-
PDF
- Split View
-
Views
-
Cite
Cite
Andrew H Karaba, William R Morgenlander, Trevor S Johnston, Camille Hage, Andrew Pekosz, Christine M Durand, Dorry L Segev, Mark A Robien, Peter S Heeger, Christian P Larsen, Joel N Blankson, William A Werbel, H Benjamin Larman, Aaron A R Tobian, Epitope Mapping of SARS-CoV-2 Spike Antibodies in Vaccinated Kidney Transplant Recipients Reveals Poor Spike Coverage Compared to Healthy Controls, The Journal of Infectious Diseases, Volume 229, Issue 5, 15 May 2024, Pages 1366–1371, https://doi.org/10.1093/infdis/jiad534
- Share Icon Share
Abstract
Kidney transplant recipients (KTRs) develop decreased antibody titers to severe acute respiratory syndrome coronavirus 2 (SARS-CoV-2) vaccination compared to healthy controls (HCs), but whether KTRs generate antibodies against key epitopes associated with neutralization is unknown. Plasma from 78 KTRs from a clinical trial of third doses of SARS-CoV-2 vaccines and 12 HCs underwent phage display immunoprecipitation and sequencing (PhIP-Seq) to map antibody responses against SARS-CoV-2. KTRs had lower antibody reactivity to SARS-CoV-2 than HCs, but KTRs and HCs recognized similar epitopes associated with neutralization. Thus, epitope gaps in antibody breadth of KTRs are unlikely responsible for decreased efficacy of SARS-CoV-2 vaccines in this immunosuppressed population.
Clinical Trials Registration. NCT04969263.
Coronavirus disease 2019 (COVID-19) vaccines are effective at inducing neutralizing antibodies (nAbs) and preventing severe disease, yet among solid organ transplant recipients (SOTRs), responses are attenuated and the risk of breakthrough remains high despite 2-dose mRNA series [1, 2]. Additional doses improve responses in some SOTRs, but many, particularly those with preceding poor responses, still fail to produce high-titer nAbs [3, 4]. Although common demographic and clinical factors (eg, advanced age, antimetabolite immunosuppression) are associated with poor responses, the mechanism(s) underlying persistent suboptimal immunogenicity remain uncertain.
A potential factor underlying the poor response in SOTRs is lack of antibody breadth across the full spike protein and/or poor response to specific key epitopes necessary for robust neutralization. Such spike epitope profiling has not been performed in SOTRs, and understanding this landscape has potential implications for optimizing vaccine formulations. Although the majority of severe acute respiratory syndrome coronavirus 2 (SARS-CoV-2) vaccine- and infection-associated nAbs target the receptor-binding domain (RBD), binding of other regions of spike can contribute to neutralization and warrant evaluation [5–7]. Notably, preexisting immunity to common cold coronaviruses (ccCoVs) may negatively impact the response to SARS-CoV-2 vaccination in SOTRs by means of antigenic imprinting and the targeting of ineffective epitopes. Due to immunosuppression, SOTRs may be biased toward epitopes with homology to previously encountered ccCoVs and rely more heavily on recall responses than healthy controls (HCs) [8, 9]. Therefore, simultaneously examining antibody epitope targets from ccCoVs and SARS-CoV-2 is important to investigate this possibility as a mechanism underlying poor responses to SARS-CoV-2 vaccines. Furthermore, understanding whether key epitope responses are missing in SOTRs is critical for vaccine development and strategy. To investigate SARS-CoV-2 antibody responses in SOTRs, we measured nAbs and mapped antibody responses to linear spike epitopes after a third dose of mRNA-based SARS-CoV-2 vaccine in a clinical trial of kidney transplant recipients (KTRs) with prior poor response to vaccination.
METHODS
Study Population and Sample Acquisition
KTRs who participated in the single-arm, open-label COVID-19 Protection After Transplant (CPAT) pilot trial (NCT04969263) and approved by the Johns Hopkins University institutional review board (IRB00288774) were included [10]. No changes were made to immunosuppression regimens as part of the trial. Blood samples were obtained on the day of vaccination prior to receiving a third dose, and 30, 90, and 180 days afterwards. Plasma was isolated by Ficoll centrifugation and samples were stored at −80°C. Participants who received a fourth dose outside of study protocol, did so 30 days prior to the 180-day time point. HCs were enrolled in an observational study of SARS-CoV-2 vaccination under Johns Hopkins IRB00027183 [4].
CoronaScan Assay (Epitope Mapping)
The CoronaScan assay is based on phage display immunoprecipitation and sequencing (PhIP-Seq) [11]. It contains epitopes from ccCoVs HKU1, OC43, 229E, and NL63; severe respiratory illness CoVs SARS-CoV-2, SARS-CoV-1, and MERS-CoV; and bat viruses BatCoV-Rp3, BatCoV-HKU3, and BatCoV-279. The design and cloning of the 56–amino acid coronavirus libraries and PhIP-Seq were performed according to a previously published protocol [11]. Antibody binding to each epitope was compared to a set of mock immunoprecipitations. Significant reactivity was defined as fold change greater than 4 and P value less than .001. For additional details see Supplementary Methods.
Anti-SARS-CoV-2 Immunoglobulin Measurement
Total immunoglobulin G (IgG) directed against SARS-CoV-2 nucleocapsid (anti-N) and full-length ancestral spike (anti-S) were measured via the Meso Scale Diagnostics (MSD) V-PLEX COVID-19 Coronavirus Panel 3 Kit according to the manufacture's protocol at a dilution of 1:5000 [4].
Live Virus Neutralization
nAb against live ancestral SARS-CoV-2 were measured as described using the SARS-CoV-2/USA-WA1/2020 strain from BEI resources [12]. Additional details can be found in Supplementary Methods.
Statistical Analysis
Differences among net spike reactivity (the average log2 fold change of spike peptide reactivity) and unique spike reactivities between sample groups were assessed via 2-sided Wilcoxon rank sum tests. Differences in enrichment rate of individual epitopes between sample groups were calculated with Fisher exact test. Correlations between virus score versus total spike IgG and between CoV peptides, as well as between all CoV peptides in the library versus neutralization, were determined via Pearson correlation. When multiple comparisons were performed, significance testing was determined via the Benjamini-Hochberg procedure. All analyses and visualizations were performed in R.
RESULTS
Cohort Characteristics
Seventy-eight of the 81 KTRs in the CPAT trial were included in the analysis; 2 participants were excluded due to insufficient sequencing reads and 1 participant was excluded due to intercurrent SARS-CoV-2 infection. Full demographic characteristics are in Supplementary Table 1. Twelve HCs were included. Limited demographic data were collected from HCs; they were younger than the KTRs, but sex, race, and vaccine type did not significantly differ.
Third Vaccine Doses Improve Response Clonality in Kidney Transplant Recipients
Prior to third doses, only 7 of 78 KTRs had any measurable spike epitope antibody reactivity, increasing to 22 of 78 at 30 days after the third vaccine. In contrast, 100% of HCs had reactivity to at least 2 epitopes by day 30 after the third vaccine (Figure 1A). Although no participant reported SARS-CoV-2 infection prior to third vaccination, 11 KTRs and 1 HC had reactivity to nucleocapsid peptide 141–196 before the third dose. Three KTRs demonstrated positive nucleocapsid antibodies by MSD at any time point; however, there was poor correlation between PhIP-Seq anti-N reactivity and anti-N positivity, indicating likely cross-reactivity with other ubiquitous antigens (Supplementary Figure 1). The number of unique spike epitopes recognized by both KTRs and HCs increased after the third dose, but KTRs continued to recognize significantly fewer epitopes than HCs (Figure 1B). Net spike reactivity was also significantly lower in KTRs than HCs before and after the third dose (Figure 1C). Epitopes in subdomain (SD) 1–2, RBD, heptad repeat (HR) 2, and near the S1/S2 cleavage site (CS) were more frequently targeted by HCs than KTRs (Figure 1D). No epitopes were more frequently targeted by KTRs compared to HCs.
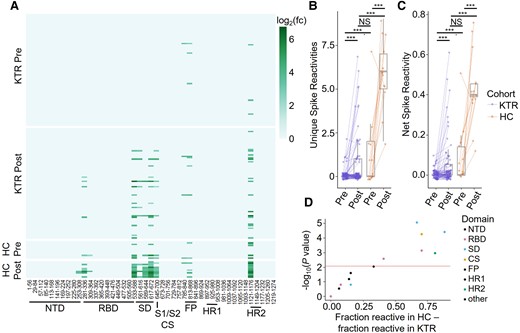
A, Heatmap of SARS-CoV-2 spike epitope reactivities for KTRs and HCs before (Pre) and 30 days after (Post) receiving a third dose of a SARS-CoV-2 mRNA vaccine. B, Number of unique spike epitope reactivities in each group. Groups were compared by Wilcoxon test. C, Net spike reactivity in each group. Groups were compared by Wilcoxon test. D, Differential reactivity to each spike epitope between HCs and KTRs after the third vaccine dose. The P value for each peptide was determined with a Fisher exact test. ***P < .001. Abbreviations: CS, cleavage site; FP, fusion peptide; HC, healthy control; HR, heptad repeat; KTR, kidney transplant recipient; log2(fc), fold change; NS, not significant; NTD, N terminal domain; RBD, receptor-binding domain; S, spike; SARS-CoV-2, severe acute respiratory syndrome coronavirus 2; SD, subdomain.
Reactivity Against ccCoVs Does Not Correlate With SARS-CoV-2 Antibody Response
To evaluate whether existing antibodies against ccCoV epitopes were associated with antibody responses to SARS-CoV-2 spike, we compared paired PhIP-Seq viral scores of the 4 ccCoVs (incorporating antibody reactivity to any antigen) before the third dose to after the third dose anti-SARS-CoV-2 spike total IgG. No significant correlations between ccCoV reactivity and anti-SARS-CoV-2 spike antibodies were found for HCs or KTRs (Figure 2A). In contrast, PhIP-Seq viral scores against SARS-CoV-2 were positively correlated with total SARS-CoV-2 spike IgG after the third dose for KTRs (Figure 2B).
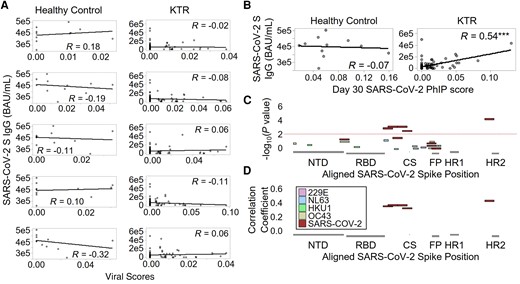
A, Viral scores for ccCoVs prior to receiving a third vaccine dose compared to total SARS-CoV-2 spike IgG after receiving a third vaccine dose for both healthy controls and KTRs. B, Viral scores of SARS-CoV-2 and total SARS-CoV-2 spike IgG were compared at the same postboost time point. C, Negative log10 P value from Pearson correlations between CoV spike epitope reactivities and NT-AUC in kidney transplant recipients. Peptides from the 4 common cold coronaviruses and SARS-CoV-2 were mapped to the SARS-CoV-2 protein. D, Pearson correlation coefficients for spike peptides that significantly correlated with NT-AUC. ***P < .001. Abbreviations: BAU, binding antibody unit; ccCoV, common cold coronavirus; CS, cleavage site; FP, fusion peptide; HR, heptad repeat; IgG, immunoglobulin G; KTR, kidney transplant recipients; NT-AUC, neutralizing titer area under the curve; NTD, N terminal domain; PhIP, phage display immunoprecipitation; RBD, receptor-binding domain; SARS-CoV-2, severe acute respiratory syndrome coronavirus 2.
Antibodies Targeting Epitopes in SD1–2, CS, and HR2 Are Most Strongly Correlated With Functional Neutralization
Epitope profiles for SARS-CoV-2 and ccCoVs were compared to neutralizing capacity using both live-virus and surrogate neutralizing assays to determine which epitope reactivities correlated with functional neutralization. No epitopes from ccCoVs were significantly correlated with neutralization using either assay, but epitopes in the RBD (533–588), SD1–2 (561–672), CS (645–700), and HR2 (1121–1176) regions of SARS-CoV-2 significantly correlated with live-virus neutralization (Figure 2C and 2D) and surrogate neutralization (Supplementary Figure 2A and 2B) of SARS-CoV-2. Several of these epitopes are outside the RBD. Therefore, correlation analysis was used to determine if reactivities to these epitopes correlated with each other. Indeed, reactivities to regions RBD (533–588), SD1–2 (561–672), CS (645–700), and HR2 (1121–1176) positively correlated with each other (Supplementary Figure 2C), suggesting that antibodies directed against these epitopes are part of a broader antiviral response. In regression analysis including the 5 peptides as predictors, the HR2 peptide and the RBD peptide have significant coefficients (P = .001 and .027, respectively).
Additional Antigen Exposure Does Not Significantly Change PhIP-Seq Profiles in Kidney Transplant Recipients
During 6 months of follow-up, 10 participants reported breakthrough infection (infected group), 28 were neither infected nor received an additional vaccine (no infection/no boost group), and 29 participants reported no infection, but did receive a fourth dose of vaccine before 180 days after the third dose (additional boost group). The demographic and clinical characteristics of these subgroups were similar aside from body mass index (BMI), which was higher among the infected group (Supplementary Table 2). Net spike reactivity scores for both the infected and additional boost groups increased or stayed the same between 30 and 180 days after the third dose, while scores for the no infection/no boost group decreased overall (Supplementary Figure 3A). Qualitatively, heatmaps for the 3 groups at the last follow-up time point appeared similar (Supplementary Figure 3B). When comparing unique spike reactivities (Supplementary Figure 3C) and net spike reactivity (Supplementary Figure 3D) there were no significant differences among the groups. There were no significant differences in reactivity of specific epitopes between the no infection/no boost group and the additional boost group (Supplementary Figure 3E). Between the infected and the no infection/no boost groups there was a trend towards more frequent targeting of an FP epitope (absolute difference of 0.39/0.40, respectively), but this did not reach statistical significance (Supplementary Figure 3F).
DISCUSSION
We report the first comprehensive anti-SARS-CoV-2 spike antibody epitope mapping for KTRs after a third dose of SARS-CoV-2 vaccine. Overall, we found poor responses to SARS-CoV-2 spike epitopes in KTRs both before and after third vaccines compared to HCs; consistent with literature demonstrating attenuated responses to SARS-CoV-2 vaccines among SOTRs [4, 13, 14]. Our data demonstrate that while KTRs are less likely to develop responses, when they do respond, they target the same epitopes within spike that are targeted by HCs. This suggests that strategies to improve immunity in this population should focus on boosting their responsiveness, potentially through adjuvants or immunosuppression reduction, but not creating novel spike constructs to push responses to key epitopes.
Reactivity against SARS-CoV-2 spike epitopes in the RBD, SD1–2, CS, and HR2 regions was closely associated with SARS-CoV-2 neutralization. This is similar to what we and others reported as immunodominant epitopes associated with neutralization in convalescent individuals [7, 11]. Reactivities to these epitopes were correlated with one another, likely indicating an overall stronger antibody response, which may confound the observed associations. Notably, reactivity in other parts of the RBD were low in both KTRs and HCs despite RBD antibodies being associated with neutralization [5]. This may be due to other RBD antibodies binding to 3-dimensional epitopes that are not well captured by PhIP-Seq.
Interestingly, we found that breakthrough infection did not significantly change net spike reactivity or unique spike reactivities compared to additional (fourth dose) boosters. This suggests that antibodies to immune-dominant spike epitopes can be elicited by either vaccination or infection.
Together these results confirm broadly attenuated spike-specific antibody responses to SARS-CoV-2 vaccination among KTRs who failed to develop robust responses to the original 2-dose series. Importantly, we did not identify a significant antigen gap in these immunosuppressed individuals, that is, KTRs can develop antibodies against key epitopes, but that due to immunosuppression and/or other factors they do so less frequently than HCs. This has important implications for vaccine design and strategy. Namely, a limited antigen vaccine, such as one containing only the RBD, is unlikely to improve responses. Rather, alternative strategies such as immunosuppression reduction, additional booster doses, or the use of adjuvanted vaccines may be more beneficial.
We previously demonstrated a negative association between antibodies against ccCoVs and SARS-CoV-2 vaccine response in vaccine-naive SOTRs [9]. In the present study, we found a negative, but not significant, association between ccCoV reactivity before the third dose and anti-SARS-CoV-2 spike total IgG after the third dose. This may indicate that antigenic imprinting can be overcome with repeated vaccination in SOTRs. Furthermore, we found no association between ccCoV reactivity and SARS-CoV-2 neutralization, adding additional evidence that ccCoV antibodies are not protective against SARS-CoV-2 [15].
This study does have limitations including explicit inclusion of KTRs who responded poorly to 2-dose mRNA vaccination and no known prior SARS-CoV-2 infections, which may reduce generalizability to the current era. Notably, however, we did not see significant differences in spike reactivity between those experiencing an additional antigen exposure through breakthrough infection or booster vaccination, as captured through a rigorous clinical trial framework. Additionally, the PhIP-Seq assay is designed to primarily detect antibodies directed against linear epitopes so there may be 3-dimensional epitopes that are differentially reactive between KTRs and HCs that were not captured by PhIP-Seq. However, other groups have demonstrated that antibodies to linear epitopes can be both potent and broad neutralizers [7].
In summary, these findings clarify that transplant recipients are capable of generating antibody response against key spike epitopes following 3-dose SARS-CoV-2 vaccination, but are less likely to do so than HCs.
Supplementary Data
Supplementary materials are available at The Journal of Infectious Diseases online (http://jid.oxfordjournals.org/). Supplementary materials consist of data provided by the author that are published to benefit the reader. The posted materials are not copyedited. The contents of all supplementary data are the sole responsibility of the authors. Questions or messages regarding errors should be addressed to the author.
Notes
Acknowledgments. The authors thank the participants of the COVID Protection After Transplant (CPAT) Study, without whom this research could not be possible; Steve Elledge for providing the CoronaScan library; and the members of the Transplant Research Center at Johns Hopkins including Diane Brown, Maggie Chahoud, Jamie Wiles, Kaitlyn Storm, and Anuj Apte.
Author contributions. A. H. K., W. R. M., W. A. W., H. B. L., and A. A. R. T. contributed conceptualization. A. H. K., W. R. M., W. A. W., C. M. D., M. A. R., C. P. L., P. S. H., H. B. L., and A. A. R. T. performed data review. A. H. K., W. R. M., and C. H. performed the formal analysis. C. M. D., D. L. S., M. A. R., C. P. L., P. S. H., W. A. W., and A. A. R. T. acquired funding. A. H. K., T. S. J., W. R. M., and A. P. performed investigations. W. R. M., A. H. K., A. P., A. A. R. T., and H. B. L. contributed methodology. A. P., C. M. D., D. L. S., W. A. W., H. B. L., and A. A. R. T contributed resources. A. H. K., W. A. W., M. A. R., C. P. L., P. S. H., H. B. L., and A. A. R. T. performed supervision. W. R. M. and C. H. contributed visualization. W. A. W., C. M. D., D. L. S., M. A. R., C. P. L., and P. S. H. contributed the trial design. A. H. K. and W. R. M. wrote the original draft. All authors reviewed and edited the manuscript.
Data availability. Proposals to access deidentified data from the CPAT trials can be submitted to the CPAT trials data coordinating center (contact: [email protected]), with transfer approved on an individual basis via formal data use agreement.
Financial support. This work was supported by the National Institutes of Health (NIH) (grant number U01 AI138897 C. M. D. and D. L. S.); and additional support from the NIH (grant numbers U01 AI134591 to C. M. D. and D. L. S., K23 AI157893 to W. A. W., R01 DK131926 to A. A. R. T., R01 GM136724 to H. B. L., and K08 AI156021 to A. H. K.).
References
Author notes
A. H. K. and W. R. M. contributed equally.
H. B. L. and A. A. R. T. are cosenior authors.
Potential conflicts of interest. D. L. S. reports receiving consulting and/or speaking honoraria from AstraZeneca, CareDx, Moderna Therapeutics, Novavax, Regeneron, Springer Publishing, Houston Methodist, Northwell Health, Optum Health Education, Sanofi, and WebMd. W. A. W. has received consulting and/or speaking fees from AstraZeneca, GlobalData, China Medical Tribune, Medical Learning Institute (CME), and advisory board fees from Novavax. A. H. K. has received consulting fees from Roche Diagnostics and Hologic, Inc; and speaking fees from PRIME Education (CME). H. B. L. is an inventor on an issued patent (US20160320406A) filed by Brigham and Women's Hospital that covers the use of PhIP-Seq for antiviral antibody detection; and is a founder of ImmuneID, Portal Bioscience, Alchemab, and Infinity Bio. All other authors report no potential conflicts.
All authors have submitted the ICMJE Form for Disclosure of Potential Conflicts of Interest. Conflicts that the editors consider relevant to the content of the manuscript have been disclosed.