-
PDF
- Split View
-
Views
-
Cite
Cite
Soraya Mezouar, Jean-Louis Mege, Changing the paradigm of IFN-γ at the interface between innate and adaptive immunity: Macrophage-derived IFN-γ, Journal of Leukocyte Biology, Volume 108, Issue 1, Jul 2020, Pages 419–426, https://doi.org/10.1002/JLB.4MIR0420-619RR
- Share Icon Share
Abstract
IFN-γ plays a critical role in the immune response to bacterial infections. It is established that IFN-γ is mainly produced by NK/ILC1 cells and T cells, and most of papers have rejected the biologic reality of alternative sources for more than 20 years. Here, we are proposing to revisit this dogma and discuss the role of macrophage-derived IFN-γ in bacterial infections. Our hypothesis is based on a panel of publications and is recently revived by our results on placenta, a chimeric organ in which the immune response is tailored to protect the fetus from mother’s immune response. The culture of purified placental macrophages is associated with a production of IFN-γ that may contribute to fetal protection from bacterial infections before eliciting a Th1-like immune response potentially pathogenic for pregnancy. Hence, macrophage IFN-γ may be a novel actor of early crosstalk between innate and adaptive immunity in the context of host defense against bacterial infections.
Introduction
IFN-γ is a canonical type 2 cytokine involved in host protection against pathogenic intracellular microorganisms and in postinfectious immunopathology.1 It activates macrophages leading to the killing of intracellular pathogens and skews immune responses toward Th1.2 In the presence of pathogenic organisms, or even in their absence, IFN-γ is able to induce an activation state of macrophages called M1 polarization, which has been recategorized according to the ligand.4 The description of IFN-γ-pathway deficiencies in humans, as well as the use of IFN-γ defective mice strengthened the understanding of its pivotal role in host defense.2 It is written in all immunology textbooks that IFN-γ is produced by a restricted number of cells including NK cells/ILC1 and T lymphocytes in contrast to type I and type III interferons. This dogma omits the papers published in the 1970s that showed that macrophages could be a source of IFN-γ.3 These publications suggest that the production of IFN-γ by macrophages may be induced by IL-12 and IL-18 (known for their major role in IFN-γ production by the lymphoid compartment), but also microbial compounds or pathogens.3–22 Unfortunately, the enthusiasm of initial papers waned when a study reported that IFN-γ was not detected at the protein level in highly purified macrophages or in NK, T-, and B-cells deficient mice.14
The recent finding that large amounts of IFN-γ are secreted by placental macrophages stimulated by Coxiella burnetii, an intracellular bacterium responsible of Q fever,17 has rekindled the debate on the meaning of macrophage-derived IFN-γ. In this review, we summarize the findings describing IFN-γ production by highly purified macrophages and the potential role of macrophage-derived IFN-γ in host defense against infections. Finally, we consider the positioning of macrophage-derived IFN-γ at the frontier between innate and adaptive immunity during infections.
IFN-γ production by macrophages: artifact or reality?
The question of IFN-γ production by macrophages is that of the purity of the considered populations. Indeed, the studies that investigated IFN-γ production by macrophages used classical isolation methods but not sophisticated approaches such as FACS sorting or single cell methods. This is largely due to the results of Schleicher et al.14 published in 2005 that attributed the source of macrophage IFN-γ to a minute number of contaminating lymphoid cells. This study showed that murine macrophages expressing CD11b+ and F4/80+ did not produce IFN-γ after stimulation by IL-12 and IL-18. The authors also reported that highly purified macrophages did not secrete IFN-γ and did not stimulate nuclear translocation of STAT4. These results were apparently reinforced when using Rag2−/−γc−/− mice, which do not contain official IFN-γ cells. Hence, bone marrow-derived macrophage (BMDMs) from Rag2−/−γc−/− mice do not secrete IFN-γ and are unable to activate STAT4. However, IFN-γ mRNA expression was found in response to IL-12, IL-18, or IL-12 plus IL-1814. This latter finding did not rule out the existence of an autocrine loop for IFN-γ, but suggested an unknown translational control of IFN-γ production. This paper was preceded by that of Bancroft et al.,23 in which SCID mice were able to express activated macrophages once infected with Listeria monocytogenes in an IFN-γ-dependent manner. The conclusion of this paper was that NK cells produce IFN-γ and macrophages produce TNF to activate the lymphoid compartment. The hypothesis according to which macrophages contribute to the production of IFN-γ and the activation of macrophages in an autocrine manner was not proposed. It is noteworthy that the study of Schleicher et al.13 only focused on the production of IFN-γ protein in response to IL-12 and IL-18 but did not investigate the response to other macrophage activators such as microbes or microbial products. In addition, the authors referred to the purity of immune cell populations, a questionable notion because immune cell populations including macrophages are extremely heterogeneous. Following the study of Schleicher et al.13 macrophage-derived IFN-γ was considered an artifact by the major part of the scientific community.
Is there a spontaneous production of IFN-γ by macrophage?
Forty years ago, Neuman and Sorg3 reported for the first time that murine BMDMs and splenic macrophages exposed to supernatants of mitogen-activated T-cells produced an acid-labile form of type II IFN. Few years later, it was found that peritoneal macrophages produced acid-labile IFN recognized by antibodies directed against IFN-α/β.24 Today, we know that this acid-labile form of IFN is in fact IFN-γ. These early studies paved the way for further investigations of the dogma that IFN-γ is produced by NK cells/ILC1 and T lymphocytes.
Several studies investigated the production of IFN-γ at both transcriptional and protein levels by murine and human macrophages. As the study of Schleicher et al.13 underscored the purity of macrophage as critical for the evaluation of IFN-γ production, we focused on studies using a purity criterion of 95–99%. Most of these studies revealed that macrophages produced IFN-γ even in the absence of agonist (Table 1). At the transcriptomic level, peritoneal macrophages and BMDMs expressed IFN-γ in the absence of stimulation.4,5,7–9 Concerning alveolar macrophages, Fenton et al.22 found that alveolar macrophages did not express IFN-γ mRNA, but they seemed to secrete IFN-γ protein.7,22 These results suggest that extracellular IFN-γ is internalized by macrophages and is recycled to be secondarily released. This may cause misinterpretation of IFN-γ detection in macrophages. Once released, it is likely that minute amounts of macrophage-derived IFN-γ prime macrophages for secondary full activation.
Macrophage (species) . | Purity/phenotype . | Stimuli . | IFN-γ production (techniques) . | References . |
---|---|---|---|---|
Peritoneal exudate (mice) | >95% F4/80+ | None IFN-γ IFN-γ + LPS | (−) mRNA (FISH) (+) mRNA (RNA-PCR, FISH) (+) protein level (ELISA) | 5 |
>95% F4/80+ | None IL-12 | (−) protein level (ELISA) (+) intracellular protein (IF) (+) mRNA (RNA-PCR) (+) protein level (ELISA) (+) intracellular protein (IF) | 6 | |
>95% F4/80+, Mac-1+ < 1% CD4+, B220+, CD8+ | None, IL-12 IL-12 + IL-18 IL-18 TNF-α ± IL-12 or IL-18 or LPS or LPS + IL-18 IL-12 + IL-18 ± IL-4 or IL-10 or IL-13 or TGF-β LPS + IL-12 | (+) mRNA (RT-PCR) (+) mRNA (RT-PCR) (+) protein level (ELISA) (−) mRNA (RT-PCR) (−) protein level (ELISA) (−) protein level (ELISA) (+) protein level (ELISA) | 88 | |
85% | None LPS | (−) mRNA (RNA-PCR) (+) mRNA (RT-PCR) after LT or NK depletion (+) intracellular protein (IF) | 4 | |
>99% F4/80+, Mac-1+ < 1% CD3+, DX-5+ | IL-12 or IL-18 or IL-12 + IL-18 | (+) mRNA (RNA-PCR) (+) protein level (ELISA) | 18 | |
99% CD68+ | IL-12 + IL-18 | (−) mRNA (RNA-PCR) (−) protein level (ELISA) | 14 | |
99% F4/80+ | L. pneumoniae ± L-12 | (+) mRNA (RT-PCR) | 10 | |
98% - 99% Mac-1+ | IL-12 + IL-18 and then LPS | (+) intracellular protein (IF) | 15 | |
BMDMs (mice) | F4/80+, Mac-3+, CD14+ CD3−, CD4−, CD8−, CD19− | None C. pneumoniae | (+) mRNA (RT-PCR) (+) mRNA (RT-PCR) (+) intracellular protein (IF) (+) protein level (ELISA) | 9 |
F4/80+, Mac-1+, I-AK+, B7.1+, CD16/32+, CD3−, CD4−, CD8−, CD19−, CD5− | IL-12 + IL-18 IL-12 or IL-18 | (+) mRNA (RT-PCR, FISH) (+) protein level (ELISA) (−) protein level (ELISA) | 19 | |
>98% Mac-1+, FcγR | IL-12 + L-18 | (+) protein level (ELISA) | 16 | |
95%-98% CD11b+, F4/80+ | IL-12 or IL-18 or IL-12 + IL-18 LPS | (−) mRNA (RNA-PCR) (-) protein level (ELISA) (+) mRNA (RT-PCR) (-) protein level (ELISA) | 14 | |
F4/80+, Mac-3+, CD14+ | C. pneumoniae | (+) mRNA in infected-wild-type cells (−) mRNA in infected-TLR4−/−, MyD88−/− or Stat−/− cells | 12 | |
Cell line (mice) | Not applicable | IL-12 or IL-18 or IL-12 + IL-18 LPS | (−) mRNA (RT-PCR) (-) protein level (ELISA) (−) mRNA (RT-PCR) | 14 |
IL-12 IL-18 | (−) protein level (ELISA) | 19 | ||
LPS | (+) mRNA (RT-PCR) | 4 | ||
Alveolar (human) | >99% esterase+ < 1% contaminating lymphocytes | None Mtb (H37Rvirulent) Mtb (H37Ravirulent) LPS or Mtb (H37R avirulent) + IL-10 Mtb (H37R avirulent) + IL-12 Mtb (H37R avirulent) + anti-IL-12 or IL-12 | (+) mRNA (RT-PCR and RT-in situ PCR) (+) protein level (ELISA) (+) protein level (ELISA) (+) protein level (ELISA) (+) mRNA (RT-PCR and RT-in situ PCR) (+) protein level (ELISA) (+) protein level (ELISA) (−) protein level (ELISA) | 22 |
>95% macrophage (from sarcoidosis patients) | None | (+) protein level (ELISA) | 20 | |
98% macrophage (from M. bovis infected mice) | None, LPS, Mtb-DPP IL-12 or LPS or live BCG IL-12 + LPS or IL-12 + live BCG IL-12 + heat-inactivated BCG or IL-12 + Mtb-DPP | (+) protein level (ELISA) for wild-type but not IL-12−/− (−) protein level (ELISA) (+) protein level (ELISA) (+) protein level (ELISA) | 7 | |
Placental (human) | CD14+ | C. burnetii | (+) protein level (ELISA) | 17 |
MDMs (human) | CD14+, CD68+ < 0.1% CD56+, CD3+ | IL-12 + IL-18 | (+) intracellular protein (ESA, IHC) | 21 |
90% CD14+ | S. typhimurium S. typhimurium + IFN-γ S. typhimurium + anti-IFN-α/β S. typhimurium + anti-IL-12 S. typhimurium + anti-IL-18 S. typhimurium + anti-IL-12 + anti-IL-18 S. typhimurium + anti-IL-12 + anti-IL-18 + anti-IFN-α/β | (+) mRNA (RNA-PCR) (+) protein level (ELISA) () protein level (ELISA) (+) protein level (ELISA) 40% compared with S. typhimurium infection (+) protein level (ELISA) 50% compared with S. typhimurium infection (+) protein level (ELISA) 30% compared with S. typhimurium infection (+) protein level (ELISA) 30% compared with S. typhimurium infection (+) protein level (ELISA) 20% compared with S. typhimurium infection | 13 |
Macrophage (species) . | Purity/phenotype . | Stimuli . | IFN-γ production (techniques) . | References . |
---|---|---|---|---|
Peritoneal exudate (mice) | >95% F4/80+ | None IFN-γ IFN-γ + LPS | (−) mRNA (FISH) (+) mRNA (RNA-PCR, FISH) (+) protein level (ELISA) | 5 |
>95% F4/80+ | None IL-12 | (−) protein level (ELISA) (+) intracellular protein (IF) (+) mRNA (RNA-PCR) (+) protein level (ELISA) (+) intracellular protein (IF) | 6 | |
>95% F4/80+, Mac-1+ < 1% CD4+, B220+, CD8+ | None, IL-12 IL-12 + IL-18 IL-18 TNF-α ± IL-12 or IL-18 or LPS or LPS + IL-18 IL-12 + IL-18 ± IL-4 or IL-10 or IL-13 or TGF-β LPS + IL-12 | (+) mRNA (RT-PCR) (+) mRNA (RT-PCR) (+) protein level (ELISA) (−) mRNA (RT-PCR) (−) protein level (ELISA) (−) protein level (ELISA) (+) protein level (ELISA) | 88 | |
85% | None LPS | (−) mRNA (RNA-PCR) (+) mRNA (RT-PCR) after LT or NK depletion (+) intracellular protein (IF) | 4 | |
>99% F4/80+, Mac-1+ < 1% CD3+, DX-5+ | IL-12 or IL-18 or IL-12 + IL-18 | (+) mRNA (RNA-PCR) (+) protein level (ELISA) | 18 | |
99% CD68+ | IL-12 + IL-18 | (−) mRNA (RNA-PCR) (−) protein level (ELISA) | 14 | |
99% F4/80+ | L. pneumoniae ± L-12 | (+) mRNA (RT-PCR) | 10 | |
98% - 99% Mac-1+ | IL-12 + IL-18 and then LPS | (+) intracellular protein (IF) | 15 | |
BMDMs (mice) | F4/80+, Mac-3+, CD14+ CD3−, CD4−, CD8−, CD19− | None C. pneumoniae | (+) mRNA (RT-PCR) (+) mRNA (RT-PCR) (+) intracellular protein (IF) (+) protein level (ELISA) | 9 |
F4/80+, Mac-1+, I-AK+, B7.1+, CD16/32+, CD3−, CD4−, CD8−, CD19−, CD5− | IL-12 + IL-18 IL-12 or IL-18 | (+) mRNA (RT-PCR, FISH) (+) protein level (ELISA) (−) protein level (ELISA) | 19 | |
>98% Mac-1+, FcγR | IL-12 + L-18 | (+) protein level (ELISA) | 16 | |
95%-98% CD11b+, F4/80+ | IL-12 or IL-18 or IL-12 + IL-18 LPS | (−) mRNA (RNA-PCR) (-) protein level (ELISA) (+) mRNA (RT-PCR) (-) protein level (ELISA) | 14 | |
F4/80+, Mac-3+, CD14+ | C. pneumoniae | (+) mRNA in infected-wild-type cells (−) mRNA in infected-TLR4−/−, MyD88−/− or Stat−/− cells | 12 | |
Cell line (mice) | Not applicable | IL-12 or IL-18 or IL-12 + IL-18 LPS | (−) mRNA (RT-PCR) (-) protein level (ELISA) (−) mRNA (RT-PCR) | 14 |
IL-12 IL-18 | (−) protein level (ELISA) | 19 | ||
LPS | (+) mRNA (RT-PCR) | 4 | ||
Alveolar (human) | >99% esterase+ < 1% contaminating lymphocytes | None Mtb (H37Rvirulent) Mtb (H37Ravirulent) LPS or Mtb (H37R avirulent) + IL-10 Mtb (H37R avirulent) + IL-12 Mtb (H37R avirulent) + anti-IL-12 or IL-12 | (+) mRNA (RT-PCR and RT-in situ PCR) (+) protein level (ELISA) (+) protein level (ELISA) (+) protein level (ELISA) (+) mRNA (RT-PCR and RT-in situ PCR) (+) protein level (ELISA) (+) protein level (ELISA) (−) protein level (ELISA) | 22 |
>95% macrophage (from sarcoidosis patients) | None | (+) protein level (ELISA) | 20 | |
98% macrophage (from M. bovis infected mice) | None, LPS, Mtb-DPP IL-12 or LPS or live BCG IL-12 + LPS or IL-12 + live BCG IL-12 + heat-inactivated BCG or IL-12 + Mtb-DPP | (+) protein level (ELISA) for wild-type but not IL-12−/− (−) protein level (ELISA) (+) protein level (ELISA) (+) protein level (ELISA) | 7 | |
Placental (human) | CD14+ | C. burnetii | (+) protein level (ELISA) | 17 |
MDMs (human) | CD14+, CD68+ < 0.1% CD56+, CD3+ | IL-12 + IL-18 | (+) intracellular protein (ESA, IHC) | 21 |
90% CD14+ | S. typhimurium S. typhimurium + IFN-γ S. typhimurium + anti-IFN-α/β S. typhimurium + anti-IL-12 S. typhimurium + anti-IL-18 S. typhimurium + anti-IL-12 + anti-IL-18 S. typhimurium + anti-IL-12 + anti-IL-18 + anti-IFN-α/β | (+) mRNA (RNA-PCR) (+) protein level (ELISA) () protein level (ELISA) (+) protein level (ELISA) 40% compared with S. typhimurium infection (+) protein level (ELISA) 50% compared with S. typhimurium infection (+) protein level (ELISA) 30% compared with S. typhimurium infection (+) protein level (ELISA) 30% compared with S. typhimurium infection (+) protein level (ELISA) 20% compared with S. typhimurium infection | 13 |
Macrophage (species) . | Purity/phenotype . | Stimuli . | IFN-γ production (techniques) . | References . |
---|---|---|---|---|
Peritoneal exudate (mice) | >95% F4/80+ | None IFN-γ IFN-γ + LPS | (−) mRNA (FISH) (+) mRNA (RNA-PCR, FISH) (+) protein level (ELISA) | 5 |
>95% F4/80+ | None IL-12 | (−) protein level (ELISA) (+) intracellular protein (IF) (+) mRNA (RNA-PCR) (+) protein level (ELISA) (+) intracellular protein (IF) | 6 | |
>95% F4/80+, Mac-1+ < 1% CD4+, B220+, CD8+ | None, IL-12 IL-12 + IL-18 IL-18 TNF-α ± IL-12 or IL-18 or LPS or LPS + IL-18 IL-12 + IL-18 ± IL-4 or IL-10 or IL-13 or TGF-β LPS + IL-12 | (+) mRNA (RT-PCR) (+) mRNA (RT-PCR) (+) protein level (ELISA) (−) mRNA (RT-PCR) (−) protein level (ELISA) (−) protein level (ELISA) (+) protein level (ELISA) | 88 | |
85% | None LPS | (−) mRNA (RNA-PCR) (+) mRNA (RT-PCR) after LT or NK depletion (+) intracellular protein (IF) | 4 | |
>99% F4/80+, Mac-1+ < 1% CD3+, DX-5+ | IL-12 or IL-18 or IL-12 + IL-18 | (+) mRNA (RNA-PCR) (+) protein level (ELISA) | 18 | |
99% CD68+ | IL-12 + IL-18 | (−) mRNA (RNA-PCR) (−) protein level (ELISA) | 14 | |
99% F4/80+ | L. pneumoniae ± L-12 | (+) mRNA (RT-PCR) | 10 | |
98% - 99% Mac-1+ | IL-12 + IL-18 and then LPS | (+) intracellular protein (IF) | 15 | |
BMDMs (mice) | F4/80+, Mac-3+, CD14+ CD3−, CD4−, CD8−, CD19− | None C. pneumoniae | (+) mRNA (RT-PCR) (+) mRNA (RT-PCR) (+) intracellular protein (IF) (+) protein level (ELISA) | 9 |
F4/80+, Mac-1+, I-AK+, B7.1+, CD16/32+, CD3−, CD4−, CD8−, CD19−, CD5− | IL-12 + IL-18 IL-12 or IL-18 | (+) mRNA (RT-PCR, FISH) (+) protein level (ELISA) (−) protein level (ELISA) | 19 | |
>98% Mac-1+, FcγR | IL-12 + L-18 | (+) protein level (ELISA) | 16 | |
95%-98% CD11b+, F4/80+ | IL-12 or IL-18 or IL-12 + IL-18 LPS | (−) mRNA (RNA-PCR) (-) protein level (ELISA) (+) mRNA (RT-PCR) (-) protein level (ELISA) | 14 | |
F4/80+, Mac-3+, CD14+ | C. pneumoniae | (+) mRNA in infected-wild-type cells (−) mRNA in infected-TLR4−/−, MyD88−/− or Stat−/− cells | 12 | |
Cell line (mice) | Not applicable | IL-12 or IL-18 or IL-12 + IL-18 LPS | (−) mRNA (RT-PCR) (-) protein level (ELISA) (−) mRNA (RT-PCR) | 14 |
IL-12 IL-18 | (−) protein level (ELISA) | 19 | ||
LPS | (+) mRNA (RT-PCR) | 4 | ||
Alveolar (human) | >99% esterase+ < 1% contaminating lymphocytes | None Mtb (H37Rvirulent) Mtb (H37Ravirulent) LPS or Mtb (H37R avirulent) + IL-10 Mtb (H37R avirulent) + IL-12 Mtb (H37R avirulent) + anti-IL-12 or IL-12 | (+) mRNA (RT-PCR and RT-in situ PCR) (+) protein level (ELISA) (+) protein level (ELISA) (+) protein level (ELISA) (+) mRNA (RT-PCR and RT-in situ PCR) (+) protein level (ELISA) (+) protein level (ELISA) (−) protein level (ELISA) | 22 |
>95% macrophage (from sarcoidosis patients) | None | (+) protein level (ELISA) | 20 | |
98% macrophage (from M. bovis infected mice) | None, LPS, Mtb-DPP IL-12 or LPS or live BCG IL-12 + LPS or IL-12 + live BCG IL-12 + heat-inactivated BCG or IL-12 + Mtb-DPP | (+) protein level (ELISA) for wild-type but not IL-12−/− (−) protein level (ELISA) (+) protein level (ELISA) (+) protein level (ELISA) | 7 | |
Placental (human) | CD14+ | C. burnetii | (+) protein level (ELISA) | 17 |
MDMs (human) | CD14+, CD68+ < 0.1% CD56+, CD3+ | IL-12 + IL-18 | (+) intracellular protein (ESA, IHC) | 21 |
90% CD14+ | S. typhimurium S. typhimurium + IFN-γ S. typhimurium + anti-IFN-α/β S. typhimurium + anti-IL-12 S. typhimurium + anti-IL-18 S. typhimurium + anti-IL-12 + anti-IL-18 S. typhimurium + anti-IL-12 + anti-IL-18 + anti-IFN-α/β | (+) mRNA (RNA-PCR) (+) protein level (ELISA) () protein level (ELISA) (+) protein level (ELISA) 40% compared with S. typhimurium infection (+) protein level (ELISA) 50% compared with S. typhimurium infection (+) protein level (ELISA) 30% compared with S. typhimurium infection (+) protein level (ELISA) 30% compared with S. typhimurium infection (+) protein level (ELISA) 20% compared with S. typhimurium infection | 13 |
Macrophage (species) . | Purity/phenotype . | Stimuli . | IFN-γ production (techniques) . | References . |
---|---|---|---|---|
Peritoneal exudate (mice) | >95% F4/80+ | None IFN-γ IFN-γ + LPS | (−) mRNA (FISH) (+) mRNA (RNA-PCR, FISH) (+) protein level (ELISA) | 5 |
>95% F4/80+ | None IL-12 | (−) protein level (ELISA) (+) intracellular protein (IF) (+) mRNA (RNA-PCR) (+) protein level (ELISA) (+) intracellular protein (IF) | 6 | |
>95% F4/80+, Mac-1+ < 1% CD4+, B220+, CD8+ | None, IL-12 IL-12 + IL-18 IL-18 TNF-α ± IL-12 or IL-18 or LPS or LPS + IL-18 IL-12 + IL-18 ± IL-4 or IL-10 or IL-13 or TGF-β LPS + IL-12 | (+) mRNA (RT-PCR) (+) mRNA (RT-PCR) (+) protein level (ELISA) (−) mRNA (RT-PCR) (−) protein level (ELISA) (−) protein level (ELISA) (+) protein level (ELISA) | 88 | |
85% | None LPS | (−) mRNA (RNA-PCR) (+) mRNA (RT-PCR) after LT or NK depletion (+) intracellular protein (IF) | 4 | |
>99% F4/80+, Mac-1+ < 1% CD3+, DX-5+ | IL-12 or IL-18 or IL-12 + IL-18 | (+) mRNA (RNA-PCR) (+) protein level (ELISA) | 18 | |
99% CD68+ | IL-12 + IL-18 | (−) mRNA (RNA-PCR) (−) protein level (ELISA) | 14 | |
99% F4/80+ | L. pneumoniae ± L-12 | (+) mRNA (RT-PCR) | 10 | |
98% - 99% Mac-1+ | IL-12 + IL-18 and then LPS | (+) intracellular protein (IF) | 15 | |
BMDMs (mice) | F4/80+, Mac-3+, CD14+ CD3−, CD4−, CD8−, CD19− | None C. pneumoniae | (+) mRNA (RT-PCR) (+) mRNA (RT-PCR) (+) intracellular protein (IF) (+) protein level (ELISA) | 9 |
F4/80+, Mac-1+, I-AK+, B7.1+, CD16/32+, CD3−, CD4−, CD8−, CD19−, CD5− | IL-12 + IL-18 IL-12 or IL-18 | (+) mRNA (RT-PCR, FISH) (+) protein level (ELISA) (−) protein level (ELISA) | 19 | |
>98% Mac-1+, FcγR | IL-12 + L-18 | (+) protein level (ELISA) | 16 | |
95%-98% CD11b+, F4/80+ | IL-12 or IL-18 or IL-12 + IL-18 LPS | (−) mRNA (RNA-PCR) (-) protein level (ELISA) (+) mRNA (RT-PCR) (-) protein level (ELISA) | 14 | |
F4/80+, Mac-3+, CD14+ | C. pneumoniae | (+) mRNA in infected-wild-type cells (−) mRNA in infected-TLR4−/−, MyD88−/− or Stat−/− cells | 12 | |
Cell line (mice) | Not applicable | IL-12 or IL-18 or IL-12 + IL-18 LPS | (−) mRNA (RT-PCR) (-) protein level (ELISA) (−) mRNA (RT-PCR) | 14 |
IL-12 IL-18 | (−) protein level (ELISA) | 19 | ||
LPS | (+) mRNA (RT-PCR) | 4 | ||
Alveolar (human) | >99% esterase+ < 1% contaminating lymphocytes | None Mtb (H37Rvirulent) Mtb (H37Ravirulent) LPS or Mtb (H37R avirulent) + IL-10 Mtb (H37R avirulent) + IL-12 Mtb (H37R avirulent) + anti-IL-12 or IL-12 | (+) mRNA (RT-PCR and RT-in situ PCR) (+) protein level (ELISA) (+) protein level (ELISA) (+) protein level (ELISA) (+) mRNA (RT-PCR and RT-in situ PCR) (+) protein level (ELISA) (+) protein level (ELISA) (−) protein level (ELISA) | 22 |
>95% macrophage (from sarcoidosis patients) | None | (+) protein level (ELISA) | 20 | |
98% macrophage (from M. bovis infected mice) | None, LPS, Mtb-DPP IL-12 or LPS or live BCG IL-12 + LPS or IL-12 + live BCG IL-12 + heat-inactivated BCG or IL-12 + Mtb-DPP | (+) protein level (ELISA) for wild-type but not IL-12−/− (−) protein level (ELISA) (+) protein level (ELISA) (+) protein level (ELISA) | 7 | |
Placental (human) | CD14+ | C. burnetii | (+) protein level (ELISA) | 17 |
MDMs (human) | CD14+, CD68+ < 0.1% CD56+, CD3+ | IL-12 + IL-18 | (+) intracellular protein (ESA, IHC) | 21 |
90% CD14+ | S. typhimurium S. typhimurium + IFN-γ S. typhimurium + anti-IFN-α/β S. typhimurium + anti-IL-12 S. typhimurium + anti-IL-18 S. typhimurium + anti-IL-12 + anti-IL-18 S. typhimurium + anti-IL-12 + anti-IL-18 + anti-IFN-α/β | (+) mRNA (RNA-PCR) (+) protein level (ELISA) () protein level (ELISA) (+) protein level (ELISA) 40% compared with S. typhimurium infection (+) protein level (ELISA) 50% compared with S. typhimurium infection (+) protein level (ELISA) 30% compared with S. typhimurium infection (+) protein level (ELISA) 30% compared with S. typhimurium infection (+) protein level (ELISA) 20% compared with S. typhimurium infection | 13 |
Does macrophage activation lead to IFN-γ production?
During infection, macrophages secrete IL-12 and IL-18 that activate NK cells and T cells to produce IFN-γ (see Graphical Abstract). In turn, IFN-γ activates macrophages and elicits their polarization into M1 macrophages. As murine and human macrophages express IL-12 and IL-18 receptors,25 we checked whether macrophage stimulation with IL-12 and/or IL-18 led to IFN-γ production. Table 1 summarizes studies providing data on the purity of macrophage populations. Several studies showed that addition of IL-12 and IL-18 to macrophages led to IFN-γ production in a synergistic manner (see above). Although this was clearly identified in primary cells from mice6,8,10,15,16,18,19 or humans,7,21 it was not confirmed in murine cell lines.14,19 Other cytokines were shown to alter macrophage production of IFN-γ. Addition of M-CSF, known to impact macrophage maturation and elicit a M2-like profile, to IL-12 and IL-18 resulted in macrophage IFN-γ production.21 Similar results were obtained when macrophages were exposed to an IFN-α/β treatment.13 Minute amounts of IFN-γ also exert an autocrine effect on murine peritoneal macrophages, as shown at the transcriptional and protein levels.5 So far, macrophage polarization following IL-12, IL-18, or M-CSF treatment has not been studied; we speculate that new categories of polarization could be uncovered.
Microbial products, which are strong M1-type activators of macrophages, may also induce IFN-γ production by macrophages (Table 1). LPS-induced IFN-γ mRNA and protein expression was demonstrated in macrophages,4,5,7,14,15 albeit there are some discordant results. For example, although IFN-γ mRNA was expressed in peritoneal macrophages4 and BMDMs14, it was not always found in mouse cell lines. The immaturity of macrophage cell lines may account for their inability to produce IFN-γ. The secretion of IFN-γ is also a source of divergent results: it has been found in peritoneal4,5 and alveolar7 primary cells, but not in BMDMs.14 The addition of IL-12, IL-18, or their combination to macrophages may enable them to secrete IFN-γ in response to LPS (Table 1).
The ability to elicit IFN-γ expression in macrophages is not restricted to bacterial products. Bacteria with macrophage tropism, including Mycobacterium tuberculosis, Mycobacterium bovis, Chlamydia pneumoniae, Legionella pneumophila, Salmonella typhimurium, and C. burnetii, have been reported as inducers of IFN-γ in macrophages (Table 1). As discussed above for LPS, full production of IFN-γ in response to bacteria may require some form of costimulation. Alveolar macrophages from M. bovis-infected wild-type (WT) mice secreted IFN-γ when stimulated by LPS or mycobacterial products.7 Alveolar macrophages from IL-12−/− mice secreted IFN-γ when they were incubated with IL-12 and M. bovis organisms or heat-inactivated bacteria7. Anti-IL-12 antibodies inhibited Mtb-induced IFN-γ secretion in human alveolar macrophages,22 thus confirming the pivotal role of IL-12 in IFN-γ production. Similarly, IFN-γ secretion by monocyte-derived macrophages (MDMs) stimulated by S. typhimurium was decreased in the presence of antibodies directed against IFNα/β and IL-12.13 C. pneumoniae 9,12 and L. pneumophila 10 infection elicited macrophage production of IFN-γ at the transcriptional and protein levels. As opposed to bacillus Calmette-Guérin (BCG) infection,7 IFN-γ mRNA expression was abolished after heat-inactivation of C. pneumoniae,9 suggesting a connection between bacterial virulence and IFN-γ mRNA induction. Altogether, the bulk of evidence shows that IFN-γ transcripts, but not secreted bioactive IFN-γ, are present in resting and cytokine or microbe-activated macrophages.
Which is the signaling pathway for IFN-γ production by macrophages?
The Fig. 1 summarizes current knowledge on macrophage signaling pathways for IFN-γ production. First, IL-12 and IL-18 activate STAT4 in mouse peritoneal macrophages, reminiscent of IL-12 and IL-18 signaling in NK and T cells.30–32 Both cytokines stimulate iNOS activity, but NO is not involved in IFN-γ production.33 In contrast, the NF-κB pathway seems to be required for IL-12- and IL-18-stimulated production of IFN-γ.8 Second, type I interferons induce IFN-γ production via the activation of STAT-1. This pathway is subverted by intracellular pathogens for IFN-γ. Indeed, macrophage responses to C. pneumoniae depend on TLR-4 and MyD88 since IFN-γ mRNA was not detected in C. pneumoniae-infected BMDMs in which TLR-4, MyD88 and STAT-4 had been invalidated.13 Among immunoregulatory cytokines, IL-4 activates STAT-6 which inhibits STAT-4 through direct interaction, highlighting the central role of STAT-4 in IFN-γ production by macrophages.
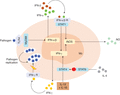
Signaling of IFN-γ autocrine/paracrine pathways in macrophages. The stimulation of STAT-4 through type I cytokines, IL-12, and IL-18 leads to IFN-γ production. Intracellular pathogens induce the production of type I IFN at the transcriptional and protein levels in a TLR-4/MyD88-dependent manner. The ligation of type I receptors also leads to the secretion of IFN-γ in a STAT-1-dependent manner, and the control of intracellular bacterial growth via the production of NO. Finally, secreted IFN-γ is sensed in an autocrine manner by macrophages permitting the set-up of their classical antimicrobial response. The regulation of IFN-γ production is under a negative control by IL-4 in a STAT-6-dependent manner
What role does macrophage-derived IFN-γ serve for antibacterial defense?
The general role of IFN-γ in host defense against bacteria is well established: its early production by NK/ILC1 cells keeps the infection in check, while its delayed release from T cells orchestrates bacterial eradication. Macrophages are both targets of NK/ILC1 and T cell-derived IFN-γ and major players of bacterial killing. The picture becomes more complex if macrophages are not only a target, but also a source of IFN-γ. We suggest that macrophage IFN-γ may provide local and systemic signals reinforcing the microbicidal response. Indeed, macrophage production of IFN-γ is associated with the control of bacterial growth.9,10,12 For example, macrophages infected with L. pneumophila,10 C. pneumoniae,12 or C. burnetii 17 exhibit IFN-γ production and antibacterial activity. The use of anti-IFN-γ antibodies or mice devoid of IFN-γ results in increased bacterial growth in infected macrophages.9,10,12 The loop involving IFN-γ and IFN-α/β is likely responsible for the microbicidal response of macrophages, as suggested by the inability of mice invalidated for TLR-4, IFN-α/β and STAT-1 to control L. pneumophila growth.9,12 These studies focused on the association between IFN-γ production by murine macrophages and effective control of bacterial infections. The example of the control of C. burnetii infection by human placental macrophages seems to extend murine findings to human cells (see below).
What place does macrophage IFN-γ hold in innate and adaptive immune responses to infections?
In the light of data reviewed above, IFN-γ should be positioned at the interface between innate and adaptive immunity during infections (Fig. 2). If macrophages produce minute amounts of IFN-γ even in the absence of stimulus, these amounts are sufficient to create a microenvironment oriented towards type 1 immune response and microbicidal activity. This context promotes the recruitment of polymorphonuclear neutrophils and dendritic cells (DCs), further sources of IFN-γ11,26,27 able to complete the autocrine loop of macrophage IFN-γ. A second wave of cell recruitment at the site of infection involves DCs and monocytes. IL-12 produced by macrophages and DCs elicits the activation of NK/ILCs and the wave of IFN-γ produced by lymphoid cells activates the microbicidal program of macrophages. Reconsidering IFN-γ production by macrophages allows a reappraisal of the early innate immune response to pathogens as driven by initial macrophage-derived IFN-γ. Delayed production of IFN-γ by macrophages in vivo could be relevant in tissues, as macrophages are prominent resident immune cells. This possibility may be encountered in placenta approaching the end of pregnancy when NK cells start to wane. In addition, we suggest that minute amounts of macrophage IFN-γ allow microbial killing without eliciting a strong Th1 immune response. So far, the analysis of macrophage polarization has focused on IFN-γ and LPS, leaving open the question of macrophage polarization in response to other agonists inducing IFN-γ in macrophages.
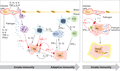
Macrophage-derived IFN-γ in the innate and adaptive responses during infections. During infection, the innate and the adaptive immunity are at least in part under the control of type I and type II IFN, respectively. The concept of IFN-γ production by macrophages is presented in red. It is based on the cooperation of neutrophils, dendritic cells, or/and macrophages. The nonlymphoid IFN-γ activates macrophages during the early phase of the innate response to infection. Resident cells, such as dendritic cells and macrophages (Mɸ) initiate the type II IFN response through the secretion of IFN-γ following their direct interaction with pathogens. Then, the release of proinflammatory cytokines and type I IFN leads to the recruitment of neutrophils (N) initiating the control of pathogen invasion (bacterial engulfment), another source of IFN-γ. Dendritic cells are also recruited at the site of infection; they are involved in the activation of NK/ILC cells and T cells via the secretion of IL-12. Activated NK/ILC cells produce IFN-γ allowing the activation of macrophages in the later phase of the innate immunity. Another scenario could be considered assuming that minute amounts of macrophage IFN-γ allow microbial killing without eliciting a strong Th1 immune response
IFN-γ production by placental macrophages
Our studies of placental macrophages strengthened our conviction that the question of macrophage-derived IFN-γ had not been fully addressed. Placental macrophages28 belong to the placental immune system, which also includes NK cells, lymphocytes, DCs,29 and mast cells.30 While the population of NK cells decreases throughout pregnancy, the number of macrophages remains pretty stable. Placental macrophages comprise decidual macrophages and Hofbauer cells according to their maternal or fetal localization and origin.29 The 2 populations are involved in placenta biology, feto-maternal tolerance and defense against infections. Their activation status varies during pregnancy from M1 (proinflammatory) to M2 (immunoregulatory) profile.28,31,32
The role of IFN-γ in pregnancy is widely demonstrated, known and accepted. IFN-γ is involved in the inhibition of the outgrowth of human trophoblast cells in vitro,31 in trophoblast apoptosis32 and invasion,33 in spiral artery remodeling34–36 and up-modulation of HLA class I expression by trophoblast cells.37 The absence of IFN-γ in mice deficient for NK cells, IFN-γ receptors or IFN-γ is associated with pregnancy complications such as defective spiral artery remodeling.38 Placental cells may be a source of IFN-γ. Indeed, IFN-γ has been found in trophoblasts,39 known to be involved in nutriment exchange and mechanical protection against infections at the maternal–fetal interface.33,40,41 Interestingly, IFN-γ is expressed in both decidual macrophages and Hofbauer cells (Table 2). We previously found that placental CD14+ macrophages from at term pregnancy over-expressed IFN-γ mRNA as compared with monocytes and MDMs in the absence of stimulation42 (gene expression omnibus, GSEE36537). In situ IFN-γ was detected at the protein level within unstimulated Hofbauer cells43 and decidual macrophages44,45 during the 3 trimesters or only the third trimester. Glucose treatment46 or infection by intracellular bacteria17 induced IFN-γ production by placental macrophages at the protein level. In contrast, their stimulation by IL-12 or IL-2 did not induce the secretion of IFN-γ during the first trimester of pregnancy.47
Macrophage population . | Purity/phenotype . | Stimuli . | IFN-γ evaluation (technique) . | Localization . | Trimester . | Refs . |
---|---|---|---|---|---|---|
Hofbauer cells | Not applicable | None | (+) intracellular protein (IHC) | Fetal | All trimesters | 43 |
CD163+ | Glucose treatment | (+) mRNA (RT-PCR) (+) protein level (ELISA) | Fetal | Ni | 46 | |
Decidual macrophages | >95% CD14+ | IL-12 or IL-2 | (−) protein level (ELISA) | Decidua | First | 47 |
86% | None | (+) protein level (ELISA) | Decidua | First | 45 | |
Not applicable | None | (+) intracellular protein (IHC) | Decidua | Third | 44 | |
Placental macrophages | >95% CD14+ | C. burnetii | (+) protein level (ELISA) | Both | Third | 17 |
Macrophage population . | Purity/phenotype . | Stimuli . | IFN-γ evaluation (technique) . | Localization . | Trimester . | Refs . |
---|---|---|---|---|---|---|
Hofbauer cells | Not applicable | None | (+) intracellular protein (IHC) | Fetal | All trimesters | 43 |
CD163+ | Glucose treatment | (+) mRNA (RT-PCR) (+) protein level (ELISA) | Fetal | Ni | 46 | |
Decidual macrophages | >95% CD14+ | IL-12 or IL-2 | (−) protein level (ELISA) | Decidua | First | 47 |
86% | None | (+) protein level (ELISA) | Decidua | First | 45 | |
Not applicable | None | (+) intracellular protein (IHC) | Decidua | Third | 44 | |
Placental macrophages | >95% CD14+ | C. burnetii | (+) protein level (ELISA) | Both | Third | 17 |
Macrophage population . | Purity/phenotype . | Stimuli . | IFN-γ evaluation (technique) . | Localization . | Trimester . | Refs . |
---|---|---|---|---|---|---|
Hofbauer cells | Not applicable | None | (+) intracellular protein (IHC) | Fetal | All trimesters | 43 |
CD163+ | Glucose treatment | (+) mRNA (RT-PCR) (+) protein level (ELISA) | Fetal | Ni | 46 | |
Decidual macrophages | >95% CD14+ | IL-12 or IL-2 | (−) protein level (ELISA) | Decidua | First | 47 |
86% | None | (+) protein level (ELISA) | Decidua | First | 45 | |
Not applicable | None | (+) intracellular protein (IHC) | Decidua | Third | 44 | |
Placental macrophages | >95% CD14+ | C. burnetii | (+) protein level (ELISA) | Both | Third | 17 |
Macrophage population . | Purity/phenotype . | Stimuli . | IFN-γ evaluation (technique) . | Localization . | Trimester . | Refs . |
---|---|---|---|---|---|---|
Hofbauer cells | Not applicable | None | (+) intracellular protein (IHC) | Fetal | All trimesters | 43 |
CD163+ | Glucose treatment | (+) mRNA (RT-PCR) (+) protein level (ELISA) | Fetal | Ni | 46 | |
Decidual macrophages | >95% CD14+ | IL-12 or IL-2 | (−) protein level (ELISA) | Decidua | First | 47 |
86% | None | (+) protein level (ELISA) | Decidua | First | 45 | |
Not applicable | None | (+) intracellular protein (IHC) | Decidua | Third | 44 | |
Placental macrophages | >95% CD14+ | C. burnetii | (+) protein level (ELISA) | Both | Third | 17 |
The investigation of pathologic pregnancies due to preeclampsia (PE) or intrauterine growth restriction (IUGR) revealed the importance of IFN-γ produced by placental macrophages. Hence, an increased production of TNF and IFN-γ by placental macrophages is associated with preterm delivery.48–51 These cytokines produced by placental macrophages are involved in the uncontrolled apoptosis of extravillous trophoblasts during PE and IUGR.52 The role of IFN-γ produced by placental macrophages is also supported by parasitic- and bacterial-mediated complications of pregnancy. IFN-γ found in the placenta of pregnant women with malaria may be associated with placenta lesions responsible for defective labyrinth vascularization of the placenta and fetal growth restriction.53,54 Following lymphocyte elimination by means of anti-CD3 monoclonal antibodies, placental blood mononuclear cells from healthy pregnant women secrete IFN-γ in response to soluble malaria antigens.55 Macrophage-derived IFN-γ is likely involved in Q fever-associated pregnancy complications, such as placentitis and in antibacterial defense of placenta.56 We recently reported that CD14+ placental macrophages from at term pregnancy released large amounts of IFN-γ following stimulation by C. burnetii,17 an intracellular bacterium responsible for Q fever and known for its placenta tropism.57–59 Using a previously reported method of isolation, we obtained placental macrophage CD14+/CD68+ with 98% purity.28 The secretion of IFN-γ by placental macrophages was sustained during the time of culture with bacteria, suggesting that macrophage-derived IFN-γ is present in early and late phases of antibacterial response. Interestingly, IFN-γ secreted by C. burnetii-stimulated placental macrophages was associated with bacterial elimination. We believe that intracellular staining of IFNγ in macrophages and the studies at single cell level will remove all the ambiguities related to Schleicher’s paper. Taken together, these results reinforce the idea that macrophages are a source for IFN-γ useful to control infections, but IFN-γ may be also involved in pathologies, especially placental pathologies.
Concluding Remarks
The purity of macrophages impacts our analysis of publications in which expression and/or production of IFNγ by macrophages is reported. The isolation techniques of macrophages lead to «classical» purity, which does not match the highly purified ones shown in Schleicher’s paper. The use of FACS-sorted or single cell methods may provide convincing conclusions for the production of IFN-γ by macrophages. In addition, the use of sensitive detection methods such as marking with magnetofluorescent liposomes or more sensitive techniques to evaluate the cytokine secretion may be beneficial. Finally, using more efficient isolation techniques and more sensitive methods could help address the last point concerning the production of IFN-γ by different macrophage populations.
The story of macrophage-derived IFN-γ is an example of the challenge of “normal science” by the discovery of “anomalies” that question the paradigm of lymphoid origin of IFN-γ. This reading inspired by Thomas S. Kuhn60 will help us understand why the observation of macrophage-derived IFN-γ 40 years ago has been without follow-up for more than 10 years. Our finding on the importance of macrophage-derived IFN-γ in response to bacteria within placenta will help reopen the issue of IFN-γ sources. Further studies on tissue macrophage populations using new technologies will be necessary to change the lymphoid origin paradigm of IFN-γ. Such a change will require a reassessment of the role of the IFN-γ in innate immunity.
Authorship
S.M and J.L.M conceived and wrote the paper.
Abbreviations
- BCG
bacillus Calmette-Guérin
- BMDM
bone marrow-derived macrophage
- CD
cluster of differentiation
- DC
dendritic cell
- DPP
derived purified protein
- ESA
ELISPOT assay
- FISH
fluorescent in situ hybridization
- GSE
genomic spatial event database
- HLA
human leukocyte antigen
- ILC1
innate lymphoid cell-1
- IUGR
intrauterine growth restriction
- LT
T lymphocyte
- MAC
macrophage antigen
- MDM
monocyte-derived macrophage
- mRNA
messenger ribonucleic acid
- MyD88
myeloid differentiation factor 88
- Ni
Not indicating
- PE
preeclampsia
- RAG
recombination-activating genes
- WT
wild-type
Acknowledgments
Soraya Mezouar was supported by a “Fondation pour la Recherche Médicale” postdoctoral fellowship (reference: SPF20151234951) and then by the “Fondation Méditerranée Infection.” This work was supported by the French Government under the “Investissements d’avenir” (Investments for the future) programme managed by the “Agence Nationale de la Recherche” (reference: 10-IAHU-03). We thank Dr. Christian Capo and Dr. Joana Vitte for their help and advice in writing the manuscript.
Disclosures
The authors declare no conflicts of interest.
References