-
PDF
- Split View
-
Views
-
Cite
Cite
Marie-Michèle Labrecque, Marie-Ève Allard, Andréa Murru, Guillaume Paré, Jason P Acker, Sylvie Lesage, Mélissa Girard, Maria J Fernandes, Prolonging neutrophil room-temperature storage with clinically approved solutions: implications for granulocyte transfusion, Journal of Leukocyte Biology, Volume 117, Issue 4, April 2025, qiae258, https://doi.org/10.1093/jleuko/qiae258
- Share Icon Share
Abstract
Granulocyte concentrates (GCs) are leukocyte preparations enriched in neutrophils that can potentially save neutropenic patients from life-threatening, antimicrobial-resistant infections. The main challenge of GC transfusions is preserving the viability and antimicrobial activity of neutrophils beyond 24 h to reduce the logistical burden on collection centers and increase the availability of this cell therapy. Thus, the aim of this study was to explore extending the ex vivo viability and antimicrobial activity of GC neutrophils up to 72 h with a unique combination of the clinically approved additives Plasma-Lyte (PL), SAGM, AS-3, and Alburex. Neutrophils isolated from healthy donors were resuspended in autologous plasma at the same concentration as in GCs, diluted with various combinations of PL, SAGM, AS-3, and/or Alburex with or without the addition of buffers, and stored at room temperature for up to 72 h. During storage, neutrophil viability, phagocytosis, and intracellular reactive oxygen species production were measured by flow cytometry. Extracellular reactive oxygen species production was measured by spectrophotometry and chemotaxis by the number of calcein-stained neutrophils that migrated toward the chemotactic peptide, N-formyl-Met-Leu-Phe (fMLF). The same assays were performed on pooled, residual leukocyte units generated by the Reveos system, after storage in the additive combination that most effectively preserved the viability and function of isolated neutrophils. The additive combination that best performed in the majority of the assays contained PL, buffers, and AS-3. Neutrophil viability was preserved for a maximum of 48 h and phagocytosis of opsonized bacteria and reactive oxygen species production up to 72 h of storage at room temperature. In contrast, fMLF-induced chemotaxis decreased by 20% after 24-h storage while extracellular reactive oxygen species production increased significantly within the same time period. Supplementing GCs prepared from pooled, residual leukocyte units with this storage solution after the standard 16- to 24-h processing period as per the blood center guidelines, did not significantly improve the preservation of neutrophil viability and function. Our findings provide proof of concept that mixtures of clinically approved additives can be tailored to significantly prolong the viability and function of freshly isolated neutrophils during room-temperature storage. The unique additive composition of this storage solution that we developed for freshly isolated neutrophils requires further optimization for use with pooled, residual leukocyte units as well as the timepoint at which the solution is added during processing to prolong the viability and functions of neutrophils in this blood product.
1. Introduction
Neutrophils are one of the first leukocytes recruited to inflamed tissues to eliminate pathogens with their diverse repertoire of defense mechanisms.1–3 Migrating neutrophils are activated when nonopsonized pathogens interact with pathogen recognition receptors, and/or opsonized pathogens interact with complement and Fc receptors.4,5 Phagocytosed pathogens are sequestered in the phagolysosome for eradication by antimicrobial peptides, proteases, a low pH, and reactive oxygen species (ROS).6–9 Neutrophils also release extracellular traps (NETs) mainly composed of DNA and antimicrobial proteins that bind and sequester pathogens.1,10 The absence of neutrophils is thus incompatible with life.
Neutropenic patients with a very low neutrophil count (>0.5 × 109/L) are susceptible to life-threatening infections.11,12 Those neutropenic patients with infections that are resistant to conventional, antimicrobial drugs can be transfused with large numbers of neutrophils in the form of a granulocyte concentrate (GC). GCs provide temporary, innate immune defenses to fight infection. According to GC guidelines, a minimum absolute neutrophil count (ANC) of 1010 neutrophils is required per GC transfusion unit.13,14 The ANC of a GC donation derived from apheresis of a healthy donor is <1010 neutrophils. Thus, GCs are obtained by apheresis of healthy donors stimulated with pharmacological agents (G-CSF and/or steroids) to increase neutrophil numbers in circulation.12 Alternatively, the minimal ANC for GCs can be achieved by pooling 10-20 ABO-matched buffy coats derived from whole blood of nonstimulated, healthy donors.15–18 GCs have a maximum storage time of 24 h as neutrophils have a short lifespan ex vivo.19,20 Several reports have thus attempted to prolong neutrophil viability in GCs to ease the pressure on donor recruitment at short notice and GC distribution logistics.20–23
Preserving neutrophil viability in GCs is challenging due to the cellular composition of this blood product. GCs are a heterogeneous suspension of leukocytes composed mostly of neutrophils, but also of red blood cells and platelets. The high cell concentration in GCs is one of the main causes of the rapid decline in nutrient supply such as glucose and a drop in pH leading to premature cell death.20,21,24–26 Klinkmann et al.27 reported that depleting GCs of residual red blood cells and platelets through various centrifugation steps significantly diminishes lactate production during storage and maintains a more stable pH preserving neutrophil viability, phagocytosis, and oxidative burst activity for up to 72 h. The additional handling required in this protocol approach, however, significantly reduces the recovery rate of neutrophils to 57%.15,16,18,27 Other attempts to prolong the ex vivo viability of neutrophils include decreasing the cell concentration in GCs and stabilizing the pH for up to 48 h by diluting GCs 8-fold with autologous plasma (AP) or infusible solutions, such as Plasma-Lyte (PL) or T-Sol.19 While neutrophil phagocytosis and oxidative burst were maintained under these experimental conditions for up to 72 h, the final volume of the diluted GC of 1 L is above the allowed transfusable volume of 0.5 L or less.20
The addition of apoptosis inhibitors to neutrophils ex vivo effectively prolongs their survival.22,23,28 The apoptosis inhibitor, Q-VD-OPh, preserves GC neutrophil viability, chemotaxis, and NETosis for up to 72 h.29 Fan et al.23 developed a storage solution composed of a combination of cell death pathway inhibitors that preserves GCs neutrophil viability, phagocytosis, microbial killing, and chemotaxis ex vivo for 3 to 5 d. Despite the significant increase in GC neutrophil lifespan by apoptosis inhibitors, the risks associated with their use for human transfusion remain unknown.
The aim of this study was to develop a clinically suitable storage solution for GCs that significantly prolongs their shelf-life by extending neutrophil ex vivo viability and function at room temperature beyond 24 h. To accelerate the translation of our findings to the clinic, we tailored a unique mixture of clinically approved additives for optimal neutrophil ex vivo survival. Two categories of additives that have not previously been tested on neutrophils were used. The first included additive solutions for red blood cells, such as SAGM and AS-3 due to their high glucose content.30 The second comprised a plasma substitute, Alburex, as it is the most effective plasma-like diluent for GCs.20,21 We also investigated the effects of PL supplemented with histidine and HEPES buffer that was reported to prolong neutrophil viability, but whose effect on neutrophil function remains unknown.19 Our findings provide proof of concept that the viability and function of freshly isolated neutrophils can be prolonged ex vivo at room temperature with clinically approved additives under experimental conditions that mimic the cell concentration and transfusion volume limits of GCs.
2. Materials and methods
2.1 Study design
We developed a storage solution comprised of a unique combination of additives using a stepwise approach (Fig. 1). We began by identifying the combinations of additives that significantly extended neutrophil viability. Those additive mixtures that prolonged neutrophil viability were then tested for their ability to also preserve neutrophil function including phagocytosis and ROS production for up to 72 h. Only those additives that most effectively maintained neutrophil viability and function were then tested for their ability to preserve neutrophil chemotactic activity. These tests were initially performed on freshly isolated neutrophils from whole blood donations of healthy donors to avoid the logistical burden of recruiting at least 5 healthy donors to prepare a single GC derived from pooled residual leukocyte units (PGC, pooled granulocyte concentrate) for each experiment. These residual leukocyte units are generated by routine whole blood processing with the Reveos system and are enriched in neutrophils.31 They closely resemble GCs prepared by pooling buffy coats from healthy donors.15–18 The protocol for the pooling of PGCs in this study was designed to closely mimic the pooling of buffy coats that are regularly used for transfusion in some blood centers. The average leukocyte concentration in PGCs of 10 residual pooled leukocyte units 80 × 106 mL−1 was determined with a cell counter (Ac•T 5diff hematology analyzer, Beckman Coulter) (result not shown). Thus, freshly isolated neutrophils were resuspended at a concentration of 80 × 106 mL−1 in AP and diluted with a volume of additive solutions that corresponds proportionally, to the maximal allowable volume that could be added to a GC bag (400 mL). To fulfill these criteria, the original neutrophil preparation resuspended in AP was supplemented with an additive mixture that diluted the neutrophil preparation by 1.48-fold. As a negative control, we resuspended neutrophils only in AP.
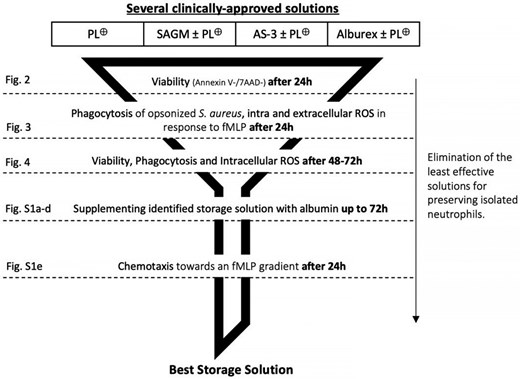
Experimental design. A funnel design was used to reduce the number of storage conditions to test as the experiments progressed. Briefly, the viability of neutrophils stored in different combinations of additives was first determined after 24 h of room temperature of storage. The additive combination(s) that was most effective at preserving neutrophil viability was then tested for its ability to preserve phagocytosis and ROS production. The same strategy was used for the subsequent stages of the experimental design until we identified the combination of additives that preserved the ability of stored neutrophils to perform chemotaxis. *PL⊕ = Plasma-Lyte + 25 mM HEPES + 3 mM histidine buffer.
The optimal storage solution developed with freshly isolated neutrophils from whole blood was then tested for its ability to prolong neutrophil viability and function in PGCs (see PGC preparation below). The pH and glucose of PGCs were also measured as they are known to affect neutrophil viability and function. This type of GC was chosen over leukapheresis donation as it is becoming the GC of choice due to logistical reasons.
2.2 Neutrophil isolation and their supplementation with room-temperature storage solutions
Neutrophils were isolated from whole blood of healthy donors who provided written informed consent to use their blood samples for this study that was performed according to the Declaration of Helsinki for studies with human subjects. This study was approved by the CHU de Québec-Université Laval Research Ethics Committee (# 2019-4493) and the Héma-Québec Research Ethics Committee (# 2018-012).
Neutrophil isolation was performed at room temperature under sterile conditions as described in Fernandes et al.32 Cells were resuspended at a concentration of 80 × 106 mL−1 in AP prior to storage at room temperature in a 96-well plate. Briefly, blood was collected in acid-citrate-dextrose (ACD) tubes (BD Vacutainer). After sedimentation of erythrocytes in 2% dextran, neutrophils were purified by centrifugation at 600 × g for 20 min in lymphocyte separation medium (Wisent Bioproducts) under aseptic conditions. Contaminating erythrocytes were removed by hypotonic lysis, and cells were resuspended in Mg2+-free HBSS (Hanks’ balanced salt solution) containing 1.6 mM CaCl2. The ability of clinically approved additives to improve neutrophil room-temperature storage by prolonging neutrophil viability and/or function was tested by diluting and supplementing the AP neutrophil suspension with different combinations of additives (Supplementary Table 1). In all cases, the addition of additives diluted the AP neutrophil suspension by 1.48-fold, a dilution factor that respects the maximal transfusion and storage bag volume of GCs. The additive combinations tested include: PL A, pH 7.4 (Baxter Healthcare Corporation) with buffers (PL⊕); SAGM (Terumo BCT); with or without PL⊕; AS-3 (Haemonetics Corporation) with or without PL⊕; Alburex® 5% and 25% (CSL Behring) with or without PL⊕; and AS-3 + Alburex + PL⊕. The buffers used include a histidine buffer (bioWORLD) at a final concentration of 3 mM and HEPES (Wisent Bioproducts) at a final concentration of 25 mM. All components and volume proportions specific to each solution are detailed in the Supplementary Material (Supplementary Table 2). Neutrophil viability and functions were measured on freshly isolated neutrophils prior to storage and 24, 48, and 72 h after storage as described below.
2.3 Preparation of GCs from pooled residual leukocyte units and their supplementation with a room-temperature storage solution
Only the preservation solution that significantly improved neutrophil viability and function was tested on PGCs. Briefly, whole blood units obtained from ABO-matched, healthy donors were stored for 16 to 24 h at room temperature prior to automated processing on the Reveos® system (Terumo BCT) with the 3C-2 program, as per Héma-Québec's standard protocol for whole blood processing. This program separates plasma, platelets, red blood cells, and residual leukocytes of each whole blood unit into different bags. Five residual leukocyte units, each with a volume of ∼12 mL, were pooled and diluted with 75 mL of male plasma in a platelet pooling bag (41910, Terumo BCT) to generate 1 PGC (timepoint: t = 16 to 24 h). As is current practice in GC processing, each PGC was irradiated with 25 Gy prior to experimental analysis.26
GC composition was determined at the Héma-Québec collection center with a cell counter (Ac•T 5diff hematology analyzer, Beckman Coulter) and a blood gas analyzer (ABL90, Radiometer). PGCs were then supplemented with PL and AS-3 with or without HEPES (0, 25 mM or 75 mM) with the same dilution factor used for freshly isolated neutrophils. The experimental conditions were as follows: PGCs were diluted with the additive solution mixture immediately after the whole blood unit storage period (16 to 24 h post-blood donation) and then stored for an additional 24 and 48 h at room temperature (40 to 48 and 64 to 72 h post-processing, respectively). A nondiluted aliquot was used as a negative control. The whole blood unit donors also provided consent to donate on the day of blood collection for PGCs, a separate blood sample of 8 mL in ACD tubes (BD Vacutainer). These samples provided baseline metrics for neutrophil viability and function for each donor (timepoint: t = 0). The viability and functional assays described above were performed on these baseline samples as well as on PGCs at various times post-blood donation (16 to 24, 40 to 48, and 64 to 72 h). Prior to the assays, PGCs were subject to erythrocyte lysis. Since neutrophils were not isolated from PGCs, we assessed neutrophil function with the same flow cytometry-based assays used for freshly isolated neutrophils and gated on the PGC neutrophil population (SSC-FSC). We also gated for monocytes as, like neutrophils, these cells play a key role in pathogen eradication during infection and are also present in PGCs.
2.4 Viability assay
Neutrophils or erythrocyte-lysed PGCs were stained with Annexin V (Becton Dickinson) and 7-AAD (7-aminoactinomycin D, from Thermo Fisher) in Ca2+-free HBSS supplemented with 0.5% BSA at room temperature in the dark for 15 min prior to flow cytometry as described in Murru et al.26 The proportion of apoptotic and necrotic cells was determined with a FACSCanto II flow cytometer (Becton Dickinson).
2.5 Phagocytosis assay
Opsonized phagocytosis was quantified by incubating neutrophils or erythrocyte-lysed PGCs with 15 µg of pHRodo Red S. aureus Bioparticles Conjugate (Molecular Probes, Life technologies) opsonized with human IgG at a ratio of 1 leukocyte: 5 bacteria, as described in Murru et al.26 Briefly, cells were centrifuged at 2,000 rpm for 15 s to synchronize phagocytosis prior to a 30-min incubation at 37 or 4 °C for comparative purposes. Phagocytosis was stopped by adding cold HBSS. Cells were kept on ice until analysis with a BD LSRII flow cytometer to determine the acidification of the phagosomal compartment with the MFI. A LSRII flow cytometer (Becton Dickinson) was used to measure the mean fluorescence intensity (MFI) emitted by pHRodo during phagocytosis, a measure of the acidification of the phagosomal compartment.
2.6 Superoxide production assay
The N-formylmethionyl-leucyl-phenylalanine (fMLF)-induced production of superoxide was determined with a cytochrome c reduction assay (Sigma-Aldrich). Neutrophils (107 cells/ml) were incubated with either 10−7 M fMLF or vehicle (DMSO) for 10 min at 37 °C in the presence of 125 μM cytochrome c. The difference between the optical density readings (550-540 nm) made with a spectrophotometer was multiplied by the extinction coefficient of cytochrome c to determine the amount of O2 − produced (nmol O2 −/106 cells) as described in Paré et al.33
2.7 Intracellular ROS assay
Neutrophils or erythrocyte-lysed PGCs were incubated for 15 min at 37 °C with 1 μM CM-H2DCFDA (Sigma-Aldrich) as described in Murru et al.26 Cells were then stimulated with 5 µM fMLF or incubated in vehicle (DMSO) prior to staining with the viability marker 7-AAD (Thermo Fisher) and flow cytometry analysis with a FACSCanto II flow cytometer. The ratio of intracellular ROS was then calculated by dividing the median fluorescence of stimulated cells by the control cells.
2.8 Chemotaxis assay
Neutrophils were stained with calcein AM (Thermo Fisher) and allowed to migrate toward different concentrations of fMLF for 1 h at 37 °C in a 96-well ChemoTX system (101–8; Neuroprobe) as described in Murru et al.26 Neutrophils were resuspended (5 × 106 cells/ml) in HBSS with 10% FBS and preincubated with 5 μg/ml of calcein AM for 30 min at 37 °C. The cells were then washed and resuspended at 3 × 106 cells/ml in HBSS/FBS with the indicated concentrations of drugs prior to migration toward different concentrations of fMLF for 1 h at 37 °C in a 96-well ChemoTX system (Neuroprobe). The fluorescence of cells in the filters was measured using a microplate fluorescence reader (FL600; Bio-Tek Instruments, Winooski, VT, USA; excitation wavelength 485 nm and emission wavelength 530 nm). Fluorescence was converted to numbers of neutrophils based on a standard curve generated by seeding known numbers of neutrophils in the bottom of the chamber. The results are expressed as percentage of migrated cells, calculated as the fluorescence of migrated neutrophils/fluorescence of 20,000 neutrophils/ml × 100, obtained from the standard curve.
2.9 Statistical analysis
All statistical nonparametric analysis was performed using the Prism GraphPad software. Neutrophil viability and function after room-temperature storage in the indicated solutions for the indicated times were compared to freshly isolated neutrophils (0 h) and to the untreated group using a one-way ANOVA with Kruskal–Wallis multiple comparison test. Stored PGCs were compared to controls using the same statistical test: cells right after collection (0 h) and after the 16- to 24-h storage of whole blood units prior to processing using the same tests. When pairing was possible, a one-way ANOVA with Friedman test multiple comparison was used. A P-value < 0.05 was considered statistically significant.
3. Results
3.1 Identification of additive solutions that best preserve neutrophil viability and antimicrobial activity for 24 h at room-temperature storage
To identify the combination of additives that significantly prolong neutrophil viability during room-temperature storage, we used the study design in Fig. 1. Freshly isolated neutrophils were resuspended in AP and stored for 24 h in the presence or absence of the indicated additives. Staining of neutrophils with Annexin V and 7-AAD revealed a significant decrease in the viability of neutrophils stored in AP alone after 24 h compared with freshly isolated neutrophils (Fig. 2, 60% compared with 90% viable neutrophils). Supplementing AP with PL buffered solution or the red blood cell additive AS-3 maintained a mean neutrophil viability above 78%. Supplementing the AP resuspended neutrophils with SAGM or the commercial albumin solution, Alburex®, decreased neutrophil viability significantly compared with freshly isolated of neutrophils (Fig. 2, 51% and 40%, respectively, compared with 90% viable neutrophils). The addition of PL⊕ to the SAGM or Alburex storage solution countered the negative effect of these solutions on neutrophil survival. Together, these observations indicate that neutrophil viability is preserved for 24 h at room temperature when neutrophils are stored in AP supplemented with AS-3, PL⊕, or PL⊕ in combination with any of the above additives.
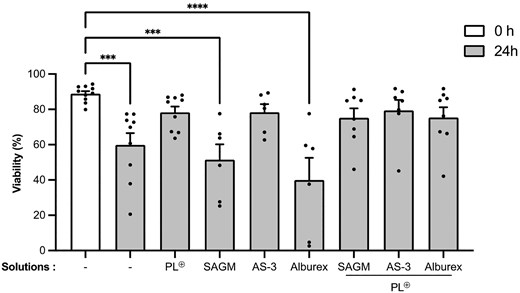
Effect of additives on neutrophil viability after 24 h of room-temperature storage. Freshly isolated neutrophils from healthy donors were resuspended in AP (white bar) at a concentration of 80 × 106 mL−1 in the presence or absence of the indicated additives prior to storage for 24 h at room temperature (gray). Viability was determined by staining with 7-AAD and Annexin V prior to flow cytometry, and expressed as percent neutrophils negative for 7-AAD and Annexin V staining (−/−). Data are presented as means ± SEM of up to 8 independent experiments (N ≥ 6). ***P < 0.001 and ****P < 0.0001 compared with the freshly isolated neutrophils group (0 h).
Having identified additive combinations that preserve neutrophil viability at room temperature for 24 h, we tested these storage solutions for their ability to preserve phagocytosis as well as intracellular and extracellular ROS production. Phagocytosis of opsonized pHRodo Red S. aureus by neutrophils stored in AP alone for 24 h at room temperature was reduced compared with freshly isolated neutrophils, but did not reach significance (Fig. 3A). Neutrophils resuspended in AP and diluted with PL⊕, PL⊕ + SAGM, or PL⊕ + Alburex maintained their ability to phagocytose. Adding AS-3 or PL⊕ + AS-3 to AP significantly enhanced the phagocytic capacity of neutrophils.
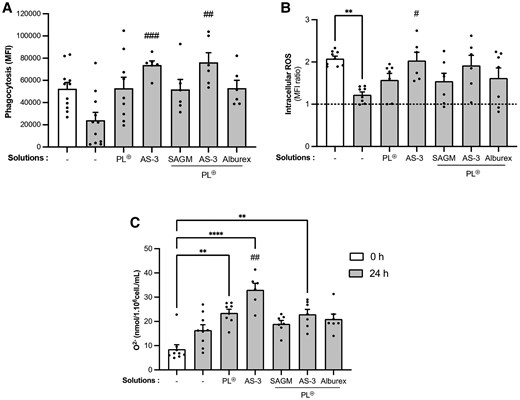
Impact of additives on neutrophil effector functions after 24 h of room-temperature storage. Freshly isolated neutrophils from healthy donors (white) were resuspended in AP at a concentration of 80 × 106 mL−1, diluted with the indicated additives as in Fig. 2, and stored for 24 h (gray). A) Phagocytosis of opsonized pHRodo Red S. aureus-conjugated bioparticles was determined by incubating neutrophils at a concentration of 107 cells/mL with 15 μg pHRodo bacterial particles for 30 min at 37 °C prior to flow cytometry. Data are expressed as MFI. B) Intracellular ROS production induced by 5 μM fMLF was determined by flow cytometry after incubation with 1 μM CM-H2DCFDA. Data are expressed as a ratio between ROS produced by stimulated neutrophils and the DMSO control (fMLF/DMSO). The dotted line represents the absence of ROS production (ratio = 1). C) Extracellular ROS production was measured by the reduction of 125 μM cytochrome c in a spectrofluorometer by neutrophils stimulated with 1 μM fMLF. Data are expressed as a subtraction of superoxide produced by activated neutrophils from that produced by neutrophils incubated in DMSO (fMLF–DMSO). Data are presented as means ± SEM of 6 experiments (n ≥ 6). **P < 0.01 and ****P < 0.0001 compared with freshly isolated neutrophil group (0 h); #P < 0.05, ##P < 0.01, and ###P < 0.001 compared with untreated neutrophils (AP) stored for 24 h.
The fMLF-induced production of intracellular ROS by neutrophils after 24 h of storage in AP alone significantly decreased compared with freshly isolated neutrophils (Fig. 3B, P < 0.01). As for phagocytosis, the addition of PL⊕, PL⊕ + SAGM or PL⊕ + Alburex to AP preserved the ability to produce intracellular ROS for 24 h. The PL⊕ + AS-3 solution also preserved ROS production. In contrast, neutrophils stored in AP-supplemented with AS-3 alone produced significantly more ROS compared with AP alone (PL⊕ + AS-3; P = 0.0754).
Extracellular superoxide (O2−) production is less stable during storage than phagocytosis or intracellular ROS formation. Neutrophils stored for 24 h in AP produced higher levels of fMLF-induced ROS than freshly isolated neutrophils, but this increased response did not reach significance. A significant increase in fMLF-induced ROS production was observed in neutrophils after 24-h storage in AP and diluted with PL⊕, AS-3, or PL⊕ + AS-3. The addition of PL⊕ + SAGM or PL⊕ + Alburex did not significantly alter ROS production after 24-h storage compared with freshly isolated neutrophils (Fig. 3C). Since excessive extracellular ROS production can be harmful to the host,34 we did not use PL⊕ and AS-3 alone in the remaining experiments.
Together, the above observations indicate that a storage buffer with AP supplemented with a combination of PL⊕ + SAGM, PL⊕ + AS-3, or PL⊕ + Alburex preserves the ability of neutrophils to phagocytose S. aureus and produce intracellular ROS in response to fMLF. The fMLF-induced extracellular ROS production was the only function that increased in all solutions tested.
3.2 Determination of combination of additives that preserve neutrophil viability and function for up to 72 h of storage at ambient temperature
To determine how long neutrophils stored in PL⊕ supplemented with AS-3, SAGM, or Alburex can be stored at room temperature without a significant loss of viability and function, we performed room-temperature storage experiments up to 72 h. As shown in Fig. 4A, the viability of neutrophils stored in AP significantly decreases to 33% and 21% after 48 and 72 h of storage, respectively. In contrast, the viability of neutrophils stored in the PL⊕ + AS-3 solution for 48 h of storage was 58% compared with 44% after 72 h of storage. Neutrophils stored in PL⊕ + SAGM and PL⊕ + Alburex solutions underwent a greater loss of viability after 72 h (38% and 36% viability, respectively).
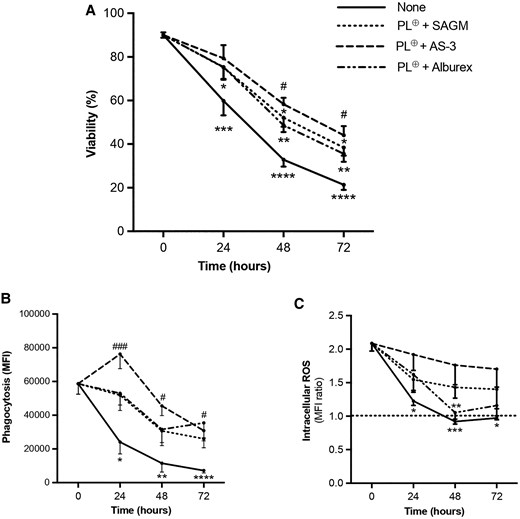
Preservation of neutrophil viability and effector functions after 72-h storage in AP supplemented with PL⊕, and SAGM, AS-3, or Alburex. Freshly isolated neutrophils (0 h) were resuspended in AP at a concentration of 80 × 106 mL−1 and diluted with PL⊕-and the indicated additives prior to storage for 72 h. A) Viability was determined by flow cytometry after 7-AAD and Annexin V staining as in Fig. 2. Viability is expressed as percent neutrophils negative for 7-AAD and Annexin V staining (−/−). B) Phagocytosis of opsonized pHRodo Red S. aureus-conjugated bioparticles was determined by flow cytometry as in Fig. 3. Data are expressed as MFI. C) Intracellular ROS production by fMLF-stimulated neutrophils was determined by flow cytometry after incubation with CM-H2DCFDA as in Fig. 3. Data are expressed as a ratio between ROS produced by stimulated neutrophils and the DMSO control (fMLF/DMSO). The dotted line represents the absence of ROS production (ratio = 1). Data are presented as means ± SEM of 4 experiments (n ≥ 6). *P < 0.05, **P < 0.01, ***P < 0.001, and ****P < 0.0001 compared with freshly isolated neutrophil group (0 h); #P < 0.05 and ###P < 0.001 compared with the corresponding untreated neutrophils (AP) stored for 48 and 72 h.
Similar to the effect of long-term storage on neutrophil viability, a significant loss in phagocytosis and ROS production was also observed in neutrophils stored in AP for 72 h. After 48 h of storage in AP, neutrophils lost 80% of their ability to phagocytose opsonized S. aureus (Fig. 4B). In contrast, all PL⊕-supplemented solutions preserved between 44% and 78% of the phagocytic capacity of neutrophils up to 72 h. AP supplemented with PL⊕ + Alburex or PL⊕ + AS-3 was most effective at preserving this neutrophil function for 72 h (P = 0.0657) compared with neutrophils stored in the absence of solutions. As for fMLF-induced ROS production, it was completely lost after 48 h of storage compared with its preservation up to 72 h in neutrophils stored in AP and diluted with PL⊕ + AS-3 and PL⊕ + SAGM (Fig. 4C). The addition of PL⊕ + Alburex to AP did not preserve ROS production as effectively as PL⊕ + AS-3 and PL⊕ + SAGM.
Together, our observations indicate that AP supplemented with PL⊕ + AS-3 solution preserves the highest level of neutrophil viability, phagocytosis, and ROS production for up to 72 h compared with AP alone or AP supplemented with PL⊕ + SAGM or PL⊕ + Alburex.
3.3 Effect of supplementing the optimized storage solution with albumin on the viability and function of neutrophils stored for up to 72 h
To further improve the preservation of neutrophils in the PL⊕ + AS-3 solution, it was supplemented with albumin (4 mg/mL) as it was reported to improve neutrophil storage.25 To avoid altering the concentration of products already in the solution (Supplementary Table 1), a more concentrated version of the Alburex solution (25%) was used. Neutrophil viability, phagocytosis of opsonized bacteria, and intracellular ROS production were preserved up to 72 h in AP supplemented with PL⊕ + AS-3 + Alburex (Supplementary Fig. 1A-C). A significant reduction in viability and function was observed after 72 h of storage compared with freshly isolated neutrophils. As demonstrated for other storage solutions, fMLF-induced superoxide production significantly increased after 24-h storage (Supplementary Fig. 1D). Together, our results indicate that albumin supplementation (PL⊕ + AS-3 + Alburex) does not improve the viability and antimicrobial activity of isolated neutrophils compared with the PL⊕ + AS-3 solution.
The ability of the PL⊕ + AS-3 storage solution to preserve chemotaxis was tested as it was the most effective solution at preserving the viability and other neutrophil functions assayed. The migration of freshly isolated neutrophils toward fMLF was maximal (74%) at a concentration of 3−8 M fMLF (Supplementary Fig. 1E). After 24-h storage in PL⊕ + AS-3, maximum migration decreased (57%, P = 0.0692) compared with freshly isolated cells, but this tendency did not reach significance. While the addition of Alburex did not significantly increase the ability of AP + PL⊕ + AS-3 to preserve neutrophil viability, phagocytosis or ROS production, it rendered stored neutrophils more sensitive to fMLF. Neutrophil migration reached its maximum of 68% at a lower concentration of fMLF (10−8 M) and remained unchanged (67%) at 3−8 M of fMLF after 24 h. Thus, the storage buffer that keeps cell viability, neutrophil phagocytosis, and ROS production rates high is composed of PL⊕ + AS-3 + Alburex.
3.4 Effect of storage on PGC diluted in PL⊕ + AS-3 + Alburex
Having significantly improved and prolonged room-temperature storage of neutrophils with the PL⊕ + AS-3 + Alburex solution, we tested its ability to have the same effect on PGCs. We began by monitoring the pH and glucose concentration as the presence of several cell types, cell concentration, and metabolism in PGCs can modify these parameters and affect neutrophil storage conditions. The pH and glucose concentration diminish rapidly in GCs.26 A minor adjustment had to be made to this storage solution as the histidine buffer in this solution induces neutrophil degranulation in PGCs, a metric of neutrophil activation (results not shown). We thus replaced the histidine buffer with different concentrations of HEPES (none, 2.5%, and 7.5%) and adjusted the final volume of the solution with AS-3 as it outperformed other clinically approved solutions in all tests.
The earliest timepoint at which whole blood can be processed into PGCs is 16 to 24 h post-blood donation (Héma-Québec guidelines). For this reason, we could only study the effect of supplementing this blood product with diluent composed of PL + AS-3 + Alburex ± HEPES after the 16 to 24 h post-blood donation timepoint. In PGCs without diluent, we observed a substantial decrease in glucose concentration that reached significance 64 to 72 h post-blood donation (Table 1). Supplementing PGCs with PL + AS-3 + Alburex, without or with 2.5% HEPES, significantly increased the glucose concentrations at 16 to 24 h post-blood donation. These higher glucose levels were maintained up to 64 to 72 h post-blood donation (Table 1). In contrast, the pH in PGCs decreased more rapidly with than without diluent and reached significance as early as 40 to 48 h post-blood donation, even with the addition of HEPES. The PGC cellular composition was not significantly affected by storage up to 72 h post-blood donation, irrespective of the diluent composition (Table 1).
The glucose concentration, pH, and leukocyte composition of PGCs prior to and after room-temperature storage.
Diluent . | Time (hours) . | Glucose (mmol/L) . | pH . | WBCs (×109/L) . | Granulocytes (×109/L) . | Lymphocytes (×109/L) . | Monocytes (×109/L) . | Erythrocytes (×1012/L) . | Platelets (×109/L) . | Hemoglobin (g/L) . | Hematocrit (%) . |
---|---|---|---|---|---|---|---|---|---|---|---|
None | 0 | 23.73 (0.34) | 6.651 (0.021) | 4.0 to 10.5a | 2.0 to 7.8a | 0.9 to 5.3a | 0.1 to 1.1a | 4.2 to 6.0a | 150 to 500a | 125 to 180a | 38 to 54a |
16 to 24 | 16.39 (0.34) | 6.885 (0.008) | 72.56 (1.61) | 43.17 (2.39) | 25.31 (3.61) | 4.03 (0.24) | 3.32 (0.09) | 230.0 (17.23) | 105.6 (3.59) | 33 (1) | |
40 to 48 | 11.72 (0.37) | 6.735 (0.020) | 71.84 (1.74) | 41.23 (2.41) | 25.11 (2.88) | 5.47 (1.03) | 3.46 (0.16) | 267.1 (39.96) | 109.3 (1.92) | 34 (1) | |
64 to 72 | 6.767 (0.59)b | 6.545 (0.018)c | 71.81 (2.64) | 41.23 (1.45) | 22.84 (2.61) | 7.52 (1.54) | 3.33 (0.16) | 291.5 (14.55) | 106.5 (1.32) | 34 (0.3) | |
AS-3⊕ | 16 to 24 | 32.43 (0.65)c | 6.706 (0.013) | … | … | … | … | … | … | … | … |
40 to 48 | 27.48 (0.79) | 6.541 (0.019)c | 83.79 (8.45) | 51.61 (9.65) | 26.65 (2.65) | 5.52 (1.05) | 4.48 (1.0) | 294.4 (41.23) | 141.4 (27.16) | 44 (9) | |
64 to 72 | 23.89 (0.66) | 6.433 (0.026)c | 75.96 (4.06) | 44.82 (3.76) | 24.29 (3.63) | 6.74 (1.32) | 4.17 (0.92) | 292.2 (34.98) | 126.3 (22.52) | 41 (8) | |
AS-3⊕ + 2.5% HEPES | 16 to 24 | 30.46 (0.88)c | 6.686 (0.015) | … | … | … | … | … | … | … | … |
40 to 48 | 25.36 (0.90) | 6.551 (0.022)c | 82.87 (9.14) | 50.46 (9.50) | 26.55 (2.75) | 5.89 (1.09) | 4.14 (0.88) | 295.4 (23.68) | 130.9 (23.32) | 41 (8) | |
64 to 72 | 21.99 (0.90) | 6.462 (0.022)c | 76.35 (2.18) | 44.54 (3.18) | 23.36 (2.23) | 7.27 (1.15) | 3.59 (0.30) | 296.5 (12.47) | 111.0 (6.06) | 36 (2) | |
AS-3⊕ + 7.5% HEPES | 16 to 24 | 26.12 (0.60) | 6.672 (0.022) | … | … | … | … | … | … | … | … |
40 to 48 | 20.77 (0.70) | 6.568 (0.025)c | 81.44 (6.81) | 49.65 (7.51) | 25.41 (2.73) | 6.31 (1,0) | 3.86 (0.53) | 297.9 (8.36) | 122.2 (13.22) | 38 (5) | |
64 to 72 | 15.93 (0.60) | 6.459 (0.028)c | 70.61 (3.94) | 43.99 (3.70) | 18.11 (1.94) | 7.61 (1.19) | 3.56 (0.36) | 298.7 (15.58) | 110.7 (7.21) | 35 (3) |
Diluent . | Time (hours) . | Glucose (mmol/L) . | pH . | WBCs (×109/L) . | Granulocytes (×109/L) . | Lymphocytes (×109/L) . | Monocytes (×109/L) . | Erythrocytes (×1012/L) . | Platelets (×109/L) . | Hemoglobin (g/L) . | Hematocrit (%) . |
---|---|---|---|---|---|---|---|---|---|---|---|
None | 0 | 23.73 (0.34) | 6.651 (0.021) | 4.0 to 10.5a | 2.0 to 7.8a | 0.9 to 5.3a | 0.1 to 1.1a | 4.2 to 6.0a | 150 to 500a | 125 to 180a | 38 to 54a |
16 to 24 | 16.39 (0.34) | 6.885 (0.008) | 72.56 (1.61) | 43.17 (2.39) | 25.31 (3.61) | 4.03 (0.24) | 3.32 (0.09) | 230.0 (17.23) | 105.6 (3.59) | 33 (1) | |
40 to 48 | 11.72 (0.37) | 6.735 (0.020) | 71.84 (1.74) | 41.23 (2.41) | 25.11 (2.88) | 5.47 (1.03) | 3.46 (0.16) | 267.1 (39.96) | 109.3 (1.92) | 34 (1) | |
64 to 72 | 6.767 (0.59)b | 6.545 (0.018)c | 71.81 (2.64) | 41.23 (1.45) | 22.84 (2.61) | 7.52 (1.54) | 3.33 (0.16) | 291.5 (14.55) | 106.5 (1.32) | 34 (0.3) | |
AS-3⊕ | 16 to 24 | 32.43 (0.65)c | 6.706 (0.013) | … | … | … | … | … | … | … | … |
40 to 48 | 27.48 (0.79) | 6.541 (0.019)c | 83.79 (8.45) | 51.61 (9.65) | 26.65 (2.65) | 5.52 (1.05) | 4.48 (1.0) | 294.4 (41.23) | 141.4 (27.16) | 44 (9) | |
64 to 72 | 23.89 (0.66) | 6.433 (0.026)c | 75.96 (4.06) | 44.82 (3.76) | 24.29 (3.63) | 6.74 (1.32) | 4.17 (0.92) | 292.2 (34.98) | 126.3 (22.52) | 41 (8) | |
AS-3⊕ + 2.5% HEPES | 16 to 24 | 30.46 (0.88)c | 6.686 (0.015) | … | … | … | … | … | … | … | … |
40 to 48 | 25.36 (0.90) | 6.551 (0.022)c | 82.87 (9.14) | 50.46 (9.50) | 26.55 (2.75) | 5.89 (1.09) | 4.14 (0.88) | 295.4 (23.68) | 130.9 (23.32) | 41 (8) | |
64 to 72 | 21.99 (0.90) | 6.462 (0.022)c | 76.35 (2.18) | 44.54 (3.18) | 23.36 (2.23) | 7.27 (1.15) | 3.59 (0.30) | 296.5 (12.47) | 111.0 (6.06) | 36 (2) | |
AS-3⊕ + 7.5% HEPES | 16 to 24 | 26.12 (0.60) | 6.672 (0.022) | … | … | … | … | … | … | … | … |
40 to 48 | 20.77 (0.70) | 6.568 (0.025)c | 81.44 (6.81) | 49.65 (7.51) | 25.41 (2.73) | 6.31 (1,0) | 3.86 (0.53) | 297.9 (8.36) | 122.2 (13.22) | 38 (5) | |
64 to 72 | 15.93 (0.60) | 6.459 (0.028)c | 70.61 (3.94) | 43.99 (3.70) | 18.11 (1.94) | 7.61 (1.19) | 3.56 (0.36) | 298.7 (15.58) | 110.7 (7.21) | 35 (3) |
AS-3⊕ = AS-3 + Plasma-Lyte + Alburex.
Data are expressed as mean (SEM). 0 h represents cells post-collection, and 16 to 24 h represents cells post-transformation.
aRanges of normal values in whole blood.
bP < 0.05 represents the comparison to values at 0 h in whole blood.
cP < 0.05 represents the comparison to values at 16 to 24 h without diluent.
N = 4 to 9 pool.
WBCs, white blood cells.
The glucose concentration, pH, and leukocyte composition of PGCs prior to and after room-temperature storage.
Diluent . | Time (hours) . | Glucose (mmol/L) . | pH . | WBCs (×109/L) . | Granulocytes (×109/L) . | Lymphocytes (×109/L) . | Monocytes (×109/L) . | Erythrocytes (×1012/L) . | Platelets (×109/L) . | Hemoglobin (g/L) . | Hematocrit (%) . |
---|---|---|---|---|---|---|---|---|---|---|---|
None | 0 | 23.73 (0.34) | 6.651 (0.021) | 4.0 to 10.5a | 2.0 to 7.8a | 0.9 to 5.3a | 0.1 to 1.1a | 4.2 to 6.0a | 150 to 500a | 125 to 180a | 38 to 54a |
16 to 24 | 16.39 (0.34) | 6.885 (0.008) | 72.56 (1.61) | 43.17 (2.39) | 25.31 (3.61) | 4.03 (0.24) | 3.32 (0.09) | 230.0 (17.23) | 105.6 (3.59) | 33 (1) | |
40 to 48 | 11.72 (0.37) | 6.735 (0.020) | 71.84 (1.74) | 41.23 (2.41) | 25.11 (2.88) | 5.47 (1.03) | 3.46 (0.16) | 267.1 (39.96) | 109.3 (1.92) | 34 (1) | |
64 to 72 | 6.767 (0.59)b | 6.545 (0.018)c | 71.81 (2.64) | 41.23 (1.45) | 22.84 (2.61) | 7.52 (1.54) | 3.33 (0.16) | 291.5 (14.55) | 106.5 (1.32) | 34 (0.3) | |
AS-3⊕ | 16 to 24 | 32.43 (0.65)c | 6.706 (0.013) | … | … | … | … | … | … | … | … |
40 to 48 | 27.48 (0.79) | 6.541 (0.019)c | 83.79 (8.45) | 51.61 (9.65) | 26.65 (2.65) | 5.52 (1.05) | 4.48 (1.0) | 294.4 (41.23) | 141.4 (27.16) | 44 (9) | |
64 to 72 | 23.89 (0.66) | 6.433 (0.026)c | 75.96 (4.06) | 44.82 (3.76) | 24.29 (3.63) | 6.74 (1.32) | 4.17 (0.92) | 292.2 (34.98) | 126.3 (22.52) | 41 (8) | |
AS-3⊕ + 2.5% HEPES | 16 to 24 | 30.46 (0.88)c | 6.686 (0.015) | … | … | … | … | … | … | … | … |
40 to 48 | 25.36 (0.90) | 6.551 (0.022)c | 82.87 (9.14) | 50.46 (9.50) | 26.55 (2.75) | 5.89 (1.09) | 4.14 (0.88) | 295.4 (23.68) | 130.9 (23.32) | 41 (8) | |
64 to 72 | 21.99 (0.90) | 6.462 (0.022)c | 76.35 (2.18) | 44.54 (3.18) | 23.36 (2.23) | 7.27 (1.15) | 3.59 (0.30) | 296.5 (12.47) | 111.0 (6.06) | 36 (2) | |
AS-3⊕ + 7.5% HEPES | 16 to 24 | 26.12 (0.60) | 6.672 (0.022) | … | … | … | … | … | … | … | … |
40 to 48 | 20.77 (0.70) | 6.568 (0.025)c | 81.44 (6.81) | 49.65 (7.51) | 25.41 (2.73) | 6.31 (1,0) | 3.86 (0.53) | 297.9 (8.36) | 122.2 (13.22) | 38 (5) | |
64 to 72 | 15.93 (0.60) | 6.459 (0.028)c | 70.61 (3.94) | 43.99 (3.70) | 18.11 (1.94) | 7.61 (1.19) | 3.56 (0.36) | 298.7 (15.58) | 110.7 (7.21) | 35 (3) |
Diluent . | Time (hours) . | Glucose (mmol/L) . | pH . | WBCs (×109/L) . | Granulocytes (×109/L) . | Lymphocytes (×109/L) . | Monocytes (×109/L) . | Erythrocytes (×1012/L) . | Platelets (×109/L) . | Hemoglobin (g/L) . | Hematocrit (%) . |
---|---|---|---|---|---|---|---|---|---|---|---|
None | 0 | 23.73 (0.34) | 6.651 (0.021) | 4.0 to 10.5a | 2.0 to 7.8a | 0.9 to 5.3a | 0.1 to 1.1a | 4.2 to 6.0a | 150 to 500a | 125 to 180a | 38 to 54a |
16 to 24 | 16.39 (0.34) | 6.885 (0.008) | 72.56 (1.61) | 43.17 (2.39) | 25.31 (3.61) | 4.03 (0.24) | 3.32 (0.09) | 230.0 (17.23) | 105.6 (3.59) | 33 (1) | |
40 to 48 | 11.72 (0.37) | 6.735 (0.020) | 71.84 (1.74) | 41.23 (2.41) | 25.11 (2.88) | 5.47 (1.03) | 3.46 (0.16) | 267.1 (39.96) | 109.3 (1.92) | 34 (1) | |
64 to 72 | 6.767 (0.59)b | 6.545 (0.018)c | 71.81 (2.64) | 41.23 (1.45) | 22.84 (2.61) | 7.52 (1.54) | 3.33 (0.16) | 291.5 (14.55) | 106.5 (1.32) | 34 (0.3) | |
AS-3⊕ | 16 to 24 | 32.43 (0.65)c | 6.706 (0.013) | … | … | … | … | … | … | … | … |
40 to 48 | 27.48 (0.79) | 6.541 (0.019)c | 83.79 (8.45) | 51.61 (9.65) | 26.65 (2.65) | 5.52 (1.05) | 4.48 (1.0) | 294.4 (41.23) | 141.4 (27.16) | 44 (9) | |
64 to 72 | 23.89 (0.66) | 6.433 (0.026)c | 75.96 (4.06) | 44.82 (3.76) | 24.29 (3.63) | 6.74 (1.32) | 4.17 (0.92) | 292.2 (34.98) | 126.3 (22.52) | 41 (8) | |
AS-3⊕ + 2.5% HEPES | 16 to 24 | 30.46 (0.88)c | 6.686 (0.015) | … | … | … | … | … | … | … | … |
40 to 48 | 25.36 (0.90) | 6.551 (0.022)c | 82.87 (9.14) | 50.46 (9.50) | 26.55 (2.75) | 5.89 (1.09) | 4.14 (0.88) | 295.4 (23.68) | 130.9 (23.32) | 41 (8) | |
64 to 72 | 21.99 (0.90) | 6.462 (0.022)c | 76.35 (2.18) | 44.54 (3.18) | 23.36 (2.23) | 7.27 (1.15) | 3.59 (0.30) | 296.5 (12.47) | 111.0 (6.06) | 36 (2) | |
AS-3⊕ + 7.5% HEPES | 16 to 24 | 26.12 (0.60) | 6.672 (0.022) | … | … | … | … | … | … | … | … |
40 to 48 | 20.77 (0.70) | 6.568 (0.025)c | 81.44 (6.81) | 49.65 (7.51) | 25.41 (2.73) | 6.31 (1,0) | 3.86 (0.53) | 297.9 (8.36) | 122.2 (13.22) | 38 (5) | |
64 to 72 | 15.93 (0.60) | 6.459 (0.028)c | 70.61 (3.94) | 43.99 (3.70) | 18.11 (1.94) | 7.61 (1.19) | 3.56 (0.36) | 298.7 (15.58) | 110.7 (7.21) | 35 (3) |
AS-3⊕ = AS-3 + Plasma-Lyte + Alburex.
Data are expressed as mean (SEM). 0 h represents cells post-collection, and 16 to 24 h represents cells post-transformation.
aRanges of normal values in whole blood.
bP < 0.05 represents the comparison to values at 0 h in whole blood.
cP < 0.05 represents the comparison to values at 16 to 24 h without diluent.
N = 4 to 9 pool.
WBCs, white blood cells.
Cell surface marker expression was used as a metric to assess neutrophil activation in PGCs. Expression of neutrophil granule markers CD66b and CD11b increases over time during room-temperature storage without diluent reaching significance after 64 to 72 h post-blood donation (Table 2). The addition of PL + AS-3 + Alburex did not prevent this up-regulation, with a significant increase in CD66b and CD11b cell surface expression at 64 to 72 h post-blood donation. In contrast, supplementing PGCs with HEPES prevented this significant increase in CD66b and CD11b expression 64 to 72 h post-blood donation. Together, these results indicate that the addition of HEPES prevents degranulation and thus neutrophil activation. We also determined the expression of CLEC12A, an inhibitory receptor expressed in gelatinase granules, as a metric of neutrophil priming. Neutrophils increase their cell surface expression of CLEC12A in circulation in response to a rise in the levels of pro-inflammatory stimuli.35 CLEC12A expression remained unchanged when stored with or without diluent in all conditions tested except for a significant increase 40 to 48 h post-blood donation in diluent alone. In contrast to PGC neutrophils, the expression levels of these cell surface markers were stable in monocytes up to 64 to 72 h post-blood donation in the presence or absence of diluents (Table 2).
Surface marker expression on granulocytes and monocytes of PGCs prior to and after room-temperature storage.
Diluent . | Cells type . | CD66b (MFI) . | CD11b (MFI) . | CD14 (MFI) . | CLEC12A (MFI) . | ||||||||||||
---|---|---|---|---|---|---|---|---|---|---|---|---|---|---|---|---|---|
0 h . | 16 to 24 h . | 40 to 48 h . | 64 to 72 h . | 0 h . | 16 to 24 h . | 40 to 48 h . | 64 to 72 h . | 0 h . | 16 to 24 h . | 40 to 48 h . | 64 to 72 h . | 0 h . | 16 to 24 h . | 40 to 48 h . | 64 to 72 h . | ||
None | Granulocyte | 2,041 (295) | 2,951 (95) | 3,974 (513) | 4,938 (290)a,b | 2,339 (230) | 3,787 (668) | 5,490 (656) | 6,327 (559)a | 819 (83) | 805 (118) | 1,038 (153) | 1,042 (177) | 4,665 (344) | 5,295 (531) | 6,325 (312) | 6,034 (142) |
Monocyte | … | … | … | … | 2,485 (343) | 3,353 (495) | 4,559 (448) | 4,847 (636) | 6,085 (859) | 7,331 (588) | 10,621 (1,273) | 9,891 (709) | 8,731 (299) | 8,232 (561) | 8,711 (306) | 7,854 (329) | |
AS-3⊕ | Granulocyte | … | … | 4,088 (604) | 4,778 (270)a,b | … | … | 5,472 (361) | 5,701 (558)a | … | … | 1,064 (176) | 1,051 (147) | … | … | 6,865 (310)a | 6,200 (89) |
Monocyte | … | … | … | … | … | … | 4,538 (340) | 4,547 (497) | … | … | 8,819 (1,276) | 9,470 (922) | … | … | 8,948 (323) | 8,077 (330) | |
AS-3⊕ + 2.5% HEPES | Granulocyte | … | … | 4,098 (430) | 4,072 (247) | … | … | 5,567 (664) | 5,231 (562) | … | … | 1,083 (196) | 876 (124) | … | … | 6,230 (271) | 5,273 (233) |
Monocyte | … | … | … | … | … | … | 4,569 (490) | 4,568 (479) | … | … | 11,123 (1,567) | 10,122 (1,048) | … | … | 8,859 (311) | 8,145 (306) | |
AS-3⊕ + 7.5% HEPES | Granulocyte | … | … | 3,337 (267) | 3,708 (399) | … | … | 5,031 (812) | 5,039 (393) | … | … | 1,040 (151) | 914 (150) | … | … | 5,779 (268) | 5,130 (383) |
Monocyte | … | … | … | … | … | … | 4,152 (604) | 4,745 (541) | … | … | 9,963 (949) | 10,817 (1,173) | … | … | 8,639 (237) | 8,042 (322) |
Diluent . | Cells type . | CD66b (MFI) . | CD11b (MFI) . | CD14 (MFI) . | CLEC12A (MFI) . | ||||||||||||
---|---|---|---|---|---|---|---|---|---|---|---|---|---|---|---|---|---|
0 h . | 16 to 24 h . | 40 to 48 h . | 64 to 72 h . | 0 h . | 16 to 24 h . | 40 to 48 h . | 64 to 72 h . | 0 h . | 16 to 24 h . | 40 to 48 h . | 64 to 72 h . | 0 h . | 16 to 24 h . | 40 to 48 h . | 64 to 72 h . | ||
None | Granulocyte | 2,041 (295) | 2,951 (95) | 3,974 (513) | 4,938 (290)a,b | 2,339 (230) | 3,787 (668) | 5,490 (656) | 6,327 (559)a | 819 (83) | 805 (118) | 1,038 (153) | 1,042 (177) | 4,665 (344) | 5,295 (531) | 6,325 (312) | 6,034 (142) |
Monocyte | … | … | … | … | 2,485 (343) | 3,353 (495) | 4,559 (448) | 4,847 (636) | 6,085 (859) | 7,331 (588) | 10,621 (1,273) | 9,891 (709) | 8,731 (299) | 8,232 (561) | 8,711 (306) | 7,854 (329) | |
AS-3⊕ | Granulocyte | … | … | 4,088 (604) | 4,778 (270)a,b | … | … | 5,472 (361) | 5,701 (558)a | … | … | 1,064 (176) | 1,051 (147) | … | … | 6,865 (310)a | 6,200 (89) |
Monocyte | … | … | … | … | … | … | 4,538 (340) | 4,547 (497) | … | … | 8,819 (1,276) | 9,470 (922) | … | … | 8,948 (323) | 8,077 (330) | |
AS-3⊕ + 2.5% HEPES | Granulocyte | … | … | 4,098 (430) | 4,072 (247) | … | … | 5,567 (664) | 5,231 (562) | … | … | 1,083 (196) | 876 (124) | … | … | 6,230 (271) | 5,273 (233) |
Monocyte | … | … | … | … | … | … | 4,569 (490) | 4,568 (479) | … | … | 11,123 (1,567) | 10,122 (1,048) | … | … | 8,859 (311) | 8,145 (306) | |
AS-3⊕ + 7.5% HEPES | Granulocyte | … | … | 3,337 (267) | 3,708 (399) | … | … | 5,031 (812) | 5,039 (393) | … | … | 1,040 (151) | 914 (150) | … | … | 5,779 (268) | 5,130 (383) |
Monocyte | … | … | … | … | … | … | 4,152 (604) | 4,745 (541) | … | … | 9,963 (949) | 10,817 (1,173) | … | … | 8,639 (237) | 8,042 (322) |
AS-3⊕ = AS-3 + Plasma-Lyte + Alburex.
Data are expressed as mean (SEM).
aP < 0.05 represents the comparison to values at 0 h in whole blood.
bP < 0.05 represents the comparison to values at 16 to 24 h without diluent.
N = 3 to 4 pool of 5 donors.
MFI, mean fluorescence intensity.
Surface marker expression on granulocytes and monocytes of PGCs prior to and after room-temperature storage.
Diluent . | Cells type . | CD66b (MFI) . | CD11b (MFI) . | CD14 (MFI) . | CLEC12A (MFI) . | ||||||||||||
---|---|---|---|---|---|---|---|---|---|---|---|---|---|---|---|---|---|
0 h . | 16 to 24 h . | 40 to 48 h . | 64 to 72 h . | 0 h . | 16 to 24 h . | 40 to 48 h . | 64 to 72 h . | 0 h . | 16 to 24 h . | 40 to 48 h . | 64 to 72 h . | 0 h . | 16 to 24 h . | 40 to 48 h . | 64 to 72 h . | ||
None | Granulocyte | 2,041 (295) | 2,951 (95) | 3,974 (513) | 4,938 (290)a,b | 2,339 (230) | 3,787 (668) | 5,490 (656) | 6,327 (559)a | 819 (83) | 805 (118) | 1,038 (153) | 1,042 (177) | 4,665 (344) | 5,295 (531) | 6,325 (312) | 6,034 (142) |
Monocyte | … | … | … | … | 2,485 (343) | 3,353 (495) | 4,559 (448) | 4,847 (636) | 6,085 (859) | 7,331 (588) | 10,621 (1,273) | 9,891 (709) | 8,731 (299) | 8,232 (561) | 8,711 (306) | 7,854 (329) | |
AS-3⊕ | Granulocyte | … | … | 4,088 (604) | 4,778 (270)a,b | … | … | 5,472 (361) | 5,701 (558)a | … | … | 1,064 (176) | 1,051 (147) | … | … | 6,865 (310)a | 6,200 (89) |
Monocyte | … | … | … | … | … | … | 4,538 (340) | 4,547 (497) | … | … | 8,819 (1,276) | 9,470 (922) | … | … | 8,948 (323) | 8,077 (330) | |
AS-3⊕ + 2.5% HEPES | Granulocyte | … | … | 4,098 (430) | 4,072 (247) | … | … | 5,567 (664) | 5,231 (562) | … | … | 1,083 (196) | 876 (124) | … | … | 6,230 (271) | 5,273 (233) |
Monocyte | … | … | … | … | … | … | 4,569 (490) | 4,568 (479) | … | … | 11,123 (1,567) | 10,122 (1,048) | … | … | 8,859 (311) | 8,145 (306) | |
AS-3⊕ + 7.5% HEPES | Granulocyte | … | … | 3,337 (267) | 3,708 (399) | … | … | 5,031 (812) | 5,039 (393) | … | … | 1,040 (151) | 914 (150) | … | … | 5,779 (268) | 5,130 (383) |
Monocyte | … | … | … | … | … | … | 4,152 (604) | 4,745 (541) | … | … | 9,963 (949) | 10,817 (1,173) | … | … | 8,639 (237) | 8,042 (322) |
Diluent . | Cells type . | CD66b (MFI) . | CD11b (MFI) . | CD14 (MFI) . | CLEC12A (MFI) . | ||||||||||||
---|---|---|---|---|---|---|---|---|---|---|---|---|---|---|---|---|---|
0 h . | 16 to 24 h . | 40 to 48 h . | 64 to 72 h . | 0 h . | 16 to 24 h . | 40 to 48 h . | 64 to 72 h . | 0 h . | 16 to 24 h . | 40 to 48 h . | 64 to 72 h . | 0 h . | 16 to 24 h . | 40 to 48 h . | 64 to 72 h . | ||
None | Granulocyte | 2,041 (295) | 2,951 (95) | 3,974 (513) | 4,938 (290)a,b | 2,339 (230) | 3,787 (668) | 5,490 (656) | 6,327 (559)a | 819 (83) | 805 (118) | 1,038 (153) | 1,042 (177) | 4,665 (344) | 5,295 (531) | 6,325 (312) | 6,034 (142) |
Monocyte | … | … | … | … | 2,485 (343) | 3,353 (495) | 4,559 (448) | 4,847 (636) | 6,085 (859) | 7,331 (588) | 10,621 (1,273) | 9,891 (709) | 8,731 (299) | 8,232 (561) | 8,711 (306) | 7,854 (329) | |
AS-3⊕ | Granulocyte | … | … | 4,088 (604) | 4,778 (270)a,b | … | … | 5,472 (361) | 5,701 (558)a | … | … | 1,064 (176) | 1,051 (147) | … | … | 6,865 (310)a | 6,200 (89) |
Monocyte | … | … | … | … | … | … | 4,538 (340) | 4,547 (497) | … | … | 8,819 (1,276) | 9,470 (922) | … | … | 8,948 (323) | 8,077 (330) | |
AS-3⊕ + 2.5% HEPES | Granulocyte | … | … | 4,098 (430) | 4,072 (247) | … | … | 5,567 (664) | 5,231 (562) | … | … | 1,083 (196) | 876 (124) | … | … | 6,230 (271) | 5,273 (233) |
Monocyte | … | … | … | … | … | … | 4,569 (490) | 4,568 (479) | … | … | 11,123 (1,567) | 10,122 (1,048) | … | … | 8,859 (311) | 8,145 (306) | |
AS-3⊕ + 7.5% HEPES | Granulocyte | … | … | 3,337 (267) | 3,708 (399) | … | … | 5,031 (812) | 5,039 (393) | … | … | 1,040 (151) | 914 (150) | … | … | 5,779 (268) | 5,130 (383) |
Monocyte | … | … | … | … | … | … | 4,152 (604) | 4,745 (541) | … | … | 9,963 (949) | 10,817 (1,173) | … | … | 8,639 (237) | 8,042 (322) |
AS-3⊕ = AS-3 + Plasma-Lyte + Alburex.
Data are expressed as mean (SEM).
aP < 0.05 represents the comparison to values at 0 h in whole blood.
bP < 0.05 represents the comparison to values at 16 to 24 h without diluent.
N = 3 to 4 pool of 5 donors.
MFI, mean fluorescence intensity.
The viability and function of PGC neutrophils and monocytes were also determined during storage in the PL + AS-3 + Alburex ± HEPES diluent. Neutrophil viability was considerably lower 16 to 24 h post-blood donation than routinely observed in freshly isolated neutrophils, 59% compared with > 85% respectively, and did not significantly change up to 64 to 72 h post-blood donation (Table 3). In contrast, the addition of diluent in the presence or absence of 7.5% HEPES significantly diminished neutrophil viability 16 to 24 h post-blood donation. Monocytes, on the other hand, were more sensitive to storage as their viability significantly diminished 40 to 48 h post-blood donation in nondiluted PGCs. A similar observation was made with PGCs diluted with PL + AS-3 + Alburex in the presence or absence of 7.5% HEPES. To preserve neutrophil and monocyte viability up to 48 h in the presence of PL + AS-3 + Alburex, the diluent has to be supplemented with 2.5% HEPES.
Effect of supplementing PGCs with PL, AS-3, and HEPES on granulocyte and monocyte functions during room-temperature storage.
Diluent . | Cells type . | Viability (%) . | Phagocytosis (MFI) . | Intracellular ROS (MFI) . | |||||||||
---|---|---|---|---|---|---|---|---|---|---|---|---|---|
0 h . | 16 to 24 h . | 40 to 48 h . | 64 to 72 h . | 0 h . | 16 to 24 h . | 40 to 48 h . | 64 to 72 h . | 0 h . | 16 to 24 h . | 40 to 48 h . | 64 to 72 h . | ||
None | Granulocyte | 70.5 (5.4) | 59.5 (5.1) | 40.4 (7.8) | 43.0 (4.8) | 23,644 (1,111) | 34,283 (3,624) | 30,428 (3,731) | 25,316 (3,591) | 1.23 (0.19) | 1.43 (0.22) | 1.33 (0.22) | 1.47 (0.13) |
Monocyte | 50.1 (8.2) | 24.7 (6.9) | 13.5 (2.3)a | 22.5 (4.0) | 20,645 (1,318) | 28,060 (3,909) | 25,261 (3,934) | 18,186 (2,917) | 1.17 (0.03) | 1.10 (0.06) | 1.17 (0.15) | 1.27 (0.09) | |
AS-3⊕ | Granulocyte | … | … | 37.9 (7.0) | 35.4 (3.3)a | … | … | 26,887 (1,966) | 19,909 (3,004)b | … | … | 1.50 (0.06) | 1.37 (0.15) |
Monocyte | … | … | 15.0 (4.2)a | 21.4 (3.1) | … | … | 20,883 (5,116) | 11,716 (1,450)b | … | … | 1.30 (0.06) | 1.13 (0.09) | |
AS-3⊕ + 2.5% HEPES | Granulocyte | … | … | 48.1 (7.6) | 44.5 (2.6) | … | … | 27,249 (2,273) | 24,543 (3,767) | … | … | 1.60 (0.12) | 1.57 (0.19) |
Monocyte | … | … | 19.5 (4.8) | 23.3 (1.5) | … | … | 22,624 (4,708) | 15,012 (1,999) | … | … | 1.30 (0.06) | 1.33 (0.18) | |
AS-3⊕ + 7.5% HEPES | Granulocyte | … | … | 43.0 (6.1) | 41.6 (4.0)a | … | … | 25,457 (1,614) | 20,503 (3,455)b | … | … | 1.60 (0.10) | 1.60 (0.15) |
Monocyte | … | … | 14.3 (3.3)a | 21.7 (3.2) | … | … | 15,372 (2,130)b | 12,015 (1,770)b | … | … | 1.43 (0.12) | 1.40 (0.10) |
Diluent . | Cells type . | Viability (%) . | Phagocytosis (MFI) . | Intracellular ROS (MFI) . | |||||||||
---|---|---|---|---|---|---|---|---|---|---|---|---|---|
0 h . | 16 to 24 h . | 40 to 48 h . | 64 to 72 h . | 0 h . | 16 to 24 h . | 40 to 48 h . | 64 to 72 h . | 0 h . | 16 to 24 h . | 40 to 48 h . | 64 to 72 h . | ||
None | Granulocyte | 70.5 (5.4) | 59.5 (5.1) | 40.4 (7.8) | 43.0 (4.8) | 23,644 (1,111) | 34,283 (3,624) | 30,428 (3,731) | 25,316 (3,591) | 1.23 (0.19) | 1.43 (0.22) | 1.33 (0.22) | 1.47 (0.13) |
Monocyte | 50.1 (8.2) | 24.7 (6.9) | 13.5 (2.3)a | 22.5 (4.0) | 20,645 (1,318) | 28,060 (3,909) | 25,261 (3,934) | 18,186 (2,917) | 1.17 (0.03) | 1.10 (0.06) | 1.17 (0.15) | 1.27 (0.09) | |
AS-3⊕ | Granulocyte | … | … | 37.9 (7.0) | 35.4 (3.3)a | … | … | 26,887 (1,966) | 19,909 (3,004)b | … | … | 1.50 (0.06) | 1.37 (0.15) |
Monocyte | … | … | 15.0 (4.2)a | 21.4 (3.1) | … | … | 20,883 (5,116) | 11,716 (1,450)b | … | … | 1.30 (0.06) | 1.13 (0.09) | |
AS-3⊕ + 2.5% HEPES | Granulocyte | … | … | 48.1 (7.6) | 44.5 (2.6) | … | … | 27,249 (2,273) | 24,543 (3,767) | … | … | 1.60 (0.12) | 1.57 (0.19) |
Monocyte | … | … | 19.5 (4.8) | 23.3 (1.5) | … | … | 22,624 (4,708) | 15,012 (1,999) | … | … | 1.30 (0.06) | 1.33 (0.18) | |
AS-3⊕ + 7.5% HEPES | Granulocyte | … | … | 43.0 (6.1) | 41.6 (4.0)a | … | … | 25,457 (1,614) | 20,503 (3,455)b | … | … | 1.60 (0.10) | 1.60 (0.15) |
Monocyte | … | … | 14.3 (3.3)a | 21.7 (3.2) | … | … | 15,372 (2,130)b | 12,015 (1,770)b | … | … | 1.43 (0.12) | 1.40 (0.10) |
AS-3⊕ = AS-3 + Plasma-Lyte + Alburex.
Data are expressed as mean (SEM).
aP < 0.05 represents the comparison to values at 0 h in whole blood.
bP < 0.05 represents the comparison to values at 16 to 24 h without diluent.
N = 3 to 4 pool of 5 donors.
Effect of supplementing PGCs with PL, AS-3, and HEPES on granulocyte and monocyte functions during room-temperature storage.
Diluent . | Cells type . | Viability (%) . | Phagocytosis (MFI) . | Intracellular ROS (MFI) . | |||||||||
---|---|---|---|---|---|---|---|---|---|---|---|---|---|
0 h . | 16 to 24 h . | 40 to 48 h . | 64 to 72 h . | 0 h . | 16 to 24 h . | 40 to 48 h . | 64 to 72 h . | 0 h . | 16 to 24 h . | 40 to 48 h . | 64 to 72 h . | ||
None | Granulocyte | 70.5 (5.4) | 59.5 (5.1) | 40.4 (7.8) | 43.0 (4.8) | 23,644 (1,111) | 34,283 (3,624) | 30,428 (3,731) | 25,316 (3,591) | 1.23 (0.19) | 1.43 (0.22) | 1.33 (0.22) | 1.47 (0.13) |
Monocyte | 50.1 (8.2) | 24.7 (6.9) | 13.5 (2.3)a | 22.5 (4.0) | 20,645 (1,318) | 28,060 (3,909) | 25,261 (3,934) | 18,186 (2,917) | 1.17 (0.03) | 1.10 (0.06) | 1.17 (0.15) | 1.27 (0.09) | |
AS-3⊕ | Granulocyte | … | … | 37.9 (7.0) | 35.4 (3.3)a | … | … | 26,887 (1,966) | 19,909 (3,004)b | … | … | 1.50 (0.06) | 1.37 (0.15) |
Monocyte | … | … | 15.0 (4.2)a | 21.4 (3.1) | … | … | 20,883 (5,116) | 11,716 (1,450)b | … | … | 1.30 (0.06) | 1.13 (0.09) | |
AS-3⊕ + 2.5% HEPES | Granulocyte | … | … | 48.1 (7.6) | 44.5 (2.6) | … | … | 27,249 (2,273) | 24,543 (3,767) | … | … | 1.60 (0.12) | 1.57 (0.19) |
Monocyte | … | … | 19.5 (4.8) | 23.3 (1.5) | … | … | 22,624 (4,708) | 15,012 (1,999) | … | … | 1.30 (0.06) | 1.33 (0.18) | |
AS-3⊕ + 7.5% HEPES | Granulocyte | … | … | 43.0 (6.1) | 41.6 (4.0)a | … | … | 25,457 (1,614) | 20,503 (3,455)b | … | … | 1.60 (0.10) | 1.60 (0.15) |
Monocyte | … | … | 14.3 (3.3)a | 21.7 (3.2) | … | … | 15,372 (2,130)b | 12,015 (1,770)b | … | … | 1.43 (0.12) | 1.40 (0.10) |
Diluent . | Cells type . | Viability (%) . | Phagocytosis (MFI) . | Intracellular ROS (MFI) . | |||||||||
---|---|---|---|---|---|---|---|---|---|---|---|---|---|
0 h . | 16 to 24 h . | 40 to 48 h . | 64 to 72 h . | 0 h . | 16 to 24 h . | 40 to 48 h . | 64 to 72 h . | 0 h . | 16 to 24 h . | 40 to 48 h . | 64 to 72 h . | ||
None | Granulocyte | 70.5 (5.4) | 59.5 (5.1) | 40.4 (7.8) | 43.0 (4.8) | 23,644 (1,111) | 34,283 (3,624) | 30,428 (3,731) | 25,316 (3,591) | 1.23 (0.19) | 1.43 (0.22) | 1.33 (0.22) | 1.47 (0.13) |
Monocyte | 50.1 (8.2) | 24.7 (6.9) | 13.5 (2.3)a | 22.5 (4.0) | 20,645 (1,318) | 28,060 (3,909) | 25,261 (3,934) | 18,186 (2,917) | 1.17 (0.03) | 1.10 (0.06) | 1.17 (0.15) | 1.27 (0.09) | |
AS-3⊕ | Granulocyte | … | … | 37.9 (7.0) | 35.4 (3.3)a | … | … | 26,887 (1,966) | 19,909 (3,004)b | … | … | 1.50 (0.06) | 1.37 (0.15) |
Monocyte | … | … | 15.0 (4.2)a | 21.4 (3.1) | … | … | 20,883 (5,116) | 11,716 (1,450)b | … | … | 1.30 (0.06) | 1.13 (0.09) | |
AS-3⊕ + 2.5% HEPES | Granulocyte | … | … | 48.1 (7.6) | 44.5 (2.6) | … | … | 27,249 (2,273) | 24,543 (3,767) | … | … | 1.60 (0.12) | 1.57 (0.19) |
Monocyte | … | … | 19.5 (4.8) | 23.3 (1.5) | … | … | 22,624 (4,708) | 15,012 (1,999) | … | … | 1.30 (0.06) | 1.33 (0.18) | |
AS-3⊕ + 7.5% HEPES | Granulocyte | … | … | 43.0 (6.1) | 41.6 (4.0)a | … | … | 25,457 (1,614) | 20,503 (3,455)b | … | … | 1.60 (0.10) | 1.60 (0.15) |
Monocyte | … | … | 14.3 (3.3)a | 21.7 (3.2) | … | … | 15,372 (2,130)b | 12,015 (1,770)b | … | … | 1.43 (0.12) | 1.40 (0.10) |
AS-3⊕ = AS-3 + Plasma-Lyte + Alburex.
Data are expressed as mean (SEM).
aP < 0.05 represents the comparison to values at 0 h in whole blood.
bP < 0.05 represents the comparison to values at 16 to 24 h without diluent.
N = 3 to 4 pool of 5 donors.
Functional assays on erythrocyte-lysed PGCs supplemented with PL + AS-3 + Alburex ± HEPES revealed that phagocytosis of opsonized S. aureus remained stable in PGCs in diluent with 2.5% HEPES up to 64 to 72 h post-blood donation (Table 3). A similar observation was made for monocytes. The production of intracellular ROS was stable under all experimental conditions tested for neutrophils and monocytes.
Taken together, these observations suggest that addition of PL + AS-3 + Alburex + 2.5% HEPES in PGCs 16 to 24 h post-blood donation leads to a favorable increase in glucose concentration without affecting leukocyte composition, viability, phagocytosis, and intracellular ROS production.
4. Discussion
A major limitation in the preparation and distribution of GCs is their short shelf-life of 24 h at room temperature. We thus aimed to improve neutrophil room-temperature storage by identifying the combination of clinically approved additives used for transfusion that preserved neutrophil viability and function up to 72 h ex vivo. We developed a unique mixture of these additives that maintained neutrophil viability for 48 h at room temperature as well as phagocytic capacity and the fMLF-induced production of intracellular ROS up to 72 h. While this storage solution significantly extends neutrophil viability and preserved certain functions at room temperature, it was less effective at preserving extracellular ROS production after 24 h. Supplementing PGCs with this storage solution 16 to 24 h post-blood donation resulted in an increase in glucose concentration and a decrease in degranulation markers on neutrophils, suggesting a potential benefit for diluting PGCs with PL + AS-3 + Alburex + 2.5% HEPES. However, this combination of reagents maintained but did not significantly improve neutrophil viability, phagocytosis, or intracellular ROS production. The difference between the results using isolated neutrophils and PGCs is most likely due to neutrophil ageing prior to adding the storage solution in PGCs and possibly to the presence of other cell types such as red blood cells and platelets.
Preserving neutrophil viability is key to their use for cell therapy. Neutrophils rapidly undergo apoptosis ex vivo as early as 6 h post-isolation.23,26 We developed a storage solution that prolongs isolated neutrophil ex vivo survival up to 48 h at room temperature. We showed that the additive solutions in this storage solution that preserve neutrophil viability are PL⊕ and AS-3 whether they are used individually or in combination. While during longer storage times neutrophils stored in PL⊕ + AS-3 lose their viability, it remains significantly higher than the viability of neutrophils stored in AP alone. PL is a solution rich in electrolytes, and AS-3 is a red blood cell additive solution that in addition to electrolytes is composed of dextrose and adenine. Thus, the prolongation of neutrophil survival is mostly due to the electrolyte and dextrose content of these solutions. In contrast, the red blood cell additive solution SAGM that has a similar composition to AS-3 but lacks citric acid, sodium citrate, and monobasic sodium phosphate (Supplementary table 1) was not as effective in maintaining neutrophil viability (Fig. 2). Similarly, the albumin-rich solution, Alburex, did not preserve neutrophil viability at the same levels as PL⊕ + AS-3. This solution may not be sufficiently nutrient rich and buffered to prolong neutrophil storage and may dilute essential storage elements found in plasma such as glucose and buffers. Combining SAGM or Alburex with PL⊕ restored neutrophil viability for up to 48 h, underscoring the role of electrolytes in PL and buffers in preserving neutrophil viability ex vivo. Our observations indicate that albumin, contained in Alburex, alone does not prolong neutrophil survival during ex vivo storage at room temperature, suggesting a role for other components present in plasma in maintaining neutrophil viability.20,21,25
The neutrophil functions that are less stable within the first 24 h of room-temperature storage are ROS production and chemotaxis (Fig. 3A, B).36–38 In contrast, phagocytosis is a robust neutrophil response that is more stable ex vivo during room-temperature storage.26 We did not observe a significant change in phagocytosis during 24-h room-temperature storage except in the presence of AS-3 that enhances this neutrophil response. This may be partly explained by the acidic nature of AS-3 (pH 5.8).30 Neutrophils in an acidic environment exhibit increased phagocytosis efficiency than neutrophils in a neutral environment.39 The drastic decrease in phagocytosis and loss of intracellular ROS production after 72 h of storage was partially countered by supplementing AP with PL⊕ + AS-3. Phagocytosis diminished by 88% in AP compared with 48% in neutrophils stored in AP supplemented with PL⊕ + AS-3 after this period of time. Approximately 80% of intracellular ROS production was preserved up to 72-h room-temperature storage in this storage buffer. Thus, electrolyte supplementation of AP preserved more than half of the capacity of neutrophils to phagocytose and produce intracellular ROS. The presence of dextrose and other components in AS-3 may also contribute to this storage buffers' ability to preserve these neutrophil functions as PL⊕ + Alburex did not have the same effect on intracellular ROS production. Regarding chemotaxis, neutrophils stored for 24 h in AP with PL⊕ + AS-3 + Alburex preserved their full migration capacity toward fMLF up to a concentration of 10−8 M fMLF. Thus, the storage solution that outperforms all those solutions tested in this study was AP supplemented with PL⊕ + AS-3 + Alburex.
Extracellular ROS has the potential to cause tissue damage if produced in large quantities. Neutrophils stored in AP supplemented with PL⊕, AS-3 or both additives produced significantly more extracellular ROS than freshly isolated neutrophils (Fig. 3C). While an increase in ROS production by GC neutrophils may be beneficial for eradicating pathogens in the context of neutropenia, this significant increase in extracellular ROS production suggests that neutrophils may be primed. This may be due to the acidity of AS-3 caused by citric acid in this additive. Immune cells are known to recognize mild acidosis (pH 6.5) as a danger signal and may release agents to induce priming.40 It has also been suggested that neutrophils in an extracellular acidic environment are generally more sensitive to chemoattractants such as fMLF than in a neutral environment (pH 7.4).39 It is probably for this reason that the addition of buffers (PL⊕) intended to maintain pH results in a reduction in extracellular ROS production while not reaching significance, even though most studies show that superoxide production is reduced at acidic pH.41 Thus, we used cell surface marker expression to determine whether the PL + AS-3 + Alburex solution primed neutrophils. We observed no significant difference in the surface expression of CD62L on neutrophils stored for 24 or 48 h in AP alone compared with those stored in PL + AS-3+ Alburex at room temperature after PMA stimulation. Thus, our storage solution does not alter the activation-induced loss of CD62L in neutrophils. Similarly, we did not observe a significant increase in CD11b or CD63 expression in neutrophils stored in our storage solution compared with those stored in AP alone after PMA stimulation (Supplementary Fig. 2). Together, these observations suggest that the neutrophils stored in our storage solution are not primed.
As an initial attempt to translate our findings in freshly isolated neutrophils to GCs, we tested the PL⊕ + AS-3 + Alburex solution on PGCs since residual leucocyte units are produced daily in most centers during whole blood processing increasing their availability.42–44 To adhere to the standard operating procedure at Héma-Québec regarding the clinical use of residual leucocyte units, we tested the ability of our storage solution to preserve neutrophil viability and function 16 to 24 h post-blood donation of whole blood by healthy donors. This was a limiting factor in our study as PGC neutrophils at this timepoint in the processing of this blood product are only 59% viable compared with 85% to 90% viability of freshly isolated neutrophils (Table 3). A 20% to 30% decrease in viability indicates that neutrophils age during the first 16 to 24 h post-blood donation. Viability diminished by a further 20% by 64 to 72 h post-blood donation. The ageing process that begun prior to adding our storage additives may explain the lack of a beneficial effect of supplementing PGCs with PL⊕, AS-3, and HEPES on neutrophil survival and function. Since our storage solution is composed predominantly of AS-3, it provides a nonnegligible source of glucose which is essential for neutrophil functions such as phagocytosis and chemotaxis (Table 1).45 Although this solution reduces baseline pH, there is no significant difference over time when comparing the same condition to others, except in the absence of storage solution or in the presence of HEPES-free solution, where a decrease is observed after 48 h of storage (pH 6.885 and 6.706, respectively) compared with post-processing pH (6.545 and 6.433, respectively). This suggests that HEPES acts as a useful buffer for pH maintenance over time.
Previous research has demonstrated a significant increase in the expression of cell surface CD66b on neutrophils in GCs prepared by leukapheresis from G-CSF or prednisone-stimulated donors during the storage, indicative of a neutrophil activation profile (Table 2).26 In this study, the expression of the surface markers CD66b and CD11b was maintained in the presence of HEPES-supplemented storage solution, indicating that the addition of HEPES prevents degranulation of specific gelatinase and secretory granules during storage.
To our knowledge, no studies have characterized GC monocytes even though the proportion of monocytes in GCs is almost 4 times higher than in circulation (Table 1). Like neutrophils, monocytes play an essential role in fighting infections. Although their counts and surface marker expression remain unchanged at 64 to 72 h post-blood donation, their viability is more affected by storage. But in circulation monocytes have a longer half-life (1 d) than neutrophils (8 to 12 h).46,47 Monocytes derived from PGCs lose their viability more rapidly than neutrophils, as early as 40 to 48 h post-blood donation, except in the presence of storage additives. Phagocytosis is only affected in the presence of HEPES-rich or HEPES-free solution. A further study of GC monocytes would provide an indication of the role played by this cell type in the therapeutic efficacy of GCs and its impact on storage. Our findings thus show that the presence of different cell types in GCs affects their preservation.
5. Conclusion
We developed a new storage solution that prolongs neutrophil room-temperature storage. This solution not only will facilitate the study of neutrophils ex vivo for basic research but also provides a clinically usable diluent to prolong PGC storage. Prior to its use in the clinic, further optimization is required such as adding the storage solution at an earlier time during the processing of PGCs (e.g. on the day of blood donation) and further tailoring its composition to significantly improve the preservation of neutrophil and monocyte viability and function in this blood product. Evaluation of the efficacy of this storage solution in murine models of granulocyte transfusions will also be necessary prior to its clinical application. Our findings provide proof of principle that neutrophil room-temperature storage ex vivo can be significantly improved with unique mixtures of clinically approved additives and has the potential to increase the availability of PGCs for patients.
Acknowledgments
The authors would like to thank the donors for the whole blood donations as well as Dr Rénée Bazin for sharing her scientific expertise and reviewing the manuscript. The authors also thank Marie-Claude Lampron and Sahra Fonseca for their assistance with the whole blood processing.
Author contributions
M.-M.L. was involved in significant contribution to performing the research, data compilation and analysis, participated in writing the manuscript, and prepared the figures. M.-È.A., A.M., and G.P. contributed to performing the research and data compilation and part of the data analysis. J.P.A. and S.L. contributed to the conceptualization, data analysis, manuscript review, and funding acquisition. M.G. contributed to the supervision of the personnel, conceptualization and methodology of the study, data analysis, manuscript review, and funding acquisition. M.J.F. was involved in funding acquisition, resources and project administration, major contribution to the conceptualization and methodology of the study, the manuscript writing as well as review, editing, visualization, and supervised the personnel. This manuscript is included in M.-M.L.'s master's thesis (link: https://corpus.ulaval.ca/server/api/core/bitstreams/6be38d81-58df-4d06-9e6a-b381f223eff0/content).
Supplementary material
Supplementary material is available at Journal of Leukocyte Biology online.
Funding
This research was supported by funding from ThéCell (Québec Cell, Tissue and Gene Therapy Network) and Héma-Québec. A partnered MITACS and Héma-Québec scholarship was awarded to M.-M.L. (IT26712).
References
Author notes
Conflict of interest. The authors declare that the research was conducted in the absence of any commercial or financial relationships that could be construed as a potential conflict of interest.