54′E, 24°46′N) is the Huangmian state forest farm, Guangxi province, China. The forest farm has been mainly engaged in the plantation of E. urophylla × grandis. This area is located in the transition zone of mid-subtropical and lower subtropical, and also characterized by a monsoon climate. Annual rainfall varies from 1750 to 2000 mm. The mean annual temperature is approximately 21°C, the minimum and maximum mean monthly temperatures are approximately 10°C in January and 29°C in July, respectively (Zhu et al. 2015). A 400-m2 experimental plot with stem density of 1425 stems·ha−1 was established in the plantation for ecological research. The soil in the experimental plot has a pH of 3.5, the organic matter content and total nitrogen content of the soil was 27.7 g·kg−1 and 1.1 g·kg−1, respectively (Zhu et al. 2015).
Although the eight different tree species are distributed in three different sites, i.e., Heshan National Filed Research Station, South China Botanical Garden and Huangmian state forest farm, these study sites are all characterized by a monsoon climate. Additionally, the soil condition was similar for these three sites, with the soil pH of 3.5–4.3, the organic matter content of 23.0–27.7 g·kg−1 and total nitrogen content of 0.7–1.2 g·kg−1. Therefore, the similar climatic and soil conditions provide comparability for data on both sap flow and tree anatomical characteristics between species. The number and characteristics of the studied trees for sap flow measurement are summarized in Table 1.
biometric characteristics of the eight studied tree species and number of sample trees for sap flow measurements
Plant species . | Code . | DBH (cm)a . | Tree height (m)a . | Sapwood area (cm2) . | Nb . |
---|---|---|---|---|---|
S. superba | SS | 8.3–22.8 (13.6) | 4.7–10.1 (6.8) | 44.0–329.9 (123.3) | 24 |
M. macclurei | MM | 6.3–29.3 (18.1) | 5.5–17.1 (10.7) | 25.1–570.6 (229.8) | 39 |
C. hystrix | CH | 12.1–12.9 (12.6) | 7.7–14.8 (10.1) | 254.4–289.3 (268.5) | 3 |
C. fissa | CF | 21.1–22.4 (21.6) | 9.4–12.4 (11.1) | 69.8–78.4 (75.5) | 3 |
E. citriodora | EC | 12.4–33.1 (21.3) | 13.7–30.1 (20.9) | 33.7–223.9 (104.9) | 15 |
E. urophylla × grandis | EE | 8.6–14.4 (11.5) | 8.6–14.4 (11.5) | 53.6–129.3 (88.5) | 15 |
A. mangium | AM | 13.4–37.5 (23.2) | 12.0–22.8 (17.8) | 49.4–304.1 (136.8) | 14 |
A. auriculaeformis | AA | 15.3–37.4 (23.9) | 14.6–22.9 (17.7) | 62.9–231.5 (122.8) | 15 |
Plant species . | Code . | DBH (cm)a . | Tree height (m)a . | Sapwood area (cm2) . | Nb . |
---|---|---|---|---|---|
S. superba | SS | 8.3–22.8 (13.6) | 4.7–10.1 (6.8) | 44.0–329.9 (123.3) | 24 |
M. macclurei | MM | 6.3–29.3 (18.1) | 5.5–17.1 (10.7) | 25.1–570.6 (229.8) | 39 |
C. hystrix | CH | 12.1–12.9 (12.6) | 7.7–14.8 (10.1) | 254.4–289.3 (268.5) | 3 |
C. fissa | CF | 21.1–22.4 (21.6) | 9.4–12.4 (11.1) | 69.8–78.4 (75.5) | 3 |
E. citriodora | EC | 12.4–33.1 (21.3) | 13.7–30.1 (20.9) | 33.7–223.9 (104.9) | 15 |
E. urophylla × grandis | EE | 8.6–14.4 (11.5) | 8.6–14.4 (11.5) | 53.6–129.3 (88.5) | 15 |
A. mangium | AM | 13.4–37.5 (23.2) | 12.0–22.8 (17.8) | 49.4–304.1 (136.8) | 14 |
A. auriculaeformis | AA | 15.3–37.4 (23.9) | 14.6–22.9 (17.7) | 62.9–231.5 (122.8) | 15 |
aValues are the variation range with means in parentheses.
bN is the number of individual trees on which sap flow sensors were deployed.
biometric characteristics of the eight studied tree species and number of sample trees for sap flow measurements
Plant species . | Code . | DBH (cm)a . | Tree height (m)a . | Sapwood area (cm2) . | Nb . |
---|---|---|---|---|---|
S. superba | SS | 8.3–22.8 (13.6) | 4.7–10.1 (6.8) | 44.0–329.9 (123.3) | 24 |
M. macclurei | MM | 6.3–29.3 (18.1) | 5.5–17.1 (10.7) | 25.1–570.6 (229.8) | 39 |
C. hystrix | CH | 12.1–12.9 (12.6) | 7.7–14.8 (10.1) | 254.4–289.3 (268.5) | 3 |
C. fissa | CF | 21.1–22.4 (21.6) | 9.4–12.4 (11.1) | 69.8–78.4 (75.5) | 3 |
E. citriodora | EC | 12.4–33.1 (21.3) | 13.7–30.1 (20.9) | 33.7–223.9 (104.9) | 15 |
E. urophylla × grandis | EE | 8.6–14.4 (11.5) | 8.6–14.4 (11.5) | 53.6–129.3 (88.5) | 15 |
A. mangium | AM | 13.4–37.5 (23.2) | 12.0–22.8 (17.8) | 49.4–304.1 (136.8) | 14 |
A. auriculaeformis | AA | 15.3–37.4 (23.9) | 14.6–22.9 (17.7) | 62.9–231.5 (122.8) | 15 |
Plant species . | Code . | DBH (cm)a . | Tree height (m)a . | Sapwood area (cm2) . | Nb . |
---|---|---|---|---|---|
S. superba | SS | 8.3–22.8 (13.6) | 4.7–10.1 (6.8) | 44.0–329.9 (123.3) | 24 |
M. macclurei | MM | 6.3–29.3 (18.1) | 5.5–17.1 (10.7) | 25.1–570.6 (229.8) | 39 |
C. hystrix | CH | 12.1–12.9 (12.6) | 7.7–14.8 (10.1) | 254.4–289.3 (268.5) | 3 |
C. fissa | CF | 21.1–22.4 (21.6) | 9.4–12.4 (11.1) | 69.8–78.4 (75.5) | 3 |
E. citriodora | EC | 12.4–33.1 (21.3) | 13.7–30.1 (20.9) | 33.7–223.9 (104.9) | 15 |
E. urophylla × grandis | EE | 8.6–14.4 (11.5) | 8.6–14.4 (11.5) | 53.6–129.3 (88.5) | 15 |
A. mangium | AM | 13.4–37.5 (23.2) | 12.0–22.8 (17.8) | 49.4–304.1 (136.8) | 14 |
A. auriculaeformis | AA | 15.3–37.4 (23.9) | 14.6–22.9 (17.7) | 62.9–231.5 (122.8) | 15 |
aValues are the variation range with means in parentheses.
bN is the number of individual trees on which sap flow sensors were deployed.
Sap-flux density (JS) and water transport
Sap-flux density was measured based on the Granier’s thermal dissipation probe (TDP) method (Granier 1987). Each TDP sensor that consists of a pair of 20 mm long, 2 mm diameter stainless steel probes was installed vertically 10–15 cm apart along the axis of the hydroactive xylem. The upper probe was heated by constant DC of 120 mA yielding a power of 0.2 W, while the lower one remained unheated. Instantaneous temperature difference between the probes can be converted into a voltage value. The data were read every 30 s and stored as 10-min averages with a DL2e data logger (Delta-T Devices, UK). Finally, the sap-flux density (g H2O·m−2·s−1) was calculated according to the following formula (Granier 1987):
where ΔTm is the temperature difference obtained under zero flow conditions, ΔT is the instantaneous temperature difference. These temperature difference data were converted to sap-flux density using the Baseliner 3.0 program developed by the Nicholas School of Environment and Earth Sciences at Duke University, USA.
Stem sap flow determined with Granier’s TDPs can be used for calculating whole-tree transpiration. To estimate tree transpiration based on sap flow measurement, we need to know the hydroactive cross sectional area of the tree stem. To avoid damage to the sample trees inside the experimental plot, we selected 15–20 trees of different diameter classes around the experimental site and obtained stem core samples using an increment borer. Sapwood depth was determined visually from coloration changes between heartwood and sapwood. The diameter at breast height (DBH) was measured at 1.3 m above the ground by a DBH ruler. We established the relationship between the DBH (cm) and sapwood area (As, cm2) calculated from the sapwood depth as follows (Vertessy et al. 1995):
where k and b are coefficients obtained through a non-linear regression analysis. Based on the measured DBH and the established formula, the sapwood area of each sample tree for sap flow measurement in each investigation plot can be obtained. The whole-tree transpiration (Et, g·s−1) was calculated as follows:
When applying formula (3), the radial variation of JS should be taken into consideration. The radial variation of JS for S. superba was determined according to a former study conducted by Zhu et al. (2012) at the same district. For the other tree species, the radial variation of JS was calculated by applying an equation for angiosperm plant proposed by Pataki et al. (2011).
Where Ji represents sap-flux density at depth i (g·m−2·d−1), Jo denotes sap-flux density in the outer 2 cm of sapwood (g·m−2·d−1) and x denotes relative sapwood depth. The average daily water transport was obtained from measurements of 15 sunny days. For S. superba, M. macclurei, C. hystrix, C. fissa and E. urophylla × grandis, average daily water transport was calculated from 1 to 15 October 2012, while that for E. citriodora and A. auriculaeformis from 1 to 15 October 2013 and for A. mangium from 24 September to 9 October 2003.
The meteorological condition for those analyzed period was similar. The daily average photosynthetically active radiation (PAR) for four native species (S. superba, M. macclurei, C. hystrix and C. fissa), two exotic species (E. citriodora and A. auriculaeformis), E. urophylla × grandis and A. mangium was 236–352, 155–258, 135–419 and 176–315 µmol·m−2·s−1, respectively, and the daily average vapor pressure deficit (VPD) was 0.6–1.4, 0.7–1.6, 0.8–1.6, 0.5–1.0 kPa, respectively. Moreover, there was adequate precipitation during mid-September for all the sites according to the meteorological conditions. The soil water content (SWC) for four native species (S. superba, M. macclurei, C. hystrix and C. fissa), two exotic species (E. citriodora and A. auriculaeformis) and E. urophylla × grandis was 0.27, 0.31 and 0.25 m3·m−3, respectively. We did not measure SWC for A. mangium plot, however, according to the research by Wang et al. (2014), the SWC during 24 September to 9 October from 2004 to 2007 for the same site ranged from 0.20 to 0.30 m3·m−3. Thus, soil water condition was similar for these three sites as well.
Additionally, there usually exists time lag between sap flow measured at breast height and the actual transpiration in canopy. Estimation of the time lag is necessary when performing analyses on sap flow response to environmental variables. We followed the method provided by Phillips et al. (1997) to calculate time lag. Also, something worth mentioning is the tree number of C. hystrix and C. fissa measured. The experiment plot (Heshan National Field Research Station) was a secondary evergreen broad-leaved forest, which has developed in the natural recovery succession for nearly 30 years since the ecological restoration campaign was launched. Such kind of secondary evergreen broadleaf forest with similar species composition as the one we chose for this experiment was common in this region. In the community, the dominant tree species were S. superba and M. macclurei with an importance value of 0.34 and 0.29, respectively. The importance value of C. hystrix and C. fissa was only 0.06 and 0.04, respectively, and there were only a few trees in the plot available for the measurement. Due to the similar initial size and environmental conditions, the DBH difference for C. hystrix or C. fissa was minor. Therefore, the number and the DBH range of C. hystrix and C. fissa measured in this research was small.
Conduit characteristics
The conduit length, diameter and density of the eight tree species were measured in this research. To avoid destruction on sample trees in the experimental plot, we selected 3 trees for each species around the plot and obtained a 2-cm long wooden core using an increment borer from each tree. The obtained wooden core was immersed in the MGA solution (38% methanol: glacial acetic acid: 70% alcohol = 5:5:90) immediately and split up into two 1-cm long segments in the laboratory, one for the observation of conduit length and the other for the determination of conduit diameter and density under the microscope.
After soaked in the MGA solution for 48 h, one 1-cm long wooden core was transferred to the segregation solution (10% chromic acid: 10% nitric acid = 1:1) and soaked for 48 h. The wooden core was then rinsed with alcohol for several times and stained with dye solution (1 g safranin + 100 ml 95% alcohol). The stained wooden core was placed on the glass slide and observed with an optical microscope (Axioplan 2 imaging, Zeiss, German) after rinsed with alcohol for several times. Pictures of xylem conduits were taken, and the length of randomly selected 50–100 conduits for each picture was measured with ocular micrometer.
After soaked in the MGA solution for 48 h, the other 1-cm long wooden core was put in boiling water for 24 h. Stem cross sections (20 µm thick) were sliced with a sliding microtome and the cross sections were observed with the same optical microscope mentioned above. For each tree, up to 50 conduits were observed for the measurement of conduit diameter and density. The diameter of elliptic conduit was calculated as the following equation: , where a and b are the length of major semi-axis and minor semi-axis, respectively (White 1991).
Environmental monitoring
In Heshan National Field Research Station of Forest Ecosystem, meteorological data including PAR (µmol·m−2·s−1), temperature (T, °C) and relative humidity (RH, %) were provided by a meteorological station within the field that is ~50 m away from the experimental site. These PAR, T and RH data were hourly averages. Additionally, soil samples were collected randomly in the experimental plots to measure water content by weighing method in 2012.
At the South China Botanical Garden site, a PAR (µmol·m−2·s−1) sensor (Li-Cor Quantum Sensor, Li-Cor, USA), a temperature and humidity sensor (HC2-S3, ROTRONIC, Switzerland) and a wind speed (u, m·s−1) sensor (AN4, Delta-T, UK) were mounted on a 18 m-high observation tower erected in a S. superba plantation that is 40 m away from the experimental site (E. citriodora and A. auriculaeformis plantations). Three SM200-05 sensors (SM200-05, Delta-T Device, UK) were buried 30 cm under the soil surface to detect the volumetric SWC (m3·m−3). All these sensors were connected to a data logger (DL2e, Delta-T Devices, UK). These sensors read every 30 s, and the 10-min averaged data were recorded.
A 23.5 m-high tower was constructed inside the experimental plot in Huangmian state forest farm to provide a platform for the installation of environmental sensors. A SKP215 quantum sensor (Sky Instruments Ltd, Powys, UK), a wind speed sensor (AN4-05, Casella, Ltd, UK) and an AT2&RHT2 sensor (Delta-T Devices, Ltd, Cambridge, UK) were installed on the tower for monitoring PAR, wind speed, temperature and RH, respectively. The soil volumetric water content at a depth of 30 cm was monitored using a SM300 sensor (Delta-T Devices, Ltd, Cambridge, UK). Environmental factors were measured every 30 s, and 10-min means were recorded in a DL2e data logger.
The VPD (kPa) was calculated using the following formula (Campbell and Norman 1998) in all the three experimental sites_ENREF_38:
where a, b and c are fixed parameters (0.611 kPa, 17.502 (unitless) and 240.97°C, respectively).
Data analysis
Statistical analyses were performed by applying R statistical software (v.3.2.3). Independent sample t-tests were used to examine whether the differences in the sapwood area between native and exotic species were significant (P < 0.05), and were also applied to test the differences in conduit length, diameter and density between native and exotic species. One-way analysis of variance followed by a Duncan’s test was used to test the differences in conduit length, diameter and density among the eight studied tree species. All figures were plotted by using Origin 9.0 (OriginLab Corp., USA).
RESULTS
Sapwood area
Relationship between sapwood area and DBH for native and exotic species is shown in Fig. 1. Sapwood area increased with the growing DBH as a power function (P < 0.05): . After taking the logarithm of the equation, it is transformed to . A higher B value (i.e., the slope of latter equation) indicated a larger increment of sapwood area with the same DBH increase. In this research native species had higher B value, suggesting the increasing range of sapwood area was larger for native species than that for exotic species with the same DBH increment. It is noteworthy that when DBH values were larger than 20 cm, sapwood area for native species was significantly higher than that for exotic species (P < 0.05) as shown in Fig. 2, indicating a larger water-conducting tissue area for native species at the same DBH value when trees grew to a size with a certain value of DBH.
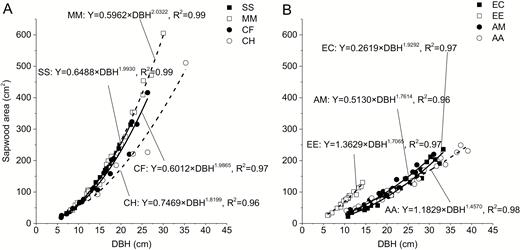
relationship between sapwood area and DBH for the native species (A) and exotic species (B). Solid square, open square, solid circle and open circle in panel A represent S. superba, M. macclurei, C. hystrix and C. fissa, respectively, while those in panel B denote E. citriodora, E. urophylla × grandis, A. mangium and A. auriculaeformis, respectively.
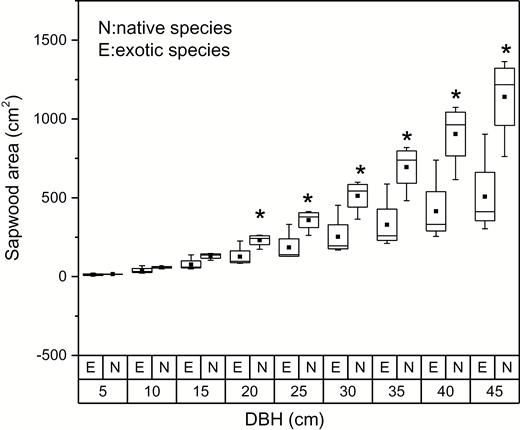
comparison of sapwood area between native and exotic tree species at different DBH values. The symbol ‘*’ indicates significant differences in sapwood area between native and exotic species (P < 0.05). The letter N and E represent native species and exotic species, respectively.
Conduit characteristics
Conduit characteristics of the eight studied tree species including conduit length, diameter and density were measured. S. superba and M. macclurei, the native species, had longer conduit length and higher conduit density than the other tree species (P < 0.05) as shown in Fig. 3 and Fig. 4. Different from conduit length and density, the average conduit diameter of exotic species (136.7 ± 16.97 µm) was significantly larger than that of native species (68.4 ± 11.83 µm; P < 0.05) as shown in Fig. 5.
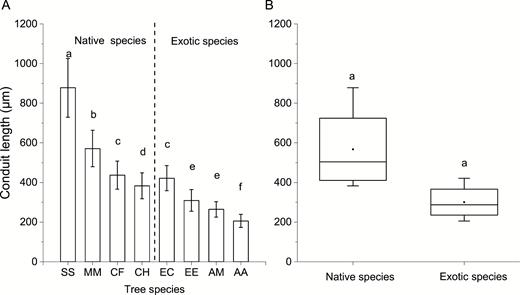
conduit length of the eight studied tree species (A) and comparison of conduit length between native and exotic species (B). Different lowercases indicate significant differences in conduit length among eight studied tree species in panel A, and significant differences between native and exotic species in panel B (P < 0.05). The meaning of abbreviations along the abscissa in panel A was listed in Table 1 (the same below).

conduit density of the eight studied tree species (A) and comparison of conduit density between native and exotic species (B). Different lowercases indicate significant differences in conduit density among eight studied tree species in panel A, and notable differences between native and exotic species in panel B (P < 0.05).
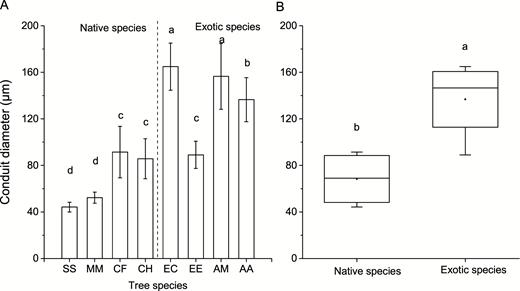
conduit diameter of the eight studied tree species (A) and comparison of conduit diameter between native and exotic species (B). Different lowercases indicate significant differences in conduit diameter among eight studied tree species in panel A, and significant differences between native and exotic species (P < 0.05) in panel B.
Water transport
The average daily water transport was obtained from measurements of 15 sunny days. We found that water transport increased with the growing DBH as a power function (P < 0.05), which is similar to the relationship between sapwood area and DBH illustrated in Fig. 1. Similarly, the exponent of the function for native species (1.6017) was higher than that for exotic species (1.2251) as shown in Fig. 6, suggesting the higher enhancement of water transport for native species with the same DBH increment.
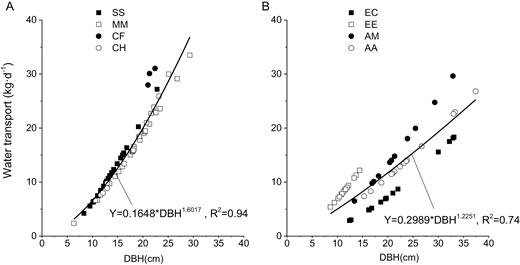
relationship between water transport and DBH for the native species (A) and exotic species (B). The meaning of abbreviations in the legend was listed in Table 1 (the same below).
Relationship between sap-flux density and conduit characteristics
Results presented in Fig. 7 showed the relation of water transport ability to conduit characteristics for the eight studied tree species. The relationship between sap-flux density and conduit length was not significant for the studied eight tree species with the P value of 0.127. The same was true for the relationship between sap-flux density and conduit diameter, density or conduit area/sapwood area ratio (P value was 0.545, 0.126 and 0.162, respectively).
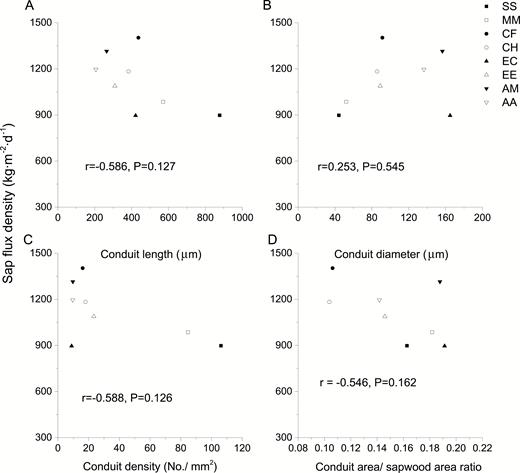
relationship between sap-flux density and conduit length (A), diameter (B), density (C) and conduit area/sapwood area ratio (D) for the eight studied tree species.
Sensitivity of sap-flux density to environmental factors and its relationship with conduit characteristics
We found that there existed a linear relationship between sap-flux density and PAR or VPD as shown in Fig. 8 and Fig. 9 (P < 0.05) with consideration of time lag. The slopes of the linear relationships suggest the sensitivity of sap-flux density to PAR or VPD. There existed no significant difference between native and exotic tree species in the sensitivity of sap-flux density to PAR and VPD (P > 0.05). The sensitivity to both PAR and VPD showed a positive relationship with conduit diameter, and a negative relationship with conduit length and density, reaching significance (P < 0.05) or marginal significance (P < 0.1) as shown in Fig. 8 and Fig. 9.
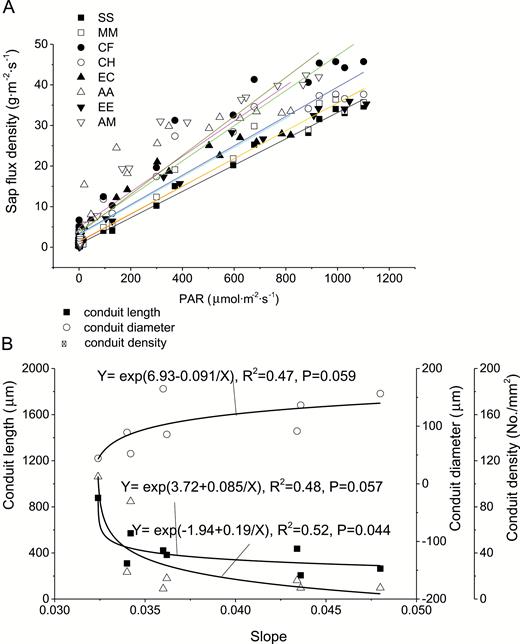
sensitivity of sap-flux density to PAR (A) and its relationship with conduit characteristics (B).
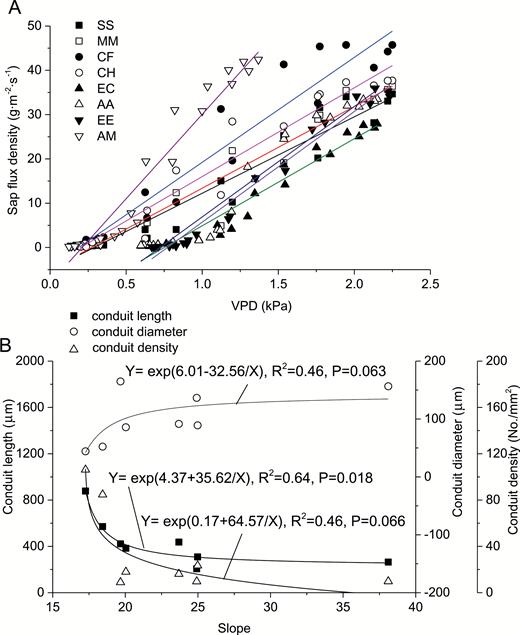
sensitivity of sap-flux density to VPD (A) and its relationship with conduit characteristics (B).
DISCUSSION
Sapwood area increased with the growing DBH as a power function for the eight studied tree species in our research. That DBH was an excellent predictor of sapwood area has been illustrated in other species as well, including boreal tree species (Bond-Lamberty et al. 2002), temperate broad-leaved tree species (Gebauer et al. 2008) and evergreen eucalypt trees in tropical regions (Eamus et al. 2000). At the same DBH, native species had a larger water-conducting tissue area than exotic species. Previous studies showed that adjustments of sapwood area occurred in response to site differences in supply and demand for water (Mencuccini and Grace 1995; Whitehead et al. 1984). In this study, the exotic species including E. citriodora, E. urophylla × grandis, A. auriculaeformis and A. mangium originally come from Australia where the climate is different from that in South China. The structural modifications over long time scales can be of the major reason for the diverse sapwood area of tree species that originate from different regions.
Small vessels are less prone to embolism (Choat et al. 2003; Gullo et al. 1995; Hacke and Sperry 2001; Hargrave et al. 1994) and have already been recognized as adaptations to avoid reduction of hydraulic capacity when cavitation occurred (Baas et al. 1983; Robert et al. 2009), while tree species with large conduit are considered to have low resistance to water flow, high conducting capacity and high risk of forming cavitation (Mauseth and Stevenson 2004). Native species had smaller conduit diameter than exotic species, suggesting that native tree species developed a safe water transport system while exotic fast-growing tree species formed an efficient system instead when merely considering conduit diameter. Moreover, scalariform plates are generally believed to enhance recovery of vessels after cavitation (Sperry 1986; Ellerby and Ennos 1998). For the studied tree species, scalariform plates were observed in S. superba and M. macclurei, suggesting that they have a better recovery capability after cavitation and safer water transport architecture.
Although there existed no significant difference in conduit density between native and exotic species, the native S. superba and M. macclurei had higher conduit density than other tree species. Schmitz et al. (2006) has demonstrated that a high vessel density optimizes the water transport under stressful conditions by remaining more vessels workable when the same number of vessels were embolized and enabling a more efficient bypass of air-filled vessels due to larger numbers of alternative pathways for water transport. Thus, S. superba and M. macclurei established a safer water transport system compared to other tree species based on the measured conduit density. Overall, native species are expected to be safer based on a physiological interpretation of the combination of measured conduit characteristics.
Relevant studies showed that increased vessel diameter promoted efficiency of water conduction dramatically (Tyree and Zimmermann 2013), and higher conduit density provided more alternative pathways resulting in a greater conductance for water transport (McCulloh et al. 2004; Tateishi et al. 2008). However, sap-flux density presented no significant relationship with conduit density or density in this research, suggesting that the increased water transport due to higher conduit density counteracted the relative low transport quantity because of small conduit diameter for those native species.
Water transport increased with the growing DBH as a power function, and the exponent for native species (1.60) was higher than that for exotic species (1.22). Although exotic fast-growing species had wide conduit diameter and therefore a higher efficiency of water conduction, the increment of water transport was lower with the same DBH increment. A larger increment of water-conducting tissue area along with the same DBH increase for native species as analyzed above may be responsible for its higher exponent of the power function. Consequently, native species presented a larger water transport at a larger DBH value under the combined effect of sap-flux density and sapwood area.
CONCLUSION
We conducted a research focusing on water utilization features along with xylem anatomy properties of native and exotic species in low subtropical China. Native species had a larger water-conducting tissue area than exotic species at the same DBH value when trees grew to a size with a certain value of DBH. Based on a physiological interpretation of measured conduit characteristics, native tree species developed a safe water transport system while exotic fast-growing tree species formed an efficient system instead. Native species presented a larger water transport at a larger DBH value under the synergistic effect of sap-flux density and sapwood area. The growth advantage of exotic fast-growing species may weaken as the DBH values increase.
ACKNOWLEDGEMENTS
This study was financially supported by the National Natural Science Foundation of China (Grant No. 41630752, 31130011, 41030638, 31300335), and the CAS/SAFEA International Partnership Program for Creative Research Teams. Sincere thanks to the staff in Heshan National Field Research Station of Forest Ecosystem, Chinese Academy of Sciences, and particular thanks to Zhipeng Chen who is coworker in the station.