-
PDF
- Split View
-
Views
-
Cite
Cite
Dylan J Tuttle, Priscila M S Castanha, Amro Nasser, Maris S Wilkins, Tamara García Galarza, Mounia Alaoui-El-Azher, Deirdre E Cuff, Prabal Chhibbar, Jishnu Das, Yijia Li, Simon M Barratt-Boyes, Robbie B Mailliard, Nicolas Sluis-Cremer, Charles R Rinaldo, Ernesto T A Marques, SARS-CoV-2 mRNA Vaccines Induce Greater Complement Activation and Decreased Viremia and Nef Antibodies in Men With HIV-1, The Journal of Infectious Diseases, Volume 229, Issue 4, 15 April 2024, Pages 1147–1157, https://doi.org/10.1093/infdis/jiad544
- Share Icon Share
Abstract
Immune dysregulation in people with human immunodeficiency virus-1 (PWH) persists despite potent antiretroviral therapy and, consequently, PWH tend to have lower immune responses to licensed vaccines. However, limited information is available about the impact of mRNA vaccines in PWH. This study details the immunologic responses to severe acute respiratory syndrome coronavirus 2 (SARS-CoV-2) mRNA vaccines in PWH and their impact on HIV-1.
We quantified anti-S immunoglobulin G (IgG) binding and neutralization of 3 SARS-CoV-2 variants of concern and complement activation in blood from virally suppressed men with HIV-1 (MWH) and men without HIV-1 (MWOH), and the characteristics that may impact the vaccine immune responses. We also studied antibody levels against HIV-1 proteins and HIV-1 plasma RNA.
MWH had lower anti-S IgG binding and neutralizing antibodies against the 3 variants compared to MWOH. MWH also produced anti-S1 antibodies with a 10-fold greater ability to activate complement and exhibited higher C3a blood levels than MWOH. MWH had decreased residual HIV-1 plasma viremia and anti-Nef IgG approximately 100 days after immunization.
MWH respond to SARS-CoV-2 mRNA vaccines with lower antibody titers and with greater activation of complement, while exhibiting a decrease in HIV-1 viremia and anti-Nef antibodies. These results suggest an important role of complement activation mediating protection in MWH.
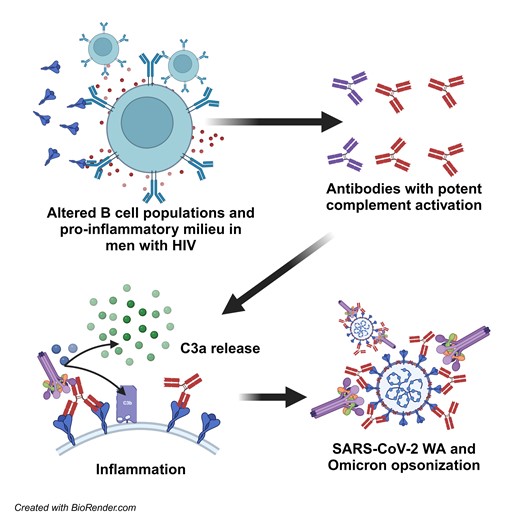
The COVID-19 mRNA vaccines Pfizer-BioNTech BNT162-b2 and Moderna mRNA-1273 are effective in preventing severe coronavirus disease 2019 (COVID-19) [1–3]. Nevertheless, there is a gap in the understanding of how these vaccines perform in people with human immunodeficiency virus-1 (PWH), who tend to respond suboptimally to conventional vaccines even with virologic control and normal CD4+ T cell counts. For example, CD4+ T cells from PWH produce less tumor necrosis factor-α (TNF-α) and interleukin 2 (IL-2) to hepatitis B virus vaccines [4], and require additional doses to induce similar seroconversion in people without human immunodeficiency virus-1 (HIV-1) [5]. Influenza vaccination induces lower immunoglobulin G (IgG) levels and memory B cell activation in PWH [6]. Similarly, severe acute respiratory syndrome coronavirus 2 (SARS-CoV-2) vaccination induces lower immunoglobulin levels in PWH [7], particularly during advanced HIV-1 disease [8].
In addition, little information exists regarding COVID-19 vaccine effects on the latent HIV-1 reservoir. Indeed, other vaccines have been shown to cause secondary transient increases in HIV-1 virus replication in PWH [9–13], indicating that these vaccines may influence HIV-1 viral replication to a degree, which could have an impact on the HIV-1 immune responses anti-Gag, anti-Nef, and anti-Env IgG on the baseline inflammatory state. Furthermore, it is known that complement activity is associated with development of severe COVID-19 outcomes [14, 15], and that virally suppressed PWH have elevated complement activation, evidenced by increased C3a and soluble membrane attack complex components in blood [16, 17]. In contrast, antibody-dependent complement activation may play an important role in mediating effective protection against disease caused by SARS-CoV-2 variants that have evaded binding of neutralizing antibodies (nAbs).
Here, we studied the humoral immune response elicited by SARS-CoV-2 mRNA vaccines and their effects on HIV-1 reservoirs in men with HIV-1 (MWH) enrolled in the MACS/WIHS Combined Cohort Study (MWCCS), a long-term natural history study of HIV-1 infection [18]. We investigated the impact of patient age, vaccine type, and time after vaccination on vaccine immune responses against SARS-CoV-2 protein variants, as well as changes in baseline proinflammatory complement activation after immunization. Residual plasma HIV-1 viremia and the latent proviral reservoir in CD4+ T cells was also investigated.
METHODS
Participants and Clinical Samples
Serum and plasma samples were collected at the Pittsburgh clinical research site of the MWCCS. All participants provided informed consent and the study was approved by the University of Pittsburgh Institutional Review Board.
ELISA Assays
Serum samples were evaluated for IgG and IgA binding to SARS-CoV-2 S1 (SinoBiological) and N (RayBiotech) proteins, the 4 human common cold coronaviruses (NL63, 229E, OC43, and HKU1; SinoBiological) [14] and HIV-1 Nef, p24, and gp120 using indirect enzyme-linked immunosorbent assays (ELISAs) described further in the Supplementary Material. The Euroimmun anti-cytomegalovirus (CMV), anti-(Epstein-Barr Virus capsid antigen[EBV-CA]), and anti-influenza A virus ELISA kits were used as per the manufacturers specifications to quantify IgG against these 3 viruses in patient serum samples.
Neutralization Assay for SARS-CoV-2
Plaque reduction neutralization tests (PRNTs) were performed as described previously [14], and in the Supplementary Material.
SARS-CoV-2 Anti-S Binding IgG Quantification
The Meso Scale Discovery (MSD) multiplex assay platform with the V-PLEX SARS-CoV-2 Panel was used to test total binding IgG levels in serum samples as per the manufacturer's specifications. Each sample was tested in duplicate, and measurements were averaged.
Complement Activation Assay
Complement activation was assessed using the bead-based flow cytometry assay described previously and in the Supplementary Material [19]. The results shown represent an average mean fluorescence intensity of the 3 independent experiments performed.
Measurement of C3 and C3a
Measurement of serum C3 and C3a levels was carried out as described previously [14].
Single-Copy Assays for Plasma HIV-1 RNA
RT-PCR assay with single-copy sensitivity targeting HIV-1 integrase RNA (iSCA) was used to quantify plasma viremia, as described previously [20].
Intact Proviral DNA Assay
Intact proviral DNA assay (IPDA) was carried out by Accelevir Diagnostics to quantify intact, total, and defective proviruses [21].
Statistical Analysis
Analyses (Prism software version 9.4; GraphPad) included use of nonparametric Mann-Whitney U tests and Kruskal-Wallis ANOVAs with Dunn post hoc test for continuous variables without pairing, Wilcoxon matched pairs tests for paired continuous variables, and Fisher exact tests for categorical variables. Spearman correlation was used to assess associations between 2 variables. Analyses were not corrected for confounders due to the small sample size. A P value of less than or equal to .05 was considered statistically significant.
RESULTS
First, we analyzed the demographic characteristics of the MWH and men without HIV-1 (MWOH) in our cross-sectional study. There were no significant differences between the participants demographic characteristics and sample collection times were similar in both groups (Table 1), nor was there a difference in time postvaccination (time between the second dose of the vaccines to the sample collection date). MWH had CD4+ T cell counts considered to be in the healthy range (253–1888 cells/μL), and distribution of the mRNA vaccine types was equivalent (P = .460) (Table 1) between groups. The 45 MWH and 94 MWOH who received 2 doses of either BNT-162b2 or mRNA-1273 were screened for anti-nucleoprotein (anti-N) IgG. Fifty-seven participants tested positive for anti-N IgG, indicating that they were previously infected with SARS-CoV-2. We then quantified anti-spike binding IgG (anti-S) and nAbs against the SARS-CoV-2 Washington (WA) strain, as well as AY.4 (Delta subvariant) and BA.1 (Omicron) variants from 82 vaccinated participants who tested negative for anti-N IgG.
Characteristic . | MWOH (n = 94) . | MWH (n = 45) . | P Value . |
---|---|---|---|
Ethnicity | |||
White, non-Hispanic | 76 (80.9) | 36 (80.0) | NA |
White, Hispanic | 4 (4.26) | 1 (2.22) | NA |
Black, non-Hispanic | 5 (5.32) | 4 (8.89) | NA |
Black, Hispanic | 1 (1.06) | 1 (2.22) | NA |
Asian/Pacific Islander | 1 (1.06) | 0 (0.0) | NA |
Unknown | 7 (7.45) | 3 (6.67) | NA |
Clinical characteristics, median (range) | |||
Age, y | 67 (31–84) | 62 (36–86) | .345 |
CD4 count, cells/μL | NA | 719.5 (253.0–1888) | NA |
Nadir CD4 count, cells/μL | NA | 669 (253–1215) | NA |
Viral load, copies/mL | NA | ≤20.0 (≤20.0–315) | NA |
Time postvaccination, d | 104 (12–249) | 101 (4–253) | .704 |
Anti-N IgG seropositivity | .267 | ||
Anti-N IgG+ | 42 (44.7) | 15 (33.3) | |
Anti-N IgG− | 52 (55.3) | 30 (66.7) | |
Vaccine type received | .460 | ||
mRNA-1273 | 42 (44.7) | 16 (35.6) | |
BNT-162b2 | 52 (55.3) | 28 (62.2) |
Characteristic . | MWOH (n = 94) . | MWH (n = 45) . | P Value . |
---|---|---|---|
Ethnicity | |||
White, non-Hispanic | 76 (80.9) | 36 (80.0) | NA |
White, Hispanic | 4 (4.26) | 1 (2.22) | NA |
Black, non-Hispanic | 5 (5.32) | 4 (8.89) | NA |
Black, Hispanic | 1 (1.06) | 1 (2.22) | NA |
Asian/Pacific Islander | 1 (1.06) | 0 (0.0) | NA |
Unknown | 7 (7.45) | 3 (6.67) | NA |
Clinical characteristics, median (range) | |||
Age, y | 67 (31–84) | 62 (36–86) | .345 |
CD4 count, cells/μL | NA | 719.5 (253.0–1888) | NA |
Nadir CD4 count, cells/μL | NA | 669 (253–1215) | NA |
Viral load, copies/mL | NA | ≤20.0 (≤20.0–315) | NA |
Time postvaccination, d | 104 (12–249) | 101 (4–253) | .704 |
Anti-N IgG seropositivity | .267 | ||
Anti-N IgG+ | 42 (44.7) | 15 (33.3) | |
Anti-N IgG− | 52 (55.3) | 30 (66.7) | |
Vaccine type received | .460 | ||
mRNA-1273 | 42 (44.7) | 16 (35.6) | |
BNT-162b2 | 52 (55.3) | 28 (62.2) |
Data are No. (%) except where indicated. Participants had received 2-dose vaccinated of either SARS-CoV-2 mRNA vaccine. Two-tailed Fisher Exact and Mann-Whitney U tests were used to compare between the groups. One participant had received heterologous mRNA vaccines and was excluded, and 43 MWH were used to determine nadir CD4 T cell count. Time postvaccination is the time between 2-dose immunization and sample collection.
Abbreviations: IgG, immunoglobulin G; MWH, men with HIV-1; MWOH, men without HIV-1; N, nucleocapsid.
Characteristic . | MWOH (n = 94) . | MWH (n = 45) . | P Value . |
---|---|---|---|
Ethnicity | |||
White, non-Hispanic | 76 (80.9) | 36 (80.0) | NA |
White, Hispanic | 4 (4.26) | 1 (2.22) | NA |
Black, non-Hispanic | 5 (5.32) | 4 (8.89) | NA |
Black, Hispanic | 1 (1.06) | 1 (2.22) | NA |
Asian/Pacific Islander | 1 (1.06) | 0 (0.0) | NA |
Unknown | 7 (7.45) | 3 (6.67) | NA |
Clinical characteristics, median (range) | |||
Age, y | 67 (31–84) | 62 (36–86) | .345 |
CD4 count, cells/μL | NA | 719.5 (253.0–1888) | NA |
Nadir CD4 count, cells/μL | NA | 669 (253–1215) | NA |
Viral load, copies/mL | NA | ≤20.0 (≤20.0–315) | NA |
Time postvaccination, d | 104 (12–249) | 101 (4–253) | .704 |
Anti-N IgG seropositivity | .267 | ||
Anti-N IgG+ | 42 (44.7) | 15 (33.3) | |
Anti-N IgG− | 52 (55.3) | 30 (66.7) | |
Vaccine type received | .460 | ||
mRNA-1273 | 42 (44.7) | 16 (35.6) | |
BNT-162b2 | 52 (55.3) | 28 (62.2) |
Characteristic . | MWOH (n = 94) . | MWH (n = 45) . | P Value . |
---|---|---|---|
Ethnicity | |||
White, non-Hispanic | 76 (80.9) | 36 (80.0) | NA |
White, Hispanic | 4 (4.26) | 1 (2.22) | NA |
Black, non-Hispanic | 5 (5.32) | 4 (8.89) | NA |
Black, Hispanic | 1 (1.06) | 1 (2.22) | NA |
Asian/Pacific Islander | 1 (1.06) | 0 (0.0) | NA |
Unknown | 7 (7.45) | 3 (6.67) | NA |
Clinical characteristics, median (range) | |||
Age, y | 67 (31–84) | 62 (36–86) | .345 |
CD4 count, cells/μL | NA | 719.5 (253.0–1888) | NA |
Nadir CD4 count, cells/μL | NA | 669 (253–1215) | NA |
Viral load, copies/mL | NA | ≤20.0 (≤20.0–315) | NA |
Time postvaccination, d | 104 (12–249) | 101 (4–253) | .704 |
Anti-N IgG seropositivity | .267 | ||
Anti-N IgG+ | 42 (44.7) | 15 (33.3) | |
Anti-N IgG− | 52 (55.3) | 30 (66.7) | |
Vaccine type received | .460 | ||
mRNA-1273 | 42 (44.7) | 16 (35.6) | |
BNT-162b2 | 52 (55.3) | 28 (62.2) |
Data are No. (%) except where indicated. Participants had received 2-dose vaccinated of either SARS-CoV-2 mRNA vaccine. Two-tailed Fisher Exact and Mann-Whitney U tests were used to compare between the groups. One participant had received heterologous mRNA vaccines and was excluded, and 43 MWH were used to determine nadir CD4 T cell count. Time postvaccination is the time between 2-dose immunization and sample collection.
Abbreviations: IgG, immunoglobulin G; MWH, men with HIV-1; MWOH, men without HIV-1; N, nucleocapsid.
mRNA-1273 Induced Higher Levels of IgG and nAbs Against WA and Delta Than BNT-1612b But Both Induce Low nAb Levels Against Delta and Omicron Variants
Binding IgG to the S protein was similar between the WA and Delta variants (P = .70), and the Delta and Omicron variants (P = .31; Figure 1A). However, there was a small increase in IgG binding between the WA and Omicron variants (P = .01). In contrast, nAbs titers against the Delta variant were significantly lower than those against the ancestral WA strain (P = .04), and this difference was exacerbated between the WA and Omicron variants (P < .0001; Figure 1A). We also noted that antibodies from most of the participants could not neutralize the Omicron variant. These results indicate that small differences between the SARS-CoV-2 variants within the spike receptor binding domain are sufficient to prevent antibodies elicited by the mRNA vaccines from neutralizing the virus despite high levels of IgG binding.
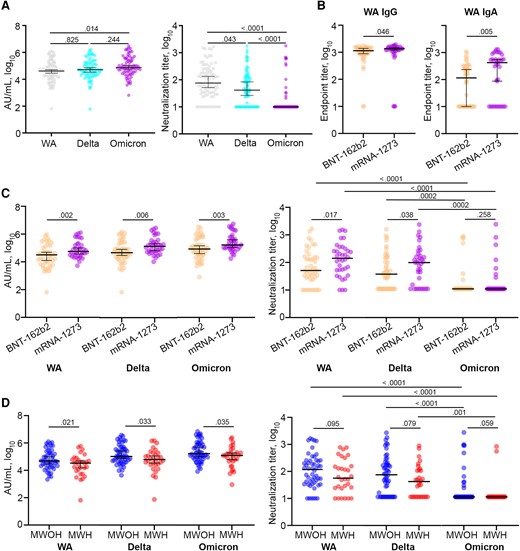
IgG binding and nAb responses elicited by COVID-19 mRNA vaccines between mRNA-1273 and BNT-162b2 vaccines in MWOH and MWH. A, Anti-spike IgG and anti-SARS-CoV-2 nAbs elicited by the mRNA vaccines against the WA, Delta, and Omicron variants were determined by MSD and PRNT, respectively (n = 82). B, Anti-spike IgG and IgA end point titers elicited by the BNT-162b2 (n = 46) and mRNA-1273 (n = 36) vaccines were determined by ELISA. C, Binding IgG and nAbs against the WA, Delta, and Omicron variants elicited by the 2 mRNA vaccines were determined by MSD and PRNT, respectively. D, Binding IgG and nAbs against the WA, Delta, and Omicron variants were determined by MSD and PRNT, respectively. All participants were 2-dose vaccinated and SARS-CoV-2 seronegative against the N antigen. Horizontal lines indicate the median and error bars 95% confidence intervals, and P values are shown above significant associations. Kruskal-Wallis ANOVA with Dunn correction was used to compare between the SARS-CoV-2 variants, and 1-tailed Mann-Whitney U tests were used to compare between MWOH (n = 52) and MWH (n = 30). Abbreviations: AU, arbitrary unit; ELISA, enzyme-linked immunosorbent assay; HIV-1, human immunodeficiency virus-1; IgG, immunoglobulin G; MSD, Meso Scale Discovery; MWH, men with HIV-1; MWOH, men without HIV-1; nAb, neutralizing antibody; PRNT, plaque reduction neutralization test; SARS-CoV-2, severe acute respiratory syndrome coronavirus 2; WA, Washington strain.
To understand the difference in the humoral response elicited by the 2 vaccines in our cohort, we compared levels of binding IgG and nAbs to the ancestral WA strain and the Delta and Omicron variants between participants who received either the mRNA-1273 or BNT-162b2 vaccine. We found that the mRNA-1273 vaccine elicited significantly higher anti-S IgG, IgA, and nAb levels against the 3 viruses assessed, consistent with other reports [22], and both vaccines elicited decreasing levels of nAbs against the Delta and Omicron variants (Figure 1B and 1C).
Virologically Controlled MWH Below Age 60 Years Elicit Lower Anti-S IgG and Neutralizing Antibody Titers Than MWOH of the Same Age to SARS-CoV-2 mRNA Vaccines
We analyzed the levels of anti-S IgG and nAbs against the WA, Delta, and Omicron variants in serum samples from 30 MWH and 52 MWOH who were fully vaccinated and had not been previously infected with SARS-CoV-2. MWH had significantly lower levels of binding IgG to the 3 variant S proteins (Figure 1D) and lower nAbs against the WA and Delta variants (Figure 1D). Neutralizing titers in both MWH and MWOH also declined significantly among the 3 variant S proteins. There was a positive association between the participant's prevaccination and postvaccination CD4+ T cell counts and nAb titers (Supplementary Figure 1A and 1B); however, the effect was small but consistent with the range of CD4+ T cells of the cohort. Also, binding IgG and nAbs against the 3 variants were negatively associated with glucose levels, cholesterol, and triglycerides in MWOH, whereas these variables positively correlated with IgG and nAbs in MWH (Supplementary Figure 1C and 1D). Binding IgG and nAbs against the 3 strains in MWOH who were fully vaccinated were not significantly different from titers of these antibodies in MWOH who had hybrid immunity. However, there was a trend for titers of binding IgG and nAbs to increase in MWH with hybrid immunity, composed of vaccination and natural infection (Supplementary Figure 2A), suggesting hybrid immunity impacts MWH to a greater degree (Supplementary Figure 2B). We further explored the dynamics of the humoral response with regards to participant age and found that age was significantly negatively associated with binding IgG and nAbs (Figure 2A and 2B). Overall, there was a 1 log10 decline in the antibody binding and neutralization levels in both MWH and MWOH. However, MWH younger than 60 years had a lower antibody response compared to MWOH of the same age (Figure 2A and 2B), particularly for nAbs against the WA and Delta viruses (P = .03 for both, data not shown). Antibody binding levels were reduced in MWOH and MWH (Figure 2C) by approximately 1 log10 over 6 months after the last immunization for all 3 SARS-CoV-2 strains. In contrast, nAbs levels showed a positive trend increasing over time (Figure 2D). This increase in neutralization titers with time could be reflective of clonal selection and affinity maturation of the antibodies, given that anti-S IgG positively correlates with nAb titers (data not shown). This suggests that the reduced level of protection against symptomatic disease is related to viral strain differences and specificity of the host antibodies rather than decay of neutralizing titers for the WA vaccine strain.
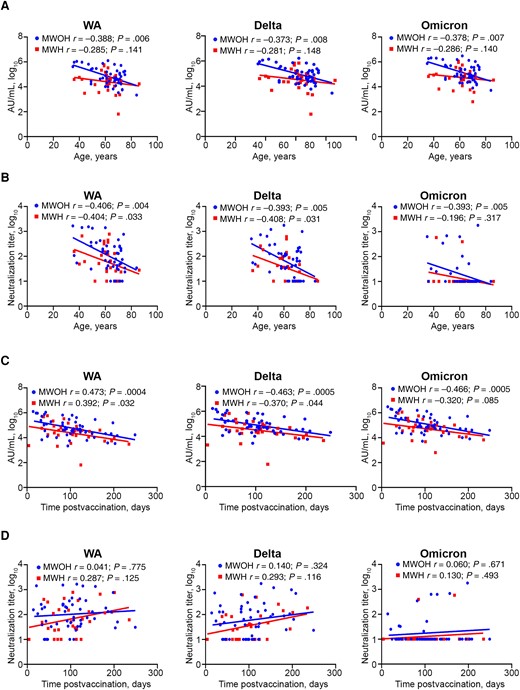
Binding IgG and nAb kinetics between MWOH and MWH with respect to age and time postvaccination. Binding IgG and nAbs against the WA, Delta, and Omicron variants were determined by MSD and PRNT, respectively. Age (A and B) and time postvaccination (C and D) were plotted against binding IgG (A and C) and nAbs (B and D) for MWOH (n = 52) and MWH (n = 30). All participants were 2-dose vaccinated and SARS-CoV-2 seronegative against the N antigen. Two-tailed Spearman correlations were used to calculate correlation coefficients for MWOH and MWH. Abbreviations: AU, arbitrary units; HIV-1, human immunodeficiency virus-1; IgG, immunoglobulin G; MSD, Meso Scale Discovery; MWH, men with HIV-1; MWOH, men without HIV-1; nAb, neutralizing antibody; PRNT, plaque reduction neutralization test; SARS-CoV-2, severe acute respiratory syndrome coronavirus 2; WA, Washington strain.
Preexisting Antibody Levels Against Endemic Human Coronaviruses Impact the Humoral Response to the SARS-CoV-2 mRNA Vaccines
It has been postulated that preexisting immunity to the endemic human coronaviruses (HKU1, NL63, OC43, and 229E) may affect the immunoglobulin response to the SARS-CoV-2 mRNA vaccines. We found that binding IgG against the WA, Delta, and Omicron variants at the postvaccination time point had a negative trend with IgG levels against the S protein of at least 1 of the 4 human coronaviruses in MWOH and MWH (Supplementary Figure 3A and 3B). Likely, this reflects competition for B-cell binding to epitopes that are similar among the coronavirus family, which was significant for 2 of the endemic coronaviruses in MWOH. Interestingly, we found a positive correlation between nAbs against the WA and Delta SARS-CoV-2 variants, and anti-S IgG against the 4 human coronaviruses in MWOH and MWH (Supplementary Figure 3A and 3B). The strength of the associations between binding IgG and human coronavirus IgG did not differ between MWOH and MWH. However, nAbs against the Delta variant showed a stronger association with HKU1, NL63, and 229E anti-S IgG levels in MWH (Supplementary Figure 3B). This implies that cross-reactivity between at least these 3 human coronaviruses have only a small interference with the SARS-CoV-2 antibody response. Nevertheless, it should remain a topic of interest because it may have implications for the immune responses of future SARS-CoV-2 variants or to post-COVID complications.
Complement Activation is Elevated in SARS-CoV-2 Vaccinated MWH
PWH have a chronic inflammatory state that could impact the capacity of the vaccine-induced antibodies to activate the classical complement cascade. We selected serum from 10 MWOH and 10 MWH who had comparable anti-S1 IgG end point titers (P = .739; Figure 3A) and measured complement fixation ability using a bead-based flow cytometry assay. We found that antibodies from MWH had a greater ability to activate classical complement than MWOH for both the WA S1 and Omicron S1 antigens (P = .009 and .003, respectively; Figure 3B). These data suggest that other effector functions of these antibodies, such as complement fixation and opsonization, can act to compensate for a lower nAb response and have a strong effect on a wide range of SARS-CoV-2 variants. In addition, we quantified endogenous levels of C3 and C3a in serum from 19 MWH and 20 MWOH at prevaccine and postvaccine time points to assess vaccine impact on complement activity. MWH presented elevated levels of C3a (P = .0002; Figure 3C) and lower levels of C3 postvaccination (P = .002; Figure 3C), leading to an increasing C3a/C3 ratio in these participants (P < .0001; Figure 3C), while in MWOH the C3 levels and the C3a/C3 ratio remained constant (Figure 3C). However, we noted that C3a showed a small but significant change postvaccination (P = .001). Furthermore, we found that MWH had greater levels of C3a at the postvaccine time point than MWOH (P = .048; Figure 3D) and a 2-fold greater increase in C3a between the time points (P = .001; Figure 3D). Taken together, these findings are consistent with the notion that immunization increased the baseline complement activity present in MWH. In addition, it suggests that the proinflammatory environment present in MWH favored the generation of antibodies with greater capacity for antibody-mediated complement activation. To investigate this, we quantified titers of anti-S1 IgG1, IgG2, IgG3, and IgG4 in the same 19 MWH and 20 MWOH. Interestingly, we did not find a significant difference in relative abundance of the IgG subclasses; however, we did note that MWOH had a greater IgG1/IgG2 ratio than MWH (Figure 3D), indicating that the immune response in MWOH has a strong TH1 character, but the response is less TH1 in MWH. Finally, we found that the C3a/C3 levels were not significantly impacted by time postimmunization in MWOH (Figure 3D), while there was a strong positive association in MWH (Figure 3D).
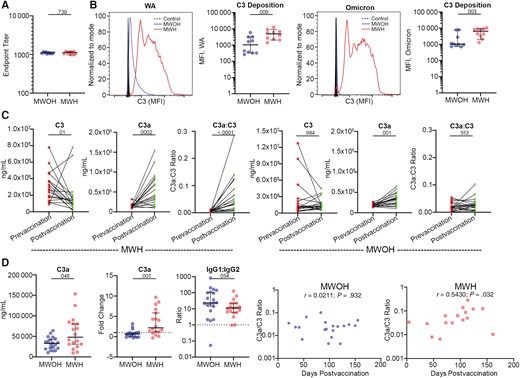
Complement activity in SARS-CoV-2 mRNA vaccinated MWOH and MWH. A, Anti-S1 end point titers between MWOH (n = 10) and MWH (n = 10) selected for complement activation assay. B, Histograms showing the C3 deposition of 1 MWOH and 1 MWH participant compared to the control, and C3 deposition by antibodies from MWOH (n = 10) and MWH (n = 10), matched by anti-S1 IgG end point titer, for the WA and Omicron variants determined by flow cytometry. C, MWH serum levels of C3 (n = 19), C3a (n = 18), and the ratio C3a:C3 (n = 18) and MWOH serum levels of C3 (n = 19), C3a (n = 19), and the ratio C3a:C3 (n = 19) were determined by capture ELISA. D, Serum C3a levels postvaccine, C3a fold change between pre- and postvaccine, and IgG1:IgG2 ratio postvaccine were determined by ELISA and compared between MWOH (n = 20) and MWH (n = 19). C3a:C3 ratios were plotted against time postvaccination for MWOH (n = 19) and MWH (n = 16). C and D, All participants were 2-dose vaccinated and SARS-CoV-2 seronegative against the N antigen. Horizontal lines indicate the median and error bars 95% confidence intervals, and P values are shown above significant associations. Two-tailed Mann-Whitney U and Wilcoxon matched pairs tests were used to compare between the groups, and Spearman correlations were used to correlated between variables. Abbreviations: ELISA, enzyme-linked immunosorbent assay; HIV-1, human immunodeficiency virus-1; IgG, immunoglobulin G; MFI, mean fluorescence intensity; MWH, men with HIV-1; MWOH, men without HIV-1; SARS-CoV-2, severe acute respiratory syndrome coronavirus 2; WA, Washington strain.
COVID-19 Vaccination Decreases Residual HIV-1 Viral Load and Anti-Nef IgG in MWH
Previous reports showed that COVID-19 vaccines triggered an elevation of HIV-1 viremia for a short period and increased the anti–HIV-1 CD8+ T-cell population. Because the MWH in our cohort had HIV-1 RNA below the normal detection level of 20 copies/mL, we used a single-copy HIV-1 RNA assay (iSCA) to quantify plasma viremia from the pre- and postvaccination time points of 19 MWH who had not been exposed to SARS-CoV-2 infection to corroborate previous reports suggesting a change in HIV-1 viremia following mRNA vaccination [17]. We found that residual HIV-1 viremia decreased significantly between the prevaccine and postvaccine time points (median 100 days; range 4–235 days; P = .004; Figure 4A). Indeed, HIV-1 RNA levels in many of these participants became undetectable by the iSCA assay (101; 4–251 days; Table 1). In investigating a potential mechanism for the decline in viral load, we chose to measure anti-Nef IgG as a proxy for increased anti–HIV-1 immune response, as it has been previously documented that the mRNA vaccines can induce activation of Nef-specific CD8+ T cells [23]. Surprisingly, the drop in the residual plasma RNA was concomitant with a significant drop in anti-Nef IgG in the same participants (P < .0001; Figure 4A), while IgG levels against p24 and gp120 did not change between the pre- and postvaccine time points (P = .388 and .870, respectively; Figure 4A). We also investigated if the levels of anti-CMV, anti-EBV, and influenza A virus IgG changed postvaccination, and found there was no significant difference (Figure 4B), suggesting that the observed decay of antibodies was specific to the Nef protein.
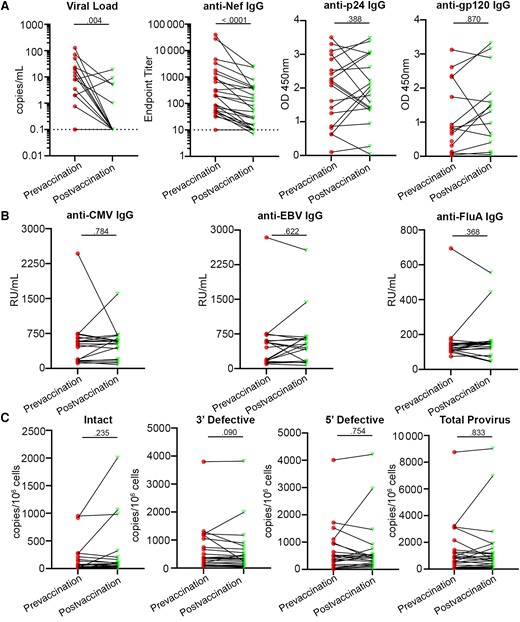
HIV-1 virologic response to the SARS-CoV-2 mRNA vaccines. A, Residual HIV-1 viremia (n = 19), anti-Nef IgG levels (n = 26), anti-p24 IgG levels, and anti-gp120 IgG levels; and (B) anti-CMV, anti-EBV, and anti-influenza A IgG levels were determined by ELISA, in participants with HIV-1. C, Assessment of intact (n = 23), 3′ defective (n = 23), 5′ defective (n = 25), and total (n = 25) proviral HIV-1 DNA was done using IPDA. All participants were 2-dose vaccinated and SARS-CoV-2 seronegative against the N antigen in all but the IPDA analysis. Two-tailed Wilcoxon matched pairs tests were used to compare between pre- and postvaccine time points. Abbreviations: CMV, cytomegalovirus; EBV, Epstein-Barr virus; ELISA, enzyme-linked immunosorbent assay; HIV-1, human immunodeficiency virus-1; IgG, immunoglobulin G; IPDA, intact proviral DNA assay; OD, optical density; SARS-CoV-2, severe acute respiratory syndrome coronavirus 2; RU, relative units.
We also quantified proviral DNA in CD4+ T cells between the prevaccine and postvaccine time points by IPDA. We found that there was no significant difference between time points in the amount of intact provirus detected (Figure 4C). We also did not find a significant difference in 3′ and 5′ defective provirus between time points (Figure 4C) and, consequently, the amount of total provirus in these participants remained constant (Figure 4C). These results suggest that there was no detectable change in the HIV-1 proviral reservoir following SARS-CoV-2 mRNA vaccination, even though HIV-1 viremia and Nef-specific IgG decreased.
DISCUSSION
We found that in MWH, BNT-162b2 and mRNA-1273 vaccines elicited similar titers of binding IgG to the Delta and Omicron variants when compared to the ancestral WA strain, consistent with previous reports [24]. Although vaccine-induced antibodies in MWOH and MWH bound at similar levels to the viruses tested, antibody binding did not correlate with neutralization of the Delta and Omicron variants. Furthermore, mRNA-1273 induced substantially greater antibody titers against the 3 viruses we investigated. Importantly, we found that the vaccines behave differently in MWH on antiretroviral therapy compared to MWOH with similar demographic characteristics and risk factors. Not only are IgG and nAb titers lower in MWH consistent with previous reports [25], but we also found that preexisting antibody levels against endemic coronaviruses appear to impact the humoral immune response elicited by these vaccines in MWOH and MWH.
Age was a significant factor determining levels of antibody binding and nAbs. There was a 1 log10 decline between participants aged 40 and 80 years. Moreover, MWH younger than 60 years had lower antibody responses than MWOH of the same age, potentially a sign of precocious immune senescence. This is to be expected as it is well documented that older individuals have reduced vaccine efficacy, which can be compounded by HIV-1 infection [26, 27]. Antibody binding decayed approximately 1 log10 over 6 months postimmunization in MWOH and MWH, whereas neutralization capacity for the vaccine-homologous viral strain was stable. This result suggests that protection mediated by these vaccines against the WA strain does not fall after 6 months and the reduced levels of protection over time and increased breakthrough infections are mainly due to variants that have immunologically escaped nAbs.
It is well established that chronic HIV-1 infection impacts the T-cell and B-cell landscape and functionality [28]. It is also known that B cells activated because of HIV-1 infection restrict the availability of naive B cells to respond to novel infections [29]. This could partially explain the lower antibody titers in MWH, evidenced by the stronger associations with Delta nAb titer and anti-S IgG against 3 of the 4 human coronaviruses. We also noted that infection with SARS-CoV-2 can increase the levels of anti-S protein antibodies in MWH to similar levels as MWOH. It has been reported that PWH are more likely to develop severe COVID-19 when infected with SARS-CoV-2 compared to PWOH [30], and these lower immunoglobulin responses correlate with less protection and more severe clinical outcomes in vaccinated MWH with advanced immunosuppression [31].
Our study is the first to investigate antibody-dependent complement activation of vaccine-elicited antibodies in MWH. Complement activation is important for clearance of infections, but these pathways have also been implicated in exacerbating the severity of COVID-19 [14, 32]. We found that antibodies from MWH were more potent for C3 deposition on WA and Omicron strains when compared to MWOH with the same anti-S IgG titers, and MWH had a greater induction of C3a. Indeed, PWH are known to have greater levels of complement factor C1q-C4 in circulation related to the chronic inflammation caused by HIV-1 [33], but how this relates to greater complement activation through vaccine-induced antibodies is unclear. It is possible that the enhanced complement activation is a double-edged sword: it may compensate for the lower nAb response, while aggravating the proinflammatory environment generated by chronic HIV-1 [16], and exacerbating existing chronic comorbidities.
To address underlying HIV-1 infection in response to mRNA vaccination, we studied 19 MWH for an average of 100 days (range, 4–235 days) postvaccination. We found a significant reduction (P = .004) in residual viral RNA in blood detected by a single-copy RNA assay within an 8-month period after COVID-19 vaccination in PWH, with a decrease in HIV-1 RNA levels in 17 of these 19 participants. This was not linked to an alteration in intact proviral DNA in CD4+ T cells. These results contrast with results that have found an increase in viral load following vaccination within a shorter period of 15 days to 3 months [9–13]. However, these results are not mutually exclusive and suggest that the initial elevation of cellular viral antigens boosts the anti–HIV-1 cellular immune responses and provides a better control of virus-producing cells. Additionally, we use anti-Nef IgG as a proxy for measuring the anti–HIV-1 response, as it has been reported that Nef-specific CD8+ T-cell responses become activated following COVID-19 mRNA vaccination [23]. We postulated that HIV-1 viremia undergoes a “blip” following vaccination that is followed by a decline due to death of infected CD4+ T cells beginning to express viral proteins. Unexpectedly, we found levels of anti-Nef IgG decreased (P < .0001) within 8 months postvaccination in our participants but we did not find the same decrease in anti-p24 and anti-gp120 IgG. It is unclear whether this will correspond to impaired control of HIV-1 viremia after longer periods, as lower anti-Nef IgG correlates with faster disease progression [34]. Alternatively, the reduction of anti-Nef antibodies could be due to lower circulating residual levels of Nef, resulting from the lower HIV-1 replication following vaccination. Further investigation into levels of Nef protein in participant serum is ongoing to test these hypotheses.
The conclusions of our study are limited to men with HIV, as only immune responses from men were analyzed due to the historical enrollment of men in the Pittsburgh Clinical Research Site of the MWCCS. Investigation into the immune responses of women with HIV is important, as women may have higher antibody titers than men, causing greater relative complement activation [35]. Further investigations in women are currently ongoing.
Overall, our study provides further compelling evidence that the SARS-CoV-2 mRNA vaccines elicit differential responses in MWOH and MWH. We also report an increased ability for vaccine-induced antibodies in MWH to activate classical complement and that this mechanism may contribute to protection against disease. Interestingly, we have noted a decline in residual HIV-1 RNA in the plasma from MWH who are mRNA vaccinated, while there was no change in the latent viral reservoir. These results lead to the conclusion that virally controlled MWH over 40 years of age should be given special attention and be recommended to be a priority to receive new vaccines containing antigens from new SARS-CoV-2 strains.
Supplementary Data
Supplementary materials are available at The Journal of Infectious Diseases online (http://jid.oxfordjournals.org/). Supplementary materials consist of data provided by the author that are published to benefit the reader. The posted materials are not copyedited. The contents of all supplementary data are the sole responsibility of the authors. Questions or messages regarding errors should be addressed to the author.
Notes
Acknowledgments. We thank and acknowledge the participants of the MACS/WIHS Combined Cohort Study for their continued involvement in the Pitt Men's Study; and Kathy Kulka and Kathy Hartle for assisting with the investigation.
Author contributions. E. T. A. M., C. R. R., R. B. M., N. S. C., and D. J. T. conceptualized and designed the study and wrote the protocol. D. J. T. and E. T. A. M. wrote the first draft of the manuscript and referred to appropriate literature and were the main authors responsible for formal data analysis. D. E. C. was responsible for data curation. D. J. T., E. T. A. M., R. B. M., N. S. C., C. R. R., A. N., M. S. W., P. M. S. C., and S. M. B. B. revised the manuscript content and reviewed and edited the manuscript. D. J. T., P. M. S. C., A. N., and M. S. W. performed all the serology tests and neutralization assays. D. J. T., A. N., and T. G. G. performed the complement assays. M. A. E. A. performed all virology measurements. Y. L., J. D., and P. C. offered statistical consulting and data analysis for the manuscript. All authors agreed with and approved the final version of the manuscript.
Data sharing. Deidentified patient data and a data dictionary are available following publication upon request to the corresponding author. Proposals will be reviewed and approved by the investigator and collaborators based on scientific merit. After approval of a proposal, data can be shared through a secure online platform after signing a data access agreement.
Financial support. This work was supported by the National Institutes of Health (NIH) National Research Service Award (grant number 5T32AI065380-15 to D. J. T.); NIH National Institute of Allergy and Infectious Diseases (NIAID) (grant number R01AI167711 to C. R. R.); NIH National Heart, Lung, and Blood Institute (NHLBI) (grant number 5U01HL146208 to C. R. R.); Office of AIDS Research (OAR/NHLBI) supplement to grant U01HL146208-03S2/S3 (to C. R. R.); and the Rustbelt Center for AIDS Research (NIAID grant number P30AI036219). T. G. G. was supported by a Centers for AIDS Research Diversity, Equity, and Inclusion Pathway Initiative supplement to NIAID grant P30AI036219.
References
Author notes
Presented in part: Immunology 2023, American Association of Immunologists, Washington, DC, May 11–13, 2023; and International Society for Vaccines Annual Congress, Lausanne, Switzerland, October 22–24, 2023.
Potential conflicts of interest. All authors: No reported conflicts of interest. All authors have submitted the ICMJE Form for Disclosure of Potential Conflicts of Interest. Conflicts that the editors consider relevant to the content of the manuscript have been disclosed.