-
PDF
- Split View
-
Views
-
Cite
Cite
Lin Xu, Mingfang Luo, Liangrong Yang, Xuetuan Wei, Xing Lin, Huizhou Liu, Encapsulation of Pannonibacter phragmitetus LSSE-09 in alginate–carboxymethyl cellulose capsules for reduction of hexavalent chromium under alkaline conditions, Journal of Industrial Microbiology and Biotechnology, Volume 38, Issue 10, 1 October 2011, Pages 1709–1718, https://doi.org/10.1007/s10295-011-0960-5
- Share Icon Share
Abstract
Cr(VI) was efficiently reduced to Cr(III) by Pannonibacter phragmitetus LSSE-09 encapsulated in liquid-core alginate–carboxymethyl cellulose capsules under alkaline conditions. Taking into account the physical properties of the capsules, the activity of encapsulated cells, and total Cr(III) concentration in the supernatant, optimal conditions (0.5% w/v sodium alginate; 2% w/v sodium carboxymethyl cellulose; 0.1 M CaCl2; 30-min gelation time) for LSSE-09 encapsulation were determined. At optimal conditions, a relatively high reduction rate of 4.20 mg g(dry weight) −1 min−1 was obtained. Total Cr(III) concentration in the supernatant was significantly decreased after reduction, because 63.7% of the formed soluble organo-Cr(III) compounds compared with those of free cells were captured by the relatively smaller porous structure of alginate capsules. The optimal pH value (9.0) for Cr(VI) reduction was not changed after encapsulation. In addition, encapsulated LSSE-09 showed no appreciable loss in activity after eight repeated cycles at 37°C, and 85.7% of its initial activity remained after 35-day storage at 4°C. The results suggest that encapsulated LSSE-09 in alginate–carboxymethyl cellulose capsules has potential biotechnological applications for the detoxification of Cr(VI)-contaminated wastewater.
Introduction
Chromium is widely used in electroplating, leather tanning, water cooling, metal finishing, and wood preservation [2, 30]. The effluents from these industries contain both hexavalent chromium, Cr(VI), and trivalent chromium, Cr(III). It is important to note that Cr(VI) is toxic to both plants and animals but Cr(III) exhibits only a little toxicity [11]. The most common approach for Cr(VI) removal is through reduction of Cr(VI) to Cr(III), followed by hydroxide precipitation of Cr(III) under alkaline conditions. Membrane separation and adsorption technology are also conventional methods to remove Cr(VI) [30]. However, these processes have been considered undesirable because of the high cost of chemicals used for Cr(VI) reduction, poor removal efficiency, and the production of large amounts of chemical sludge [20]. The development of suitable methods for detoxification of Cr(VI)-containing wastewaters has attracted worldwide attention recently.
Microbial reduction of the toxic Cr(VI) to the less toxic Cr(III) represents a promising detoxification process which is efficient and cost-effective [37]. The bioconversion of Cr(VI) by free bacterial cells has been widely investigated [6, 14, 23, 36]. However, the free cells suffer from Cr(VI) toxicity, which may cause cell damage and loss of activity [29]. Immobilizing techniques can enhance the stability and mechanical strength of the biocatalyst, and facilitate its recovery and reuse [9, 31, 39]. Industrial application of microbial reduction requires efficient methods to immobilize the cells, yielding a biocatalyst with high activity and stability compared with free cells. Among various immobilizing techniques, calcium alginate-gel entrapment has been widely used because its gelling process can be easily performed under mild conditions and alginate itself is inert and nontoxic [12, 26, 27].
Some studies have reported Cr(VI) reduction with immobilized bacterial species [16, 18, 29]. But entrapping cells in gel beads results in a decrease of biocatalytic activity caused by diffusional limitations and steric hindrance [22], a decrease of polymeric matrix strength caused by the increase of cell density, and cell leakage caused by gel surface breakage [19]. Encapsulation techniques using alginate gels as a semipermeable membrane to entrap cells within a liquid core were developed to overcome these disadvantages [10, 27]. Alginate capsules can be prepared by the interfacial gelation process with the addition of sodium carboxymethyl cellulose (CMC) as a thickening agent to maintain the spherical shape of the liquid droplets [17]. Alginate is cross-linked and thus solidified with the outward diffusion of divalent metallic ions (Ca2+ or Ba2+) from the inner liquid core. To date, a number of cells have been successfully encapsulated in alginate capsules with high activity retention and enhanced stability [7, 10, 15, 19].
However, to our knowledge, a study on the reduction of Cr(VI) with encapsulated whole cells has not been reported. Additionally, several research groups have reported that significant amounts of soluble organo-Cr(III) compounds remained in the supernatant of bacterial cultures and did not precipitate after Cr(VI) reduction [8, 25, 34, 40], even though the reduced Cr(III) is thought to be insoluble Cr(OH)3 when the pH ranges from 7 to 10 [33]. This problem restrained the biotechnological application of bacterial Cr(VI) reduction because the Environmental Protection Agency (EPA) in the USA has set a maximum contaminant level of 50 μg l−1 for total chromium (including Cr(VI) and Cr(III)) in drinking water [1]. Pannonibacter phragmitetus LSSE-09 was previously isolated from industrial sludge. It shows a strong potential to intracellularly reduce Cr(VI) to Cr(III) aerobically and anaerobically with acetate as an electron donor under alkaline conditions [40]. The present study was conducted to determine the capability of live cells of strain LSSE-09 encapsulated in alginate capsules to reduce Cr(VI) and remove soluble organo-Cr(III) compounds. It is the first to evaluate the encapsulation of bacteria cells for Cr(VI) reduction along with organo-Cr(III) removal. The optimal encapsulation conditions were determined on the basis of the physical properties of alginate capsules, the encapsulated cell activity, and total Cr(III) concentration in the supernatant after reduction.
Materials and methods
Materials
All chemicals used in this study including potassium dichromate (K2Cr2O7, analytical reagent grade), anhydrous calcium chloride (CaCl2, analytical reagent grade), sodium chloride (NaCl, analytical reagent grade), tryptone, yeast extract, sodium alginate (SA), and sodium carboxymethyl cellulose (CMC) were purchased from Sinopharm Chemical Regent Beijing Co., Ltd.
Preparation of LSSE-09-containing alginate capsules
The Cr(VI)-reducing strain LSSE-09 was isolated from industrial sludge of a chromate factory in Henan province, China [40]. This strain was inoculated into 250-ml flasks containing 100 ml pH 7.0 Luria–Bertani medium (10 g tryptone, 10 g NaCl, 5 g yeast extract, and 1 l deionized water) and cultured aerobically at 37°C and 150 rpm for 12 h. Strain LSSE-09 was harvested by centrifugation (4,000g) for 15 min at 4°C, washed twice with deionized water, and then suspended in 50 mM pH 7.2 Tris–HCl buffer. Tris–HCl buffer (50 mM, pH 7.2) was used as a universal solvent to dissolve all the chemicals.
Encapsulation of strain LSSE-09 was performed via an extrusion process followed by interfacial gelation [3]. To maintain the spherical shape of the capsules, CMC was used as a thickening agent and dissolved in CaCl2 solution of different concentrations (0.05, 0.10, 0.20, and 0.30 M) to give a final content of 2% w/v. Strain LSSE-09 was then added to the CMC–CaCl2 solution to obtain a cationic mixture with a bacteria content of 2.0 g l−1. The mixture was stirred for 30 min at room temperature. Two milliliters of the aforementioned CMC–CaCl2–(LSSE-09) solution was extracted into a syringe and extruded through an injection needle (inner diameter 0.45 mm) into 100 ml of SA solution (0.5% w/v), with a fixed distance of 20 cm from the needle head to the surface of SA solution. The following steps were conducted as described by Jiang and co-workers with minor modification [17]. The SA solution was stirred during the whole gelation process to facilitate mass transfer and to prevent the capsules from sticking together. After a specific gelation time (10–120 min), the mixture was poured into 200 ml of deionized water and stirred gently for 5 s before filtering through a piece of gauze. The unreacted SA on the surface of capsules was removed with 10 ml of Tris–HCl buffer. To reinforce the alginate membrane, the capsules were transferred into CaCl2 solution with the same concentration as that in the liquid drops and allowed to further cross-link for 10 min. Finally, the capsules were stored in Tris–HCl (50 mM, pH 7.2) buffer at 4°C.
Characterization of LSSE-09-containing capsules
Diameter and membrane thickness
The external diameter of the alginate capsules was measured by using a calibration micrometer. For membrane thickness measurement, capsule samples were cut in half and the thickness was measured under a polarizing microscope (Leica, DM LM/P). Values for two perpendicular diameters and four membrane thicknesses at positions 90° apart were measured for each capsule. Average diameter and average membrane thickness were obtained by analysis of 10 randomly selected capsules from each capsule batch.
Morphology
The micromorphology of the capsules was observed and measured by scanning electron microscopy (SEM; JSM 6700F, Jeol) after lyophilization.
Microbial reduction of Cr(VI)
Bioconversion of Cr(VI) to Cr(III) under anaerobic conditions by either free or encapsulated LSSE-09 with a dry cell weight of 0.2 g l−1 was carried out and compared. The reaction kinetics and cell activity were studied by measuring the amount of Cr(VI) and total Cr(III) in the supernatant.
LSSE-09-containing alginate capsules and alginate beads were introduced into 50-ml glass bottles containing 20 ml of 50 mg l−1 Cr(VI) and 3,000 mg l−1 acetate, both dissolved in Tris–HCl buffer (50 mM, pH 9.0). Acetate was used as an external electron donor and was essential to improve the reduction rate [40]. Each bottle was sparged with nitrogen for 3 min, introduced via a syringe needle through the self-sealing rubber septum. Air displacement was achieved by inserting another syringe needle as the outlet. Cr(VI) reduction experiments were performed at 37°C with shaking at a speed of 150 rpm. At different time intervals, aliquots of solution were withdrawn using a syringe and centrifuged at 12,000g for 5 min. The supernatants were analyzed for residual Cr(VI) and total Cr(III) concentrations.
In addition, a comparative study on the activity of free and encapsulated LSSE-09 with reaction pH ranging from 7 to 10 and 50 mg l−1 Cr(VI) was also performed. The Michaelis constant (K m) and the maximum reaction rate (V max) were determined by varying the substrate concentration from 20 to 60 mg l−1 at pH 9.0. In this experiment, all tested Cr(VI) could be completely reduced to Cr(III) by encapsulated LSSE-09. The kinetic constants were calculated by fitting the initial rate data to a double-reciprocal Lineweaver–Burk plot of 1/V [mg min−1 g cells−1] versus 1/[Cr(VI)] (mg l−1) derived from a linear transformation of the Michaelis–Menten equation [24].
The analysis of Cr(VI) in aqueous samples was performed using a UV–VIS spectrophotometer (UNICO7200, USA) at 540 nm after complexation with 1,5-diphenylcarbazide [13]. Total Cr(III) concentration in the supernatant was measured by an inductively coupled plasma atomic emission spectrometer (ICP-OES, Perkin Elmer Optima 7000DV, USA).
Storage stability
Recycling stability
Total Cr(III) concentration in the supernatant was measured at the end of each batch.
Results and discussion
Formation and characterization of LSSE-09-containing alginate capsules
Morphology
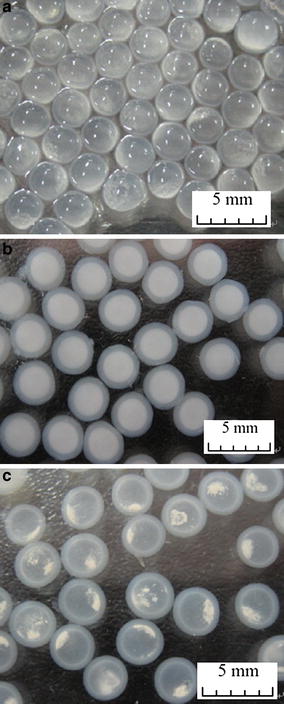
LSSE-09-containing capsules (CaCl2 concentration 0.3 M): a fresh capsules; b fresh capsules suspended in Tris–HCl buffer; c capsules suspended in Tris–HCl buffer after 7 days’ storage
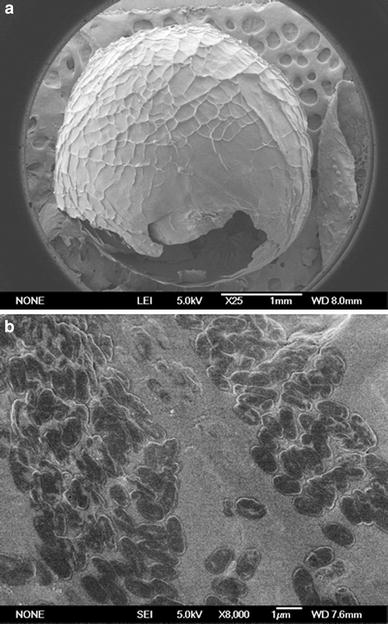
SEM photographs of the capsule: a the whole capsule; b the internal surface of the capsule
As described in Sect. “Preparation of LSSE-09-containing alginate capsules,” the capsules were prepared by a capsule formation stage and a reinforcement stage. During the capsule formation stage, the cross-linking of the interfacial alginate molecules with Ca2+ ions was initiated instantaneously when CaCl2–CMC–(LSSE-09) solution was added dropwise into the alginate solution. It was mainly Ca2+ ions that diffused between the alginate chains, binding preferentially to guluronic acid blocks (G-blocks) of the alginate [35]. Thus, with the outward diffusion of Ca2+ ions from the inside core, an external membrane around the droplet formed. Along the diffusion direction, the concentration of Ca2+ ions reduced because of the ionic cross-linking. The concentration gradient of Ca2+ ions between the core and the outside solution favored the diffusion of Ca2+ ions from the core to the outside, resulting in an inhomogeneous dense-to-loose structure. At the reinforcement stage, the outer surface of the SA membrane formed by further cross-linking between the free G-blocks and Ca2+ ions. Finally, the capsule with a CMC liquid core and porous alginate membrane formed [3, 4, 17, 21].
Gelation conditions
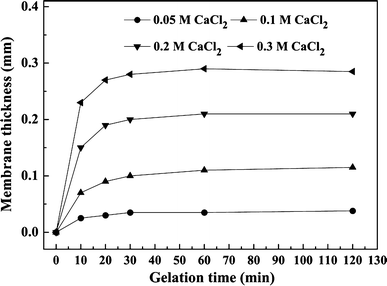
Growth of membrane thickness with gelation time at different CaCl2 concentrations (0.5% w/v SA)
Microbial reduction of Cr(VI) and cell activity
Effect of gelation time on reduction rate
LSSE-09 cells were physically entrapped in SA capsules, and the liquid core provided the cells inside sufficient space to move, resulting in a much easier accessibility and contact between cells and substrate compared with those of calcium alginate-gel entrapments. A previous study showed that strain LSSE-09 could reduce Cr(VI) to Cr(III) by the action of an intracellular reductase [40]. This enzyme-mediated Cr(VI) reduction process consists of four successive stages: diffusion of Cr(VI) ions from the outside into the liquid core of the capsules, uptake of Cr(VI) by strain LSSE-09, reduction by the intracellular reductase, and extracellular extrusion. The first two stages were significantly influenced by diffusion resistance which controlled the reduction rate.
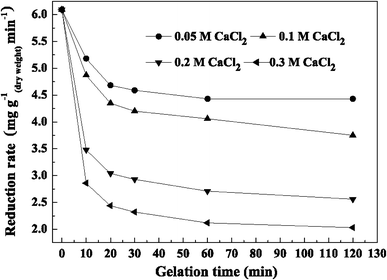
Reduction rate with gelation time at different CaCl2 concentrations
Furthermore, it was observed that the higher the concentration of CaCl2, the thicker the capsule membranes and thus the lower the resulting reduction rate. Reduction rate with 0.05 M CaCl2 was higher than with the other concentrations tested. When the gelation time was 30 min, reduction rates were 4.59 mg g(dry weight) −1 min−1 for 0.05 M CaCl2 and 4.20 mg g−1 min−1 for 0.1 M CaCl2, respectively, which were 75.4 and 69.0% compared with that of free cells, respectively. The lowest reduction rate was 2.32 mg g−1 min−1at 30 min when the CaCl2 concentration was 0.3 M.
Effect of gelation time on total Cr(III) concentration in the supernatant
As described in Sect. “Morphology,” each LSSE-09-containing capsule comprised a CMC liquid core and porous alginate membrane. To date, a number of investigations have been devoted to characterizing the pore size of Ca–alginate membranes. Because of the strengthened blocking effect of the membrane, it was found that some enzymes with different molecular weights ranging from 152 to 290 kDa were efficiently encapsulated in alginate capsules without significant leakage [4, 5, 17, 38]. As previously indicated, considerable soluble Cr(III) end-products which were deduced to be soluble organo-Cr(III) compounds were found in the supernatant after reduction [32, 40]. In this experiment, total Cr(III) concentration in the supernatant after reduction was investigated to establish whether the formed organo-Cr(III) compounds could be confined to the capsules or not, even though their molecular weights and species were not clear.
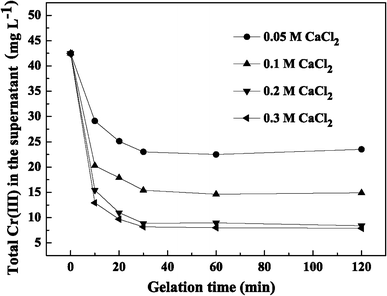
Total Cr(III) in the supernatant with gelation time at different CaCl2 concentrations
In addition, this method showed higher activity than other gel entrapment methods which used spherical gel beads with the same diameter (3 mm) as ours. A polyvinyl alcohol (PVA)-based immobilization technique was utilized for entrapping the Microbacterium liquefaciens MP30 for use in Cr(VI) reduction, but the chromate reducing activity of PVA–borate and PVA–alginate cell beads was only 50% of that of free cells as a result of higher mass transfer limitations [29]. Humphries and co-workers [16] developed agar and agarose cell beads for the immobilization of Desulfovibrio vulgaris NCIMB 8303 and Microbacterium sp. NCIMB 13776. The obtained beads showed similar initial reduction rates compared with those of free cells, but the beads showed much lower reduction rates than encapsulated LSSE-09 capsules, as well as weaker stability.
To determine the final optimal conditions for LSSE-09 encapsulation, the physical properties, the reduction rate, and total Cr(III) concentration in the supernatant should be taken into account. When the gelation time was 30 min, the membrane thickness increased to its maximum value and total Cr(III) concentration in the supernatant reached a lower level without obvious decrease. The highest reduction rate (5.27 mg g−1 min−1) was achieved at 0.05 M CaCl2. However, the capsules prepared at 0.10 M CaCl2 showed lower Cr(III) concentration and superior physical properties while also possessing an acceptable reduction rate (4.81 mg g−1 min−1). Therefore, the LSSE-09-containing alginate capsules prepared with 0.10 M CaCl2 and a 30-min gelation time were suggested as the optimal ones. The following further studies were carried out with capsules prepared under these conditions.
Effect of pH on cell activity
A comparative study on activity of free and encapsulated LSSE-09 with reaction pH value was performed. Their respective specific activity at optimal pH value was taken as 100%. pH is one of the most important factors influencing protein structure and the solution chemistry of the insoluble support. Protein–support interaction and surface properties of a protein are strongly influenced by the pH [28]. In this study, the activities of free and encapsulated LSSE-09 were significantly affected by pH values because Cr(VI) reduction was mediated by an intracellular enzyme.
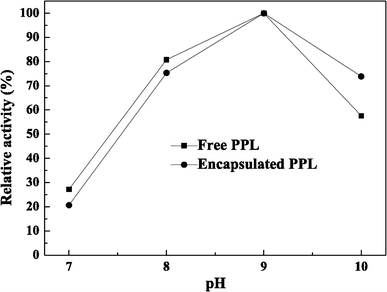
Cr(VI) reduction by free or encapsulated LSSE-09
Cr(VI) reduction by either free or encapsulated LSSE-09 was conducted in a sealed glass bottle under anaerobic conditions and a small amount of acetate was used as an electron donor to stimulate the reduction rate.
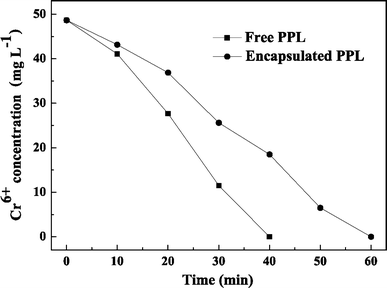
Kinetic parameters for free or encapsulated LSSE-09
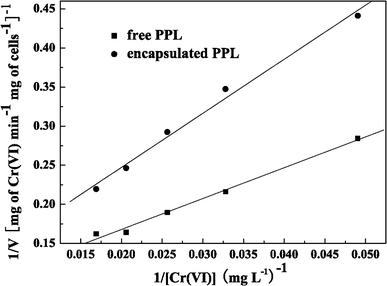
Typical Lineweaver–Burk plots for free and encapsulated LSSE-09 (pH 9.0, 37°C)
For Cr(VI) reduction by free cells of LSSE-09, the nonspecific half-saturation or Michaelis–Menten constant (K m) was estimated to be 44.44 mg l−1 and the maximum nonspecific reduction rate or maximum velocity (V max) was estimated to be 11.25 mg of Cr min−1 g of cells−1. For encapsulated LSSE-09, K m and V max were estimated to be 63.64 mg l−1 and 9.19 mg of Cr min−1 g of cells−1, respectively. After encapsulation, the maximum reaction rate V max was lowered, which was due to the additional diffusion resistance to the substrate Cr(VI) caused by the alginate membrane and the viscous CMC liquid core [17]. The increase in K m after encapsulation indicated a weaker binding between the substrate Cr(VI) molecules and the encapsulated LSSE-09.
Storage stability
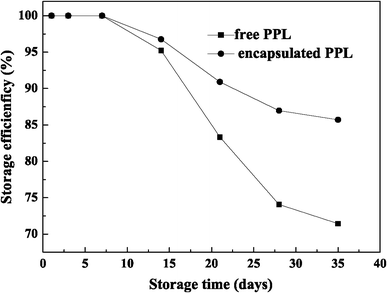
The encapsulated LSSE-09 has a distinct advantage over free cells in long-term storage. Because the LSSE-09-containing alginate capsule has a liquid core–membrane structure, which could provide a biomimetic microenvironment for those encapsulated LSSE-09 cells, the biocompatible alginate membrane effectively prevents any unfavorable influence from the outside storage environment [17]. Additionally, because Cr(VI) reduction was enzyme-mediated, some enzyme arising from cell decay could possibly be captured in the capsules and could reduce Cr(VI) to Cr(III).
Recycling stability
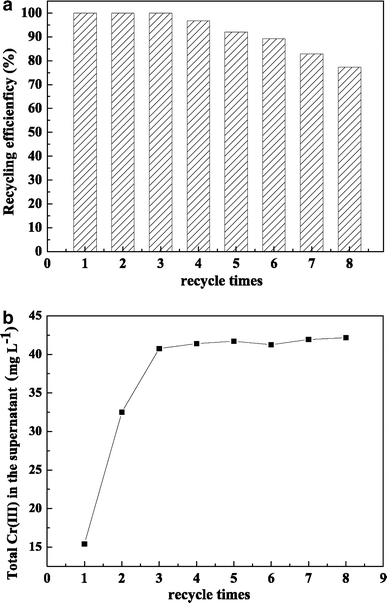
Recycling stability of encapsulated LSSE-09: a recycling efficiency of encapsulated LSSE-09; b total Cr(III) in the supernatant after each batch
Another trend should be noticed: Cr(III) concentration in the supernatant was originally 15.41 mg l−1, but it increased to 32.50 and 40.76 mg l−1 after the second and third batch cycles, respectively (Fig. 10b). After that, it reached a plateau with only a minor increase, which could be attributed to the saturation of captured organo-Cr(III) compounds relative to the porous structure in whole capsules. This system shows great promise for the detoxification of Cr(VI)-contaminated wastewater. The regeneration of the porous structure of the capsules is under further investigation.
Conclusions
Cr(VI) was efficiently reduced to Cr(III) by P. phragmitetus LSSE-09 encapsulated in liquid-core alginate–carboxymethyl cellulose capsules. The membrane thickness, the encapsulated reduction rate, and total Cr(III) concentration in the supernatant were significantly affected by CaCl2 concentration and gelation time. 0.1 M CaCl2 and 30-min gelation time were selected as optimal conditions to prepare LSSE-09-containing alginate capsules and a relatively high reduction rate of 4.20 mg g−1 min−1 was obtained. As much as 63.7% of the formed soluble organo-Cr(III) end-products compared with those of free cells were captured by the relatively smaller porous structure after reduction. The encapsulated LSSE-09, which had the same optimal pH for cell activity as that in the free form, was stable for eight repeated cycles without any appreciable loss in activity. In addition, the encapsulated LSSE-09 exhibited improved storage stability compared with free LSSE-09, and 85.7% of the initial activity was preserved after 35-day storage at 4°C. Encapsulated LSSE-09 therefore has great advantages over its free form and has potential biotechnological applications in the detoxification of Cr(VI)-contaminated wastewater.
Acknowledgments
This work was financially supported by the National Basic Research Program of China (No. 2007CB613507).
References
Goosen MFA (1993) Biomedical application of immobilised cells. In: Goosen MFA (ed) Fundamentals of animal cell encapsulation and immobilization. CRC, Boca Raton