-
PDF
- Split View
-
Views
-
Cite
Cite
Farnam Ajamian, Ramses Ilarraza, Yingqi Wu, Katherine Morris, Solomon O Odemuyiwa, Redwan Moqbel, Darryl J Adamko, CCL5 persists in RSV stocks following sucrose-gradient purification, Journal of Leukocyte Biology, Volume 108, Issue 1, Jul 2020, Pages 169–176, https://doi.org/10.1002/JLB.4MA0320-621R
- Share Icon Share
Abstract
Respiratory syncytial virus (RSV) is associated with bronchiolitis in infancy and the later development of asthma. Research on RSV in vitro requires preparation of a purified RSV stock. The objective for this work was to develop best methods for RSV purification, while monitoring the samples for potential contaminating proinflammatory mediators. Using polyethylene glycol concentration, and sucrose-gradient ultracentrifugation, we collected samples at each step of purification and measured the values of RSV titer, total protein (µg/mL), and proinflammatory cytokines (ELISA). We analyzed the efficacy of each step in the purification procedure. In so doing, we also determined that despite optimal purification methods, a well-known chemokine in the field of allergic disease, CCL5 (RANTES), persisted within the virus preparations, whereas other cytokines did not. We suggest that researchers should be aware that CCL5 appears to co-purify with RSV. Despite reasonable purification methods, a significant level of CCL5 (RANTES) persists in the virus preparation. This is relevant to the study of RSV-induced allergic disease.
Introduction
Respiratory syncytial virus (RSV) is one of the most common causes of significant lower respiratory tract infection during infancy and early childhood. There has also been an association of RSV bronchiolitis with the later development of atopy.1 Research on RSV in vitro requires preparation of a purified RSV stock, which can be challenging. Although there are studies outlining basic purification procedures,2–5 no studies appear to address the efficacy of the purification procedure and the ability to remove potential contaminating proinflammatory mediators generated by the virus itself during preparation in cell culture. The presence of such contaminants could give rise to false experimental results and others have shown concern for the presence of such contaminants in their virus stock.6
Our objective was to evaluate the steps in RSV purification concerning viral titer and removal of potential contaminating inflammatory mediators. Whereas a highly concentrated virus titer was achieved, and other proinflammatory mediators were removed, we found that the chemokine CCL5 (RANTES) was not removed from the virus preparation. CCL5 is recognized for its potent chemotactic effect in recruiting allergic inflammatory cells.7 Compared to other common airway viruses, RSV has been strongly associated with allergic inflammatory responses.8 We suggest that our data are quite important for researchers working with RSV. This paper may also shed new light on our understanding of the association between RSV and the development of allergic inflammation.
Materials and Methods
RSV propagation in cell culture
Mycoplasma-free HEp-2 cells (1.5 × 107 cells, ATCC CCL-23) were seeded on polystyrene tissue culture flasks (225 cm2) 36–48 h before the start of experiments. Cells were grown in complete DMEM containing 0.1 mM nonessential amino acids, 1 mM sodium pyruvate, 100 U/L penicillin, 100 mg/L streptomycin, and 10% FBS (Invitrogen, Burlington, Ontario, Canada). Recombinant enhanced green fluorescent protein-expressing RSV (rgRSV)9 was used in some experiments (a generous gift from Drs. Peter L. Collins and Mark E. Peeples, The Ohio State University College of Medicine, Ohio, USA). A nonfluorescent, RSV strain A2 (ATCC VR-1540) (hRSV) was also used in place of the rgRSV. The use of the rgRSV improved our ability to quantify RSV using a plaque assay compared to nonfluorescent RSV. HEp-2 cell monolayers (at 50–70% confluence) were infected with a multiplicity of infection (MOI) of 0.1 to 0.2, previously suggested as an optimal concentration to avoid defective interfering particles.10 After 1 h of incubation on a slow-moving rocker at room temperature (RT) followed by 1 h incubation at 37°C and 5% CO2, cells were nourished with fresh complete medium containing 10% FBS and kept in incubator. After 24 h, 50% of the medium was replaced with fresh medium containing 2% FBS.
RSV cell harvesting
Cells were checked regularly for signs of RSV infection by fluorescence microscopy and/or syncytia formation. Cells were harvested after 48–72 h when more than 50% of cells were detached from the cell culture flasks due to cytopathic effects. Using a cell scraper, the remaining attached cells were collected and pooled with the virus-rich supernatant.
Stages of RSV purification
We optimized a protocol based on previous work2,3 (see Fig. 1 outlining the steps). All steps of the purification were carried out on ice or under cold conditions (4°C) and, all work was performed in a biosafety hood under sterile conditions except for periods of centrifugation. The mixture of supernatant and cell debris was vortexed in a 50 mL conical tube for 1 min and centrifuged at 3200 ×g for 5 min. The virus-rich supernatant was collected and kept on ice. One aliquot was stored at −80°C for cytokine/protein analysis (sample A) whereas a second aliquot was mixed with equivalent amount of 40% sucrose (w/w) in NT buffer (150 mM NaCl, 50 mM Tris-HCL, pH 7.5) and stored (−80°C) for virus quantification. A minimum of 20% sucrose ensures virus stability in the freezer until virus titration assays can be completed.11
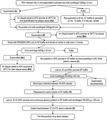
Shown are steps of respiratory syncytial virus purification used and the labeling for total protein, cytokine quantification, and virus quantification through plaque assay
To increase virus titers, we detached cell-associated virus using sonication. This method was chosen in preference to cell freezing because we found that a freeze-thaw cycle lowered viral titers by as much as one third (data not shown). The pellet was resuspended in 25 mL of NT buffer, which was sonicated at 12 W for five consecutive 1 s pulses, after 1 min of vigorous vortexing. A total of 12 W was chosen based on a comparison of 4, 8, and 16 W in a separate experiment (data not shown). The sonicated cell suspension was remixed with the cell supernatant. To remove larger pieces of cell debris, the mixture was centrifuged (3200 ×g for 20 min) and the supernatant separated from the pellet (sample B). Virus particles in the supernatant were pulled out of solution and concentrated using polyethylene glycol 6000 (PEG6000, Sigma-Aldrich, Oakville, ON, Canada) in NT buffer (50% w/v) followed by centrifugation (3200 ×g for 20 min). The pellet was diluted in NT buffer containing 20% sucrose (w/w) up to 20× of its original volume (sample C).
To remove all the contaminants with less than 1.13 g/cm3 density from the solution, the sample was layered on 30% sucrose (w/w) and ultracentrifuged (17,000 RPM for 60 min, 4°C, SW40Ti swinging bucket rotor, Beckman XL-90 ultracentrifuge, ON, Canada). This method yielded a dense pellet that was difficult to resuspend. To avoid this, we layered 1 mL of 60% sucrose in NT-buffer at the bottom of the ultracentrifuge tube to form a high-density cushion. An opaque band formed between 30% and 60% layer and was collected and diluted with a similar volume of NT-buffer (with no added sucrose) (sample D). Finally, the mixture was layered on top of a discontinuous gradient of 37%, 47%, and 60% sucrose in NT-buffer (corresponding to 1.16, 1.21, and 1.29 g/cm3 density) and was centrifuged for 2 h at 165,000 ×g (40,000 RPM, 4°C, SW40Ti, Beckman XL-90 ultracentrifuge). These densities were chosen due to high concurrence of RSV particles expected between 1.16 and 1.21 g/cm3 levels.3,4,12 As predicted, a distinct visible band formed in the interface between 37% and 47%. This was collected and saved as the final virus stock (sample E) (Fig. 2).
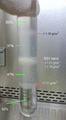
Shown is the image of final step in sucrose-gradient ultracentrifugation of respiratory syncytial virus (RSV) preparation. The collected RSV-rich band is between 37% and 47% of sucrose (w/w) that corresponds to 1.16 and 1.21 g/cm3 of mass density, respectively
RSV quantification
HEp-2 cells were seeded in 24-well plates (80,000 cells per well) 24 hr earlier, resulting in the desired 70% confluent monolayer (37°C, 5% CO2) for the day of experiment. HEp-2 cells were chosen over Vero cells because they appear to form larger syncytial plaques needed for quantification.13 Ten-fold serial dilutions of the various sample conditions (A, B, C, D, E) were prepared in DMEM with 2% FBS and distributed in triplicate in wells with 70% confluent monolayer. Inoculated cells were incubated 1 h on a rocker at RT and 1 h at 37°C (5% CO2). Infected cell supernatants were removed and the infected cells were topped up with complete DMEM containing 2% carboxy methyl cellulose. After 24 to 48 h (37°C, 5% CO2), bright green spots were counted by fluorescent microscopy; each spot representing one PFU. To confirm the accuracy of the fluorescent counts, plates were kept for up to 7 d (37°C, 5% CO2) allowing the typical syncytial plaques to mature. Cells were fixed (1% gluteraldehyde, 1 h at RT). Monolayers were stained with crystal violet (0.0375% w/v in water, 5 min). Syncytial plaques (seen as clearings without violet staining) were counted using 5× magnification.
Protein quantification
The Compat-Able Protein Assay Preparation Reagent Kit (Pierce Biotechnology, Rockford, IL, USA) was used to eliminate interfering substances including sucrose and PEG from samples. Protein concentration in various steps of purification was measured using BCA Protein Assay Kit (Pierce Biotechnology).
Cytokine/chemokine measurement
At the various stages, samples were saved for cytokine testing by a commercially available multi-array ELISA service (Aushon Biosystems, Woburn, USA) to measure CCL5 (RANTES), TNF, and IFN-α. Samples were diluted ×10 in PBS containing 0.1% HSA to eliminate potential interference from sucrose or PEG in these measurements. We further confirmed the CCL5 concentration in sample E using a single-analyte ELISA assay (R&D Systems, Minneapolis, MN, USA).
Western blot analysis for CCL5 protein
Aliquots of sample E (20 µl of 1:5 dilution) were loaded per gel lane and run through the Any KD Mini-PROTEAN Precast gel (Bio-Rad, Mississauga, Ontario, Canada). Recombinant human CCL5 (R&D Systems, 10 ng/lane) was used as positive control for the assay. Loading buffer contained 5% β-mercaptoethanol (120 mM final concentration) and both samples and standards were denatured for 5 min at 95°C before loading on to the gel. Following transfer of separated proteins, nitrocellulose membranes were blocked overnight (4°C) with Odyssey Blocking Buffer (Licor, NB, USA) and then incubated (1 h, RT) with mouse anti-RSV Fusion protein (Millipore, Billerica, MA, USA) and goat anti-human CCL5 (R&D Systems). The membrane was probed for 45 min (RT) using donkey IRDye800-labeled anti-mouse IgG and donkey IRDye700Dx-labeled anti-goat IgG (Rockland Immunochemicals, Gilbertsville, PA, USA) antibodies and scanned for infrared signal using the Odyssey Imaging System (LI-COR Biosciences, Lincoln, NE, USA). Precision Plus Protein Standards (Bio-Rad) was used to estimate the molecular weight of apparent bands.
Sequence comparison analysis of CCL5 and RSV proteins
We analyzed sequence identities of the 11 RSV proteins compared to human CCL5 (Protein Database at NCBI). The Protein Database at NCBI is a compendium of various information sources including protein sequence as well as translation of DNA/RNA coding sequences (SwissProt, PIR, PRF, PDB, GenBank, RefSeq, TPA). We analyzed RSV proteins NS1 (Protein Database access number NP_056856.1), NS2 (NP_056857.1), Nucleocapsid (gp03, NP_056858.1), Phosphoprotein (gp04, NP_056859.1), Matrix protein (gp05, NP_056860.1), Small Hydrophobic protein (gp06,NP_056861.1), Attachment protein (G-protein, NP_056862.1), Fusion protein (F-protein, NP_056863.1), Matrix protein 2 (gp09, ORF1, NP_056864.1), Matrix protein 2 (gp09, ORF2, NP_056865.1) and Polymerase protein (NP_056866.1). We compared these proteins against CCL5-precursor reported sequence (NP_002976); CCL5 precursor protein comprises both the signal peptide (amino acids 1–23) and the active peptide (amino acids 24–91) for CCL5. Thus, the complete sequence of both the precursor and the active forms of CCL5 were compared with RSV proteins. Sequence analysis was done using the online SIM Alignment Tool for protein sequences from the Swiss Institute of Bioinformatics (http://ca.expasy.org/tools/sim-prot.html), based on a previously reported informatic algorithm.14
Statistical analysis
Each value of n represents one purification experiment. Results are reported as mean ± sem except in few instances where the median proved to be a better representative. Data were analyzed by the Kruskal-Wallis test with Dunn correction to identify statistical differences between means, using GraphPadPrism version 3.0 (GraphPad Software, Inc., La Jolla, CA, USA). P values >0.05 were considered as nonsignificant.
Results
The concentration of RSV with each step of purification
Each step in the protocol was measured for the amount of RSV using a plaque assay. Sample A was the initial cell free supernatant from the Hep-2 cells and the reference point for virus titer (2.3 ± 1.24 × 107 PFU/mL) (Table 1 and Fig. 3). The addition of cell-associated virus (sample B) increased the virus yield by 26.4% ± 5.9 (n = 3, P < 0.05). Approximately half (52 ± 1.3%, n = 3) of the amount of virus in this large volume of media was precipitated and lost in the pellet of the PEG concentration step. A small amount of RSV was then lost through the discarded supernatant of the PEG concentration step (1.6 ± 0.31%, n = 3) (sample Bc). Consistent with previous reports, total virus yield continued to drop in the next two steps of the sucrose purification. The final recovery in step E was 25 ± 7.3% of the initial harvest.
Changes in absolute and relative concentration of respiratory syncytial virus particles in different steps of purification
. | Steps in viral purification . | ||||
---|---|---|---|---|---|
. | A . | B . | C . | D . | E . |
Relative infectivitya | 1 | 0.887 | 6.90 | 5.00 | 5.95 |
Absolute infectivity (×10)7(PFU/mL)b | 2.298 (±1.24) | 2.608 (±1.6) | 17.65 (±15.7) | 45.68 (±39.8) | 27.95 (±23.8) |
Protein concentration (µg/mL)b | 1310 (±159) | 1249 (±105) | 822 (±100) | 441 (±114) | 638 (±60) |
Infectivity/total protein (×104)(PFU/pg)a | 0.895 | 1.13 | 2.558 | 15.9 | 10.7 |
. | Steps in viral purification . | ||||
---|---|---|---|---|---|
. | A . | B . | C . | D . | E . |
Relative infectivitya | 1 | 0.887 | 6.90 | 5.00 | 5.95 |
Absolute infectivity (×10)7(PFU/mL)b | 2.298 (±1.24) | 2.608 (±1.6) | 17.65 (±15.7) | 45.68 (±39.8) | 27.95 (±23.8) |
Protein concentration (µg/mL)b | 1310 (±159) | 1249 (±105) | 822 (±100) | 441 (±114) | 638 (±60) |
Infectivity/total protein (×104)(PFU/pg)a | 0.895 | 1.13 | 2.558 | 15.9 | 10.7 |
Numbers represent median of the values for three separate experiments. Infectivity in step A is considered as a start point and all others are compared to A.
Numbers represent mean (±sem) of the values for three separate experiments.
Changes in absolute and relative concentration of respiratory syncytial virus particles in different steps of purification
. | Steps in viral purification . | ||||
---|---|---|---|---|---|
. | A . | B . | C . | D . | E . |
Relative infectivitya | 1 | 0.887 | 6.90 | 5.00 | 5.95 |
Absolute infectivity (×10)7(PFU/mL)b | 2.298 (±1.24) | 2.608 (±1.6) | 17.65 (±15.7) | 45.68 (±39.8) | 27.95 (±23.8) |
Protein concentration (µg/mL)b | 1310 (±159) | 1249 (±105) | 822 (±100) | 441 (±114) | 638 (±60) |
Infectivity/total protein (×104)(PFU/pg)a | 0.895 | 1.13 | 2.558 | 15.9 | 10.7 |
. | Steps in viral purification . | ||||
---|---|---|---|---|---|
. | A . | B . | C . | D . | E . |
Relative infectivitya | 1 | 0.887 | 6.90 | 5.00 | 5.95 |
Absolute infectivity (×10)7(PFU/mL)b | 2.298 (±1.24) | 2.608 (±1.6) | 17.65 (±15.7) | 45.68 (±39.8) | 27.95 (±23.8) |
Protein concentration (µg/mL)b | 1310 (±159) | 1249 (±105) | 822 (±100) | 441 (±114) | 638 (±60) |
Infectivity/total protein (×104)(PFU/pg)a | 0.895 | 1.13 | 2.558 | 15.9 | 10.7 |
Numbers represent median of the values for three separate experiments. Infectivity in step A is considered as a start point and all others are compared to A.
Numbers represent mean (±sem) of the values for three separate experiments.
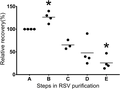
Relative recovery of respiratory syncytial virus in consecutive steps of the purification procedure. Each dot represents the total amount of virus (PFU) in a given volume (mL) per step of the experiment (total volume collected in mL) × virus titer (PFU/mL). The calculated values of the initial harvests (A) were assigned a normalized ratio of 100%. For downstream purification steps (B to E), the values shown were calculated and normalized compared with the initial harvest (A)
The concentration of total protein had a declining trend throughout the purification steps
The amount of total protein was measured in each step of purification. The supernatant of HEp2 cells infected with RSV showed the highest measured value (1310 ± 159 µg/mL; Table 1). However, this value was reduced by 50% when measured by the last step of purification (638 ± 60 µg/mL).
Unlike other cytokines, CCL5 cannot be removed using sucrose-gradient purification
We determined the quality of our purification method in relation to removal of contaminating proteins (cytokines/chemokines), as measured by ELISA at each step. As expected, TNF (49.4 ± 8.4 pg/mL) and IFN-α (7.55 ± 1.0 pg/mL) were detected in the supernatant of infected Hep-2 cells (sample A). As expected, their levels fell below the level of detection following the PEG concentration step (sample C). As expected, CCL5 was also present in the sample A (15,820 ± 1395 pg/mL, n = 3), but despite discarding 99.4 ± 0.39% of the volume in the PEG step (sample Bc), CCL5 levels remained elevated in the pellet. The total amount of CCL5 before the PEG (sample B) was 1624 ± 812 ng (volume multiplied by concentration). Only 41.0 ± 10.9% of this total CCL5 was eliminated in the PEG supernatant (785 ± 526 ng; sample Bc). In addition, after resuspending the PEG pellet at 20 times dilution, CCL5 levels surprisingly increased to an average of 61,784 ± 18,722 pg/mL. Extrapolating these values based on the dilution, the levels of CCL5 in the virus-rich pellet at PEG step were 112 ± 38 fold greater than in the initial harvest (step A). Despite sucrose purification steps, CCL5 levels remained elevated at 5301 ± 2491 pg/mL (sample E).
Sensitivity of Western blot analysis was not enough to detect CCL5 in the RSV stock
We used Western blot analysis to further confirm our ELISA data. Recombinant human CCL5 (10 ng/lane), used in the assay as positive control, was detected with a clear band corresponding to about 9 KD. F protein of RSV was detected as a band of about 72 KD in sample E. We were not able to see any signal in the predicted size range of CCL5 in the virus sample (Fig. 4A, n = 3). To elucidate the detection limit of the anti-CCL5 Western blot technique, we ran a slot blot analysis of different concentrations of CCL5. Unfortunately, values below 3 ng/lane were not within the detection limit of Western blot analysis, which is the level likely present in the RSV sample (Fig. 4B).
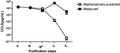
Western blot analysis of respiratory syncytial virus (RSV) stock (sample E) for detection of CCL5. A—20 uL of either 1:5 RSV stock dilution or CCL5 standard (containing 10 ng/lane) were loaded to each well. The nitrocellulose membrane was incubated with anti-RSV fusion protein and goat anti-human CCL5 before scanning for infrared signal using the Odyssey Imaging System. Western blot was not able to detect CCL5 in RSV sample. B—Slot analysis of CCL5: serial dilutions of CCL5 standard were loaded to different slots on a nitrocellulose membrane prior to incubation with anti-CCL5 antibody and subsequent probing with donkey anti-goat IgG (IRDye700Dx). Values of CCL5 less than 3 ng/lane were not detectable by Western blot analysis
CCL5 and RSV do not share similarities in protein sequence
We considered the possibility that a protein derived from RSV resembles CCL5, thereby generating false positive results in our ELISA-based assays. Our sequence analysis on human CCL5 compared to RSV proteins NS1, NS2, nucleocapsid (gp03), phosphoprotein (gp04), matrix protein (gp05), small hydrophobic protein (gp06), attachment protein (G-protein), fusion protein (F-protein), matrix protein 2 (gp09, ORF1, and 2), and polymerase protein yielded less than 50% sequence homology in any 10-aa length fragments analyzed for any combination. The highest identity with CCL5, found in segments of 10 or more amino acids were for the polymerase protein (5 out of 11 nonconsecutive amino acids, 45%) and NS2, phosphoprotein, small hydrophobic protein, and G-protein (4 of 10 nonconsecutive amino acids, 40%). The longest sequence of contiguous amino acids with 100% identity was 4, and it comprises two regions in the polymerase protein (CCL5 precursor protein amino acids 51–54, compared with RSV polymerase protein amino acids 1668–1671, and CCL5 amino acids 69–72 to Polymerase amino acids 1277–1280) and one in the matrix protein (CCL5 amino acids 86–89, compared with matrix protein amino acids 175–178). These values were considered too low for nonspecific antibody cross-reactivity due to sequence homology.
Discussion
The initial intent of this work was to develop best methods for RSV purification and confirm the reasons for each step in the methods. Purification of RSV using sonication lead to the best release of undetached viral particles from cell debris and a remarkable effect on virus enrichment. Approximately 25% improvement compared with using a freeze-thaw cycle. This was a critical step in purification, because it allowed us to start with the highest virus titer possible.
Thereafter it was expected that we see a significant loss of RSV yield during the ensuing purification steps. The largest loss was following the PEG concentration step, used to bind virus and pull it out of the dilute solution into a pellet. Approximately half the virus titer was lost by the PEG step (sample C). This loss did not appear to be a failure of PEG to capture the virus, as only 1.3% of the virus was measured in PEG supernatant. Further, we observed at each subsequent step of purification approximately 50% loss of virus. Thus, we hypothesize that these drops in virus titer were not due to loss of virus particles, but rather to loss of functional (infectious) virus. Unlike many other viruses, RSV loses function in RT, and to a lesser extent in ice-cold conditions. Even at 4°C, there is loss of virus infectivity compared to minus 80°C.11 Further, it is well known that for long-term storage, RSV needs a stabilizing agent (e.g., sucrose) to prevent loss of virus infectivity.11 Despite having sucrose in steps D and E, significant losses were observed. We suggest that the lability of RSV from thermal damage may play a major role in the loss of virus titer.
Despite the loss of virus number, the advantage of this purification process is the ability to concentrate RSV into the smallest volume possible. Under typical in vitro experimental conditions, it is important to achieve the required MOI without adding unnecessary medium. Such media could have unwanted factors released from the infected cell line used to propagate the virus. Due to large reductions in volume at each step, the relative and absolute concentration of viral infectivity increased successively (Table 1). As expected, the concentration of total protein also declined (Table 1). There are many contaminant proteins from infected cells that are removed. An important determinant of the quality of virus purification is the net ratio of infectivity to total protein (PFU/pg). We observed the intended goal of combining increasing virus titers with decreasing total protein following each purification step (Table 1). This ratio was almost 18 times higher in sample D in comparison to the initial harvest. We believe that the small loss of RSV infectivity in the last step of purification is due to the dilution of the virus with sucrose, not present in the previous step. This dilution is difficult to avoid if one wants to isolate the entire purified band of virus. Overall, when planning to create a virus stock, it is important to optimize procedures for the highest viral unit per volume, minimizing the inevitable loss of virus titer during purification process. We believe this was accomplished.
We used sucrose as a density gradient rather that iodixanol.4 Given our interest in co-culturing RSV with live immune cells,3 we considered sucrose to be a better alternative, based on the current lack of knowledge as to the immunologic effects of iodixanol.15 Although there still could be some concern about the osmotic effect of sucrose, we have calculated that at 1:100 dilution of RSV, the resultant osmolarity in culture media would be within a physiologically normal and acceptable range (20% sucrose in a 1:100 dilution equals a 6.3 mM increase in osmolarity for a 280–300 mOs/L culture media).
When we began this work our hypothesis was that cytokines and chemokines would be removed from the virus stock, as they should be free in solution (Fig. 5). In contrast, we saw that whereas we removed a substantial portion of free contaminants including CCL5, were unable to completely remove CCL5. This suggests a novel characteristic of CCL5 that it is in some way associated with the virus. We tested TNF, IFN-α, and CCL5 as some examples of a broad range of chemokine/cytokines that might be induced by RSV in HEp-2 cells.16,17 Our TNF and IFN-α data showed that the PEG concentration step alone was sufficient to remove these cytokines. In contrast, the PEG step not only failed to reduce CCL5 concentration, but instead increased the concentration of CCL5 over 100-fold (112 ± 38, n = 3) in comparison to sample B (these numbers have been extrapolated from readings at sample C). Further, the sucrose steps also failed to remove CCL5. It was surprising that the several steps of dilution, did not reduce the CCL5 levels. Putting into numbers, the initial harvest was diluted by a factor of 425 ± 19 (n = 3) up to last step of purification. Given this, we mathematically predicted that the concentration of CCL5 at step B would decrease by more than 400-fold by step E. Instead, the CCL5 levels were concentrated similar to the RSV. This observation suggests that the majority of CCL5 was not virus free, but somehow was associated with RSV particles (Figs. 5 and 6).
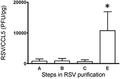
The ratio of RSV concentration (PFU/mL) to CCL5 concentration (pg/mL) increases significantly by the final step of purification. CCL5 was measured by ELISA at each purification step (n = 3). Error bars represent sem. P < 0.05
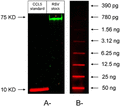
Measured concentrations of CCL5 at different purification steps plotted against a mathematically predicted pattern of free running molecules. Throughout purification, there were several steps to dilute a typical soluble low molecular weight substance (>400×) from step A to step E (dilution factor: 425 ± 19, n = 3). If CCL5 behaved like a free-running molecule, the mathematically predicted concentrations of CCL5 are shown from step B to step E (open circles). In contrast, filled squares show the measured levels of CCL5 per purification step (n = 3). Error bars represent sem
We considered the possibility that RSV proteins may share sequence homology with CCL5, leading to a false positive result from the ELISA-based assays. In fact, sequences of human CCL5 and RSV proteins showed less than 50% homology in any 10-aa length fragment analyzed for any combination. Considering that the longest sequence of identical contiguous amino acids was 4, and this was for RSV protein not associated with the surface of the viral particle (the RNA-dependent RNA-polymerase and one of the matrix proteins) these values were considered too low to account for nonspecific cross-reactivity due solely to sequence homology. There is a possibility that secondary and tertiary structures, 3D folding and interactions between RSV proteins may result in CCL5-like structures. However, such an event is even more unlikely if we take into account the fact that similar results were obtained in two different ELISA kits, which are based on antibodies from distinct sources. However, we acknowledge that in silico sequence analysis alone is insufficient to rule out such possibility.
It is well established that host cell proteins can be incorporated into RSV particles during virus formation.18 This experience is not unique to RSV, as other viruses including human immunodeficiency virus 119 or influenza20 have shown a similar ability. In a more recent report, almost 25 host cell proteins have been detected in a purified RSV stock.21 Incorporation of host cell proteins span the range from proteins involved in cell signaling22 to those of cellular skeleton, for example, actin.23 It has been rationalized that those proteins with a role in virus assembly and maturation may get inserted into viral particles or remain attached to viral progeny following release from the host cell.21 During purification of virus, these proteins also become purified, because they form part of the assembled virus. It remains unclear whether CCL5 is a part of the RSV capsule is attached or forms multimers that share similar density as that of RSV. To our knowledge, this is the first time that CCL5 is suggested as a host cell protein co-purified with RSV.
Negatively charged glycosaminoglycans (GAGs) like heparan sulfate on cell membranes play important roles in binding to and entrance of many viruses to target cells.24 RSV is among those viruses where heparin-binding domains on its F and G proteins interact with GAGs on target cells. Blocking these interactions with soluble heparin diminishes RSV infectivity.25–27 CCL5 binds with selectivity to GAGs and with highest affinity to heparin.28 Treatment of epithelial cells with recombinant CCL5 (5–20 µg/mL) is reported to inhibit RSV infection in vitro.29 Antibodies against CCR1, CCR3, CCR4, or CCR5 (receptors for CCL5), did not inhibit such infection. We speculate that the exogenous CCL5 in the study may have competed with CCL5 present on RSV. Exogenous CCL5 could attach to heparan sulfate on the cell surface, preventing access for the CCL5 on the RSV envelope; this could impair binding of RSV and infectivity. Ectromelia virus E163 (a murine poxvirus pathogen) counteracts the antiviral action of chemokines, by producing a glycoprotein, which blocks GAG-binding epitopes of different chemokines.30 With our suggestion that CCL5 is part of the RSV capsule, the role of CCL5 may be to help RSV attach and enter target cells through CCL5 affinity to heparan sulfate on cell membranes. This novel role for CCL5 incorporated to RSV may be an independent mechanism for RSV attachment or additional to the role of heparin binding domains on RSV’s F and G proteins.
It should be emphasized that despite the failure to remove all contaminating CCL5, we did obtain a high infectivity to CCL5 ratio (PFU/pg) (Fig. 5). This high ratio creates an RSV stock with high MOI but minimal contaminating chemokine. At a 1:100 dilution of the stock, which is a typical dilution in our experimental settings, this corresponds to values of CCL5 below 100 pg/mL. This would likely be below the minimum concentration of CCL5 reported with potential immunomodulatory effect in an in vivo study.31 We suggest that it is possible that in vitro studies could be affected by this amount. For example, if the virus (±UV killing) were incubated with leukocytes, would they drive chemotaxis. Such ideas should be considered in study designs.
In conclusion, whereas our study provides details of the necessary steps required for RSV purification, we also show that despite reasonable purification methods, a significant level of CCL5 persisted in the virus preparation. We propose that researchers using RSV should be aware of this issue. Beyond purification, our data may also shed new light on the mechanism of RSV-induced allergic disease. CCL5 is a critical chemokine in allergic inflammation including chemotaxis of effector cells and T cell activation.32–35 RSV is reported to induce a more allergic like inflammatory response compared to other airway viruses. Though the exact mechanisms are not yet defined, high levels of CCL5 in RSV bronchiolitis infants are associated with increased risk of recurrent wheezing in later childhood.33–36 If RSV and CCL5 are biologically linked, then our data may have significant impact on our understanding of the biology of RSV in the context of allergic airway diseases.
Authorship
F.A., D.A., S.O., R.I. conceived of the presented idea. F.A., S.O., R.I., Y.W., and K.M. performed or directly supervised the experiments. F.A. and D.A. wrote the manuscript with support of R.M. and R.I. D.A. and R.M. supervised the project.
Abbreviations
- Aa
amino acid
- GAG
Glycosaminoglycan
- MOI
Multiplicity of infection
- PEG
Polyethylene glycol
- RSV
Respiratory syncytial virus
Acknowledgments
The study was funded by AllerGen, NCE, the Canadian Institutes for Health Research (CIHR), a Faculty of Medicine and Dentistry 75th Anniversary Award at University of Alberta and an RL Jones GlaxoSmithKline Pulmonary Studentship Award. Dr. D.J.A. was an AHFMR Clinical Investigator and Dr. R.M. an AHFMR Medical Scientist.
References