-
PDF
- Split View
-
Views
-
Cite
Cite
Dasvinder Kambo, Ryan K Danby, Constraints on treeline advance in a warming climate: a test of the reproduction limitation hypothesis, Journal of Plant Ecology, Volume 11, Issue 3, June 2018, Pages 411–422, https://doi.org/10.1093/jpe/rtx009
- Share Icon Share
Abstract
Several mechanistic hypotheses have been developed to explain the existence of alpine treelines worldwide. The reproduction limitation hypothesis (RLH) postulates that reproductive processes such as seed production and dispersal are restricted in treeline environments, thereby limiting the establishment of new individuals in advance of extant treelines. Despite its popularity, no study has tested this hypothesis in a comprehensive fashion. In this experiment, we attempted to answer whether there are enough viable seeds being dispersed beyond treeline for sexually mediated treeline advance, and what the implications of climate change might be on these processes.
We established 30 plots across two aspects (north vs. south) and three elevational habitats (forest, treeline and tundra) in a white spruce (Picea glauca) boreal forest-alpine tundra ecotone in southwest Yukon, Canada. In each plot, tree characteristics, seed production and predispersal damage were measured. Additionally, eight dispersal trays were positioned in each plot to measure seed rain, and germination trials with and without predation exclosures were constructed in a subset of plots to quantify dispersal and germination success.
Results were highly variable both temporally and spatially. In 2014, a mast year, 69% of adult trees produced cones compared to 0.4% in the following year. Higher density of trees in forest plots compared to treeline and tundra resulted in greater seed production at lower elevations. Across all plots, 88% of seeds were damaged before dispersal or were not viable. Treeline plots had significantly greater predispersal damage. Seed rain was greater in south-facing plots than north-facing plots. Less than 2% of seeds produced on the landscape were dispersed into Tundra plots, located 50 m above treeline. There was a net movement of seeds from the north-facing slope to the south at our study site, likely due to prevailing winds during the dispersal period. Germination counts were more than double on north-facing slopes and one-third higher inside exclosures. Cumulatively, the results provide some evidence for the RLH. Collectively, the high amount of predispersal damage and non-viable seeds, variability associated with dispersal and significant seed predation can functionally influence treeline dynamics. These findings suggest that global treeline distribution models, which rely largely on temperature, may not be entirely accurate for predicting treeline advance—at least at finer temporal scales. Many stochastic factors need to align temporally for successful advance, which is likely to result in a lag of many decades between the period of temperature amelioration and an increased number of trees beyond extant treelines.
INTRODUCTION
Global temperatures have been rising since the 1850s, culminating in a 0.85°C global average rise between 1880 and 2012 (IPCC 2013). As temperatures continue to increase, thermal influence will continue to impact global ecological communities and biome boundaries (ACIA 2004; Chapin et al. 1995; Kaplan and New 2006; Sturm et al. 2001). The single largest biome boundary that is predicted to be impacted is between the boreal forest and tundra, an ecotone referred to as treeline. Position of the treeline ecotone is largely correlated with a growing season mean root-zone temperature of 6–8°C (Körner and Paulsen 2004; Paulsen and Körner 2014). As climate change continues to push this rooting-zone isotherm further north and to higher elevations, treelines are also expected to advance (Grace et al. 2002).
Despite predictions of global treeline advance, a review by Harsch et al. (2009) demonstrated that treeline has advanced at only 87 of 166 sites since 1900 AD, despite temperature warming at a majority of them. The nonresponse of treelines to temperature has often been considered ‘lag’ (Rannow 2013), where individual trees have simply not had sufficient time to establish and fill the climatic niche. Körner (1998) identified categories of functional mechanisms to explain the presence of thermal treelines and these categories have subsequently been used by other researchers to contextualize treeline position and climate-treeline lag (Fajardo and Piper 2014; Kroiss and HilleRisLambers 2015; Millar et al. 2015; Rannow 2013). One of the categories, the reproduction limitation hypothesis (RLH), has gained much attention in suggesting that treeline lag may be caused by a wide range of reproductive-related mechanisms and four seed-related factors have been the focus of particular research attention: (i) limited seed production, (ii) non-viability and predispersal damage of seeds, (iii) limited seed dispersal, and (iv) seed predation and failure to germinate (Elliott 1979; Malanson 1997; Meunier et al. 2007; Munier et al. 2010; Sirois 2000; Piotti et al. 2009; Wheeler et al. 2011). Cumulatively, these constraints may play a large role in influencing the successful establishment of new individuals beyond treeline ecotones, thereby limiting potential treeline advance.
At lower latitudes and in low elevation boreal, spruce-dominated forests, seed production occurs cyclically through seed masts which serve as catalysts for mass germination, predation saturation and potential recruitment (Kelly 1994; Krebs et al. 2012; LaMontagne and Boutin 2007). In these habitats, there is a high degree of synchrony among individuals; all produce cones or all experience cone failure (Dale et al. 2011). In the predominately white spruce (Picea glauca) boreal forests of North America, studies have suggested that seed production is explained by precipitation and temperature (Juday et al. 2003; Krebs et al. 2012; Roland et al. 2014). Long-term studies in British Columbia (Canada) have linked episodic white spruce cone production to every seven years for low elevation and 12 years for high elevation studies, while low elevation sites in Alaska mast at 10–12 year frequencies (Coates et al. 1994, Juday et al. 2003). Regardless, because climate has been established as an important factor for seed production, a warming climate is therefore likely to influence reproductive output of trees at treeline (Roland et al. 2014). In elevational treeline studies, seed masts are less commonly reported, but do occur; Danby and Hik (2007a) assert that seed masts were responsible for episodic treeline advance in white spruce in Yukon. However, the synchrony between mast events at treeline and climate-correlated treeline advance has not been definitively established, and seed-related variables other than seed production may also influence treeline advance (Kroiss and HilleRisLambers 2015).
Predispersal damage to seeds, including underdeveloped seeds and insect predation, can act as a reproductive constraint to treeline advance by limiting the amount of viable seeds (Jameson et al. 2015; Sirois et al. 1999; Sirois 2000; Wilmking et al. 2012). However, despite its potential importance, few studies have quantified predispersal damage at treeline and its constraints on advance are not well understood (Payette et al. 1982). The third reproductive constraint is dispersal. Limited seed dispersal from source populations can, when combined with other factors, lead to stagnant conifer treelines (Dufour-Tremblay et al. 2012). In long-term models, dispersal limitation has been shown to significantly decrease rate of potential treeline advance (Dullinger et al. 2004). In some studies, suboptimal dispersal has been shown to limit treeline advance to as little as 30 m over 1000 years, despite ideal climate conditions (Slatyer and Noble 1992). Conversely, some alpine sites have demonstrated long distance spruce seed dispersal greater than 300 m elevation (Piotti et al., 2009), suggesting a high degree of local and regional differences.
The fourth seed-related mechanism associated with the RLH is predation. Seed predation can negatively influence treeline by decreasing the number of viable seeds on the landscape available for germination and subsequent recruitment. It has also been shown to damage germinants, potentially leading to changes in plant community composition at treeline (Herrero et al. 2011; Moen et al. 2008). At some treeline sites, predation has been shown to account for the removal of 10–30% of tree seeds on the landscape (Munier et al. 2010; Wheeler et al. 2011).
Each of the four reproductive constraints influencing the number of seeds available for germination have been studied individually in various treeline systems, but there has been no study to-date that has examined all of these factors at one site. This is clearly insufficient, as the relative seed losses attributable to each factor can only be identified when all factors are studied simultaneously. This study aimed to test the treeline RLH at a white spruce treeline in Canada’s Yukon Territory by quantifying seed losses from production through to germination, while also measuring dispersal, all in one location. The study was guided by one central research question: Are there ‘enough’ viable seeds being dispersed beyond treeline for sexually mediated advance? To answer that question, four testable research questions were identified:
(i) How many seeds are produced in subalpine forest and treeline zones?
(ii) How many seeds are lost due to predispersal damage?
(iii) How many seeds are being dispersed in each zone of the ecotone?
(iv) After accounting for predation, how many seeds can successfully germinate on the landscape?
Each of these components may be more or less limiting in different years and in different conditions. As such, we were also interested in quantifying variability across the landscape, not just across elevation, and in multiple years. To this end, we studied ecotones on both north and south-facing slopes, which are known to be substantially different in southwest Yukon (Danby and Hik 2007a; Harper et al. 2011), over the course of two years.
METHODS
Study area
This study was conducted in the Kluane Mountain Ranges of southwest Yukon (61.428°N, 139.485°W). Although balsam poplar and trembling aspen (Populus balsamifera and P. tremuloides) do exist, the dominant forest and treeline-forming species is white spruce (Picea glauca) and the first year of the study (2014) coincided with a known mast year. Along the east-west flowing creek, the treeline ecotone exists between 1320 and 1370 m elevation (Danby and Hik 2007a). The north-facing slope is characteristic of krummholtz treeline, while south-facing slope is characteristic of diffuse treeline (Harsch and Bader 2011). Plant species composition varies across the ecotone and between aspects. Sedges (Carex spp.) and feathermosses are the dominant ground cover on north-facing slopes whereas woody shrubs such as Salix spp., Betula glandulosa and Potentilla fruticosa are the dominant ground cover on south-facing slopes. The differences observed in community composition are due to active layer depth, soil temperature and solar insolation. Mean active layer depth at treeline is 80.1 cm (σm = 2.8), but varies spatially (Dearborn and Danby 2017; submitted). The closest meteorological station is at Burwash Landing (61.371°N, 139.040°W; 806 m elevation), 26 km to the southeast.
There have been multiple research projects in the Yukon–Alaska region studying treeline advance in areas previously and currently underlain by permafrost (Lloyd et al. 2003; Lloyd and Fastie 2003; Roland et al. 2013). Several of these studies have indicated that north and south-facing slopes have responded differently to climate warming (e.g. Danby and Hik 2007a; Dial et al. 2007; Stueve et al. 2011). Contemporary treeline advance in the region may also be linked to shrub advance (Dial et al. 2016), especially on the south-facing slopes where shrub density is higher (Dearborn and Danby 2017; submitted), suggesting that future changes in the boreal-tundra ecotone may involve changes in species composition and not just distribution (Roland et al. 2016).
Experimental design
Thirty 900 m2 plots (30 × 30 m) were established in a full factorial design in July 2014 with aspect (north vs. south-facing slopes) and habitat (forest, treeline and tundra) as independent factors and plot as a random factor (n = 5 plots per aspect:habitat combination; Fig. 1). The north and south-facing slopes occurred within the same mountain valley and were directly across from each other. Treeline plots were established first on both aspects in comparable areas of tree density (mean Nelev: 1354 m elevation (SD: 8.75), mean Selev: 1351 m elevation (SD: 12.40)), and defined as the highest elevational area where individuals had evidence of cone production or had a minimum height of 2 m. Although this definition is inconsistent with some other treeline studies that use only tree height to characterize treeline position (Kullman 1979), we believed it was necessary to use cone production as this study aims to highlight sexual reproductive constraints of treeline advance. Treeline plots on the same aspect were ~200 m from one another. The subsequent forest and tundra plots were established at a minimum elevational range of 50 m below and above respective treeline plots (mean 70.8 m).

schematic diagram of the experimental design. A total of 30 plots, measuring 30 × 30 m, were constructed and equally divided by aspect and habitat (n = 5) in SW Yukon, Canada. Each plot contained eight seed collection trays for measuring dispersal and eight sandpaper squares (each with 10 seeds) for measuring herbivory.
Seed production and predispersal damage
In each of the forest and treeline plots (n = 20), the number of trees was counted and their height and diameter measured. A handful of trees in each plot existed as multiple stems emanating from the same root system; these individuals were classified as one tree. The number of cones on each tree was counted in the field when estimates were fewer than 50 per tree. When field estimates exceeded 50, a visual index, developed specifically for white spruce, was used (LaMontagne et al. 2005). Digital photographs were obtained of each tree canopy with cones. The number of cones on each tree was then counted using the batch cell counter feature in Image J software (v.1.50a July 2015; National Institute of Mental Health).
Five trees were randomly selected from each plot for cone harvesting during a 3-day period in late August 2014. Ten cones were harvested from each tree (hereafter referred to as a sample), placed in paper bags and transported to Queen’s University where they were kept at room temperature for 30 days until they were fully open and then subsequently refrigerated at 5°C for four months.
Seeds were separated from cones using an agitation table and seed wings were removed by hand. Although other less labour-intensive methods have been used in seed processing, this manual process was necessary to minimize any damage that might affect subsequent germination tests. Seeds were individually counted by hand on a white tray (for increased contrast). The isolated, cleaned seeds were weighed and subsequently placed in a paper envelope and returned to the refrigerator for storage.
Seeds were assigned to one of six categories: physical damage, empty, aborted, fungal damage, non-viable and potential to germinate. ‘Physical damage’, defined as having holes or partially missing seed coat, was assessed by randomly selecting 100 seeds per sample and individually determining presence or absence of damage using a hand lens. The number of damaged seeds was divided by 100 to yield physical damage as a proportion (PD). To confirm zero viability, 100 damaged seeds were randomly selected for germination trials, yielding 0% success.
Fifty seeds without physical damage were randomly chosen from each sample for germination trials. Seeds were laid on a petri dish lined with cotton and placed in a germination chamber (daily conditions: 16 hours at 25°C, 70% humidity with 80 mmol/m2 light; 8 hours at 15°C, 75% humidity in darkness; Jameson et al. 2015; Sirois 2000). Watering of seeds and monitoring for cotyledon emergence occurred daily. After a total of 21 days, 72 hours with no-net changes, three responses were recorded: emerged cotyledon (‘potential to germinate’; PTG), cracked seeds with no cotyledon (‘non-viable’; NVI1) and no change (NC). Ten randomly selected seeds marked ‘no change’ were collected from each petri dish for additional analysis. These seeds were dissected under a microscope and placed into one of four categories: no gametophyte + no embryo (‘empty seeds’; ES), gametophyte + no embryo (‘aborted seed’; AB), mold/blackened seed interior (‘fungal damage’; FG) and healthy gametophyte + embryo (‘non-viable’; NVI2). The number of empty, aborted and fungal damaged seeds from each sample were divided by 10 to yield proportion damage of each respective type (since 10 NC seeds were randomly selected from each sample for this analysis), multiplied by NC and divided by 50 to yield an estimated proportion of damaged type per sample (since 50 undamaged seeds were chosen for germination trials). The proportion damage of NVI2 was multiplied by NC, summed with NVI1, and divided by 50 to yield the estimated proportion of non-viable seeds per sample. The number of seeds with emerged cotyledons (PTG) was divided by 50 to yield an estimated proportion of seeds per tree with the potential to germinate.
All values were scaled to a per cone level by dividing by 10 (the number of cones per sample), and then scaled to a plot level by multiplying the per cone value by the number of cones counted within each plot. Plot-level values were then expressed on a per 1000 m2 basis by dividing by 0.9 (since all plots were 900 m2) in order to more easily facilitate comparison with other published studies.
Seed dispersal
In August 2014, eight aluminum trays (52.7 × 32.5 cm), lined with gardening felt and covered with 2.5 cm mesh chicken wire were installed in each of the 30 plots (n = 240). For each plot, five 30 m transects were laid out parallel to the slope. At 5 m intervals, presence/absence of erect shrubs (≥30 cm) was recorded to yield an estimate of shrub cover per plot. Trays were placed either under shrubs or in the open accordingly. Wherever possible, a standardized configuration was used (Fig.1). Trays were left over winter and contents collected in July 2015 and again in August 2016. Spruce seeds were counted for each tray, summed by plot, multiplied by plot area (900 m2) and divided by 0.9 to yield seed rain per 1000 m2 per year.
Seed germination and predation
Predation was assessed over two temporal scales: late summer predation and annual predation. To account for late summer predation, 10 seeds were glued to medium grit sandpaper (5 × 5 cm) using a non-toxic aerosol adhesive, a method derived from Marino et al. (2005) and Wheeler et al. (2011). Eight sandpaper squares were distributed throughout each plot over a 6-week period beginning in July 2014. The number of seeds removed from each sandpaper square was used as an indicator of predation pressure. To assess annual predation, 60 seed germination quadrats, each measuring 50 × 50 cm, were constructed in a 3-factor design (n = 5): (i) aspect (north, south), (ii) habitat (forest, treeline and tundra) and (iii) treatment (exclosed, open). Complete exclosures were constructed around half of the germination quadrats using wooden stakes and 2.5 cm mesh chicken wire. The size of the mesh was chosen to keep out vertebrate predators while simultaneously allowing seeds to fall through naturally at similar volumes compared to open quadrats. This allowed for comparisons to be made between exclosed and open quadrats. The seeds used for field germination were collected in Watson Lake, Yukon (60.0°N, 128.8°W) in 2011 and stored at the National Tree Seed Centre in Fredericton (New Brunswick, Canada; NTSC number: 9275443.0). The NTSC in-lab germination success rate was 69%. For each quadrat, 100 seeds were sown on the surface of the moss/soil (underneath the litter layer) in August 2014. Initial germination success was measured by counting the number of emerged germinants per quadrat in July 2015. Germinant survival was measured by counting the number of germinants present in 2016 and dividing it by the initial germination counts.
Statistical analysis
Statistical analyses were carried out using R (version 3.2.1, 2015). All data were tested for normality and homogeneity of variance before analysis; tree density, seed and cone numbers were log transformed to fit parametric test assumptions. To avoid artificially inflating degrees of freedom, values were averaged at the plot level for statistical analysis.
Tree density and size, seed and cone data were analyzed using a two-factor analysis of variance (ANOVA) to test for differences between aspect and habitat. When significant interaction terms were present, aspect and habitat were collapsed to a single factor (e.g. North Forest, South Forest, North Treeline, South Treeline), and analyzed with an ANOVA and post-hoc Tukey HSD to explore the interactive effects (Quinn and Keough 2002; Rosnow and Rosenthal 1989). We felt this was a necessary step to explore significant biological differences in aspect:habitat combinations, which could have implications for treeline in a changing climate.
A two-factor, multinomial logistic regression was used to determine predispersal damage differences between aspect and habitat. For this design, each sampled seed was treated as an observation and pooled by aspect:habitat combination. A multinomial regression was necessary to determine the probability of seed damage for each aspect:habitat combination in a given damage class. Six single factor ANOVAs were used to determine whether seed loss by damage type differed amongst each aspect:habitat combination.
A two-factor ANOVA was used to compare seed rain amongst aspect and habitat. To determine whether seed rain was significantly different than seed production, two sample t-tests were used for each aspect:habitat combination. A three-factor ANOVA was used to compare germination differences amongst predation treatments, aspect and habitat. A two-factor ANOVA was used to measure germinant survival among aspect and habitat.
RESULTS
Seed production and predispersal damage
Tree density was 6.3 times greater (67.6 vs. 10.8 trees/1000 m2) in forest plots compared to treeline plots (P < 0.0001) with no significant difference between aspects. This did not change when clumps of trees with multiple stems were treated as single individuals. Diameter at breast height was 6.3 cm and 4.2 cm greater on south-facing slopes (P = 0.0003) and in forest plots (P = 0.008), respectively. Tree height differences presented with a significant interaction term (P = 0.03); thus, a single interactive ANOVA was conducted in which the only significant result was from north-facing treeline plots, which were 3.2 m shorter compared to other plots (P < 0.0001; Fig. 2).

mean tree height (in meters) for each aspect × habitat combination. Abbreviations: FN = forest-north aspect, FS = forest-south aspect, TN = treeline-north aspect, and TS = treeline-south aspect. Error bars represent 1 standard error.
In 2014, out of 705 adult trees, 488 produced cones (69%), whereas in 2015 only three trees produced cones (0.4%). Thus, the remainder of the results presented here are values from 2014. The number of seeds produced was 5.5 times higher (2.9 × 105 vs. 5.4 × 104 per 1000 m2) in forest compared to treeline plots (P < 0.0001; outlier removed), with aspect playing no significant role (P = 0.56). This difference was driven by the higher number of cones per plot (P < 0.0001), a function of the higher tree density, as cones per tree and seeds per cone were not significantly influenced by aspect or habitat (P > 0.05). Seed and cone measurements were significantly correlated with tree size (Table 1). Germination success was positively correlated with cone length (r = 0.271, P = 0.007), seed weight (r = 0.417, P < 0.0001), number of seeds per cone (r = 0.308, P = 0.002) and tree height (r = 0.257, P = 0.01).
a two-tailed Pearson correlation coefficient matrix between cone, seed and tree variables
. | Cone width . | Seed weight . | Seed count . | Tree DBH . | Tree height . | Germinated seeds . |
---|---|---|---|---|---|---|
Cone length | **0.487 | **0.533 | **0.399 | 0.216 | 0.190 | *0.271 |
Cone width | **0.381 | **0.384 | 0.034 | 0.063 | 0.117 | |
Seed weight | **0.577 | 0.111 | 0.224 | **0.417 | ||
Seed count | 0.022 | 0.068 | *0.308 | |||
Tree DBH | **0.621 | 0.159 | ||||
Tree height | 0.257 |
. | Cone width . | Seed weight . | Seed count . | Tree DBH . | Tree height . | Germinated seeds . |
---|---|---|---|---|---|---|
Cone length | **0.487 | **0.533 | **0.399 | 0.216 | 0.190 | *0.271 |
Cone width | **0.381 | **0.384 | 0.034 | 0.063 | 0.117 | |
Seed weight | **0.577 | 0.111 | 0.224 | **0.417 | ||
Seed count | 0.022 | 0.068 | *0.308 | |||
Tree DBH | **0.621 | 0.159 | ||||
Tree height | 0.257 |
Total number of samples for each correlation is 99. Bold refers to significance of P <0.05, single asterisk refers to significance of P <0.01, and double asterisk refers to significance of P <0.001. Abbreviation: DBH, diameter at breast height.
a two-tailed Pearson correlation coefficient matrix between cone, seed and tree variables
. | Cone width . | Seed weight . | Seed count . | Tree DBH . | Tree height . | Germinated seeds . |
---|---|---|---|---|---|---|
Cone length | **0.487 | **0.533 | **0.399 | 0.216 | 0.190 | *0.271 |
Cone width | **0.381 | **0.384 | 0.034 | 0.063 | 0.117 | |
Seed weight | **0.577 | 0.111 | 0.224 | **0.417 | ||
Seed count | 0.022 | 0.068 | *0.308 | |||
Tree DBH | **0.621 | 0.159 | ||||
Tree height | 0.257 |
. | Cone width . | Seed weight . | Seed count . | Tree DBH . | Tree height . | Germinated seeds . |
---|---|---|---|---|---|---|
Cone length | **0.487 | **0.533 | **0.399 | 0.216 | 0.190 | *0.271 |
Cone width | **0.381 | **0.384 | 0.034 | 0.063 | 0.117 | |
Seed weight | **0.577 | 0.111 | 0.224 | **0.417 | ||
Seed count | 0.022 | 0.068 | *0.308 | |||
Tree DBH | **0.621 | 0.159 | ||||
Tree height | 0.257 |
Total number of samples for each correlation is 99. Bold refers to significance of P <0.05, single asterisk refers to significance of P <0.01, and double asterisk refers to significance of P <0.001. Abbreviation: DBH, diameter at breast height.
Across all plots, 88% of all seeds were either non-viable or affected by predispersal damage; north-facing treeline plots averaged the highest seed loss due to these factors, equivalent to 95% (Fig. 3). Across all damage types, forest plots, compared to treeline, were 46.8% less likely to have non-viable seeds or seed damage (P < 0.0001), while north-facing plots, compared to south-facing, were 26% more likely to have non-viable seeds or seed damage (P < 0.0001). With the exception of non-viable seeds, the trend of increased probability of damage in forest and north-facing plots held when damage was considered individually by type (Table 2). However, there were differences in the characterization of damage for each aspect:habitat combination (Fig. 4).
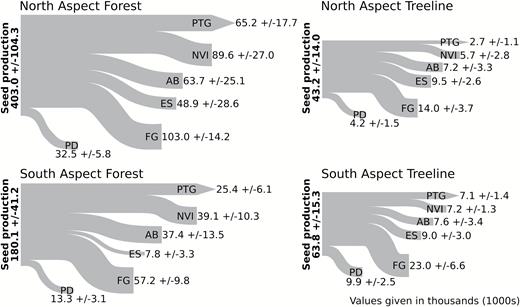
flow diagram illustrating the proportion of total seed production lost to each predispersal damage type in the four aspect × habitat combinations. Values represent mean values ± 1 standard error, scaled to a per 1000 m2 basis. Abbreviations: AB = aborted seeds, ES = empty seeds, FG = fungal damage, NVI = non-viable seeds, PD = physical damage, PTG = potential to germinate.
output from multinomial logistic regression summarizing the probability of predispersal damage type across aspect and habitat
Damage type . | Log-odds estimates (b) . | W-statistic . | Odds ratio . |
---|---|---|---|
Physical damage | |||
Foresthabitat | −0.78 | **81.1 | 0.46 [0.39, 0.55] |
NorthAspect | 0.21 | 5.9 | 1.23 [1.04, 1.45] |
Aborted seeds | |||
Foresthabitat | −0.35 | **19.2 | 0.70 [0.60, 0.82] |
NorthAspect | 0.24 | **9.3 | 1.27 [1.09, 1.48] |
Non-viable seeds | |||
Foresthabitat | 0.03 | 0.2 | 1.03 [0.88, 1.21] |
NorthAspect | 0.15 | 3.8 | 1.16 [1.00, 1.35] |
Empty seeds | |||
Foresthabitat | −1.6 | **322.8 | 0.20 [0.17, 0.24] |
NorthAspect | 0.75 | **79.83 | 2.12 [1.80, 2.50] |
Fungal damage | |||
Foresthabitat | −0.7 | **101.38 | 0.49 [0.43, 0.57] |
NorthAspect | 0.1 | 2.14 | 1.11 [0.97, 1.27] |
Damage type . | Log-odds estimates (b) . | W-statistic . | Odds ratio . |
---|---|---|---|
Physical damage | |||
Foresthabitat | −0.78 | **81.1 | 0.46 [0.39, 0.55] |
NorthAspect | 0.21 | 5.9 | 1.23 [1.04, 1.45] |
Aborted seeds | |||
Foresthabitat | −0.35 | **19.2 | 0.70 [0.60, 0.82] |
NorthAspect | 0.24 | **9.3 | 1.27 [1.09, 1.48] |
Non-viable seeds | |||
Foresthabitat | 0.03 | 0.2 | 1.03 [0.88, 1.21] |
NorthAspect | 0.15 | 3.8 | 1.16 [1.00, 1.35] |
Empty seeds | |||
Foresthabitat | −1.6 | **322.8 | 0.20 [0.17, 0.24] |
NorthAspect | 0.75 | **79.83 | 2.12 [1.80, 2.50] |
Fungal damage | |||
Foresthabitat | −0.7 | **101.38 | 0.49 [0.43, 0.57] |
NorthAspect | 0.1 | 2.14 | 1.11 [0.97, 1.27] |
The reference predispersal damage category for the analysis is ‘potential to germinate’. Treeline habitat and south-facing slopes were compared to forest habitat and north-facing slopes, resulting in the same estimates (statistical redundancy), thus they were removed from the table. The bold/italics of the Wald statistics (W), refers to significance of P <0.05, single asterisk refers to significance of P <0.01, and double asterisk refers to significance of P <0.001. The odds ratio (exp (b)) has been recorded along with the 95% confidence interval in brackets.
output from multinomial logistic regression summarizing the probability of predispersal damage type across aspect and habitat
Damage type . | Log-odds estimates (b) . | W-statistic . | Odds ratio . |
---|---|---|---|
Physical damage | |||
Foresthabitat | −0.78 | **81.1 | 0.46 [0.39, 0.55] |
NorthAspect | 0.21 | 5.9 | 1.23 [1.04, 1.45] |
Aborted seeds | |||
Foresthabitat | −0.35 | **19.2 | 0.70 [0.60, 0.82] |
NorthAspect | 0.24 | **9.3 | 1.27 [1.09, 1.48] |
Non-viable seeds | |||
Foresthabitat | 0.03 | 0.2 | 1.03 [0.88, 1.21] |
NorthAspect | 0.15 | 3.8 | 1.16 [1.00, 1.35] |
Empty seeds | |||
Foresthabitat | −1.6 | **322.8 | 0.20 [0.17, 0.24] |
NorthAspect | 0.75 | **79.83 | 2.12 [1.80, 2.50] |
Fungal damage | |||
Foresthabitat | −0.7 | **101.38 | 0.49 [0.43, 0.57] |
NorthAspect | 0.1 | 2.14 | 1.11 [0.97, 1.27] |
Damage type . | Log-odds estimates (b) . | W-statistic . | Odds ratio . |
---|---|---|---|
Physical damage | |||
Foresthabitat | −0.78 | **81.1 | 0.46 [0.39, 0.55] |
NorthAspect | 0.21 | 5.9 | 1.23 [1.04, 1.45] |
Aborted seeds | |||
Foresthabitat | −0.35 | **19.2 | 0.70 [0.60, 0.82] |
NorthAspect | 0.24 | **9.3 | 1.27 [1.09, 1.48] |
Non-viable seeds | |||
Foresthabitat | 0.03 | 0.2 | 1.03 [0.88, 1.21] |
NorthAspect | 0.15 | 3.8 | 1.16 [1.00, 1.35] |
Empty seeds | |||
Foresthabitat | −1.6 | **322.8 | 0.20 [0.17, 0.24] |
NorthAspect | 0.75 | **79.83 | 2.12 [1.80, 2.50] |
Fungal damage | |||
Foresthabitat | −0.7 | **101.38 | 0.49 [0.43, 0.57] |
NorthAspect | 0.1 | 2.14 | 1.11 [0.97, 1.27] |
The reference predispersal damage category for the analysis is ‘potential to germinate’. Treeline habitat and south-facing slopes were compared to forest habitat and north-facing slopes, resulting in the same estimates (statistical redundancy), thus they were removed from the table. The bold/italics of the Wald statistics (W), refers to significance of P <0.05, single asterisk refers to significance of P <0.01, and double asterisk refers to significance of P <0.001. The odds ratio (exp (b)) has been recorded along with the 95% confidence interval in brackets.

mean percentage seed loss attributed to the six categories of predispersal damage for each aspect × habitat combination. Error bars represent 1 standard error. Abbreviations: PD = physical damage, AB = aborted seeds, NVI = non-viable seeds, ES = empty seeds, FG = fungal damage, PTG = potential to germinate, FN = forest-north aspect, FS = forest-south aspect, TN = treeline-north aspect, TS = treeline south aspect.
Seed dispersal
Year 1 seed rain was 6.9 and 93.3 times greater in forest plots compared to treeline and tundra plots, respectively (P < 0.0001). Treeline plots had 13.5 times greater seed rain than tundra plots. Across all plots, the south aspect had 1.4 times greater seed rain compared to the north aspect (P = 0.04). The difference between seed rain and production was significant in forest-north plots only (P = 0.04); however, non-significant trends demonstrated that tundra, south-aspect forest and treeline plots tended to be seed sinks, while north-aspect forest and treeline plots tended by seed sources (Table 3). Year 2 seed rain was minimal across all plots; only nine seeds were found in all dispersal trays combined, of which five were in forest-south, three were in forest-north and one in treeline-south plots.
the difference between seed production and dispersal for each aspect × habitat combination
. | South . | North . |
---|---|---|
Tundra | 0 | 0 |
4310 (1771) | 2219 (996) | |
+4310 | +2219 | |
Treeline | 63766 (15258) | 43213 (14014) |
71427 (29193) | 16472 (7210) | |
+7661 | −26741 | |
Forest | 180196 (41232) | 402931 (104273) |
332295 (60360) | 277110 (67220) | |
+152099 | −125821 |
. | South . | North . |
---|---|---|
Tundra | 0 | 0 |
4310 (1771) | 2219 (996) | |
+4310 | +2219 | |
Treeline | 63766 (15258) | 43213 (14014) |
71427 (29193) | 16472 (7210) | |
+7661 | −26741 | |
Forest | 180196 (41232) | 402931 (104273) |
332295 (60360) | 277110 (67220) | |
+152099 | −125821 |
Values are number of seeds per 1000 m2. The top value in each cell refers to mean seed production, the middle value refers to mean seed rain, and the bottom (bolded) value is the difference (mean seed rain − mean seed production). Values in brackets represent one standard error.
the difference between seed production and dispersal for each aspect × habitat combination
. | South . | North . |
---|---|---|
Tundra | 0 | 0 |
4310 (1771) | 2219 (996) | |
+4310 | +2219 | |
Treeline | 63766 (15258) | 43213 (14014) |
71427 (29193) | 16472 (7210) | |
+7661 | −26741 | |
Forest | 180196 (41232) | 402931 (104273) |
332295 (60360) | 277110 (67220) | |
+152099 | −125821 |
. | South . | North . |
---|---|---|
Tundra | 0 | 0 |
4310 (1771) | 2219 (996) | |
+4310 | +2219 | |
Treeline | 63766 (15258) | 43213 (14014) |
71427 (29193) | 16472 (7210) | |
+7661 | −26741 | |
Forest | 180196 (41232) | 402931 (104273) |
332295 (60360) | 277110 (67220) | |
+152099 | −125821 |
Values are number of seeds per 1000 m2. The top value in each cell refers to mean seed production, the middle value refers to mean seed rain, and the bottom (bolded) value is the difference (mean seed rain − mean seed production). Values in brackets represent one standard error.
Seed germination and predation
Results from the late summer predation experiment showed significant differences with respect to aspect only (P < 0.0001), with south-facing slopes having 14% more predation than north-facing slopes. However, these results were driven by high values on tundra-south (interaction; P < 0.0001). The annual predation experiment yielded different results, where significant differences were detected with respect to aspect, habitat, treatment and the interaction between aspect and habitat. Germination patterns did not change between aspect and habitat; rather, significant interaction term in the model was driven by low germination count on the south-facing slope. When aspect and habitat were combined as a single factor within the model, the significant interaction between treatment and group disappeared. Statistical significance was detected with respect to aspect (P < 0.0001), habitat (P < 0.0001) and predation treatment (P = 0.0009). Germination count was 142% and 34% greater on north-facing slopes and in exclosed quadrats, respectively. Germination count at treeline was 32% and 71% greater than at forest and tundra habitats, respectively (Fig. 5). Germinant survival after one year showed significant differences with respect to aspect (P < 0.0001) and habitat (P < 0.0001), but not predation treatment (P = 0.704). Survival was 47% higher on north-facing slopes and 17% and 18% higher in treeline plots compared to the forest and tundra plots, respectively (Table 4).
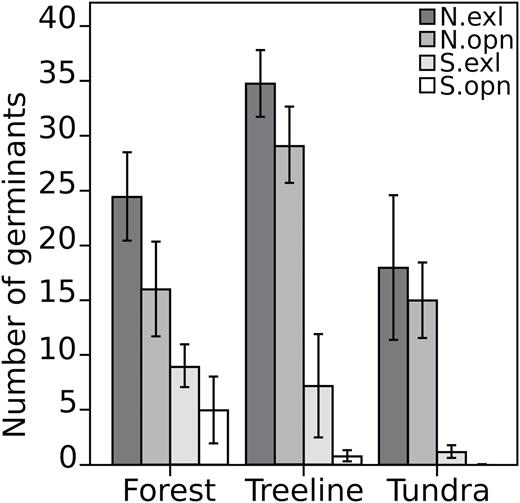
mean number of germinants per plot (±1 standard error) in the field germination experiment. One hundred seeds, collected in Watson Lake, YT, by the National Tree Seed Centre, were planted in a 3-factor design (aspect × habitat × treatment). The number of germinants was counted one year later. Lab germination rate was 69%. Abbreviations: N.exl = north aspect with herbivory exclosure, N.opn = north aspect without exclosure, S.exl = south aspect with herbivory exclosure, S.opn = south aspect without exclosure.
the number of germinants in 2015 and 2016 for each aspect × habitat × treatment combination
. | . | North . | Survival (%) . | South . | Survival (%) . | ||
---|---|---|---|---|---|---|---|
. | . | 2015 . | 2016 . | 2015 . | 2016 . | ||
Forest | Open | 16.0 (4.3) | 9.2 (2.3) | 57.5 | 6.3 (3.6) | 0.8 (0.6) | 12.7 |
Exclosed | 24.5 (4.0) | 14.5 (2.3) | 59.2 | 9.0 (1.9) | 1.6 (0.6) | 17.8 | |
Treeline | Open | 29.2 (3.5) | 21.8 (2.7) | 74.7 | 2.0 (0.0) | 0.2 (0.2) | 10.0 |
Exclosed | 34.8 (3.1) | 27.8 (2.4) | 80.0 | 7.2 (4.7) | 1.4 (0.9) | 20.4 | |
Tundra | Open | 15.0 (3.4) | 6.4 (1.6) | 42.7 | 2.0 (0.6) | 0.0 (0.0) | 0 |
Exclosed | 18.0 (6.6) | 8.8 (3.2) | 48.9 | 0.0 (0.0) | 0.0 (0.0) | n/a |
. | . | North . | Survival (%) . | South . | Survival (%) . | ||
---|---|---|---|---|---|---|---|
. | . | 2015 . | 2016 . | 2015 . | 2016 . | ||
Forest | Open | 16.0 (4.3) | 9.2 (2.3) | 57.5 | 6.3 (3.6) | 0.8 (0.6) | 12.7 |
Exclosed | 24.5 (4.0) | 14.5 (2.3) | 59.2 | 9.0 (1.9) | 1.6 (0.6) | 17.8 | |
Treeline | Open | 29.2 (3.5) | 21.8 (2.7) | 74.7 | 2.0 (0.0) | 0.2 (0.2) | 10.0 |
Exclosed | 34.8 (3.1) | 27.8 (2.4) | 80.0 | 7.2 (4.7) | 1.4 (0.9) | 20.4 | |
Tundra | Open | 15.0 (3.4) | 6.4 (1.6) | 42.7 | 2.0 (0.6) | 0.0 (0.0) | 0 |
Exclosed | 18.0 (6.6) | 8.8 (3.2) | 48.9 | 0.0 (0.0) | 0.0 (0.0) | n/a |
Values given for 2015 and 2016 are means with bracket values representing one standard error.
the number of germinants in 2015 and 2016 for each aspect × habitat × treatment combination
. | . | North . | Survival (%) . | South . | Survival (%) . | ||
---|---|---|---|---|---|---|---|
. | . | 2015 . | 2016 . | 2015 . | 2016 . | ||
Forest | Open | 16.0 (4.3) | 9.2 (2.3) | 57.5 | 6.3 (3.6) | 0.8 (0.6) | 12.7 |
Exclosed | 24.5 (4.0) | 14.5 (2.3) | 59.2 | 9.0 (1.9) | 1.6 (0.6) | 17.8 | |
Treeline | Open | 29.2 (3.5) | 21.8 (2.7) | 74.7 | 2.0 (0.0) | 0.2 (0.2) | 10.0 |
Exclosed | 34.8 (3.1) | 27.8 (2.4) | 80.0 | 7.2 (4.7) | 1.4 (0.9) | 20.4 | |
Tundra | Open | 15.0 (3.4) | 6.4 (1.6) | 42.7 | 2.0 (0.6) | 0.0 (0.0) | 0 |
Exclosed | 18.0 (6.6) | 8.8 (3.2) | 48.9 | 0.0 (0.0) | 0.0 (0.0) | n/a |
. | . | North . | Survival (%) . | South . | Survival (%) . | ||
---|---|---|---|---|---|---|---|
. | . | 2015 . | 2016 . | 2015 . | 2016 . | ||
Forest | Open | 16.0 (4.3) | 9.2 (2.3) | 57.5 | 6.3 (3.6) | 0.8 (0.6) | 12.7 |
Exclosed | 24.5 (4.0) | 14.5 (2.3) | 59.2 | 9.0 (1.9) | 1.6 (0.6) | 17.8 | |
Treeline | Open | 29.2 (3.5) | 21.8 (2.7) | 74.7 | 2.0 (0.0) | 0.2 (0.2) | 10.0 |
Exclosed | 34.8 (3.1) | 27.8 (2.4) | 80.0 | 7.2 (4.7) | 1.4 (0.9) | 20.4 | |
Tundra | Open | 15.0 (3.4) | 6.4 (1.6) | 42.7 | 2.0 (0.6) | 0.0 (0.0) | 0 |
Exclosed | 18.0 (6.6) | 8.8 (3.2) | 48.9 | 0.0 (0.0) | 0.0 (0.0) | n/a |
Values given for 2015 and 2016 are means with bracket values representing one standard error.
DISCUSSION
Our results demonstrate that in mast years the production and dispersal of seeds is sufficient enough that it should not limit treeline advance. Instead, seed loss due to predispersal damage and post-dispersal predation are more important limitations in these years. However, seed production and dispersal drop to effectively zero in non-mast years, and cumulative seed losses are higher on north-facing slopes and at the uppermost elevational habitats within the forest-tundra ecotone. This results in substantial temporal and spatial variability in the relative importance of reproductive factors limiting treeline advance, suggesting that even under favorable climate, treeline expansion can be limited.
Climate, specifically growing degree-days and maximum temperature of the warmest month, has been associated with reproductive potential in spruce (Messaoud et al. 2007; Meunier et al. 2007; Sirois et al. 1999), with 670–700 GDD5 (growing degree-days >5°C) being required for embryo filling in white spruce (Zasada et al. 1992). As climate warms, total seed production is therefore likely to increase; however, past research also suggests that seed predation may increase in a warming environment (Brown and Vellend 2014). In southwest Yukon, multiple predators exist that consume white spruce such as snowshoe hares, red-backed voles, deer mice, red squirrels, ground squirrels, ptarmigans and grouse (Boutin et al. 2006; Krebs et al. 2014). Some of these predators collect seeds directly from the tree (Archibald et al. 2012; Peters et al. 2003), leaving signs of physical damage. We did not find signs of large-scale damage, rather at our sites, physical damage was likely caused by the larvae of spruce cone flies (Strobilomyia neanthracina) or to a lesser extent, gall midges (Cecidomyiidae spp.), known seed predators for white spruce in northern British Columbia and southern Yukon (Deasley 2014). From 100 samples, 92% of trees had seeds with at least some physical damage associated with the presence of S. neanthracina larvae. Although it is unlikely that insects have strong selective pressures on white spruce cones in Yukon (Deasley 2014), there is evidence of increasing insect populations limiting expansion at other sites (Herrero et al. 2011), a trend which could increase with climate change.
The general characterization of predispersal damage was similar across all aspect:habitat combinations, with fungal damage responsible for the highest seed loss and physical damage responsible for the lowest. Without genetic analysis it is difficult to ascertain the type of fungus responsible. Other studies have shown similar blackened seeds with white spruce and have attributed it to seven different types of fungal damage (Mittal and Wang 1987). Additionally, due to the high level of fungal damage, it was difficult to observe whether the seeds contained embryos—thus, it is entirely likely that fungi-damaged seeds were also aborted seeds. The largest discrepancy in predispersal damage between aspect:habitat combination was with the number of empty seeds, in particular, the high proportion of no gametophytes in treeline compared to forest plots. Specifically, treeline-north plots had 23% of loss attributed to empty seeds, resulting in lower than average values for potential to germinate. The high values were likely due to pollination failure (Fowler and Park 1983).
Spruce is wind pollinated and pollination success is strongly tied to tree density and tree height (Arista and Talavera 1996; Fowler and Park 1983; Stewart et al. 1998; Zasada et al. 1978). Wind dispersed pollen has been shown to travel long distances (Ashley 2010; Clark et al. 1998), with some estimates demonstrating that white spruce pollen can travel up to 3 km (O’Connell et al. 2007); suggesting that the likelihood of genetic isolation at treeline, located 50 m elevation above forest plots, to be low. At our study site, there were no significant differences in tree density between aspects, suggesting that the high number of empty seeds in north-facing treeline plots is due to lower pollination rates in shorter trees. The smaller trees in the north-facing treeline plots grow in a more krummholtz-type fashion, a known response to high wind habitats (Körner 2003, 2012). This strategy may lead to increased individual survival, but also leads to lower fertilization.
Unsurprisingly, across all plots, seed rain was positively correlated with seed production (R2 = 0.61, P < 0.0001; Dobbs 1976; Piotti et al. 2009). Spruce cones open in late August or early September at high elevation sites in southwest Yukon. Climate and weather patterns greatly influence seed dispersal during that 2-week period, which occurs every 6–8 years during a mast cycle (Dobbs 1976; Krebs et al. 2012; Waldron 1965). Patch dynamics also influence dispersal successes, for example, long distance-dispersal events are increasingly more important in patchier treelines, such as the one found on our north-facing slopes (Lloyd et al. 2003). This is in contrast with more abrupt treelines, like those found on our south-facing slopes, which tend to be maintained by soil properties (Sullivan et al. 2015).
In our study, over a one-year period coinciding with a seed mast, there was measurable seed rain in tundra plots. However, the quality and origin of seeds from the dispersal trays could not be assessed due to a 100% failure rate, likely caused by over heating from the metal trays resulting in desiccation. Additionally, there was a net loss of seeds (production − seed rain) from the north-facing forest and treeline plots, and a net gain to the south-facing forest and treeline plots (Table 3). North-facing treeline plots had the largest discrepancy between seed production and seed rain (62%) compared to the other three groups. Two possible explanations for this trend exist: (i) seeds on the north-facing slopes disperse with greater ease and (ii) prevailing wind patterns. Dispersal ability is based on the interplay of many factors including seed weight, seed-wing size and height of seed release from seed tree (Holtmeier and Broll 2010). In our study, seed weight was lower on north-facing slopes (P = 0.05) and in treeline plots (P < 0.0001). These lighter seeds can travel further than the heavier seeds on the south-facing slopes under consistent low to mid-wind sites (Schopmeyer 1974; Zasada et al. 1978).
Second, there is evidence for a prevailing northerly wind pattern in the region (i.e. from north-facing to south-facing slopes) during the period of seed dispersal. Although synoptic-scale patterns in SW Yukon usually favour westerly winds in summer and northwesterly in winter (Klock and Mullock 2000), the presence of a predominant low-pressure system in the Gulf of Alaska (Nickling and Brazel 1985), multiple glaciers and strong topographic relief create complex wind patterns which make it difficult to discern local wind patterns. Past research in the Kluane region has demonstrated a heavy influence of the St. Elias Icefield and its radiating valley glaciers, resulting in predominantly south-southeasterly winds during late summer and early fall (Nickling and Brazel 1985), and wind data from the Environment Canada meteorological station in Burwash Landing (climate.weather.gc.ca), 26 km southeast of our study site confirms this. When wind data from Burwash Landing was analyzed within the specific seed dispersal period of our study (21 August to 15 September 2014), the average direction was 182° (mean speed: 17.7 km/h, SD: 8.6). Collectively, this provides evidence that the higher seed rain (relative to production) on the south-facing slope was a function of predominantly northerly winds. Still, it is important to note that while average wind direction provides some insight, there remains a high degree of annual, weekly and even daily stochasticity, in addition to temperature and precipitation patterns, that can influence seed dispersal. For example, high precipitation, strong downslope winds or below-average temperatures during August–September months could lead to below-average cone opening and/or complete dispersal failure.
After dispersal of viable seeds to a suitable location, the next major step in the potential advance of treeline is germination. Successful germination can be affected by several factors, including adequate temperature, moisture, substrate availability, protection from desiccating wind and predation (Fraser 1971; Green and Venn 2012; Hancock et al. 2005; Resler et al. 2005; Sveinbjörnsson et al. 2002). Germination count was more than double on the north-facing slope compared to the south-facing slope, which was a function of substrate differences. At our site, the ground cover of north-facing slopes consisted of feathermosses, which have been shown to have increased moisture retention and decreased temperature range compared to mineral or organic substrates (Wheeler et al. 2011), which were predominant on the south-facing slope. Additionally, germination count was greater in north-facing treeline plots compared to the forest or tundra plots. This could be a result of tradeoff between two factors. Low elevation forest plots are wind-protected and experience reduced soil temperature extremes due to shading, while more sunlight reaches substrate-level vegetation in tundra plots. Treeline plots could potentially provide advantages of both elevations. Differences in substrate could also explain why field germination results did not always match PTG numbers estimated in-lab. For example, PTG estimates were highest in forest-north plots and lowest in treeline-north plots. This underlines the importance of dispersal for germination success: the trees that produce the most viable seeds due to higher pollination success are not always located in areas where germination success is optimized. Germination count was also higher in exclosed quadrats compared to open quadrats throughout all plots, but the effect of predation was highest in forest and treeline plots, suggesting fewer seed predators in tundra plots. The hypothesis of decreasing effects of predation from source (in this case, forest) seed populations is well established in the literature and is commonly referred to as the Janzen-Connell hypothesis (Connell 1971; Janzen 1970). Although we did not actively observe predation, it was likely that post-dispersal damage in our study site was caused by snowshoe hares, voles, ground squirrels and mice (Dale et al. 2011; Deasley 2014; Krebs et al. 2014). Similar to initial germination count, seedling survivorship was also higher on the north-facing slopes and in treeline quadrats; however, there was no difference in survivorship between exclosed and open quadrats, suggesting post-dispersal predation may be driven by seed predators and not germinant herbivory (Krebs et al. 2014; Jameson et al. 2015). While germinant herbivory may not be critical in our study, others have shown it to be a potentially limiting factor for treeline advance, suggesting tree species and site-specific variation (Cairns and Moen 2004; Herrero et al. 2011; Speed et al. 2010).
Collectively, our results imply that north-facing slopes have an advantage for seedling establishment over south-facing slopes. The seed source for the tundra-north is likely forest-north, where the seeds are produced at more than double the amount compared to forest-south, and has the highest PTG. When combined with low levels of predation in tundra plots, this could explain why spruce seedling density at and above treeline is more than three times that measured on south-facing slopes (Danby and Hik 2007b).
CONCLUSION
Our results demonstrate that a large number of reproductive factors need to align temporally before significant treeline advance can occur; and while climate change will undoubtedly influence some of these factors, others are inherently stochastic and would not be directly affected by climate change in the short term. A significant amount of research has shown that climate can influence tree growth and seed production (Allen et al. 2014; Hacket-Pain et al. 2015), suggesting that a changing climate can potentially result in increased viable seed production and/or increased frequency of seed masts. But even if the magnitude or frequency of masts increases, our predispersal damage and viability assessments show that only 5–15% of seeds being produced in the forest-tundra ecotone currently have the capacity to germinate. An increase in viability would also be required to facilitate treeline advance. Continued climate warming may decrease the amount of empty or aborted seeds, but it could also increase the frequency of insect and/or fungal damage. During the short period of cone opening in mast years, weather patterns which are suboptimal for dispersing seed beyond treeline habitat could lead to a complete absence of seed dispersal into the tundra until the next major cone-producing year; this highlights the importance of mast frequency. Upon successful dispersal, seeds must land on substrate that retains a sufficient amount of moisture and must experience optimum temperature for germination; both of which are annually highly variable and more likely to be found on the north-facing slope. Seeds must also contend with predation before they can successfully germinate. Germinants that do successfully establish must then contend with other subsequent factors that reduce survivorship, including the harsh climatic conditions beyond treeline (e.g. Danby and Hik 2007b). The sum of these processes may potentially explain why, despite climate warming at many sites globally, fewer treelines have advanced than might be expected. Ultimately, the stochastic nature of reproductive mechanisms may outweigh the deterministic effects of climate warming at many of these sites.
ACKNOWLEDGEMENTS
We would like to thank the Kluane First Nation and Lù’àn Män Ku Dän (Kluane Lake People) for giving us permission for this research to be conducted on their land. We would also like to thank the Arctic Institute of North America’s Kluane Lake Research Station and its managers, Sian Williams and Lance Goodwin, for logistical support as well as Courtenay Jacklin and Katherine Dearborn for field assistance. Funding for this project was provided by the Natural Sciences and Engineering Research Council of Canada, Canada Foundation for Innovation, The Royal Canadian Geographical Society, and Canadian Polar Commission.
Conflict of interest statement. None declared.
REFERENCES
IPCC (