-
PDF
- Split View
-
Views
-
Cite
Cite
Mary E. Wikswo, Renjie Hu, Gregory A. Dasch, Laura Krueger, Aaron Arugay, Keith Jones, Barry Hess, Stephen Bennett, Vicki Kramer, Marina E. Eremeeva, Detection and Identification of Spotted Fever Group Rickettsiae in Dermacentor Species from Southern California , Journal of Medical Entomology, Volume 45, Issue 3, 1 May 2008, Pages 509–516, https://doi.org/10.1093/jmedent/45.3.509
- Share Icon Share
Abstract
Dermacentor occidentalis Marx and Dermacentor variabilis (Say) commonly bite humans in California. These Dermacentor species may play a role in transmitting spotted fever group (SFG) rickettsiae to humans in many parts of the state where Dermacentor andersoni Stiles, a known vector for the etiologic agent of Rocky Mountain spotted fever, Rickettsia rickettsii, is absent. However, the specific rickettsial agents present in these ticks and their current prevalence are poorly understood. In total, 365 D. occidentalis and 10 D. variabilis were collected by flagging vegetation at 16 sites in five counties of southern California. The presence of SFG rickettsial DNA in these ticks was detected with rOmpA and GltA gene polymerase chain reaction (PCR) assays. The rickettsial species were identified by sequencing PCR amplicons. Of 365 D. occidentalis, 90 (24.7%) contained R. rhipicephali DNA, 28 (7.7%) contained DNA of unclassified genotype 364D, two (0.55%) contained R. bellii DNA, and one (0.3%) contained R. rickettsii DNA. Of 10 D. variabilis, four (40%) contained only R. rhipicephali. Four new genotypes of R. rhipicephali were discovered. For the first time, we detected R. rickettsii in D. occidentalis. Our study provides the first molecular data on the prevalence and species identification of SFG rickettsiae circulating in populations of these California ticks. Because neither D. variabilis nor R. rickettsii were abundant, 364D should be evaluated further as a potential cause of human SFG rickettsioses in southern California.
Rocky Mountain spotted fever (RMSF) is a potentially fatal human illness that is caused by Rickettsia rickettsii throughout the Americas. The primary vectors for RMSF in the United States are the American dog tick, Dermacentor variabilis (Say), in the eastern and central United States and limited areas of California, and the wood tick, Dermacentor andersoni Stiles, in the mountainous areas of the western United States and parts of southwestern Canada (Burgdorfer 1975). The majority of human RMSF cases in the United States have been attributed to transmission by one of these two vectors, but cases of RMSF also have been reported outside the known distribution of both D. andersoni and D. variabilis (Chapman et al. 2006). The first recorded human case of RMSF in California occurred in 1903 (Emmons 1973). Between 1903 and 1938, 188 cases were reported in California, nearly all in the Modoc Plateau area where D. andersoni occurs. From 1950 to 1973, 16 well-documented RMSF cases were reported outside the Modoc Plateau area, the areas where D. andersoni is absent, suggesting that other ticks might be responsible for occasional transmission of the disease in the state (Rotramel et al. 1976). More recently, 29 cases of RMSF in total were reported in California during 2002–2006, none of which were in the Modoc Plateau area and 14 of which occurred in southern California (CDHS 2006).
Rickettsia rickettsii also has been found in tick species other than D. variabilis and D. andersoni, including Rhipicephalus sanguineus (Latreille) within the continental United States (Demma et al. 2005, Garrison et al. 2007, Wikswo et al. 2007) and Amblyomma cajennense (F.), Amblyomma aureolatum (Pallas), and Haemaphysalis leporispalustris (Packard) in Central and South America (de Rodaniche 1953, Fuentes et al. 1985, Guedes et al. 2005, Acosta et al. 2006, Pinter and Labruna 2006). Moreover, laboratory studies have implicated several other tick species, including Dermacentor occidentalis Marx and Dermacentor parumapertus Neumann as possible vectors for R. rickettsii, both of which can be found in parts of southern California where RMSF has been reported (Rotramel et al. 1976, CDHS 2006).
In the early 1980s, spotted fever group (SFG) rickettsiae were detected in questing D. occidentalis collected in California using hemolymph testing, isolation, and serotyping by mouse serum microimmunofluorescence assay (Lane et al. 1981a,b, Philip et al. 1981), but the presence of R. rickettsii in this tick species has never been reported. The unnamed SFG rickettsia isolate 364D, which is commonly found in D. occidentalis from California, is a closely related but distinct serotype from R. rickettsii (Lane et al. 1981a,b; Philip et al. 1981). 364D seems to be less virulent than prototypical isolates of R. rickettsii in guinea pigs, and it has not been shown to cause human disease (Philip et al. 1981). However, it has been speculated that 364D may be responsible for causing a SFG human illness very similar to RMSF, especially in areas where R. rickettsii may not be present (Lane et al. 1981a).
In California, D. occidentalis is the tick most commonly found attached to humans (Merten and Durden 2000), and it is the tick most frequently collected from habitats without D. andersoni where recent RMSF cases were suspected to have been contracted (Rotramel et al. 1976). D. occidentalis is also one of the ticks most commonly found on vegetation in California (Rotramel et al. 1976; Lane et al. 1981a,b). D. variabilis, however, is much less likely to be found on humans (Merten and Durden 2000), and it tends to concentrate around urban centers, presumably because of denser canine populations (Rotramel et al. 1976). D. occidentalis plays a significant role in both human and animal diseases in the United States. The causative agents of tularemia, bovine anaplasmosis, and Q fever have been isolated from D. occidentalis, and D. occidentalis is the most significant cause of tick paralysis in deer and livestock in California (Parker et al. 1929, Herms and Howell 1936, Cox 1940, Loomis and Bushnell 1968). D. occidentalis is also a potential vector for the agents causing RMSF and Colorado tick fever (Brown et al. 2005).
The purpose of this study was to use molecular assays to determine the prevalence and genetic characteristics of tick-borne rickettsial agents currently circulating in human-biting ixodid ticks from southern California.
Materials and Methods
Collection of Ticks and DNA Preparation.
In total, 16 sites for tick collection were chosen from five counties in the southern part of California: Los Angeles, Orange, Riverside, Santa Barbara, and Ventura (Table 1). The criteria for site selection were as follows: 1) part of a long-term tick population study (two sites in Los Angeles and eight sites in Riverside), 2) potential exposure sites for RMSF cases (three sites in Orange), and 3) ongoing tick surveys (two sites in Santa Barbara and one site in Ventura) (Table 2). Adult questing D. variabilis and D. occidentalis were collected between 27 April and 28 June 2006 and 8 November 2006–29 January 2007 by the flagging method. Ticks were placed in sealed containers with moist paper and stored at ambient temperature until processed. Ticks were identified to species using standard taxonomic keys as described previously by Furman and Loomis (1984).
Tick collections and PCR assay results by site, county, gender, species, and total
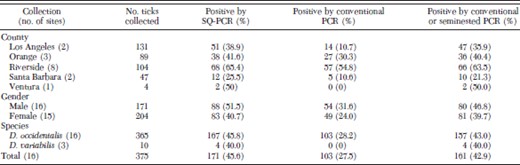
Tick collections and PCR assay results by site, county, gender, species, and total
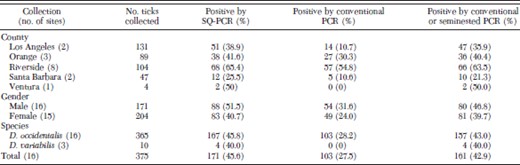
Origin and characteristics of SFG rickettsiae detected in Dermacentor ticks from southern California
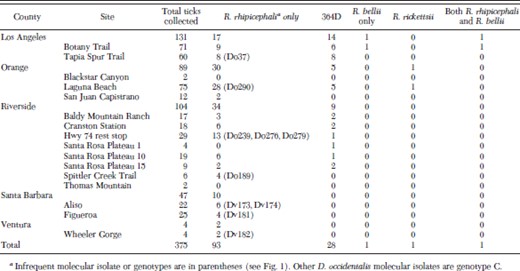
Origin and characteristics of SFG rickettsiae detected in Dermacentor ticks from southern California
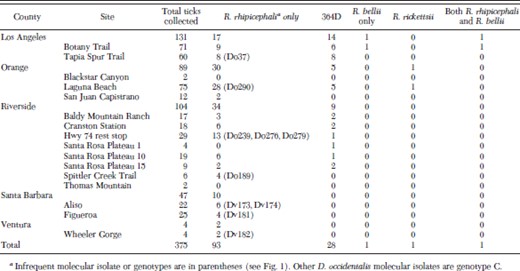
Ticks were individually surface disinfected in 10% bleach and 70% ethanol, washed three times with sterile distilled water, dried, and bilaterally bisected. One half was preserved in SRM buffer (0.218 M sucrose, 5 mM potassium glutamate buffer, pH 7.0, supplemented with 1% Renografin-76 [E.R. Squibb & Sons, Inc., Princeton, NJ] and 5 mM MgCl2) and kept at −80°C for future possible isolation of rickettsiae; the second half was frozen in liquid nitrogen, and crushed into powder by using Kontes pestles (Kimble-Kontes, Vineland, NJ). The powder was then resuspended in Nuclei Lysis Solution (Promega, Madison, WI) supplemented with EDTA and proteinase K (QIAGEN, Valencia, CA) and incubated overnight at 56°C. DNA was extracted using a Wizard SV 96 Genomic DNA Purification System (Promega) in conjunction with a Biomek 2000 Laboratory Automation Workstation (Biomek, Fullerton, CA) as described previously (Moriarity et al. 2005). All DNA were eluted with 100 μl of distilled water and stored at 4°C.
Detection of SFG Rickettsiae.
SYBR Green PCR (SQ-PCR) was performed to detect the 547–701-nucleotide (nt) fragment of rompA of the SFG rickettsiae (Eremeeva et al. 2003). The primers used are listed in Table 3. All reactions were conducted in 20 μl by using SYBR Green PCR reagents (Applied Biosystems, Foster City, CA) and set up in 96-well plates by using the Biomek 2000 Laboratory Automation Workstation (Biomek); amplification, data acquisition, and data analysis were carried out with an i-Cycler and iQ Real-time PCR detection system software version 3.1.7050 (Bio-Rad, Hercules, CA). Each assay contained 4 μl of tick DNA, 0.0625 μM final concentration of each forward and reverse primer, 3 mM MgCl2, 1 mM dNTP, and 0.125 U of AmpliGold Taq polymerase (Applied Biosystems). DNA from R. sibirica strain K-1 (246) grown in VERO E6 cells was used as a positive control. The PCR cycling conditions consisted of a primary denaturation step at 95°C for 3 min, followed by 40 cycles of denaturation at 95°C for 20 s, annealing at 57°C for 30 s, and elongation at 65°C for 30 s. The reaction completed with a final elongation step of 72°C for 5 min. Positive samples were detected with threshold cycle (Ct) values of 19.9–37.5 corresponding to 105 to five rickettsial DNA copies per sample tested (Eremeeva et al. 2003). None of the DNA negative control samples generated fluorescence signals. Positive control samples were detected with an average Ct value of 20.5.
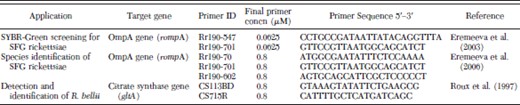
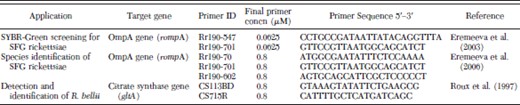
Conventional and Seminested PCR Amplifications.
DNA was PCR amplified with Rr190.70 and Rr190.701 primers for the primary step and Rr190.70 and Rr190.602 for the seminested step as described previously (Rozental et al. 2006). All reactions were performed using a TaqPCR Master Mix kit (QIAGEN) and an Eppendorf master cycler (Eppendorf, Westbury, NY). Two microliters of tick DNA was used for primary PCR reactions, and 1 μl of primary PCR product used for all nested and seminested PCR. Primers were synthesized by the CDC Core Facility, Atlanta, GA, and they were used at a final concentration of 0.8 μM (Table 3). Typical PCR reactions consisted of a primary denaturation step at 95°C for 3 min, followed by 35–40 cycles of denaturation for 20–30 s at 95°C, annealing at 50–57°C for 30 s, and elongation for 1 min at 65–72°C. All reactions were completed with a final elongation for 5 min at 72°C. DNA from R. sibirica strain K-1 (246) grown in VERO E6 cells was used as a positive control.
For the detection and identification of R. bellii, a conventional PCR assay for the citrate synthase gene (gltA) was conducted (Table 3) (Roux et al. 1997). Reactions were performed using a TaqPCR Master Mix kit (QIAGEN), set up in 96-well plates by using the Biomek 2000 Laboratory Automation Workstation (Biomek) and used an Eppendorf master cycler (Eppendorf). Two microliters of tick DNA was used. DNA from R. bellii strain OSU 85–388 grown in VERO E6 cells was used as a positive control.
Conventional and seminested PCR amplicons were detected by electrophoresis in 1.2% agarose gel stained with 0.5 μg/ml ethidium bromide. Amplicon bands of the expected size were excised from the gel and the DNA recovered using Wizard PCR Preps according to the manufacturer’s instructions (Promega). The purified amplicon was sequenced in both directions with the ABI PRISM BigDyeTM Terminator Cycle 3.1 Sequencing kit (Applied Biosystems). Excess of sequencing reagents was removed using a DyeEx 2.0 kit (QIAGEN), and sequenced products were resolved on a 3100 Sequencer (Applied Biosystems). The data were analyzed using Accelrys GCG with SeqLab Interface (Accelrys Software, San Diego, CA). Homologous sequences were detected using the National Center for Biotechnology Information (NCBI) Basic Local Alignment Sequence Tool (BLAST) search engine. Unique sequences generated in this study were submitted to the NCBI GenBank under the following accession numbers: EU109175, EU109176, EU109177, EU109178, EU109179, EU109180, and EU109181. The restriction profile of OmpA gene amplicons was analyzed in silico by using NEBcutter version 2.0 (Vincze et al. 2003).
Statistical Analyses.
Rickettsial prevalence by county, month, gender, and tick species were assessed for significance using Pearson’s chi-square analysis. Fisher exact test was applied to the 2 by 2 contingency table for prevalence by species, in which at least one cell value was less than 5. Because only four ticks were collected in Ventura County, the chi-square test could not be applied to the contingency tables for prevalence by county. For the purpose of analysis, ticks from Ventura County were combined with those from Santa Barbara County because the collection sites in the two counties were near one another, and the date of collection was only 1 d apart. The Wilcoxon rank sum test was used to analyze the significance of the difference in Ct value between ticks positive for R. rhipicephali DNA and those positive for 364D DNA. For all tests, significance was determined at α = 0.05.
Results
PCR Assays on Tick DNAs for SFG Rickettsiae.
Dermacentor occidentalis were collected from all of the 16 sites in five southern California counties (365 total), whereas D. variabilis (10 total) were collected from only three sites in two counties (Tables 1 and 2). Nine of the D. variabilis were collected from Santa Barbara County, and one D. variabilis was collected from Ventura County. The number of ticks collected at each site ranged from 2 to 75. More ticks (34.9%) were collected from Los Angeles County than any of the other counties, whereas only four ticks (1.1%) were collected from Ventura County. Slightly more females than males were collected, representing 54.4% of the total ticks, respectively.
SQ-PCR testing results indicated that 171 ticks (45.6%; n = 375) contained SFG rickettsial DNA for the 547–701-nt fragment of rompA, with Ct values ranging from 19.9 to 37.5. All other tick DNA specimens had Ct values >37.5, and they were considered negative for SFG rickettsial DNA. Fifty-eight (33.9%) of 171 positive ticks had Ct values of 19.9–30, corresponding to ≈102 to <105 rickettsial DNA copy per test sample, and 113 (66.1%) ticks had Ct values of 30.1–37.5 (five to <102 rickettsial DNA copy per test sample). Gel electrophoresis of SQ-PCR products from nine ticks that tested positive by that method but that were negative by seminested PCR, and SQ-PCR products from 10 samples that were only weakly positive by seminested PCR, all detected bands of the expected size (data not shown).
In total, 167 (45.8%) of 365 D. occidentalis and four (40.0%) of 10 D. variabilis tested positive for SFG rickettsia DNA by SQ-PCR (P = 0.7608); among them, 88 were male ticks (51.5% of all males) and 83 were female ticks (40.7% of all females) (Table 1). The difference in prevalence of rickettsial infection between males and females was not significant (P = 0.06). Prevalences did differ significantly by county and month of collection (P < 0.0001 for both). Riverside County (visited in November and December 2006) had the highest prevalence by SQ-PCR (65.4%; n = 104). Santa Barbara County (visited in June 2006) had the lowest prevalence (25.5%; n = 47). By month, 55 (69.6%) of 79 ticks collected in December tested positive by SQ-PCR, whereas only 14 (27.5%) of 51 ticks collected in June tested positive.
Amplification of Rickettsial DNA by Conventional and Seminested PCR.
Conventional and seminested PCR were further used to amplify the larger fragment of rompA that is necessary for species identification of spotted fever group rickettsiae (Regnery et al. 1991, Roux et al. 1996). Of the 171 ticks tested positive by SQ-PCR, 103 (60.2%) were positive after primary PCR targeting 70–701 nt of rompA, and most contained sufficient amount of the amplicon required for sequencing. Tick DNA negative or only weakly positive by conventional PCR was then amplified by seminested PCR for the 70–602-nt fragment of the OmpA gene. In total, 101 ticks were tested using seminested PCR, 95 (94.1%) of which were positive. Altogether, conventional and seminested PCR detected the presence of a rompA fragment in 161 ticks (94.2%) of the 171 ticks that tested positive by SQ-PCR (Table 1).
The presence of R. bellii DNA was detected in two female D. occidentalis (0.6% of all D. occidentalis) by using conventional PCR to amplify the citrate synthase (gltA) gene of R. bellii. Both of these ticks came from Botany Trail in Los Angeles County (Table 2).
Sequence Identification of Rickettsial Species by DNA Sequencing.
Sequence identification of the amplified fragments was obtained for 123 (71.9%) of the 171 tick DNA samples that tested positive by SQ-PCR (Table 2). The 70–602-nt amplicons from the remaining 48 samples were obtained in insufficient amounts to be sequenced successfully. Of these latter 48 tick DNA specimens, three (6.3%) had Ct values of 30 or less, 12 (26.1%) had Ct values between 30.1 and 35, and 33 (67.4%) had Ct values 35.1 or higher, suggesting that low DNA copy numbers in the sample or partial DNA degradation may have negatively affected PCR efficiency in obtaining the larger rompA amplicon.
The nucleotide sequence of 532 bp of the 5′-end rompA of fragments amplified from 94 (25.1%) of 375 tick DNA samples had 99% sequence identity to the sequence of the homologous rompA fragment of the R. rhipicephali reference strain 3-7–♀-6 isolated from Rh. sanguineus from Mississippi (U43803), but all amplicons (four from D. variabilis and 90 from D. occidentalis) had one or more single-nucleotide polymorphisms (SNPs) (genotypes C-F) that differed from the 3-7–♀-6 sequence genotype A (Fig. 1), and each lacked the additional RsaI site described in genotype B by Gage et al. (1994). The R. rhipicephali sequences found in 84 of 94 D. occidentalis and four of four D. variabilis (genotype C; EU109177) differed from the reference strain (genotype A) at nt 14C>T, 288T>C, and 393T>C (Fig. 1). Genotype D (EU109175) had only one point mutation 393T>C, detected in two D. occidentalis. Genotype E (EU109176) had two point mutations, 288T>C and 393T>C, detected in three D. occidentalis. Genotype F (EU109178) was found in one D. occidentalis; this genotype differed from the reference sequence of R. rhipicephali in four SNPs found at the following positions: 14C>T, 70C>T, 288T>C, and 393T>C. R. rhipicephali DNA was detected in ticks collected from every county, although it was not detected at three sites where four ticks or fewer were collected. DNA of 364D was not detected in ticks from Santa Barbara or Ventura counties or from the two sites each in Orange and Riverside counties where fewer than 12 ticks were collected.

Differences between the reference rompA sequence of R. rhipicephali and the sample sequences obtained from Dermacentor ticks. Do, D. occidentalis; Dv, D. variabilis, Genotype A: rompA fragment of the R. rhipicephali reference strain 3-7-♀-6 (U43803) from Rh. sanguineus. The rompA fragment of R. rhipicephali 3-7-♀-6 was resequenced during this study and a sequence identical to that of U43803 was obtained. CA142 and CA751 from California have the same RsaI 70-602 rompA restriction pattern but sequences are unknown. Genotype B: 666332A and CA871 isolates from Oregon and California, respectively (Gage et al. 1994). The RsaI phenotype of the 70-602 rompA fragment differs from genotypes A and C-F but sequences are presently not available. Genotype C (EU109177; 88 samples): Do10, Do14, Do21, Do22, Do27, Do33, Do47, Do67, Do75, Do75a, Do78, Do92, Do102, Do103, Do119, Do127, Do128, Do138, Do141, Do147, Do149, Do159, Do161, Do163, Do172, Dv173, Dv174, Dv181, Dv182, Do186, Do190, Do191, Do192, Do201, Do202, Do203, Do205, Do206, Do215, Do223, Do227, Do229, Do234, Do238, Do240, Do241, Do242, Do243, Do247, Do 251, Do253, Do254, Do264, Do265, Do266, Do267, Do271, Do277, Do278, Do288, Do291, Do292, Do301, Do302, Do303, Do309, Do317, Do319, Do322, Do323, Do324, Do326, Do328, Do329, Do330, Do334, Do336, Do340, Do341, Do343, Do348, Do349, Do350, Do352, Do358, Do361, Do365, Do374. Genotype D (EU109175): Do37, Do276. Genotype E (EU109176): Do239, Do279, Do290. Genotype F (EU109178): Do189.
The 532 bp of the 5′-end rompA rickettsial nucleotide sequence found in 28 D. occidentalis (7.7%; EU109181) had 100% sequence identity to the sequence of the homologous rompA fragment of the 364D genotype (Table 2). The rickettsial nucleotide sequence from one male D. occidentalis Do295 (0.3%; EU109179), which was collected from Laguna Beach, Orange County, had 100% sequence identity to the sequence of the homologous rompA fragment of the R. rickettsii reference strain Sheila Smith (85,860-86,351 nt from AADJ01000001).
The nucleotide sequence EU109180 of the 602 bp of the 5′-end gltA found in two ticks had 100% sequence identity to the sequence of the homologous gltA fragment of R. bellii reference strain RML369-C (U59716) but differed by two bases from that of R. bellii OSU85–389 (NZ_AARC01000001, gi:109727823); the two strains were isolated from D. variabilis collected in Arkansas and Ohio, respectively. One of these ticks, Do127, also contained DNA of the most common genotype C detected for R. rhipicephali, suggesting coinfection with R. rhipicephali and R. bellii may occur. None of the other ticks analyzed contained any detectable combination of R. rickettsii, R. rhipicephali, and 364D DNA as mixed sequences were not observed.
Discussion
In California, the only prior prevalence studies of SFG rickettsiae in D. occidentalis and D. variabilis were conducted in 14 counties in the 1970s and used hemolymph testing of ticks and serotyping of isolates (Lane et al. 1981a,b; Philip et al. 1981). The current study provides the first molecular data on the prevalence and species identification of SFG rickettsiae in these ticks in several counties of southern California and corroborates some conclusions of the earlier studies. Our study also reports the first molecular detection of R. rickettsii in D. occidentalis from California. This result raises additional questions regarding the potential role of D. occidentalis in the epidemiology of RMSF in California and elsewhere. For the first time in California, we also identified the presence of R. rhipicephali DNA in D. variabilis, although the agent was previously detected in one D. variabilis from South Carolina (Burgdorfer et al. 1978). Although detection of DNA of a SFG rickettsia cannot establish the presence or origin of viable agent, especially in engorged ticks, it is likely that the viable agent was present in the questing ticks studied here as shown previously by hemolymph testing of similar tick cohorts (Lane et al. 1981a,b; Philip et al. 1981).
The prevalence of DNA of SFG rickettsiae in D. occidentalis and D. variabilis established in this study varied greatly between counties. The overall prevalence ranged from 65.4% for Riverside County to 25.5% for Santa Barbara County. Because ticks were collected during different months but only once at each site, it is unclear whether these apparent differences in prevalence are due to sampling location, seasonality, or both. The counties of Santa Barbara, Ventura, and Los Angeles were visited during April, May, and June, and they had a mean prevalence of infected ticks of 35.7% (range, 21.3–50%); Orange and Riverside counties were only visited during November, December, and January, with a mean prevalence of 54.9% (range, 40.4–63.5%). According to the National Weather Service (2007), the average temperature at the winter collection sites was 13.4°C, and the average at the summer sites was 17.7°C. Consequently, the relatively small difference in temperatures in these southern California counties during the sampling months suggests that the variations in prevalence may be affected more by microhabitats or other unknown factors than by the temperature alone. Standardized collections of these ticks need to be completed and compared with assess true differences in SFG prevalence between sites. However, similar variations in the prevalence of tick infection between counties were also observed in other studies in California (Lane et al. 1981b, Lane et al. 1981a, Philip et al. 1981).
Previous SFG rickettsia studies conducted in California used isolation of rickettsiae from D. occidentalis, D. variabilis, Ixodes pacificus (Cooley & Kohls), and H. leporispalustris ticks and serotyping to identify individual SFG rickettsiae (Lane et al. 1981a,b; Philip et al. 1981). Isolates of SFG rickettsiae identified as R. rhipicephali were found most frequently and in ≈2–22% of D. occidentalis studied. Rickettsiae identified as 364D (range, 0.34–1.8%) from D. occidentalis and R. bellii (range, 0.23–0.46%) from D. occidentalis and D. variabilis were less common (Lane et al. 1981a, Philip et al. 1981). We observed that Dermacentor from which R. rhipicephali DNA was detected had a slightly lower average Ct value than those from which 364D DNA was detected (30.3 and 32.4, respectively; P < 0.05). This corresponded to lower rickettsial DNA copy numbers in ticks infected with 364D, which may indicate that hemolymph test used in previous studies is likely less efficient at detecting 364D infection than R. rhipicephali infection. It may explain some of the differences in infection prevalence between our study and previous work. One of the previous studies in California also reported two D. occidentalis coinfected with R. rhipicephali and R. bellii (Lane et al. 1981a). No R. rickettsii were detected in any of the 6500 ticks tested by hemolymph assay, even in areas with known RMSF cases (Lane et al. 1981a,b; Philip et al. 1981).
Overall, the prevalences of spotted fever rickettsiae in ticks found in our study were much higher than those reported previously. This is most likely due to the higher sensitivity of SQ-PCR compared with hemolymph testing for detection of rickettsiae in ticks. SQ-PCR can detect as few as five copies of a cloned plasmid in stock cultures and works well to detect DNA of SFG rickettsiae in clinical and field samples (Eremeeva et al. 2003). We have demonstrated the value of SQ-PCR in routine screening of large numbers of ticks for SFG rickettsiae. Gel electrophoresis of SQ-PCR product from 10 ticks that tested positive by that method but negative by seminested PCR showed that product of the expected molecular size was being amplified. Eight of the 10 ticks had high Ct values ≥35, corresponding to very low copy numbers of rickettsial DNA in the specimens tested. This may explain the difficulty in amplifying by conventional PCR and sequencing rickettsial DNA from these ticks and confirmed that SQ-PCR is more sensitive in detecting rickettsial DNA than conventional and seminested PCR of OmpA gene. SQ-PCR also requires less time than conventional PCR methods and no gel electrophoresis for detection of product; therefore, large numbers of samples can be analyzed with fully automated equipment. Automated methods based on work by Moriarity et al. (2005) for DNA extraction, SQ-PCR, and conventional PCR were successfully applied in this study.
Our study provides the first molecular data on the prevalence and species identification of SFG rickettsiae in D. occidentalis and D. variabilis collected in southern California. We sequenced 123 specimens of the 532-bp fragment of rompA of SFG rickettsiae from these two tick species. Ninety-four (76.4%) of 123 were identified as R. rhipicephali. This represents an overall prevalence of 25.1% in the 375 ticks tested. Four new genotypes of R. rhipicephali were identified; each had predicted RsaI restriction profiles of the homologous rompA fragment identical to those of R. rhipicephali isolate 3-7–♀-6 and D. occidentalis isolate 666332A from Oregon (Fig. 1, genotype A), which differed from the restriction profiles CA871, CA142, and CA751 isolates from California D. occidentalis (genotype B) described previously (Gage et al. 1994). However, the genotypes C–F identified in our study are distinct in sequence from the reference R. rhipicephali strain 3-7–♀-6 from Rh. sanguineus, which we sequenced again for this study, as they contained one to four SNPs that did not affect their RsaI or PstI profiles from those of genotype A (Fig. 1). Although none of these SNPs led to the insertion of a stop codon in the sequence of rompA, one SNP (14C>T) led to a change in the amino acid sequence, indicating that possible minor rOmpA antigenic variation exists among isolates of R. rhipicephali. Differences in plaque type among isolates of R. rhipicephali with genotypes A and B have been noted previously (Philip et al. 1981, Gage et al. 1994), but it is not known whether genotypes C–F also differ in biological phenotypes. Previous studies have indicated that D. variabilis artificially infected with R. rhipicephali can transovarially transmit the rickettsiae to the F1 generation, but rickettsial infection could not be maintained into the F2 or further generations (Macaluso et al. 2001, 2002). Niebylski et al. (1999) also demonstrated that D. andersoni from Montana "naturally" infected with R. rhipicephali exhibited significantly lower fecundity, although this result was not replicated with artificially infected D. variabilis (Macaluso et al. 2001). However, the specific tick origin and genotypic characteristics of the R. rhipicephali strains they used were not stated in these articles. Consequently, whether differences in the tick origin of isolates of R. rhipicephali or their genotypes may affect their maintenance and stability in different tick species requires further investigation. We have obtained several isolates of 364D and R. rhipicephali from the ticks studied here; their biological, genetic, and antigenic characterization will be described elsewhere.
Twenty-eight amplicons contained nucleotide sequences identical to that reported previously for the 364D isolate, representing 7.5% of all ticks collected. Similar to previous studies, 364D SFG rickettsiae were only found in D. occidentalis, although the prevalence found in this study is higher than the prevalences reported in previous studies of D. occidentalis in California (range, 0.4–5.3%) (Lane et al. 1981a,b; Philip et al. 1981).
DNA of R. bellii and R. rickettsii were rarely detected in the current study, with overall prevalences of 0.5 and 0.3%, respectively. Our figure for R. bellii in D. occidentalis was slightly less than that reported by Lane et al. (1981a,b) (range, 1.3–1.4%), although Philip et al. (1981) detected no R. bellii in any of 800 D. occidentalis collected from California.
The prevalence of R. rickettsii detected in D. occidentalis in this study (0.3%) was similar to the prevalence of R. rickettsii detected in D. variabilis in Ohio counties with reported RMSF cases (range, 0.1–0.6%) and to the prevalence of R. rickettsii found in A. aureolatum from an area of Brazil endemic for Brazilian spotted fever (0.89%; n = 669) (Pretzman et al. 1990, Pinter and Labruna 2006). However, it is slightly lower than those found in Rh. sanguineus from California and Arizona (1.6 and 2.9%, respectively) and in A. cajennense from another endemic area of Brazil (minimal prevalence, 1.3%) (Demma et al. 2005, Guedes et al. 2005, Wikswo et al. 2007). Although the prevalence of R. rickettsii in D. occidentalis determined in our study is quite low compared with 364D and R. rhipicephali, it may still be enough to account for human RMSF cases in areas of California where R. rickettsii-infected D. variabilis or Rh. sanguineus are absent or not abundant.
Because the evidence for R. rickettsii was only found in one D. occidentalis and one Rh. sanguineus in both the current and previous studies, respectively (Lane et al. 1981a,b; Philip et al. 1981, Wikswo et al. 2007), it remains unclear whether this represents an incidental spillover of R. rickettsii from its primary vector-reservoir interaction or an important part of the natural maintenance cycle of the human pathogen. Similarly, whether the high prevalence of R. rhipicephali and 364D in D. occidentalis and R. rhipicephali in D. variabilis detected in California may possibly interfere with the acquisition of R. rickettsii by these ticks as has been proposed for R. peacockii in D. andersoni (Burgdorfer et al. 1981, Niebylski et al. 1997) needs to be further evaluated. It seems possible that either 364D or another agent may cause human rickettsioses in areas where D. andersoni is absent. It was speculated that 364D may be responsible for some cases of RMSF-like infection based on indirect serological evidence, although it has never been proven to cause human disease (Lane et al. 1981a). Additional molecular characterization and/or isolation of rickettsiae from humans and animals suffering from RMSF-like infections in California are needed to elucidate the true cause(s) of these SFG rickettsioses.
Acknowledgements
We thank John Krebs for assistance in obtaining mortality and morbidity data for RMSF from California and Richard Davis and Megan Henry for assistance in the field. This research was conducted in partial fulfillment of the requirements for an MPH from the Rollins School of Public Health of Emory University (to M.W.).
The findings and conclusions are those of the authors and do not necessarily represent the views of the Centers for Disease Control and Prevention.