-
PDF
- Split View
-
Views
-
Cite
Cite
Katy C K Lam, Moses K N Lam, C S Chim, Godfrey C F Chan, James C B Li, The functional role of surface molecules on extracellular vesicles in cancer, autoimmune diseases, and coagulopathy, Journal of Leukocyte Biology, Volume 108, Issue 5, Nov 2020, Pages 1565–1573, https://doi.org/10.1002/JLB.3MR0420-067R
- Share Icon Share
Abstract
Extracellular vesicles (EVs) are nanosized particles that have emerged as mediators for intercellular communication in physiologic and pathologic conditions. EVs carry signaling information on their bilipid membrane as well as cargo within, allowing them to perform a wide range of biologic processes and contribute to pathophysiologic roles in a wide range of diseases, including cancer, autoimmune diseases and coagulopathy. This review will specifically address the function of surface molecules on EVs under normal and diseased conditions, as well as their potential to emerge as therapeutic targets in clinical settings, and the importance of further research on the surface topography of EVs.
1 Introduction
Extracellular vesicles (EVs) are a heterogeneous group of cell-derived membranous vesicles, broadly classified into three main groups of vesicles. Exosomes are the smallest in size and are less than 150 nm in diameter and are formed when multivesicular bodies fuse with the plasma membrane and released to extracellular space. Microvesicles and apoptotic bodies are larger in size (50–1000 nm and 500–2000 nm in diameter, respectively) and emerge from the outward budding of plasma membrane of living and dying cells respectively.1–3 Whereas the contents of EVs, including proteins, lipids, and nucleic acids such as microRNAs (miRNAs) are relatively well studied, the surface components of EVs are less so. Nonetheless, technological advances open up the possibility of detecting and phenotyping EV surface molecules extensively with the use of flow cytometry, Western blotting, EV arrays, and surface plasmon resonance imaging, among others.4 Surface molecules on EV surfaces have been shown to have multiple functions, such as facilitation of trafficking and uptake of intracellular information by recipient cells, antigen presentation, and pathogenesis of certain diseases. They are also potential candidates for biomarkers and therapeutic targets, which can be useful for diagnosis, tracking of disease progression, prognosis, and treatment under a clinical setting.
2 EV Trafficking and Uptake by Recipient Cells
It has been established that the trafficking and uptake of EVs from one cell to another is an important mechanism of signaling between distant cells.5 The uptake of EVs by recipient cells can be achieved by two major pathways, either by fusion with the plasma membrane of the recipient cell or by endocytosis.6–10 A number of surface molecules are shown to be responsible for the interaction between EVs and recipient cells for their uptake (Fig. 1). Tetraspanins, a four-transmembrane protein family,11 have been shown to be responsible for cell adhesion, signaling, and protein interaction.12 Exosomes enriched in tetraspanins are able to selectively target recipient cells by expressing different subtypes of tetraspanin on the surface of exosomes.5 For instance, it has been shown that differences in the tetraspanin web in exosomes derived from BSp73AS cell line (AS) can lead to uptake by different cells. This preferential binding is thought to be the result of tetraspanin recruiting additional protein molecules. Depending on whether the exosomal surface expresses tetraspanin 8 (Tspan8) and which molecules are associated with Tspan8 (such as integrin β4) if it is present on the surface, these exosomes will be preferentially taken up by different cells, for example, AS-Tspan8-β4 exosomes are taken up by kidney cells; AS-Tspan8 exosomes by endothelial cells, through the binding of CD49 on the endothelial cell surface; AS exosomes without Tspan8 on the surface are taken up by pericytes.13,14 Other proteins have also been reported to affect EV internalization by recipient cells. For example, scavenger receptor type B-1 (SR-B1) in high-density lipoproteins (HDL) has been shown to play a role in EV uptake, as its blockage by synthetic nanoparticle mimic of HDL decreases uptake of melanoma-derived EV into cells.15 Glycans on the surface of EVs are also important for exosome uptake by cells. In particular, sialic acids on the terminal sites of glycans are responsible for the uptake and internalization of adipose tissue-derived mesenchymal stem cells (ADSCs) exosomes by CD11b+ antigen presenting cells (APCs) via siglec binding.16 There has also been indication of a role of proteoglycans in the uptake of EVs, as the inhibition of CD44 inhibits the internalization of EVs originated from mesenchymal stem cells (MSCs) by tubular epithelial cells.17,18 However, there is also research evidence suggesting that not all EV surface proteoglycans are associated with EV docking on cell surface.19 Fibronectin, abundant on the EVs from microvascular endothelial cells, has also been shown to play a role in their internalization.20 Phosphatidylserine (PS), a phospholipid abundant on the surface of EVs, may also be involved in exosomal internalization as it has been shown to bind to TIM4, a phagocytic receptor. Its role has been indicated when the uptake of leukemic cell derived-exosomes decreases by blocking TIM4.1 Overall, these data suggested that various EV surface molecules play a role in EV internalization by recipient cells.
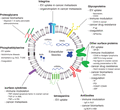
Depicts the general functions of different EV surface molecules in diseases. These surface molecules play a role in EV (and the cargo consisting of nucleic acids, proteins, and lipids) trafficking and uptake by recipient cells, as well as the pathogenesis of various diseases. AML = acute myeloid leukemia; autoAg = autoantigen; CRC = colorectal cancer; EGFR = epidermal growth factor receptor; Ig-BCR = immunoglobulin B-cell receptor; IgG = immunoglobulin G; miRNAs = microRNAs; MM = multiple myeloma; P-gp = P-glycoprotein; PDI = protein disulfide isomerase; RA = rheumatoid arthritis; SR-B1 = scavenger receptor type B-1; SLE = systemic lupus erythematosus; TF = tissue factor; T1DM = type I diabetes mellitus; and vWF = von Willebrand factor
3 EV Surface Molecules and Their Role in Diseases
3.1 Cancer
The functional role of EVs has been most widely studied in cancer diseases. The discovery of MHC class II molecules, which are responsible for antigen presentation to CD8+ T cells,21 on the surface of EVs secreted by B lymphocytes is the first indication that EV surface molecules are involved in modulating immune response and interacting with host immune system.22 Previous experiments have demonstrated that multiple cancer types, including pancreatic, colorectal, and lung cancer, can secrete EVs that carry CD44v7/8 and CCR6 on the surface and deliver them to monocytes.23 Up-regulation in CD44v has been proven to increase the ability to react with hyaluronic acid (HA) in monocytes, is associated with the differentiation of monocytes to tissue macrophages, thereby supporting activated T-cell survival.24 A decrease in proinflammatory cytokine production in B lymphocytes and reduced interaction between CD4+ T cells and APCs have also been demonstrated by blocking CD44v,25 indicating a potential role in immunologic stimulation via CD44v transferred from the surface of tumor-derived EVs (TEVs). Surface CD30 ligand on classical Hodgkin lymphoma-derived EVs has been shown to interact with CD30+ granulocytes and stimulate IL-8 release,26 a cytokine that has been indicated in suppressing apoptosis in neutrophils.27 Heat shock protein (HSP)-70 expressed on the surface of EVs derived from lung cancer is responsible for proinflammatory cytokine production by MSCs via TLR2, which in turn results in improved survival of tumor cells.28 Together, these findings suggest that TEV surface molecules can induce host immune response. By contrast, TEVs have also been shown to suppress host immune response. The expression of Fas ligand (FasL) and TNF-related apoptosis factor on the surface of EVs derived from ovarian cancer, for example, has been shown to at least partially account for the apoptosis of lymphocytes, thus allowing tumor cells to escape from immune surveillance.29 In a similar fashion, prostate cancer-derived EVs also express surface FasL, which is responsible for the induction of CD8+ T cells apoptosis.30 Exosomal surface CD39 and CD73 have also been shown to impair T-cell function by promoting adenosine production.31 Membrane-associated TGF-β1 on EVs derived from acute myeloid leukemia (AML) lymphoblasts is responsible for suppressing NK cells function by down-regulation of NK2GD, an NK-cell activating receptor, via the SMAD pathway, hence reducing NK cell cytotoxicity.32 EV membrane-associated TGF-β can also modulate the expression of immune receptors on APCs, such as MHC classes I and II molecules and CD40, demonstrated when APCs are treated with melanoma-derived EVs expressing TGF-β on the surface. Another mechanism by which the expression profile of MHC molecules on APCs can be altered is by the transfer of EV surface MHC from tumor cells to APCs. Both mechanisms can shift the APCs toward an immunosuppressive state.33 All in all, differential expression of EV surface molecules changes the behavior of host immune system that can either benefit or impair the survival of tumor cells.
The role of EVs in cancer metastasis is well described by numerous studies. Their functions range from altering tumor cell phenotype and acquiring more malignant and prometastatic features, to determining organotropic migration, to altering distant niche in favor of metastasis by primary tumor cells.3
Surface molecules on EVs play a significant part in mediating tumor cell metastasis. Integrins, for example, can direct cancer cells to secondary organs by specifically expressing different subtypes on the surface of EVs. TEVs expressing α6β4 integrin and α6β1 integrin favor metastasis to lungs whereas those expressing αvβ5 integrin are liver tropic,34 suggesting that specific EV surface molecule arrangement is essential for determining the site of metastasis by tumor cells. In addition to directing tumor cells to different metastatic sites, integrins also play a large role in influencing tumor cell invasiveness. It has been shown that α4 integrins on the surface of EVs derived from prostate cancer cell lines are able to increase tumor cell adhesion to vitronectin via AKT-dependent pathway, as well as enhancing its invasive capability. Indeed, the degree of α4 integrin expression on EVs positively correlates with a higher Gleason score in human prostate cancer samples, suggesting that integrins can determine tumor aggressiveness.31 Fibronectin, captured by heparan sulfate proteoglycans on the surface of multiple myeloma (MM)-derived exosomes, is able to bind to heparan sulfate on the surface of endothelial cells and promote endothelial cell invasion.35 Thus, surface molecules on EVs are able to determine the invasiveness of the tumor. The influence of EV surface molecules on cancer stroma is also to be noted, as it can provide a tumor microenvironment in favor of tumor cell transitioning to metastasis.36 It has been found that betaglycan-associated TGF-β1 on the surface of TEVs generates a tumor-promoting environment by triggering the differentiation from fibroblasts to myofibroblasts (of which cancer-associated fibroblasts is a subtype),37,38 which supports tumor metastasis.39,40 Membrane-associated TGF-β on EVs secreted by osteosarcoma cells can also stimulate IL-6 secretion by MSCs, generating a proinflammatory and ultimately prometastatic phenotype for lung metastasis, a common site for distant metastasis in osteosarcoma patients.41 Other molecules may also be involved, including epidermal growth factor receptor (EGFR), though its functional significance still warrants further exploration.42 Overall, surface molecules are important players in promoting cancer metastasis to distant sites.
Several studies have illustrated EVs as part of the mechanistic pathway in drug resistance in cancer cells, which can cause relapse, distant metastasis, and an overall reduction in survival rate among cancer patients.43 One of the mechanisms that can be employed is that EVs can express decoy molecules on their surface, allowing cancer drugs to bind to EVs instead of their target tumor cells. An example of this can been seen in breast cancer, in which Her2+ tumor cells can secrete exosomes containing surface Her2, which trastuzumab can bind to, essentially disabling the drug.31 Another one of the most widely observed mechanism for multidrug resistance (MDR) is the overexpression of P-glycoprotein (P-gp), a plasma membrane efflux transporter encoded by the MDR1 gene.44 It has been shown that the expression of P-gp is not limited to plasma membrane but also on EV surface as well. Secreted by drug-resistant cancer cells, EVs carrying P-gp on their surface can translocate P-gp to the plasma membrane, thus promoting the development of drug resistance in previously drug-sensitive cancer cells.45–47
Therefore, EVs are able to confer chemoresistance in cancer cells, eventually leading to a poor prognosis in cancer patients.
Given the role of EVs in multiple aspects of cancer progression, it is imperative that biomarkers both on and inside EVs are identified. Multiple specific surface protein biomarkers are identified in various types of cancer. Using human colorectal cancer (CRC) cell line LIM1215, more than 10 surface proteins are identified as specific markers, including EGFR and claudins.48
Fibronectin on circulating EVs in breast cancer patients has also been identified as a possible biomarker.49 Immunoglobulin B-cell receptor on the surface of MM tumor-derived exosomes (TDEs) can be detected by FITC-conjugated idiotype-binding peptides, opening up the possibility of early detection of TDEs shed by MM cells and early clinical evaluation of disease progression.50 Exosomal surface markers can also be used to predict survival rate. In nonsmall cell lung cancer (NSCLC), for example, membrane-bound EGFR is an established prognostic marker and is strongly associated with the overall survival of the patients.51 Whereas many of the identified surface biomarkers are proteins, other molecules can also function as such. Glypican-1, a surface proteoglycan enriched on TEVs, can potentially be used as a screening marker for detecting early pancreatic adenocarcinoma,52 notorious for its aggressiveness and poor prognosis.53 Another study using phospholipid MALDI-MS analysis identified over 35 differentially regulated lipids on the surface of EVs that can potentially be used to identify gefitinib resistance in NSCLC, a tyrosine-kinase inhibitor that can be used to target NSCLC cells with EGFR overexpression,54 opening up the possibility of using surface biomarkers for evaluating drug resistance.
3.2 Autoimmune diseases
Previous research has highlighted the antigen presenting ability of EVs. Because they are able to carry MHC class I and II molecules of the APC of origin, they can potentially activate T cells directly by presenting antigens on the surface of EV, though EV-mediated activation of T cells is less effective.55 In patients with autoimmune diseases, autoantigens characteristic of said disease can also be displayed on the surface of EVs.56 Fibrinogen and vimentin, among a few of the known autoantigens in rheumatoid arthritis (RA), have been found to be displayed on the surface of EVs in the synovial fluid, which can form immune complexes (ICs) that can trigger inflammatory response when citrullinated. These ICs on EV surface can, for example, trigger leukotriene secretion by neutrophils57 or stimulate chemotaxis.58 Altered levels of complements on the surface of EVs are also associated with the pathology of autoimmune diseases such as systemic lupus erythematosus (SLE). SLE patients are found to have lower levels of surface C3b and C3bi fragments on EVs, which are known binding targets for opsonization and subsequent phagocytosis. It has been proposed that diminished C3b and C3bi could be linked to reduced phagocytosis of EVs. Reduction in the clearance of complements is an important mechanism in SLE that can prolong complement activation and systemic chronic inflammation.57,59 Immunoglobulin expression on EVs, particularly IgG, is also associated with a higher level of anti-dsDNA in SLE patients’ plasma and a higher disease activity.60 In particular, platelet-derived EVs opsonized with IgG from these patients stimulates production of proinflammatory cytokines by monocytes. By contrast, the presence of surface IgMs on the EVs derived from SLE patients’ plasma is negatively correlated with SLE disease activity.61 Surface cytokines from patients’ EVs are also able to modify the behavior of immune cells in favor of prolonged inflammation. A membranous form of TNF-α is found on synovial fluid-derived EVs from RA patients, which leads to sustained proliferation of T cells and resistance to apoptosis.62 All in all, the surface of EVs in patients with autoimmunity displays unique chemical signatures that are able to interact with the immune system and the cells within, and contribute to the pathogenesis of various autoimmune diseases.
It is presumed that the contents and surface structure of EVs are distinctive depending on the cell of origin and the nature of the disease.2 On top of that, the lack of good biomarkers for some autoimmune diseases such as multiple sclerosis prompts the search for them in EVs.63 Numerous studies have identified a number of proteins, mRNAs, miRNAs, and lipids in EVs that are potential candidates for diagnostic and prognostic markers, of which a few are surface molecules, including PS. PS-EVs are associated with SLE features compared to PS + EVs, though they might not be directly associated with disease activity.64 PS might also be a potential biomarker for indication of microvascular complications and coagulation in type I diabetes mellitus.65 However, further research on identifying EV surface biomarkers for autoimmune diseases is warranted as there are currently few studies on this topic.
3.3 Coagulopathy-associated diseases
Platelet-derived EVs are highly procoagulant, largely due to the presence of PS and tissue factor (TF) on EV surface.66,67 PS is highly anionic, and is able to interact with cationic γ-carboxyglutamic acid (GLA) domains in clotting proteins FVII, FIX, FX, and prothrombin, subsequently promoting recruitment of other coagulation factors. TF can bind to FVII/FVIIa and initiate coagulation.68 In healthy individuals, it has been speculated that the TF on circulating platelet-derived EVs is either nonfunctional, or has very low activity in generating thrombin.67 Both PS and TF are important players in coagulation and cardiometabolic diseases by promoting aberrant coagulation and thrombus formation. TF + EVs are associated with cancer-associated venous thromboembolism (VTE) in multiple types of cancer, in which cancer patients (with or without VTE) are found to have a higher level of TF + EVs in plasma, and are associated with lower survival rates.69–71 TF can therefore be considered for clinical biomarkers for assessing the risk of cancer-associated VTE,67 though other studies found no correlation between the level of TF + EV and future VTE in cancer patients.72 TF is also a candidate for biomarkers for other diseases associated with coagulopathy, including atherosclerosis,73 type II diabetes mellitus (T2D),74,75 hereditary thrombophilia,68 and antiphospholipid syndrome.76 The role of PS in platelet activation is also indicated in patients with atherothrombosis.74 In addition to TF and PS, other surface molecules are also highlighted for their potential pathogenic significance or as potential biomarkers. Thrombus formation is highly dependent on GPIIb/GPIIIa, a fibrinogen receptor. EV surface GPIIIa is reduced after treatment with pravastatin, a drug that has been shown to reduce platelet aggregation,77,78 suggesting a possible pathogenic role in thrombosis for GPIIb/GPIIIa in T2D patients, though its definitive functional significance requires further confirmation. Protein disulfide isomerase (PDI), expressed on the surface of EVs, also contributes to platelet aggregation in T2D patients.79 EV-associated P-selectin (CD62P) may also be involved in atherothrombotic diseases.80 CD62P on thrombi contributes to accumulation of fibrin, accumulation of inflammatory cells, and recruitment of TF-bearing EVs, all of which can lead to subsequent thrombus formation.81 Whether CD62P on the surface of EV serves the same functions requires further investigation. Endothelium-derived EVs can also carry surface components that cause aberrant thrombosis. In thrombotic thrombocytopenic purpura, endothelium-derived EVs are found to express surface von Willebrand factor (vWF) and E-selectin (CD62E), which may enhance platelet aggregation and the stability of the platelet aggregates.82,83
Overall, it has been shown that molecular components essential for platelet aggregation, thrombin formation, and hemostasis can be found on the surface of EVs, and may exert functional effects in various coagulopathy-related diseases.
4 The Therapeutic Significance of EV Surface Molecules
EVs are membrane-bound cargos that can display a unique lipid, protein, and carbohydrate signature. These surface components contribute to EV uptake by recipient cells, interaction within the host immune system, and the pathogenesis of many diseases. Therapeutic interventions involving specifically EV surface molecules can be centered around two potential strategies, as described in the next two sections.
4.1 Blocking surface components on EVs involved in uptake and pathogenesis
Several pathways have been proposed with regards to the uptake of EVs, though the detailed mechanisms are still not completely understood. Nevertheless, there has been an interest in blocking EV uptake by recipient cells by blocking the surface components involved to prevent the delivery of key signaling molecules in various diseases. PS blocking by diannexin has been proposed as a potential way to achieve uptake inhibition. A reduction in size of human glioma xenograft in mice is observed after treatment with diannexin, suggesting a potential clinical application by EV uptake inhibition.84 However, PS has its own physiologic functions, and inhibiting EV surface PS might not be a specific method for EV uptake inhibition in vivo. For example, PS acts as “eat-me” signals on the surface of apoptotic cells for macrophages and PS-bearing exosomes contribute to anti-inflammatory functions during the clearance of apoptotic cells.85 Blocking PS can potentially interfere with normal apoptosis. An in-depth understanding of the pathways involved in EV uptake is required to identify more specific molecular targets for uptake inhibition.
Blocking specific signaling molecules on the surface involved in pathogenic mechanisms might also be therapeutically significant. For example, tumor size reduction is seen when monoclonal antibody against EV surface FasL is used in a melanoma model.86 However, like in the case of PS inhibition, blocking signaling molecules on EV surface in vivo might not be specific enough as they are likely involved in normal biologic process and cellular functions. Further research is warranted in order to figure out strategies that can target signaling molecules on EVs from a specific population of cells and minimize off-target effects.
4.2 Engineering the surface of EVs
Modifications of surface components on EVs are still at an infancy stage. However, some attempts have been made to increase target specificity of the engineered EVs. One of the earlier successful techniques used is termed “exosome display,” in which specific proteins can be artificially expressed on the surface of EVs. Lactadherin, with an EGF domain and a C1C2 domain that can target exosomes, is used for incorporation of soluble proteins. The EGF domain is replaced with the desired protein and transfected to EV producing cell lines, thus allowing the expression of the chimeric constructs on EV surface.87
Another method involves transfecting plasmid encoding for the desired protein or protein constructs with the target protein and proteins found abundantly on the surface of EVs to the EV producing cell lines.
This has been done successfully with the central nervous system specific rabies viral glycoprotein (which binds to acetylcholine receptor) and murine Lamp2b found abundantly on exosomal surface.
However, the proteins might not necessarily be expressed on the surface of EV or even on the plasma membrane of the cell lines used.88,89 Ex vivo fusing of genetically modified exosomes with liposomes displaying a specific surface protein using repeated freeze-thaw method has also been reported to produce hybrid exosomes expressing the target protein and modestly increase their uptake.89 Other methods include using copper-catalyzed azide alkyne cycloaddition (click chemistry) to conjugate molecules onto the surface of exosomes with preservation of sizes of the exosomes and their ability to adhere to and be internalized by recipient cells,90 which may pave the way for conjugating specific ligands of choosing on the surface of EVs with minimal disturbance to the EV’s functionality. Another study describes deploying a “cloaking” method by applying the conjugation of streptavidin and modified glycerol-phospholipid-PEG conjugates on cardiosphere-derived EVs (CDC-EVs), which can be used as an anchoring platform to decorate EV surface with antibodies and other proteins for targeted delivery.91
Antibodies, with a highly specific antigen binding activity, are prime candidates for targeted delivery of therapeutic products.92 Earlier studies have already demonstrated the ability of specific antibody kappa light chains on the surface of exosome-like particles binding to antigens on the surface of T cells, facilitating the delivery of miRNA-150 as part of the EV cargo, thereby inhibiting T-cell mediated contact sensitivity in mice, opening up the possibility of modifying EV surface antibody composition to achieve targeted molecule delivery for cancer or autoimmune disease treatment.93
Custom-made EVs may prove to be an important asset in the development of pharmaceutic agents. One study has attempted generating exosomes displaying carcinoembryonic antigen and Her2 and found an enhanced T- and B-cell response in animals vaccinated with these exosomes, suggesting that recombinant EVs may be materials for antitumor vaccines by presenting desired antigens on their surface.74 Another study has attempted to use exosomes derived from T cells genetically engineered to express chimeric antigen receptors (CAR-T cells), a cell type rapidly emerging as immunotherapy against tumors, as direct immunotherapeutic agents against multiple tumor cell lines in vivo, which has shown potent antitumor effect in mouse xenograft models. These exosomes express CAR like the T cells they are derived from, which allowed targeted binding to tumor cells.94 It may also open up the possibility of tissue-specific drug delivery system by inserting specific proteins on EV cargos. Promising attempts have been made by creating a doxorubicin-delivering platform using exosomes derived from immature dendritic cells. Engineered to express Lamp2b fused with αv-integrin specific iRGD peptide, these exosomes can target specifically αv-integrin expressing tumor cells. Doxorubicin delivered with this method can achieve pronounced suppression of tumor growth in vivo, with less cardiotoxicity than free doxorubicin delivery.95 The system using surface Lamp2b as part of the delivery platform can potentially be modified to target any population of tumor cells by associating a specific ligand to Lamp2b and loading a desired drug into the Lamp2b expressing EVs; for example, delivering imatinib to IL3 receptor-overexpressing chronic myeloid leukemia cells by engineering Lamp2b-IL3 expressing EVs.96 Targeted drug delivery using EVs may improve on current targeted therapy in cancer patients and allowing prophylaxis against metastasis by exploiting the role of EVs in organotropism during metastasis.
5 Concluding Remarks
Several studies have highlighted the functional significance of EV surface molecules in EV trafficking and uptake, in various pathologic processes and immunologic modulation, as well as in therapeutics. However, these studies are relatively few in number and are scattered across many different fields in medicine. One of the major setbacks in the research of EV surface molecules might lie in technological limitations. Flow cytometry, for example, is one of the most widely used method to detect EV surface proteins, but it is limited by the small sizes of EVs (exosomes particularly, as they are the smallest among EVs).97 Newer methods involving the usage of microchips for EV array are being developed for multiplexed analysis of EV surface protein markers, which can expose the EVs to a panel of different protein markers, though currently only tetraspanins are relatively well tested.69 A thorough evaluation of the use of microchips is needed in order for them to be widely used for detection of EV surface biomarkers with high sensitivity. Development in quantitative analysis for molecules other than proteins should also be encouraged as most of the surface characterizations concern proteins only. The role of EV surface molecules in the context nonpathologic conditions is also another void in our understanding of EVs and further research on how physiological functions are influenced by EVs is warranted. Given that clinical trials on EVs is already under way,74 exploiting EV surface molecules for the development of therapeutic agents is also an exciting research direction for clinical usage.
Abbreviations
- ADSCs
adipose tissue-derived mesenchymal stem cells
- AML
acute myeloid leukemia
- APCs
antigen-presenting cells
- AS
BSp73AS cell line
- autoAg
autoantigen
- CAR-T cells
chimeric antigen receptor expressing T-cells
- CDC-EVs
cardiosphere-derived EVs
- CRC
colorectal cancer
- EGFR
epidermal growth factor receptor
- EV
extracellular vesicles
- FasL
Fas ligand
- GLA
γ-carboxyglutamic acid
- HA
hyaluronic acid
- HDL
high-density lipoproteins
- HSP
heat shock protein
- ICs
immune complexes
- Ig-BCR
immunoglobulin B-cell receptor
- IgG
immunoglobulin G
- MDR
multidrug resistance
- miRNA
microRNA
- MM
multiple myeloma
- MSCs
mesenchymal stem cells
- NSCLC
nonsmall cell lung cancer
- P-gp
P-glycoprotein
- PDI
protein disulfide isomerase
- PS
phosphatidylserine
- RA
rheumatoid arthritis
- SLE
systemic lupus erythematosus
- SR-B1
scavenger receptor type B-1
- T1DM
type I diabetes mellitus
- T2D
type II diabetes mellitus
- TDEs
tumor-derived exosomes
- TEVs
tumor-derived EVs
- TF
tissue factor
- Tspan8
tetraspanin 8
- vWF
von Willebrand factor
- VTE
venous thromboembolism
Acknowledgments
We are grateful for Laalaa H.T. Law’s advice on the review draft prior to the first submission. The research work was supported by Ho Yuk Ching (Ching Ping) Research Fund for Molecular Chinese Medicine Laboratory to J.C.B.L.
Authorship
M.K.N.L. and K.C.K.L. formulated the idea and prepared the manuscript. J.C.B.L. initiated, formulated the idea, and revised the manuscript. C.S. Chim and Godfrey C.F. Chan have initiated the idea. All authors read, revised, and approved the final manuscript.
Disclosures
The authors declare no conflicts of interest.
References