-
PDF
- Split View
-
Views
-
Cite
Cite
Chen Hou, Lincheng Zhou, Hao Zhu, Xinyu Wang, Niran Hu, Fang Zeng, Liyuan Wang, Hang Yin, Mussel-inspired surface modification of magnetic@graphite nanosheets composite for efficient Candida rugosa lipase immobilization, Journal of Industrial Microbiology and Biotechnology, Volume 42, Issue 5, 1 May 2015, Pages 723–734, https://doi.org/10.1007/s10295-015-1602-0
- Share Icon Share
Abstract
By the facile adhesion way, the novel composite complex by polydopamine (PDA) and magnetic graphite nanosheets (Fe3O4@GNSs) has been successfully synthesized. The resulting composite was characterized by means of scanning electron microscopy, transmission electron microscopy, Fourier transform infrared spectra, and Raman spectra, X-ray diffraction, X-ray photoelectron spectroscopy, and vibrating sample magnetometry. Meanwhile, the PDA functionalized Fe3O4@GNSs (Fe3O4@GNSs-PDA) was applied for Candida rugosa lipase (CRL) immobilization covalently without any toxic coupling agent. Combining the superior physical properties and chemical stability of Fe3O4@GNSs and the well biocompatibility, functional characteristics of PDA, the Fe3O4@GNSs-PDA composite displayed several advantages, including the high enzyme capacity, enzyme activity and stability and a decrease in enzyme loss. Our work demonstrated that the mussel-inspired Fe3O4@GNSs can be extended to many other applications such as biocatalytic, genetic and industrial.
Chen Hou and Hao Zhu contributed equally to this work.
This paper is dedicated to memory of pro. Yanfeng Li, who passed away recently.
Electronic supplementary material
The online version of this article (doi:10.1007/s10295-015-1602-0) contains supplementary material, which is available to authorized users.
Introduction
Immobilization of biocatalysts has been generally adapted for synthesis of high stereoselectivity and enantioselectivity products with the consideration of green, economic and sustainable chemistry. Enzymes are an important group of biocatalysts superior to chemical catalysts because of their high effectiveness, high specificity, and green reaction conditions [1]. Enzymes have found practical use in industrial processes and are extensively used in new fields, such as bioengineering [2], food industry [3], pharmaceuticals [4], fine chemical industry, biosensors, and biofuel cells [5]. However, the poor catalytic activity, stability and selectivity as well as recycling problems of free enzymes limited the applications in extensive fields [6]. In order to increase the performance of enzymes, many supporting materials have been developed including polysaccharide [7], inorganic materials [8, 9], organic polymeric carriers [10], and natural polymers [11], etc. Nevertheless, aiming at design facile, universal, economical, and environmental friendly supporting materials in balancing the key factors, which determine the efficiency of biocatalysts, including surface area, mass transfer resistance, and effective enzyme loading still remains challenging.
Graphite, with the excellent mechanical, thermal, optical, and electrical properties [12], simulated ever-increasing attention, and various kinds of graphite series based matrix and hybrids have been prepared for compound and immobilization [13–15]. Combining the merits of the unique magnetic properties of Fe3O4 nanoparticles and chemical stability, non-toxicity of graphite nanosheets, the magnetic nanoparticles doped graphene or graphite composites have been caused excited interests and used as carriers of drug delivery, purification and detection of biomolecules, immobilization of enzymes, and enrichment of proteins recently [16–19]. Chen and co-workers fabricated electrically and magnetically bi-functional graphene/γ-Fe2O3 hybrid aerogels for immobilizing β-glucuronidase [20]. Ma et al. [21] developed a cost-effective way to prepare Fe3O4 and graphite nanosheets composite to immobilize glucoamylase covalently by an inverse co-precipitation method. Taking advantage of the high loading capacity and abundant hydrophilic groups of graphene oxide (GO), and large surface areas and hydrophobicity of reduced graphene oxide (rGO), Chen et al. [22] prepared strong magnetic GO-Fe3O4 and rGO-Fe3O4 for the immobilization of protein and the enrichment of peptides, respectively. Li et al. [23] introduced an easy one-pot polyol approach to hybridize size- and density-tunable graphene-Fe3O4 composites for the immobilization of porcine pancreatic lipase. Despite these developments, there is still a need for some chemical modification schemes of magnetic graphene or graphite nanosheets that can derivatize a variety of materials in bio-applications.
Dopamine (DA) mimics the repetitive catechol-amine structure of 3,4-dihydroxyl-l-phenylalanine found in mussel’s foot protein, which has excellent adhesion property with virtually all organic and inorganic surfaces [24]. Polydopamine (PDA) has recently been introduced by Lee et al. who reported its versatility for various substrates and feasibility for facile functionalization with spontaneous oxidative polymerization of DA under mild conditions [25]. Since then, dopamine was utilized as a versatile and intriguing starting material for almost all kinds of solid surface modifications very extensively due to the green, simple, fast, and low-cost synthesis approach [26–28]. Especially, the coated polydopamine films also can be used for the step-functionalization with organic species for the creation of functional organic ad-layers [29, 30].
Although the utilization of magnetic graphite nanosheets and DA for immobilization has been reported several times, the composite that combines the excellent properties of both of them by facile adhesion way is still very innovative. Herein, inspired by the adhesion mechanism of mussels, we utilized DA as an attractive functional monomer for the modification of Fe3O4 decorated graphite nanosheets (Fe3O4@GNSs) for enzyme immobilization.
In this work, we prepared the non-toxic, high affinity and rapid magnetic response magnetic graphite nanosheets composite via a one-pot hydrothermal approach, and the polydopamine-coated Fe3O4@GNSs composite was then fabricated by in situ polymerization of dopamine. Then enzymes could be immobilized on polydopamine-coated Fe3O4@GNSs composite via Schiff base reaction. The Candida rugosa lipase (CRL) was used as the target enzyme because of the specific property and the increased activity when bound to an interface [31]. Thanks to the combination of high capacity, superior physical stability of GNSs and well biocompatibility, functional characteristics of PDA, the Fe3O4@GNSs-PDA composite shows the high performance as an enzyme immobilization supporting material. Moreover, the enzyme immobilized on Fe3O4@GNSs-PDA composite would promise to be a novel nano-biosensor and nano-reactor for the catalytic reaction.
Materials and methods
Materials
Natural flake graphite (NFG) 50 BS mesh, with the purity of 99 wt % was used as a precursor for the synthesis of GNSs supplied by ShanDong Qingdao Tianhe Graphite Company (China). Dopamine hydrochloride (DA), ethylene glycol (EG), sodium acetate (NaAc) and FeCl3∙6H2O were purchased from AiHua Fine Chemicals Co., Ltd. (China). Candida rugosa lipase (CRL, Type VII) and bovine serum albumin (BSA) were purchased from Sigma Chemical Co. Other chemicals and reagents were of analytical grade, obtained from Tianjing Chemical Reagent Company (China).
Characterization
Fourier transform infrared (FTIR) spectra were obtained in transmission mode on a FT-IR spectrometer (American Nicolet Corp., Model 170-SX) using the KBr pellet technique. Raman spectra were carried out via a Reinishaw confocal spectrometer with 633 nm laser. Powder X-ray diffraction (XRD, Rigaku D/MAX-2400 X-ray diffractometer with Ni-filtered Cu Kα radiation (λ = 1.54056)) was used to investigate the crystal structure of the composites. All spectra were recorded under the same experimental conditions. The morphologies of the Fe3O4@GNSs and Fe3O4@GNSs-PDA were characterized by a field-emission scanning electron microscope (SEM, Hitachi S-4800, Japan), transmission electron microscope (TEM, FEI Tecnal G2F30) equipped with energy-dispersive X-ray spectroscopy (EDX, Oxford Instrument), high angle annular dark field (HAADF) and scanning transmission electron microscopy (STEM) to elucidate the dimensions and the structural details of the composites. The surface composition and oxidation state of the samples were performed by X-ray photoelectron spectroscopy (XPS, ESCALAB210). Magnetization measurements were characterized on a vibrating sample magnetometer (Lakeshore 7304, USA) at room temperature. The thermal stability of samples was studied with a thermogravimetry (TG) analyzer (STA449C, Netzsch, Germany) at heating rate of 10 °C min−1 in a nitrogen atmosphere.
Preparation of GNSs
GNSs were prepared according to the previous work of our group [21]. Typically, the mixed acidic intercalating agent was collocated with 70 % concentrated sulfuric acid (45 g) and 68 % nitric acid (15 g). Then 20 g of NFG was added slowly to the mixed acid in a small portion, and 2.2 g KMnO4 was dissolved into this mixture. The NFG was oxidized for 50 min under continuous stirring. Afterwards, the resulting solid mixture was washed with distilled water and a neutral elution solution through a centrifuge process was obtained; the precipitate was dried at 60 °C. The dried sample, i.e., graphite oxide (GO) was heat-treated abruptly at 600 °C for 15 s to obtain expanded GO. Then, dimethyl formamide (DMF) was used as dispersing agent to peel off the expanded GO. The prepared expanded GO was immersed in a dimethyl formamide (DMF) solution (2 mg/ml) in an ultrasonic bath and sonicated for 12 h, and lastly was filtered and dried to produce the graphite nanosheets (GNSs).
Preparation of Fe3O4@GNSs
An easy one-pot method was employed for preparation of Fe3O4@GNSs composite via a solvothermal method. In a typical procedure, GNSs (0.1 g) was added into ethylene glycol (30 ml) and ultrasounded under drastic stirring for 1 h. Then FeCl3∙6H2O (0.487 g) and sodium acetate (NaAc, 0.973 g) were dispersed into the aforementioned solution under continuous stirring so that Fe3+ chemically bonded onto expanded GO. After complete dissolution, the rich dark brown solution was transferred into a 50 mL Teflon reactor and heated at 200 °C for 8 h. After cooling down to room temperature (~20 °C), the solid product was gained by magnetic separation, and washed with deionized water and ethanol repeatedly several times. Finally the product was dried in vacuum at 40 °C for 8 h to obtain the uniform Fe3O4 nanoparticles embedded in GNSs.
Preparation of polydopamine modified Fe3O4@GNSs
The surface modification of Fe3O4@GNSs can be easily manipulated using dopamine with spontaneous oxidative polymerization under mild conditions. In a typical procedure, Fe3O4@GNSs (50 mg) was sonicated in a buffered solution (10 Mm Tris–HCl buffer, pH 8.5) for 30 min, and then a corresponding amount of dopamine (2 mg/ml) was added into the above suspension under vigorous stirring for a desired reaction time. The PDA-coated Fe3O4@GNSs was thoroughly rinsed with deionized water and ethanol repeatedly and then dried in a vacuum at room temperature for 8 h.
Immobilization of CRL
The CRL was immobilized onto Fe3O4@GNSs-PDA composite mainly via covalent binding [32]. Necessary quality of support was put into CRL solution (m/v, 1 %); then the lipase immobilization was carried out at 30 °C in a shaking-table with rotational speed at 120 rpm min−1 for 5 h. After the reaction was completed, the immobilized CRL was obtained by magnetic separation and washed with phosphate buffer (0.1 M, pH = 7.0) several times to remove the unreacted CRL. Especially, the reaction solution and washing solution were collected to assay the amount of residual lipase. The ICRL was kept at 4 °C prior to use.
During the immobilization procedure, the amount of lipase added and reaction time on the activity of immobilized lipase were investigated. The relative activity was obtained after incubation under different amount of lipase added (100–500 mg/g support), immobilizing time (1–9 h), respectively.
Determination of immobilization efficiency and lipase activity
The immobilization efficiency was expressed by the amounts of enzyme bounded on supports of unite mass, and using BSA as the standard to determine the amount of enzyme by the Bradford method [33]. The enzymatic activities of free and immobilized lipase were measured by the titration of the fatty acid which comes from the hydrolysis of olive oil [34] and reverse titration was adopted. One unit of lipase activity (U) is defined as the amount of enzyme needed to hydrolyze olive oil liberating 1.0 μmol of fatty acid per min in the assay condition.
The relative activity (%) is the ratio between the activity of each sample and the maximum activity of the sample.
The residual activity (%) is the ratio between the activity of each sample and the initial activity of the sample.
All data used in these formulas are the average of triplicate of experiments.
Properties of Fe3O4@GNSs-PDA immobilized CRL
Effect of pH and temperature of free and immobilized lipase activities
A certain amount of free and immobilized CRL was incubated in 0.1 M 50 ml phosphate buffer under various pH (3.0–10.0) at 37 °C for 30 min with continuous stirring, respectively. Then the enzymatic activities were determined and the relative activities were calculated.
The effect of temperature on the activities of free and immobilized CRL was investigated in the temperature range 20–90 °C for 30 min. The relative activities were calculated.
Thermal stability of free and immobilized lipase activities
Thermal stabilities of the free CRL and immobilized CRL were determined by measuring the activities following incubation in 50 ml phosphate buffer (0.1 M, pH = 7.0) at 50 °C for 240 min with continuous stirring. A sample was removed at 30 min interval and assayed for enzymatic activity. Residual activity was calculated as mentioned above.
Effect of denaturant of free and immobilized lipase activities
A series of concentrations of urea-phosphate buffer solution (0.1 M, pH = 7.0) were added into the hydrolysis reaction of olive oil using free and immobilized lipase at 30 °C, respectively. Then the residual activity of free lipase and of the ICRL was calculated.
Reusability of ICRL
The reusability of the immobilized CRL was determined by hydrolysis of olive oil by the recovered lipase after magnetic separation and compared with that of the first running (activity defined as 100 %).
Results
Preparation and characterization of Fe3O4@GNSs-PDA
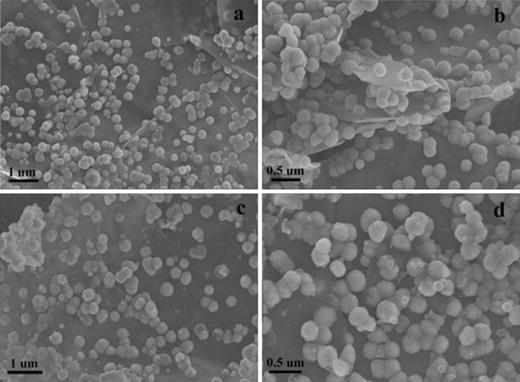
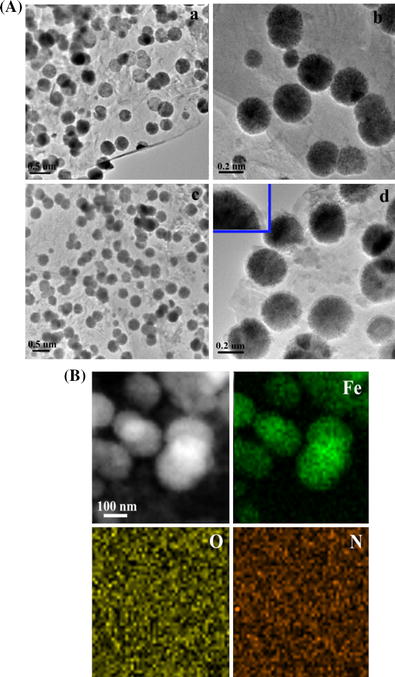
A TEM images of Fe3O4@GNSs (a and b), and Fe3O4@GNSs-PDA (c and d), B STEM image and corresponding iron, oxygen, and nitrogen elemental mapping of Fe3O4@GNSs-PDA composite
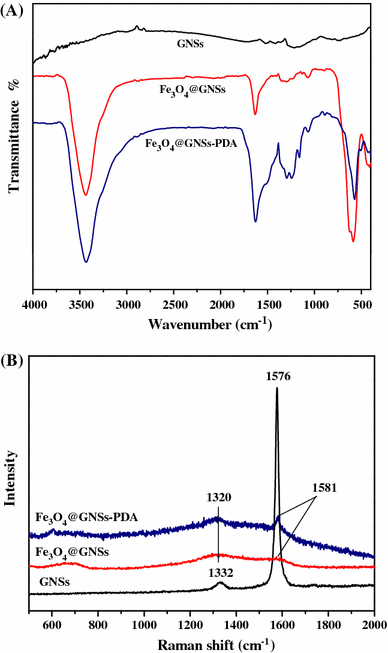
a FT-IR spectra of GNSs, Fe3O4@GNSs and Fe3O4@GNSs-PDA, b Raman spectra of GNSs, Fe3O4@GNSs, and Fe3O4@GNSs-PDA
In Raman spectrum (Fig. 3b), GNSs shows D-band peak at 1,332 cm−1 and G-band peak at 1,576 cm−1, respectively [41]. The G-band is due to the first-order scattering of E2g mode and is associated with the in-plane vibration of sp2 carbon domains in a 2D hexagonal lattice, while the D-band arises from the κ-point phonons of A1g mode and is related to the vibration of sp3 carbon domains in plane terminations of disordered graphite [42]. Compared with pristine GNSs, the D- and G-bands of Fe3O4@GNSs become lower and broader, suggesting a higher level of disorder of the graphite layers during the functionalization process [43]. The G-bands of Fe3O4@GNSs, occur at 1,581 cm−1, which is up shifted by 5 cm−1 compared to that of GNSs clearly demonstrates that there is a strong interaction between the Fe3O4 and GNSs. In the Raman spectra of Fe3O4@GNSs-PDA, the slightly split peak at 1,320 cm−1 with the sharp peak at 1,581 cm−1 can be characterized as the stretching and deformation of aromatic rings of PDA [44] which confirms the existence of PDA in Fe3O4@GNSs-PDA composite. What is more, it can be seen from Fig. 3b that these two characteristic peaks became sharper and more obvious than that of Fe3O4@GNSs at the same position, which resulted from the overlap of the two peaks between PDA and the typical D- and G-bands of Fe3O4@GNSs. The similarity in FT-IR and Raman spectroscopy further supported the affinity between Fe3O4@GNSs and PDA film.

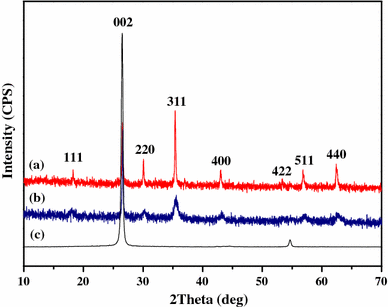
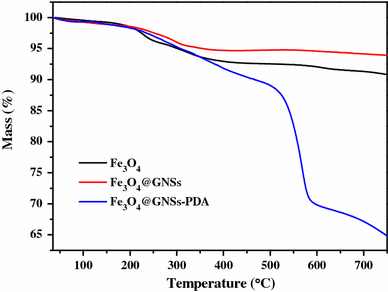
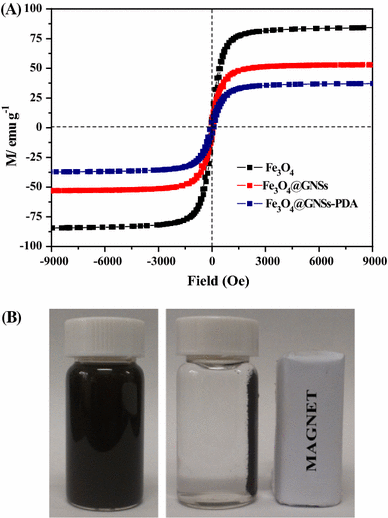
a Magnetic hysteresis loops of Fe3O4, Fe3O4@GNSs and Fe3O4@GNSs-PDA, b Schematic of the simple magnetic separation of Fe3O4@GNSs-PDA
Activity of Fe3O4@GNSs-PDA immobilized CRL
Optimum conditions of ICRL
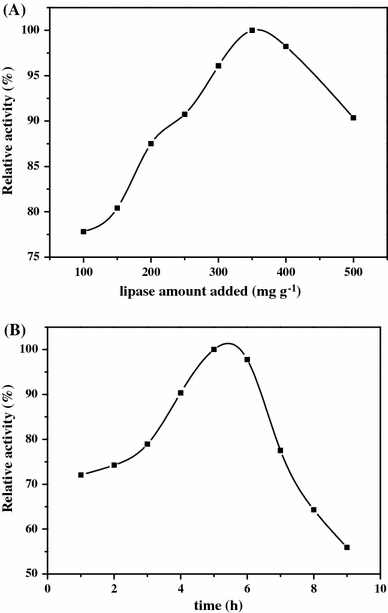
a The effect of the amount of CRL added, b The effect of the immobilization time of CRL. The enzyme immobilization was conducted in phosphate buffer solution (0.1 M, pH = 7.0) at 37 °C
Figure 8b shows the result of the immobilizing time of CRL. It can be seen that the optimum immobilizing time is 5 h, after 6 h the relative activity of ICRL decreased sharply. The main reason is that an appropriate reaction time contributed to couple the lipase on Fe3O4@GNSs-PDA, while the excessive coupling time may lead to the inactivation of CRL.
As a result, the optimum conditions of immobilizing lipase were obtained: amount of lipase was 350 mg g−1 support, reaction time was 5 h at pH value of the reaction system was 7.0. The bounded protein of the immobilized lipase under this condition was 337.96 mg g−1 support and the activity yield of the ICRL was 65.43 % (Table 1). Therefore, the results obtained in our work seemed to be quite promising.
Optimal conditions of immobilizing lipase
Support . | Lipase amount added (mg/g) . | Time (h) . | pH . | Protein bound (mg/g support) . | Immobilization yield (%) . | Activity recovery (%) . |
---|---|---|---|---|---|---|
Fe3O4@GNSs-PDA | 350 | 5 | 7 | 337.96 | 96.56 | 65.43 |
Support . | Lipase amount added (mg/g) . | Time (h) . | pH . | Protein bound (mg/g support) . | Immobilization yield (%) . | Activity recovery (%) . |
---|---|---|---|---|---|---|
Fe3O4@GNSs-PDA | 350 | 5 | 7 | 337.96 | 96.56 | 65.43 |
Optimal conditions of immobilizing lipase
Support . | Lipase amount added (mg/g) . | Time (h) . | pH . | Protein bound (mg/g support) . | Immobilization yield (%) . | Activity recovery (%) . |
---|---|---|---|---|---|---|
Fe3O4@GNSs-PDA | 350 | 5 | 7 | 337.96 | 96.56 | 65.43 |
Support . | Lipase amount added (mg/g) . | Time (h) . | pH . | Protein bound (mg/g support) . | Immobilization yield (%) . | Activity recovery (%) . |
---|---|---|---|---|---|---|
Fe3O4@GNSs-PDA | 350 | 5 | 7 | 337.96 | 96.56 | 65.43 |
Discussion
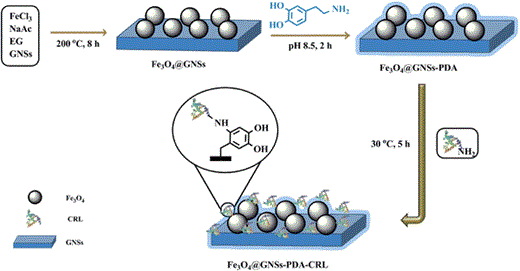
Schematic illustration of the synthesis process used to produce Fe3O4@GNSs-PDA and CRL immobilized on Fe3O4@GNSs-PDA
In this work, the self-polymerization of DA formed a thin, surface-adherent PDA film onto Fe3O4@GNSs under a controllable condition. Its bioactive surface can graft macromolecules directly via Schiff base formation between animo group of macromolecules and quinone group of PDA [32] along with some physical effects by the noncovalent functionalities such as amino and hydroxyl groups as well as π–π bonds [29]. Compared with many related works which usually use some synthetic reagents (such as glutaraldehyde, epichlorohydri, formaldehyde, carbodiimide (EDC), and hexamethy-lene-1,6-diamino-carboxysulphonate (HDACS)) as the cross-linkers between supporting materials and enzymes [45, 46], we provided a fast, effective, and most importantly, non-toxic process for lipase immobilization. Additionally, the chemical stability, high affinity of GNSs also contributed to the effective lipase immobilization. Fe3O4 nanoparticles embedded in GNSs not only acted as an easy separation tool in Fe3O4@GNSs-PDA composites, but also mitigated the restacking and aggregation of GNSs during the one-pot process. The principle of CRL immobilized on Fe3O4@GNSs-PDA composite is illustrated in Fig. 9.
Optimal conditions of enzymatic activity
The effect of pH value on the activity of ICRL is given in Fig. S1A. The optimal pH of both free CRL and ICRL is 7.0. In addition, compared to the pH endurance of free CRL, ICRL showed enhanced stability especially in the alkaline zone. It can be explained that by covalent attachment, the configuration of lipase was fixed on the surface of Fe3O4@GNSs-PDA so the tolerability of CRL to pH in surroundings increased [49]. The abundant–OH/-O− pairs and the–NH2/-NH3+ pairs on PDA made it a zwitter-ion under different pH range, which could tune the local pH value. Thus the immobilized CRL would stay in the buffer region against the environmental mutation. This indicates that the lipase immobilized on Fe3O4@GNSs-PDA possesses markedly improved adaptability in a wide pH range, which can greatly expand the applications of lipase in chemical and biocatalytic industry.
The operating temperature range is another important factor in limiting the practical applications of lipase. Figure S1B shows the effect of temperature of the solutions on the hydrolysis activity of free and immobilized lipase for olive oil. The optimum reaction temperature of both free and immobilized lipase is 30 °C, while the activity of free lipase decreased sharply with the increase of the temperature. Compared with free lipase, the ICRL kept its relative activity up to 80 % in the temperature range of 20–60 °C and exhibited the high relative activity of 42 % at 90 °C, revealed much superb heat endurance than that of the free lipase. The increase of the optimum reaction temperature for the immobilized CRL may be due to the covalent combination between the Fe3O4@GNSs-PDA and lipase as well as the change of the conformational integrity in lipase structure. Moreover, the protective effect provided by the flexible structure of the prepared Fe3O4@GNSs-PDA also conducted a tough performance with enzymes from denaturation at high temperature.
The strong thermal stability is one of the critical factors in the industrial applications. Figure S2A shows the residual activity of free and immobilized lipase at 50 °C on the hydrolysis reaction of olive oil. Both the free CRL and ICRL exhibited the similar trend: the residual activity declined along with the reaction time prolonged. While the ICRL declined less and more slowly compared with the free form. After 150 min, free CRL lost the activity while ICRL kept it at 30 % and did not lose activity when the reaction time was at 240 min. These results probably resulted from the excellent thermal tolerance, good mechanical hardness and high specific surface area of the prepared supporting material, which protected the CRL from unfolding and prevented conformational transitions.
A denaturant such as urea can cause the protein to unfold, which may highly restrict the industrial applications of lipase. In order to investigate the influence of denaturant on the inactivation of lipase, the effect of urea with different concentrations on the hydrolysis activity of free and immobilized lipase was determined at 30 °C in phosphate buffer (0.1 M, pH = 7.0). From Fig. S2B we can see the activity of CRL shows a progressive loss with the concentration increase of urea. The CRL showed a reasonable decrease and significantly prevented from denaturation after immobilization on Fe3O4@GNSs-PDA composite. The ICRL kept the excellent residual activity of 87 % in 3 M urea and still held it at about 75 % in 6 M urea. However, free lipase possessed only 21 % activity in 3 M urea and deactivated totally when the concentration of urea was up to 6 M, respectively. One of the possible reasons is the lipase was attached on Fe3O4@GNSs-PDA mainly via covalent bonding, and it is possibly conducted a higher activation energy for the molecules to recognize and prevented the ternary structure of lipase from denaturation [50].
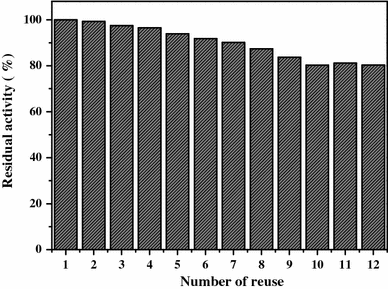
Number of reuses of the immobilized CRL. The recovered CRL was washed with phosphate buffer solution (0.1 M, pH = 7.0) thoroughly and reused for the next cycle. The enzyme activity was compared with the first running (activity defined as 100 %)
Conclusion
In this work, mussel-inspired magnetic graphite composite was successfully synthesized via the simple dip-coating way of dopamine. Then the as-prepared composite was used to immobilize CRL covalently under mild conditions and avoided any other toxic functional chemicals. The novel Fe3O4@GNSs-PDA composite was proved to possess high magnetization, a uniform well-defined structure and high affinity towards lipase immobilization. By means of measuring various properties of ICRL, the Fe3O4@GNSs-PDA composite was demonstrated to have high capacity and well biocompatibility for lipase immobilization, and the Fe3O4@GNSs-PDA immobilized CRL showed excellent abilities in environmental stability and catalytic activities. Significantly, the ICRL retained 80 % activity after 12 times of recycling for batch hydrolysis of olive oil emulsion. Overall, the successful application in enzyme immobilization using the adhesive magnetic graphite composite confirmed the suitability for various biochemical applications.
Acknowledgments
The authors thank the financial supports from the National Natural Science Foundation of China (No. 21374045), the scientific research ability training of under-graduate students majoring in chemistry by the two patters based on the tutorial system and top students (J1103307) and the Opening Foundation of State Key Laboratory of Applied Organic Chemistry (SKLAOC-2009-35).
Conflict of interest
The authors declare no competing financial interest.