-
PDF
- Split View
-
Views
-
Cite
Cite
Marwan Hassani, Guus Leijte, Niklas Bruse, Matthijs Kox, Peter Pickkers, Nienke Vrisekoop, Leo Koenderman, Differentiation and activation of eosinophils in the human bone marrow during experimental human endotoxemia, Journal of Leukocyte Biology, Volume 108, Issue 5, Nov 2020, Pages 1665–1671, https://doi.org/10.1002/JLB.1AB1219-493R
- Share Icon Share
Abstract
Acute infection is characterized by eosinopenia. However, the underlying mechanism(s) are poorly understood and it is unclear whether decreased mobilization/production of eosinophils in the bone marrow (BM) and/or increased homing to the tissues play a role. The objective of this study was to investigate the differentiation and activation status of eosinophils in the human BM and blood upon experimental human endotoxemia, a standardized, controlled, and reproducible model of acute systemic inflammation. A BM aspirate and venous blood was obtained from seven healthy volunteers before, 4 h after, and 1 week after intravenous challenge with 2 ng/kg endotoxin. Early progenitors (CD34+/IL-5Rα+), eosinophil promyelocytes, myelocytes, metamyelocytes, and mature eosinophils were identified and quantified in the bone marrow and blood samples using flowcytometry based on specific eosinophil markers (CD193 and IL-5Rα). Activation status was assessed using antibodies against known markers on eosinophils: Alpha-4 (CD49d), CCR3 (CD193), CR1 (CD35), CEACAM-8 (CD66b), CBRM 1/5 (activation epitope of MAC-1), and by plasma cytokine analysis. Four hours after endotoxin administration, numbers of mature eosinophils in the blood and in the BM markedly declined compared with baseline, whereas numbers of all eosinophil progenitors did not change. The remaining eosinophils did not show signs of activation or degranulation despite significantly increased circulating levels of eotaxin-1. Furthermore, the expression of CD49d and CD193 on eosinophils was lower compared to baseline, but normalized after 7 days. Together these data imply that circulatory eosinopenia after an innate immune challenge is mediated by CD49d-mediated homing of eosinophils to the tissues.
1 Introduction
Eosinophils are cytotoxic granulocytes that are typically involved in host defense against multicellular parasites.1 Apart from their immune function, eosinophils are also thought to be important for homeostatic functions,2 such as maintenance of metabolic homeostasis,3 tissue remodeling4 and T-cell selection in the thymus.5 On the other hand, eosinophils play a pathological role in diseases such as asthma and eosinophilic esophagitis.6,7 In asthma, these cells are recruited to the airways after allergic or nonallergic stimuli and are responsible for bronchial airway inflammation.8 In eosinophilic esophagitis, eosinophils are present in the esophageal tissue but their role in disease pathogenesis remains unresolved.7 Most of these type 2 (T2) driven diseases are accompanied with eosinophilia in the circulation.9,10
In contrast, eosinophil numbers are markedly reduced in response to bacterial infection.11 This phenomenon was initially believed to result from enhanced release of adrenal glucocorticoids.12 However, adrenalectomized mice subjected to acute pneumococcal infection still displayed eosinopenia in the absence of enhanced circulating corticosterone levels.11 Therefore, the disappearance of eosinophils from the circulation must be mediated by a different mechanism. So far, this mechanism has not been elucidated and especially hard to address in humans. Understanding of the underlying mechanism might teach us more about type 2 immunity, since there is clear cross-talk between T2 immunity and innate immune responses. This is clearly illustrated by studies showing that exposure to environmental LPS can affect the clinical course of allergic diseases, including asthma.13 In accordance, it was demonstrated that CD14, a co-receptor for LPS, is one of the risk genes for asthma.14 We have previously published that experimental human endotoxemia (a model of acute systemic inflammation in humans in vivo) results in eosinopenia within 2 h after endotoxin administration.15 However, we did not establish whether this was due to decreased mobilization/production of eosinophils in the bone marrow (BM) or a result of increased homing from the blood to the tissues. In the present study, we investigated the maturation and activation status of eosinophils in the BM and blood compartments of healthy volunteers during homeostasis and during experimental endotoxemia in order to delineate the mechanisms underlying the previously observed eosinopenia. These data were complemented by determining eosinophil modulating cytokines and chemokines in blood plasma.
2 Materials and Methods
2.1 Ethics, subjects, and study design
In this study, we included 7 healthy male volunteers who participated in a human endotoxemia study performed at the Radboud university medical center in Nijmegen, the Netherlands (ref. no. NL61136.091.17). This study was approved by the local ethics review board (CMO Arnhem-Nijmegen; reference no. 2017–3607) and was conducted in compliance with declaration of Helsinki (Forteleza, 2013); International Conference on Harmonisation Good Clinical Practice guidelines, and the rulings of the Dutch Medical Research Involving Human Subjects Act. Written informed consent was obtained from all study participants. All individuals were thoroughly screened and found to be healthy. Among the exclusion criteria were use of medication, history or signs of atopic disease, and signs of acute infection. All subjects underwent an intravenous endotoxin challenge according to a standardized protocol described in detail elsewere.16 In short, a single dose of 2 ng per kilogram of bodyweight LPS (derived from Escherichia coli O:113, Lot #94332B1, National Institutes of Health, Bethesda, MD) was injected (t = 0 h) to induce a controlled systemic inflammatory response. Bone marrow and blood were sampled at three timepoints: at baseline (7 days before endotoxin administration), 4 h after endotoxin administration, and in 6 out of 7 volunteers 7 days after endotoxin administration (Fig. 1).
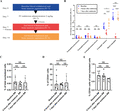
Study flow chart and the number of eosinophils and their progenitors after endotoxin challenge. (A) Study design. Seven healthy volunteers underwent a baseline bone marrow aspiration and vena puncture (blue). Seven volunteers were challenged with an intravenous dose of 2 ng/kg lipopolysaccharide (LPS) at day 7 and a second bone marrow aspiration and blood withdrawal 4 h later (red). Finally, 6 volunteers underwent a final bone marrow aspiration and blood withdrawal at day 14 (orange). (B) Number of eosinophils in the bone marrow and blood, and their progenitors (from eosinophil promyelocyte stage and further) in the bone marrow are depicted as a percentage of total nucleated cells. (C) Number of EoPs (CD34+ and IL-5Rα+ cells) in the bone marrow is depicted as a percentage of total nucleated cells or (D) as a percentage of CD34+ cells. (E) Number of CD34+ cells is depicted as a percentage of total nucleated cells. Results are plotted as median ± IQR (interquartile range). A Wilcoxon matched-pairs signed rank test was performed. NS, not significant. *P ≤ 0.05; **P ≤ 0.01; ****P ≤ 0.0001
2.2 Sample processing
Blood was collected in sodium heparin tubes (Vacuette® Greiner bio-one, Kremsmünster, Austria) and bone marrow aspirates were collected in syringes containing a sodium heparin solution (3:1 ratio). Thereafter, erythrocytes were lysed using an ice cold isotonic lysis buffer consisting of 150 mM NH4Cl, 10 mM KHCO3, and 0.1 mM NA2EDTA. Leukocytes were washed and resuspended in a staining buffer consisting of PBS supplemented with 0.32% w/v trisodium citrate and 10% w/v human plasma albumin solution. Samples were immediately transported on ice to the UMCU and further processed within 90 min. For determination of plasma cytokines, ethylenediaminetetraacetic acid anticoagulated blood was centrifuged directly after withdrawal (2000 × g at 4°C for 10 min), and stored at –80°C until further analysis.
2.3 Flow cytometry
One million cells were stained with antibodies for 30 min at a concentration of 40 million cells per ml on ice in the UMCU and washed twice before analysis on the LSR Fortessa flow cyometer (BD, Mountain View, CA, USA). An overview of the antibodies that were used for staining is provided in Supplementary Table 1. Eosinophils and their progenitors in blood and bone marrow were identified as published before (Supplementary Fig. 1A and B).17 Also early eosinophil progenitors (EoPs) were identified as was published before (Supplementary Fig. 1C and D).18
2.4 Cytokine analysis
Cytokine concentrations were determined in one batch using a multiplex assay (Milliplex, Millipore, Billerica, MA, USA) according to the manufacturers’ guidelines.
2.5 Data analysis and representation
FlowJo v10 (LLC, Ashland, OR, USA) was used to analyze the flow cytometry data and to determine the different median fluorescence intensities. Statistical analysis was performed using GraphPad Prism 7.04 (Graphpad Software, La Jolla, CA, USA). Data are presented as median (interquartile range). A Wilcoxon matched-pairs signed rank test was performed to compare two groups. A P-value of <0.05 was considered statistically significant.
3 Results and Discussion
3.1 Subject characteristics
Blood and BM samples were collected from 7 healthy male volunteers at baseline (day 0) and 4 h after endotoxin administration (day 7), and from 6 volunteers 1 week after endotoxin administration (day 14, Fig. 2A). Age was 23 (19–28) years (median, [Q1–Q3]), weight 85 (85–87) kg, height 1.84 (181–185), m and BMI 25.0 (24.8–25.9) kg/m2. Blood eosinophil count at baseline was 0.19 (0.17–0.26) × 106/ml. No adverse effects occurred during the conduct of the study.
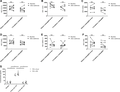
Change of surface markers on mature eosinophils in the BM and circulation after endotoxin administration. Median fluorescence intensity is plotted for the different surface markers of mature eosinophils in bone marrow and blood at baseline and 4 h after endotoxin. (A) CD11b, (B) CD35, (C) CD66b, (D) CD62L, (E) CBRM 1/5 (antibody that only recognizes the activated epitope of Mac-1), and (F) CD64. Individual data points are shown. (G) Level of TNF-α in plasma and bone marrow supernatant is plotted for the different time points (baseline, 4 h after endotoxemia and 1 week after endotoxemia). Median with interquartile range is indicated. A Wilcoxon matched-pairs signed rank test was performed. NS, not significant. *P ≤ 0.05
3.2 Decrease in the number of mature eosinophils in bone marrow and blood during experimental human endotoxemia
We have previously shown that the number of eosinophils in the circulation markedly decrease during experimental human endotoxemia within 2 h.15 This decrease remained present for at least 8 h and was restored to baseline levels after 24 h.15 To determine whether this eosinopenia is mainly a result of decreased production in the bone marrow or increased homing to the tissue, we studied the effect of endotoxin administration on eosinophils and their progenitors in the BM. At baseline, the median percentages of total nucleated cells (Q1–Q3) of promyelocytes, myelocytes, metamyelocytes, and mature eosinophils in the BM were 0.13% (0.05–0.31%), 0.28% (0.12–0.34%), 0.22% (0.11–0.35%), and 1.4% (1.3–1.8%), respectively (Fig. 1B). The median percentage of blood eosinophils at baseline was 3.2% (2.7–5.2%). Four hours after endotoxin administration, the percentage of progenitors (promyelocytes, myelocytes, and metamyelocytes) in BM did not significantly change (Fig. 1B). Also, the number of early EoPs as a percentage of total white blood cells (WBC) or as a percentage of total CD34+ cells in the BM did not change (Fig. 1C and D, respectively). Furthermore, the proportion of BM CD34+ cells within the total WBC was also not attenuated after endotoxin administration. However, one should take into account that only one time point (t = 4 h) was measured and changes might have occurred at other moments. On the other hand, in our earlier work, we have established that the number of eosinophils in the circulation was already restored within 24 h after endotoxin administration, which also suggested no alterations in BM eosinophilopoiesis.15
In contrast, the percentage of mature eosinophils in the BM decreased significantly to 0.67% (0.54–1.0%), albeit to a lesser extent than in the circulation which showed a decrease to 0.20% (0.13–0.24%) (see Fig. 1B). The absolute number of mature eosinophils also decreased in blood and bone marrow after endotoxemia despite an increase in the total white blood cell count in the peripheral blood (Supplementary Fig. 2). One week after the endotoxin challenge, the number of cells both in the circulation and in the BM was normalized (Fig. 1B). So, endotoxin administration most likely resulted in mobilization of only the mature eosinophils from the BM to the circulation. The situation in the peripheral blood was more complex and likely mediated by an additional mechanism: cells may disappear from the circulation due to increased margination in the sinusoids of liver and spleen,19,20 or by transmigration and homing into distant tissues such as gut and adipose tissue (see review).2 Indeed, more circulatory eosinophils transmigrated into the tissue of patients with asthma in response to a bronchial segmental challenge with endotoxin,21 which is possibly initiated by the release of chemoattractants by neutrophils.22
3.3 Effect of acute experimental inflammation on surface markers of eosinophils
To address whether endotoxin leads to activation or degranulation of eosinophils in the BM or blood, a selection of surface markers was measured: CD11b, CD35, CD66b, CD62L, and CBRM 1/5 (recognizes an activation epitope of MAC-1). No differences in marker expression on the different eosinophil precursors were measured during experimental endotoxemia (Supplementary Fig. 3). The same was true for most surface proteins on mature eosinophils in the BM and circulation (Fig. 2). We have also measured activation markers on mature eosinophils in the circulation of 6 new donors at baseline and after endotoxemia on a fast (<25 min) and fully automated flow cytometer (Aquios, Beckman Coulter, Pasadena, CA, USA) as was described in detail before23 in order to decrease the effects of in vitro manipulation of cells to a minimum. Again no change in expression of activation markers after endotoxemia was found (Supplementary Fig. 4), indicating that in vitro manipulation of eosinophils did not play a significant role in our protocol.
Therefore, blood and BM eosinophils did not seem to exhibit an activated or degranulated phenotype during endotoxemia. This is surprising, as TNF-α increased after endotoxin administration in blood and in the bone marrow (see Fig. 2G).24 This cytokine can stimulate eosinophils in vitro that can lead to degranulation.25 However, the concentration of TNF-α used in vitro (100 ng/ml) was much higher than the concentration reached in vivo (0.5 ng/ml, see Fig. 2G).24,25
On the other hand, following endotoxin administration some other markers showed slight but significant alterations. For instance, CD193 expression on mature eosinophils in the BM was attenuated after endotoxin challenge (Fig. 3A). This difference was not observed in the circulation (Fig. 3A). One week after endotoxin challenge, expression of CD193 on BM mature eosinophils returned to normal levels (Fig. 3A). In an earlier study, we have found that CD193 is slightly up-regulated upon maturation of eosinophils in the bone marrow.17 So, a possible explanation as to why CD193 expression is reduced after endotoxin administration is “rejuvenation” of the BM compartment, since it is plausible that only the most mature eosinophils are released into the circulation during acute inflammation. The unchanged CD193 expression on circulatory eosinophils supports this hypothesis. Additionally, we found that the expression of CD49d on only circulatory eosinophils was also attenuated after endotoxin administration (Fig. 3B). This decrease returned to normal after 1 week. The decrease in CD49d expression on blood eosinophils may imply that CD49d is involved in the recruitment of eosinophils to tissues, leaving behind the cells with the lowest expression as also suggested by the work of Johansson and colleagues.26 This is also supported by the finding that treatment of human umbilical vein endothelial cells (HUVEC cells) with LPS resulted in adherence of eosinophils to these cells via the interaction between VLA-4 (integrin dimer composed of CD49d and CD29) and VCAM-1.27
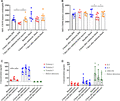
Expression of CD193 and CD49d a on mature eosinophils and cytokine concentration in plasma before and after endotoxin. Median fluorescence intensity (MFI) is plotted for two different surface markers of mature eosinophils in bone marrow and blood at baseline (blue), 4 h after endotoxin administration (red) and 1 week after endotoxin administration (orange): (A) CD193, (B) CD49d, and (C) Eotaxin 1–3 concentrations in the plasma are indicated. Red shows eotaxin-1, blue shows eotaxin-2, and green shows eotaxin-3. (D) IL-5, IL-3, and GM-CSF concentrations in the plasma are also indicated. Red shows IL-5, blue shows IL-3, and green shows GM-CSF. Medians ± IQR (interquartile range) are indicated. A Wilcoxon matched-pairs signed rank test was performed. All conditions were tested, but only statistical significant results are indicated. *P ≤ 0.05 and **P ≤ 0.01
3.4 Effect of endotoxin administration on plasma cytokine levels
The eosinophil-specific chemokines, eotaxin 1, 2, and 3, were measured in plasma at baseline, 4 h and 1 week after experimental endotoxemia. Eotaxin 3 was not detectable at all three time points (Fig. 3C). Eotaxin 1 and 2 both increased in blood following endotoxemia, but this increase was only statistically significant for eotaxin-1 (Fig. 3C), although eotaxin-2 showed an obvious trend (P = 0.078). Both chemokines returned to baseline level after 1 week (Fig. 3C). Since, we also observed a decrease in CD49d expression on circulatory eosinophils (Fig. 3B), it is tempting to speculate that systemic endotoxin challenged led to eosinophil transmigration via VLA-4 to the tissues in response to local production of eotaxin-1.28 In contrast, l-selectin did not seem to be involved in transmigration of eosinophils as its expression did not change (Fig. 2D and Supplementary Fig. 4C). This is different from the role of l-selectin in neutrophils where it has been shown that LPS can activate neutrophils through this receptor.29
The plasma levels of IL-3, IL-5, and GM-CSF were close to their detection limits in the plasma during steady state (Fig. 3D). Following 4 h and 1 week after endotoxemia, the level of these cytokines did not show evident alterations (Fig. 3D).
Our findings implicate that the eosinophil compartment is influenced by endotoxin-induced systemic inflammation beyond the Th2/ILC2-induced allergic type of response. This nonallergic mechanism that is present during endotoxin-induced innate immune responses might be important in homing of eosinophils to tissues that contain these cells also in homeostasis such as gut,30 uterus,31 thymus,5 and adipose tissue.3 It is intuitive that such homeostatic homing is not associated with cytotoxic activation of the cells as the risk for collateral damage to the tissue is too large. Similar mechanisms might also contribute to recruitment of eosinophils to tissues in allergic diseases as the gene for the LPS co-receptor CD14 is a risk gene for allergic asthma.14 However, in our model it is unknown whether LPS can interact with the airway epithelium. Therefore, the mechanisms induced by LPS-induced systemic inflammation might not be part of the mechanisms operational in allergic disease where epithelial derived cytokines/alarmins are involved in the control of eosinophil homing from the periphery to the airways. In conclusion, we propose a model in which experimental human endotoxemia induces a partial mobilization of mature eosinophils from the BM into the circulation and a large mobilization of circulatory eosinophils into tissues without signs of activation, degranulation, or enhanced production of eosinophil precursors. This mechanism is probably regulated through VLA-4 and eotaxin-1.
Authorship
M.H. designed and performed experiments. N.V. and L.K. supervised experimental design and data analysis. M.H. wrote manuscript. L.K. helped draft and revise the manuscript. N.V. and L.K. revised the manuscript. M.K., P.P., and G.L. designed the human endotoxemia study. G.L. and N.B. performed endotoxemia experiments. All authors read and approved the final manuscript.
Abbreviations
Acknowledgments
The authors thank Manita Bremmers, the physician assistant, who performed the bone marrow aspirations. The authors thank Jelle Gerretsen of the Department of Intensive Care Medicine, Nijmegen for his help during the preparation of the samples.
Disclosures
The authors declare no conflicts of interest.
References