-
PDF
- Split View
-
Views
-
Cite
Cite
Ping Zheng, Fang Liu, Jinzhao Long, Yuefei Jin, Shuaiyin Chen, Guangcai Duan, Haiyan Yang, Latest Advances in the Application of Humanized Mouse Model for Staphylococcus aureus, The Journal of Infectious Diseases, Volume 228, Issue 6, 15 September 2023, Pages 800–809, https://doi.org/10.1093/infdis/jiad253
- Share Icon Share
Abstract
Staphylococcus aureus (S. aureus) is an important pathogen for humans and can cause a wide range of diseases, from mild skin infections, severe osteomyelitis to fatal pneumonia, sepsis, and septicemia. The mouse models have greatly facilitated the development of S. aureus studies. However, due to the substantial differences in immune system between mice and humans, the conventional mouse studies are not predictive of success in humans, in which case humanized mice may overcome this limitation to some extent. Humanized mice can be used to study the human-specific virulence factors produced by S. aureus and the mechanisms by which S. aureus interacts with humans. This review outlined the latest advances in humanized mouse models used in S. aureus studies.
Staphylococcus aureus (S. aureus), a gram-positive bacterium, is a dangerous pathogen of humans and livestock that can cause both community- and hospital-acquired infections, which result in substantial morbidity and mortality. S. aureus infections range from relatively harmless localized skin infections to life-threatening systemic conditions, including bacteremia, sepsis, and septicemia [1–4]. S. aureus is also notorious for frequently causing reinfection with the same strain, suggesting natural infections hardly induce acquired immunity [5–8]. In addition, antibiotic resistance to S. aureus is a growing threat to clinical treatment [9]. In particular, methicillin-resistant S. aureus (MRSA) is a major problem, not only in the hospital setting but also in the community, causing a significant economic burden. In contrast to hospital-acquired strains, community-acquired strains of S. aureus infect otherwise healthy individuals. The MRSA strain USA300 has been the predominant cause of community-associated MRSA (CA-MRSA) infections, which infects healthy, hospitalized, and postinfluenza patients in the context of pneumonia [10, 11]. Also of concern is the fact that MRSA is often resistant to multiple antibiotic classes and will continue to generate resistance to any antibiotic developed in the future [12]. Therefore, there is an urgent need to develop vaccines to target multidrug-resistant S. aureus strains. However, both active and passive immunization strategies have thus far failed to show efficacy in humans [5, 13].
Mouse models have been used frequently to study the pathogenesis and the specific host pathway of S. aureus infections, as well as the role of virulence factors [14]. Mice are the backbone of in vivo immune experiments and reflect human biology quite well in many aspects [15]. A number of S. aureus-infected mouse models have already been established, such as gastrointestinal infections [16], pneumonia [17], sepsis [18], skin and soft tissue infections (SSTI) [19], osteomyelitis [20], joint infections [21], endocarditis [22], and nasal colonization [23]. These models expand our knowledge in not only host-pathogen interaction in S. aureus infections but also potential therapeutic strategies for S. aureus infections. However, conventional mouse models still have many inevitable limitations [24]. The mouse is not a natural host for human clinical S. aureus isolates and requires genetic adaptation to cause transmissible diseases in mice, which highlights how caution is required when extrapolating results from mice studies to the clinic [25, 26]. Mice and humans differ significantly in innate and adaptive immune systems and cardiovascular systems, which leads to a major drawback in the mouse models of S. aureus infections [15, 27]. Specifically, mice neutrophils do not express defensins, whereas neutrophils are an abundant source of human leukocyte defensins [15]. Numerous S. aureus vaccine candidates showed promising results in conventional mouse models but failed to offer protection in human clinical trials, which means mice lack the necessary components to truly model S. aureus infections [28, 29]. Humans are colonized with S. aureus early in life, while experimental mice are not, which may account for the vaccine failure [30]. Additionally, host specificity of several S. aureus virulence factors, such as the bicomponent leucocidin which interacts with human-specific receptors, makes their analysis impossible in conventional mice [2, 31]. Some S. aureus virulence factors preferentially target the human version of their receptors, such as Panton-Valentine leukocidin (PVL), leukocidin AB (LukAB), and γ-hemolysin CB (HlgCB) [10]. It is known that neutrophils play an important role in controlling S. aureus infections, and the cytotoxic activity of PVL, LukAB, HlgCB, and HlgAB in mice neutrophils is lower than that in human neutrophils [32]. Other reasons, such as the complete lack of susceptibility to low concentrations of α-toxin in mice, differences in the composition of the gut and vaginal microbiota of mice and humans, and the need for more colony-forming units to induce the same infection level in mice as in humans, all call for the increased use of humanized mice [27].
Humanized mice have emerged as an attractive small animal model to simulate human diseases [33]. They consist of immunodeficient mice xenotransplanted with human cells or tissues and/or expressing human gene products [34], allowing human-specific pathogens to infect and replicate within them, and can develop functional human-specific immune responses to an array of diseases [35]. Various human cells or tissues have been transplanted into mice, including immune system components, liver cells, skin tissue, pancreatic islets, to generate various types of humanized mice [36]. The most common humanized approach is to inject mice intravenously with human CD34+ hematopoietic stem cells, which give humanized mice the potential to mimic the human immune defense. Researchers have used humanized mice to study the S. aureus toxin PVL [37, 38] and LukAB [31]. According to the classification of S. aureus infection sites, there are 5 established models, including skin infections [38–42], pneumonia [43, 44], osteomyelitis and muscle infections [44–46], bloodstream infections [31], and intraperitoneal infections [47] (Figure 1). The humanized mouse model generation method applied to S. aureus infections is shown in Figure 2. Note that a review on this topic was published in 2017 [10], where 3 studies were included, mainly focusing on the models of intraperitoneal infections, SSTI, and pneumonia. Since then, 8 studies have emerged with more humanized mouse models of S. aureus infections. Hence, recent advances in the application of humanized mice for S. aureus infections are reviewed here. Table 1 summarizes the general information regarding humanized mouse models used in S. aureus infections.
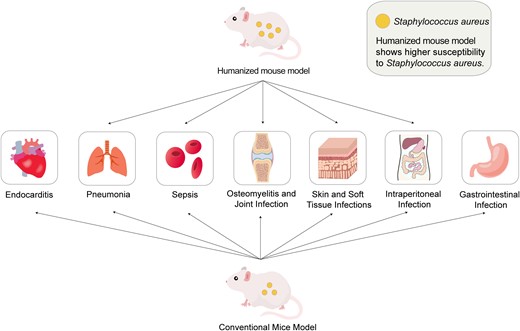
The application of conventional mouse models and humanized mouse model in Staphylococcus aureus infections.
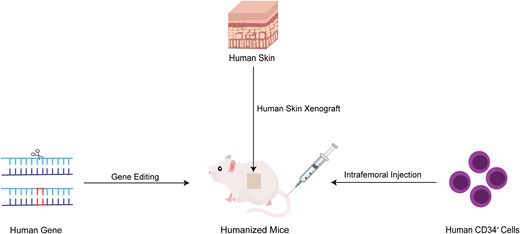
The generation of humanized mice in Staphylococcus aureus infections.
Ref. . | S. Aureus Stain . | Mouse Stain . | Humanized Manner . | Main Findings . |
---|---|---|---|---|
Skin infection model | ||||
[42] | MRSA USA300, spa null mutant, agr mutant, α-hemolysin mutants, PVL mutants, phenol-soluble modulin (Psm) (psmα, psmβ, or hld) single and triple mutants | NSG mice | Graft human neonatal foreskin | 22% of S. aureus isolated from patients with chronic infections of atopic dermatitis had an agr mutant-like phenotype. The increased intracellular persistence of toxin-deficient mutants was found. MRSA USA300 bacteria escape from the endosome to cause keratinocyte pyroptosis and WT MRSA USA300 and agr null mutant induce autophagy in human skin grafts. Induction of autophagy enhances MRSA persistence through effects on the inflammasome. |
[38] | CA-MRSA strain (CST5) and its isogenic PVL knockout strain | NSG mice | Intrahepatic injection of human CD34+ cells | Humanized NSG mice showed enhanced susceptibility to S. aureus skin and soft tissue infection. PVL induced dermopathology in the humanized NSG mouse model, which following adoptive transfer of human neutrophils. PMX53, a human C5aR inhibitor, blocked PVL-induced cellular cytotoxicity in vitro and reduced the size difference of lesions induced by the PVL+ and PVL−S. aureus, but PMX53 also reduced recruitment of neutrophils and exacerbated the infection. |
[41] | USA300 SF8300 (MRSA) | SCID.bg mice | Graft human abdominal skin | S. aureus transiently colonized the outer stratum corneum of healthy human skin. Noninvasive MRSA causes local inflammation in the underlying skin layers. Neutrophil recruitment to the skin reduced bacterial numbers on the skin surface. Neutrophils mediated the transiency of MRSA colonization. |
[39] | CA-MRSA USA300 | NSG mice, SRG rat | Cotransplants of human full-thickness fetal skin, autologous lymphoid tissues, and autologous hematopoietic stem cells | The hSIS-humanized NSG mouse model supported the development of full-thickness human skin, autologous lymphoid tissues (thymus and spleen), and human immune cells. The hSIS-humanized SRG rat model supported the development of full-thickness human skin, thymus tissues, and human immune cells. The human skin xenograft in immunodeficient rodent models supported CA-MRSA infection. |
[40] | CA-MRSA (Southwest Pacific clone, ST30, spa t019) strain, USA300 LAC (MRSA wild type, ST8, spa t008), and its isogenic mutant defective in vraR. | NOD/SCID mice | Intrahepatic injection of human CD34+ cells | Humanized mice were more sensitive to MRSA SSTI infection than BALB/c with an increased skin lesion size and bacterial burden at the infection site. Neutrophils from humanized mice were more susceptible to MRSA supernatant than that from BALB/c. There were reduction of virulence and increased susceptibility towards the human immune system in the ST30ΔvraR strain. |
Pneumonia model | ||||
[37] | S. aureus USA300 LAC, lukAB, lukS-F/PV (Δpvl) and complemented mutant (Δpvl/pvl+) | NSG mice | Intrahepatic injection of human CD34+ cells; Implantation of thymic tissue from the same donor under the kidney capsule | Humanized mice expressed large numbers of human leukocytes in the lungs, blood, and bone marrow. Severity of pulmonary infection in humanized mice with S. aureus increased. S. aureus induced cytokine expression in humanized mice. PVL expression involved in the pathogenesis of S. aureus lung infection and targeted to the human macrophage population. |
[43] | S. aureus stain Newman | C57BL/6 mice | Injection of the BAC-cloned HLA-DP401-H2-Aβ1−/− gene into mouse fertilized oocytes | Infection with S. aureus Newman did not alter the percentage of immune cells in the spleen and thymus from the HLA- DP401- H2- Aβ1 humanized mice. Increased expression of HLA- DP401 was observed in the thymus of the humanized mice infected with S. aureus. |
Intraperitoneal infection model | ||||
[47] | S. aureus strain PS80 | NSG mice | Intrahepatic injection of human CD34+ cells | S. aureus infection was aggravated in humanized mice, compared with wild-type or nonengrafted mice. The humanized mice displayed a significantly reduced survival percentage, increased weight loss, and a more rapid increase in bacterial burden. S. aureus infection induced T-cell activation, apoptosis, and Fas receptor expression in humanized but not wild-type mice. |
Osteomyelitis model | ||||
[44] | S. aureus USA300 LAC strain | NSG mice | Intrahepatic injection of human CD34+ cells | HuNSG mice had increases in weight loss, osteolysis, bacterial dissemination to internal organs, and numbers of SACs, during the establishment of implant-associated MRSA osteomyelitis compared to NSG controls (P < .05). Flow cytometry and immunohistochemistry demonstrated greater human T-cell numbers in infected versus uninfected huNSG mice (P < .05), and that T-bet+ human T cells clustered around the SACs, suggesting S. aureus-mediated activation and proliferation of human T cells in the infected bone. |
Thigh muscle infection model | ||||
[45] | S. aureus USA300 LAC*lux | NSG mice | Intrahepatic injection of human CD34+ cells | Humanized mice proved not only to be more susceptible to MRSA infection than wild-type or “murinized” mice, but displayed furthermore inferior survival and signs of systemic infection in an otherwise localized infection model. Human and murine cytokine levels in blood and at the primary site of infection were strongly elevated in huNSG mice compared to all control groups. Differences in human and murine immune cell lineages surfaced during the infection, with human monocyte and B-cell numbers in blood and bone marrow being significantly reduced at the later time point of infection. Murine monocytes in contrast behaved conversely by increasing cell numbers. |
[46] | MRSA LAC*lux | NSG mice, NSG-SGM3 mice | Intrahepatic injection of human CD34+ cells | Humanized NSG-SGM3 mice showed strong human immune cell engraftment and develop anemia during prolonged course of humanization. MRSA thigh infection led to strongly reduced survival of huSGM3 mice. Humanized mice showed a tendency towards higher numbers of S. aureus in the respective organs. Stronger response of human immune cells in humanized NSG-SGM3 than in humanized NSG mice was found. The stronger the humanization, the higher the vulnerability to S. aureus infection. |
Bloodstream infection model | ||||
[31] | S. aureus USA300 LAC | C57BL/6 mice | Using CRISPR-mediated gene editing to encode a humanized LukAB-binding region in the murine CD11b I-domain. | LukAB binding to the CD11b I-domain is species specific and CD11b I-domain is subject to positive selection in primates and rodents. Humanizing CD11b renders mice susceptible to S. aureus infection, a phenotype mediated by LukAB. |
Ref. . | S. Aureus Stain . | Mouse Stain . | Humanized Manner . | Main Findings . |
---|---|---|---|---|
Skin infection model | ||||
[42] | MRSA USA300, spa null mutant, agr mutant, α-hemolysin mutants, PVL mutants, phenol-soluble modulin (Psm) (psmα, psmβ, or hld) single and triple mutants | NSG mice | Graft human neonatal foreskin | 22% of S. aureus isolated from patients with chronic infections of atopic dermatitis had an agr mutant-like phenotype. The increased intracellular persistence of toxin-deficient mutants was found. MRSA USA300 bacteria escape from the endosome to cause keratinocyte pyroptosis and WT MRSA USA300 and agr null mutant induce autophagy in human skin grafts. Induction of autophagy enhances MRSA persistence through effects on the inflammasome. |
[38] | CA-MRSA strain (CST5) and its isogenic PVL knockout strain | NSG mice | Intrahepatic injection of human CD34+ cells | Humanized NSG mice showed enhanced susceptibility to S. aureus skin and soft tissue infection. PVL induced dermopathology in the humanized NSG mouse model, which following adoptive transfer of human neutrophils. PMX53, a human C5aR inhibitor, blocked PVL-induced cellular cytotoxicity in vitro and reduced the size difference of lesions induced by the PVL+ and PVL−S. aureus, but PMX53 also reduced recruitment of neutrophils and exacerbated the infection. |
[41] | USA300 SF8300 (MRSA) | SCID.bg mice | Graft human abdominal skin | S. aureus transiently colonized the outer stratum corneum of healthy human skin. Noninvasive MRSA causes local inflammation in the underlying skin layers. Neutrophil recruitment to the skin reduced bacterial numbers on the skin surface. Neutrophils mediated the transiency of MRSA colonization. |
[39] | CA-MRSA USA300 | NSG mice, SRG rat | Cotransplants of human full-thickness fetal skin, autologous lymphoid tissues, and autologous hematopoietic stem cells | The hSIS-humanized NSG mouse model supported the development of full-thickness human skin, autologous lymphoid tissues (thymus and spleen), and human immune cells. The hSIS-humanized SRG rat model supported the development of full-thickness human skin, thymus tissues, and human immune cells. The human skin xenograft in immunodeficient rodent models supported CA-MRSA infection. |
[40] | CA-MRSA (Southwest Pacific clone, ST30, spa t019) strain, USA300 LAC (MRSA wild type, ST8, spa t008), and its isogenic mutant defective in vraR. | NOD/SCID mice | Intrahepatic injection of human CD34+ cells | Humanized mice were more sensitive to MRSA SSTI infection than BALB/c with an increased skin lesion size and bacterial burden at the infection site. Neutrophils from humanized mice were more susceptible to MRSA supernatant than that from BALB/c. There were reduction of virulence and increased susceptibility towards the human immune system in the ST30ΔvraR strain. |
Pneumonia model | ||||
[37] | S. aureus USA300 LAC, lukAB, lukS-F/PV (Δpvl) and complemented mutant (Δpvl/pvl+) | NSG mice | Intrahepatic injection of human CD34+ cells; Implantation of thymic tissue from the same donor under the kidney capsule | Humanized mice expressed large numbers of human leukocytes in the lungs, blood, and bone marrow. Severity of pulmonary infection in humanized mice with S. aureus increased. S. aureus induced cytokine expression in humanized mice. PVL expression involved in the pathogenesis of S. aureus lung infection and targeted to the human macrophage population. |
[43] | S. aureus stain Newman | C57BL/6 mice | Injection of the BAC-cloned HLA-DP401-H2-Aβ1−/− gene into mouse fertilized oocytes | Infection with S. aureus Newman did not alter the percentage of immune cells in the spleen and thymus from the HLA- DP401- H2- Aβ1 humanized mice. Increased expression of HLA- DP401 was observed in the thymus of the humanized mice infected with S. aureus. |
Intraperitoneal infection model | ||||
[47] | S. aureus strain PS80 | NSG mice | Intrahepatic injection of human CD34+ cells | S. aureus infection was aggravated in humanized mice, compared with wild-type or nonengrafted mice. The humanized mice displayed a significantly reduced survival percentage, increased weight loss, and a more rapid increase in bacterial burden. S. aureus infection induced T-cell activation, apoptosis, and Fas receptor expression in humanized but not wild-type mice. |
Osteomyelitis model | ||||
[44] | S. aureus USA300 LAC strain | NSG mice | Intrahepatic injection of human CD34+ cells | HuNSG mice had increases in weight loss, osteolysis, bacterial dissemination to internal organs, and numbers of SACs, during the establishment of implant-associated MRSA osteomyelitis compared to NSG controls (P < .05). Flow cytometry and immunohistochemistry demonstrated greater human T-cell numbers in infected versus uninfected huNSG mice (P < .05), and that T-bet+ human T cells clustered around the SACs, suggesting S. aureus-mediated activation and proliferation of human T cells in the infected bone. |
Thigh muscle infection model | ||||
[45] | S. aureus USA300 LAC*lux | NSG mice | Intrahepatic injection of human CD34+ cells | Humanized mice proved not only to be more susceptible to MRSA infection than wild-type or “murinized” mice, but displayed furthermore inferior survival and signs of systemic infection in an otherwise localized infection model. Human and murine cytokine levels in blood and at the primary site of infection were strongly elevated in huNSG mice compared to all control groups. Differences in human and murine immune cell lineages surfaced during the infection, with human monocyte and B-cell numbers in blood and bone marrow being significantly reduced at the later time point of infection. Murine monocytes in contrast behaved conversely by increasing cell numbers. |
[46] | MRSA LAC*lux | NSG mice, NSG-SGM3 mice | Intrahepatic injection of human CD34+ cells | Humanized NSG-SGM3 mice showed strong human immune cell engraftment and develop anemia during prolonged course of humanization. MRSA thigh infection led to strongly reduced survival of huSGM3 mice. Humanized mice showed a tendency towards higher numbers of S. aureus in the respective organs. Stronger response of human immune cells in humanized NSG-SGM3 than in humanized NSG mice was found. The stronger the humanization, the higher the vulnerability to S. aureus infection. |
Bloodstream infection model | ||||
[31] | S. aureus USA300 LAC | C57BL/6 mice | Using CRISPR-mediated gene editing to encode a humanized LukAB-binding region in the murine CD11b I-domain. | LukAB binding to the CD11b I-domain is species specific and CD11b I-domain is subject to positive selection in primates and rodents. Humanizing CD11b renders mice susceptible to S. aureus infection, a phenotype mediated by LukAB. |
Abbreviations: BAC, bacterial artificial chromosome; CA-MRSA, community-associated methicillin-resistant S. aureus; HLA, human leukocyte antigen; hSIS, human skin and immune system; huNSG, humanized NSG; LAC, Los Angeles County; LukAB, leukocidin A/B; NSG-SGM3, NOD.Cg-Prkdcscid Il2rgtm1Wjl Tg(CMV-IL3, CSF2, KITLG)1Eav/MloySzJ); MRSA, methicillin-resistant S. aureus; NOD, nonobese diabetic; NSG, NOD/SCID/IL2rγnull; PVL, Panton-Valentine leucocidin; SACs, staphylococcal abscess communities; SCID, severe combined immune deficiency; SRG, Sprague–Dawley-Rag2tm2hera Il2rγtm1hera.
Ref. . | S. Aureus Stain . | Mouse Stain . | Humanized Manner . | Main Findings . |
---|---|---|---|---|
Skin infection model | ||||
[42] | MRSA USA300, spa null mutant, agr mutant, α-hemolysin mutants, PVL mutants, phenol-soluble modulin (Psm) (psmα, psmβ, or hld) single and triple mutants | NSG mice | Graft human neonatal foreskin | 22% of S. aureus isolated from patients with chronic infections of atopic dermatitis had an agr mutant-like phenotype. The increased intracellular persistence of toxin-deficient mutants was found. MRSA USA300 bacteria escape from the endosome to cause keratinocyte pyroptosis and WT MRSA USA300 and agr null mutant induce autophagy in human skin grafts. Induction of autophagy enhances MRSA persistence through effects on the inflammasome. |
[38] | CA-MRSA strain (CST5) and its isogenic PVL knockout strain | NSG mice | Intrahepatic injection of human CD34+ cells | Humanized NSG mice showed enhanced susceptibility to S. aureus skin and soft tissue infection. PVL induced dermopathology in the humanized NSG mouse model, which following adoptive transfer of human neutrophils. PMX53, a human C5aR inhibitor, blocked PVL-induced cellular cytotoxicity in vitro and reduced the size difference of lesions induced by the PVL+ and PVL−S. aureus, but PMX53 also reduced recruitment of neutrophils and exacerbated the infection. |
[41] | USA300 SF8300 (MRSA) | SCID.bg mice | Graft human abdominal skin | S. aureus transiently colonized the outer stratum corneum of healthy human skin. Noninvasive MRSA causes local inflammation in the underlying skin layers. Neutrophil recruitment to the skin reduced bacterial numbers on the skin surface. Neutrophils mediated the transiency of MRSA colonization. |
[39] | CA-MRSA USA300 | NSG mice, SRG rat | Cotransplants of human full-thickness fetal skin, autologous lymphoid tissues, and autologous hematopoietic stem cells | The hSIS-humanized NSG mouse model supported the development of full-thickness human skin, autologous lymphoid tissues (thymus and spleen), and human immune cells. The hSIS-humanized SRG rat model supported the development of full-thickness human skin, thymus tissues, and human immune cells. The human skin xenograft in immunodeficient rodent models supported CA-MRSA infection. |
[40] | CA-MRSA (Southwest Pacific clone, ST30, spa t019) strain, USA300 LAC (MRSA wild type, ST8, spa t008), and its isogenic mutant defective in vraR. | NOD/SCID mice | Intrahepatic injection of human CD34+ cells | Humanized mice were more sensitive to MRSA SSTI infection than BALB/c with an increased skin lesion size and bacterial burden at the infection site. Neutrophils from humanized mice were more susceptible to MRSA supernatant than that from BALB/c. There were reduction of virulence and increased susceptibility towards the human immune system in the ST30ΔvraR strain. |
Pneumonia model | ||||
[37] | S. aureus USA300 LAC, lukAB, lukS-F/PV (Δpvl) and complemented mutant (Δpvl/pvl+) | NSG mice | Intrahepatic injection of human CD34+ cells; Implantation of thymic tissue from the same donor under the kidney capsule | Humanized mice expressed large numbers of human leukocytes in the lungs, blood, and bone marrow. Severity of pulmonary infection in humanized mice with S. aureus increased. S. aureus induced cytokine expression in humanized mice. PVL expression involved in the pathogenesis of S. aureus lung infection and targeted to the human macrophage population. |
[43] | S. aureus stain Newman | C57BL/6 mice | Injection of the BAC-cloned HLA-DP401-H2-Aβ1−/− gene into mouse fertilized oocytes | Infection with S. aureus Newman did not alter the percentage of immune cells in the spleen and thymus from the HLA- DP401- H2- Aβ1 humanized mice. Increased expression of HLA- DP401 was observed in the thymus of the humanized mice infected with S. aureus. |
Intraperitoneal infection model | ||||
[47] | S. aureus strain PS80 | NSG mice | Intrahepatic injection of human CD34+ cells | S. aureus infection was aggravated in humanized mice, compared with wild-type or nonengrafted mice. The humanized mice displayed a significantly reduced survival percentage, increased weight loss, and a more rapid increase in bacterial burden. S. aureus infection induced T-cell activation, apoptosis, and Fas receptor expression in humanized but not wild-type mice. |
Osteomyelitis model | ||||
[44] | S. aureus USA300 LAC strain | NSG mice | Intrahepatic injection of human CD34+ cells | HuNSG mice had increases in weight loss, osteolysis, bacterial dissemination to internal organs, and numbers of SACs, during the establishment of implant-associated MRSA osteomyelitis compared to NSG controls (P < .05). Flow cytometry and immunohistochemistry demonstrated greater human T-cell numbers in infected versus uninfected huNSG mice (P < .05), and that T-bet+ human T cells clustered around the SACs, suggesting S. aureus-mediated activation and proliferation of human T cells in the infected bone. |
Thigh muscle infection model | ||||
[45] | S. aureus USA300 LAC*lux | NSG mice | Intrahepatic injection of human CD34+ cells | Humanized mice proved not only to be more susceptible to MRSA infection than wild-type or “murinized” mice, but displayed furthermore inferior survival and signs of systemic infection in an otherwise localized infection model. Human and murine cytokine levels in blood and at the primary site of infection were strongly elevated in huNSG mice compared to all control groups. Differences in human and murine immune cell lineages surfaced during the infection, with human monocyte and B-cell numbers in blood and bone marrow being significantly reduced at the later time point of infection. Murine monocytes in contrast behaved conversely by increasing cell numbers. |
[46] | MRSA LAC*lux | NSG mice, NSG-SGM3 mice | Intrahepatic injection of human CD34+ cells | Humanized NSG-SGM3 mice showed strong human immune cell engraftment and develop anemia during prolonged course of humanization. MRSA thigh infection led to strongly reduced survival of huSGM3 mice. Humanized mice showed a tendency towards higher numbers of S. aureus in the respective organs. Stronger response of human immune cells in humanized NSG-SGM3 than in humanized NSG mice was found. The stronger the humanization, the higher the vulnerability to S. aureus infection. |
Bloodstream infection model | ||||
[31] | S. aureus USA300 LAC | C57BL/6 mice | Using CRISPR-mediated gene editing to encode a humanized LukAB-binding region in the murine CD11b I-domain. | LukAB binding to the CD11b I-domain is species specific and CD11b I-domain is subject to positive selection in primates and rodents. Humanizing CD11b renders mice susceptible to S. aureus infection, a phenotype mediated by LukAB. |
Ref. . | S. Aureus Stain . | Mouse Stain . | Humanized Manner . | Main Findings . |
---|---|---|---|---|
Skin infection model | ||||
[42] | MRSA USA300, spa null mutant, agr mutant, α-hemolysin mutants, PVL mutants, phenol-soluble modulin (Psm) (psmα, psmβ, or hld) single and triple mutants | NSG mice | Graft human neonatal foreskin | 22% of S. aureus isolated from patients with chronic infections of atopic dermatitis had an agr mutant-like phenotype. The increased intracellular persistence of toxin-deficient mutants was found. MRSA USA300 bacteria escape from the endosome to cause keratinocyte pyroptosis and WT MRSA USA300 and agr null mutant induce autophagy in human skin grafts. Induction of autophagy enhances MRSA persistence through effects on the inflammasome. |
[38] | CA-MRSA strain (CST5) and its isogenic PVL knockout strain | NSG mice | Intrahepatic injection of human CD34+ cells | Humanized NSG mice showed enhanced susceptibility to S. aureus skin and soft tissue infection. PVL induced dermopathology in the humanized NSG mouse model, which following adoptive transfer of human neutrophils. PMX53, a human C5aR inhibitor, blocked PVL-induced cellular cytotoxicity in vitro and reduced the size difference of lesions induced by the PVL+ and PVL−S. aureus, but PMX53 also reduced recruitment of neutrophils and exacerbated the infection. |
[41] | USA300 SF8300 (MRSA) | SCID.bg mice | Graft human abdominal skin | S. aureus transiently colonized the outer stratum corneum of healthy human skin. Noninvasive MRSA causes local inflammation in the underlying skin layers. Neutrophil recruitment to the skin reduced bacterial numbers on the skin surface. Neutrophils mediated the transiency of MRSA colonization. |
[39] | CA-MRSA USA300 | NSG mice, SRG rat | Cotransplants of human full-thickness fetal skin, autologous lymphoid tissues, and autologous hematopoietic stem cells | The hSIS-humanized NSG mouse model supported the development of full-thickness human skin, autologous lymphoid tissues (thymus and spleen), and human immune cells. The hSIS-humanized SRG rat model supported the development of full-thickness human skin, thymus tissues, and human immune cells. The human skin xenograft in immunodeficient rodent models supported CA-MRSA infection. |
[40] | CA-MRSA (Southwest Pacific clone, ST30, spa t019) strain, USA300 LAC (MRSA wild type, ST8, spa t008), and its isogenic mutant defective in vraR. | NOD/SCID mice | Intrahepatic injection of human CD34+ cells | Humanized mice were more sensitive to MRSA SSTI infection than BALB/c with an increased skin lesion size and bacterial burden at the infection site. Neutrophils from humanized mice were more susceptible to MRSA supernatant than that from BALB/c. There were reduction of virulence and increased susceptibility towards the human immune system in the ST30ΔvraR strain. |
Pneumonia model | ||||
[37] | S. aureus USA300 LAC, lukAB, lukS-F/PV (Δpvl) and complemented mutant (Δpvl/pvl+) | NSG mice | Intrahepatic injection of human CD34+ cells; Implantation of thymic tissue from the same donor under the kidney capsule | Humanized mice expressed large numbers of human leukocytes in the lungs, blood, and bone marrow. Severity of pulmonary infection in humanized mice with S. aureus increased. S. aureus induced cytokine expression in humanized mice. PVL expression involved in the pathogenesis of S. aureus lung infection and targeted to the human macrophage population. |
[43] | S. aureus stain Newman | C57BL/6 mice | Injection of the BAC-cloned HLA-DP401-H2-Aβ1−/− gene into mouse fertilized oocytes | Infection with S. aureus Newman did not alter the percentage of immune cells in the spleen and thymus from the HLA- DP401- H2- Aβ1 humanized mice. Increased expression of HLA- DP401 was observed in the thymus of the humanized mice infected with S. aureus. |
Intraperitoneal infection model | ||||
[47] | S. aureus strain PS80 | NSG mice | Intrahepatic injection of human CD34+ cells | S. aureus infection was aggravated in humanized mice, compared with wild-type or nonengrafted mice. The humanized mice displayed a significantly reduced survival percentage, increased weight loss, and a more rapid increase in bacterial burden. S. aureus infection induced T-cell activation, apoptosis, and Fas receptor expression in humanized but not wild-type mice. |
Osteomyelitis model | ||||
[44] | S. aureus USA300 LAC strain | NSG mice | Intrahepatic injection of human CD34+ cells | HuNSG mice had increases in weight loss, osteolysis, bacterial dissemination to internal organs, and numbers of SACs, during the establishment of implant-associated MRSA osteomyelitis compared to NSG controls (P < .05). Flow cytometry and immunohistochemistry demonstrated greater human T-cell numbers in infected versus uninfected huNSG mice (P < .05), and that T-bet+ human T cells clustered around the SACs, suggesting S. aureus-mediated activation and proliferation of human T cells in the infected bone. |
Thigh muscle infection model | ||||
[45] | S. aureus USA300 LAC*lux | NSG mice | Intrahepatic injection of human CD34+ cells | Humanized mice proved not only to be more susceptible to MRSA infection than wild-type or “murinized” mice, but displayed furthermore inferior survival and signs of systemic infection in an otherwise localized infection model. Human and murine cytokine levels in blood and at the primary site of infection were strongly elevated in huNSG mice compared to all control groups. Differences in human and murine immune cell lineages surfaced during the infection, with human monocyte and B-cell numbers in blood and bone marrow being significantly reduced at the later time point of infection. Murine monocytes in contrast behaved conversely by increasing cell numbers. |
[46] | MRSA LAC*lux | NSG mice, NSG-SGM3 mice | Intrahepatic injection of human CD34+ cells | Humanized NSG-SGM3 mice showed strong human immune cell engraftment and develop anemia during prolonged course of humanization. MRSA thigh infection led to strongly reduced survival of huSGM3 mice. Humanized mice showed a tendency towards higher numbers of S. aureus in the respective organs. Stronger response of human immune cells in humanized NSG-SGM3 than in humanized NSG mice was found. The stronger the humanization, the higher the vulnerability to S. aureus infection. |
Bloodstream infection model | ||||
[31] | S. aureus USA300 LAC | C57BL/6 mice | Using CRISPR-mediated gene editing to encode a humanized LukAB-binding region in the murine CD11b I-domain. | LukAB binding to the CD11b I-domain is species specific and CD11b I-domain is subject to positive selection in primates and rodents. Humanizing CD11b renders mice susceptible to S. aureus infection, a phenotype mediated by LukAB. |
Abbreviations: BAC, bacterial artificial chromosome; CA-MRSA, community-associated methicillin-resistant S. aureus; HLA, human leukocyte antigen; hSIS, human skin and immune system; huNSG, humanized NSG; LAC, Los Angeles County; LukAB, leukocidin A/B; NSG-SGM3, NOD.Cg-Prkdcscid Il2rgtm1Wjl Tg(CMV-IL3, CSF2, KITLG)1Eav/MloySzJ); MRSA, methicillin-resistant S. aureus; NOD, nonobese diabetic; NSG, NOD/SCID/IL2rγnull; PVL, Panton-Valentine leucocidin; SACs, staphylococcal abscess communities; SCID, severe combined immune deficiency; SRG, Sprague–Dawley-Rag2tm2hera Il2rγtm1hera.
SKIN INFECTION MODELS
Skin is one of the most common sites of host immune response against S. aureus infections [48]. To date, there have been many studies of pathogen-human interplay in S. aureus skin infections using mice [48–50]. Recently, researchers have developed humanized mouse models of S. aureus skin infections. To more closely mimic human skin, Soong et al [42] grafted nonobese diabetic (NOD)/severe combined immune deficiency (SCID)/IL2rγnull (NSG) mice with human neonatal foreskin to study the MRSA adaptation to human keratinocytes, which is a form of humanized mouse model.
Tseng et al [38] developed a humanized mouse model of S. aureus skin infections in NSG mice engrafted with human umbilical CD34+ cells. Increased susceptibility to S. aureus SSTI was found in humanized mice compared to control mice. In humanized mice infected with lower doses of S. aureus, there was a significant difference in lesion sizes which correlated well with the engraftment efficacy. This study also demonstrated human polymorphonuclear leukocytes produced in humanized mice were responsive to PVL, consistent with a study by Hongo et al [51] that found mouse polymorphonuclear leukocytes to be resistant to PVL, while human polymorphonuclear leukocytes were susceptible. PVL induced dermonecrosis in NSG mice following adoptive transfer of human neutrophils, consistent with the results of a previous study demonstrating the important cytotoxic role of PVL in human neutrophils [52].
Since then, to investigate how healthy adult human skin responds to colonizing MRSA, Schulz et al [41], using a xenograft human skin model, showed transient colonization of xenografted human skin stratum corneum by MRSA in vivo at low doses of topical inoculation. Colonization of the stratum corneum of human skin occurred with MRSA-induced recruitment of polymorphonuclear leukocytes to the tissue in vivo, without evident penetration of the bacteria. In the absence of polymorphonuclear leukocytes, the bacterial count increased significantly, suggesting a role for polymorphonuclear leukocytes in controlling MRSA colonization of human skin stratum corneum. This model contributed to the development of humanized mouse models, which is the only model of MRSA transient human skin colonization with great promise.
Agarwal et al [39] reported the development of human skin and immune system (hSIS)-humanized NSG mouse models by transplanting with human fetal spleen, thymus, and liver tissues, autologous fetal liver-derived hematopoietic stem cells, and human skin tissues, supporting the development of full-thickness human skin, autologous lymphoid tissues (thymus and spleen), and human immune cells. In the hSIS-humanized NSG mouse model, readily detectable levels of human monocytes/macrophages in the blood and human skin were observed, suggesting the humanized mice can provide a means for studying S. aureus skin infections.
To investigate the role of vancomycin resistance-associated sensors/regulators (VraSR) in S. aureus SSTI, Barua et al [40] generated VraR mutants that inactivate the VraSR system in clinical strains of CA-MRSA representing serotype ST30, due to the VraS and VraR proteins enabling the bacteria to respond to environmental stress and survive. Organotypic keratinocyte fibroblast coculture (3-dimensional skin model) and SSTI humanized mice were constructed to study the effect of inactivation of the VraSR system on S. aureus virulence. The humanized mice showed an increased susceptibility to infections with increased lesion size and bacterial burden, compared with BALB/c mice. Additionally, preparations of polymorphonuclear leukocytes from humans and humanized mice exhibited more significant sensitivity to MRSA culture filtrate than BALB/c mice, indicating humanized mice could mimic the human immune system, which could be a more suitable animal model for studying MRSA SSTI. The size of skin lesions induced by wild-type ST30 was significantly increased in inoculated humanized mice compared to the isogenic ST30ΔvraR mutant strain.
Together, the above studies illustrate the role of humanized mouse models in skin lesions induced by S. aureus, and provide the basis and insight for subsequent studies.
PULMONARY INFECTION MODELS
S. aureus is a pervasive pathogen capable of causing various infections of the respiratory tract, whose range extends from symptomless colonization to fulminant necrotizing pneumonia [53]. To date, there have been 2 studies using humanized mice to research S. aureus pulmonary infections.
Prince et al [37] observed PVL expression contributed to S. aureus pathogenesis in pulmonary infections in humanized mice. Humanized mice bore large numbers of human leukocytes in the lungs, blood, and bone marrow, as well as an increased severity of lung infections. Humanized mice contained a higher bacterial burden compared to both C57BL/6J and nonhumanized NSG mice. In addition, adequate human cells were found in the lungs of humanized mice to induce strong human cytokine expression. Moreover, elevated numbers of human immune cells correlated with increased severity of S. aureus infections, as further demonstrated by using interleukin 3 (IL-3)/granulocyte-macrophage colony-stimulating factor (GM-CSF) knock-in mice, and the IL-3/GM-CSF humanized mice showed greater susceptibility to S. aureus versus humanized NSG mice.
Li et al [43] also established a humanized mouse model of pneumonia caused by S. aureus. In this study, tissue-specific expression was found for HLA-DPA1/DPB1 molecules in these transgenic mice, and increased expression of HLA-DP401 was observed in the thymus after S. aureus infections, suggesting such humanized mice could be employed to elucidate the role of the HLA-DP gene in autoimmune susceptibility and development. Another study also demonstrated transgenic mice expressing HLA-DR molecules responded more robustly to superantigens and their response to pathogenic S. aureus mimicked that of humans [54].
INTRAPERITONEAL INFECTION MODEL
One study of intraperitoneal infections with S. aureus using humanized mice is available to date. Knop et al [47] developed a humanized mouse model of S. aureus intraperitoneal infections in NSG mice engrafted with human CD34+ hematopoietic stem cells, showing humanized mice displayed significantly increased mortality and lower survival rates, more weight loss, and higher concentrations of pathogens in different organs, compared with wild-type and nonengrafted mice. Furthermore, the activation of T cells was more marked in humanized mice than in wild-type mice. Apoptosis caused by S. aureus infections in human T cells was observed, but not in mice T cells. Additionally, increased expression of Fas receptor and caspase-3 was observed in humanized mice but not in wild-type mice. The introduction of humanized mice as a viable animal model in this study allows for further differentiation of S. aureus pathogenesis under human-like conditions, providing new perspectives for subsequent studies.
OSTEOMYELITIS AND MUSCLE INFECTION MODELS
S. aureus is the main cause of osteomyelitis [55]. To date, there have been many studies using traditional mouse models to study osteomyelitis and muscle infections caused by S. aureus [56–62]. However, there has been only 1 study on osteomyelitis and 2 studies on thigh muscle infections caused by S. aureus using humanized mice. Muthukrishnan et al [44] implanted human hematopoietic stem cells in NSG mice (huNSG) and constructed an S. aureus osteomyelitis model. It was found that humanized mice exhibited exacerbated susceptibility to S. aureus infections. S. aureus infections induced a significant upregulation of human CD3+ T cells, and their subpopulation CD4+ T helper cells and CD8+ cytotoxic T cells, in huNSG compared to controls. huNSG mice had increased in weight loss, osteolysis, bacterial dissemination to internal organs, and a number of staphylococcal abscess communities (SACs), during the establishment of implant-associated MRSA osteomyelitis compared to NSG controls. In addition, T-bet+ human T cells clustered around the SACs, suggesting S. aureus-mediated activation and proliferation of human T cells existed in the infected bone.
Hung et al [45] inoculated huNSG with S. aureus USA300 LAC∗ lux by local infections of the thigh muscles. Experimentally, huNSG mice showed more susceptibility to localized MRSA infections compared to wild-type NSG mice, wild-type BALB/c mice, and murinized NSG mice. Monitoring of bacterial burden and local spread during S. aureus infections revealed humanized mice exhibited significantly higher signal intensities than that of all other groups. Compared with controls, the huNSG mice continued to lose significant weight throughout the infections and showed additional signs of severe illness, and the survival rate of the huNSG mice was significantly lower. Human and murine cytokine levels in the blood and at the primary site of infection were dramatically higher in huNSG mice. During S. aureus infections, differences in the immune cell profiles of humans and mice emerged, and the number of human monocytes and B cells in the blood and bone marrow significantly reduced in the later stage of infections. In contrast, the number of mice monocytes showed an increase. Based on this study, Hung et al [46] established the same model of localized S. aureus thigh muscle infections in huNSG-SGM3 (humanized NOD-scid IL2Rgnull-3/GM/SF) mice. Due to the genetic integration of human SCF, GM-CSF, and IL-3 genes, NSG-SGM3 bore a large number of human monocytes and neutrophils. HuNSG-SGM3 mice had a higher humanization rate and stronger increase of human immune cells, slightly reduced hemoglobin and red blood cell levels, and increased number of reticulocytes compared with huNSG mice. Compared with the nonhumanized group, the huNSG and huNSG-SGM3 groups showed increased susceptibility to deep tissue infection by S. aureus, while huSGM3 mice showed extreme vulnerability. The bacteria burden in organs of humanized mice was higher than that in the controls. In addition, these 2 studies believed the extent of humanization was positively correlated with the higher susceptibility of mice to S. aureus. huSGM3 mice may be the next-generation humanized mice with improved bone marrow reconstruction, which likely will accelerate the progress of research into human diseases in the future.
BLOODSTREAM INFECTION MODEL
S. aureus bloodstream infections are one of the most common serious bacterial infections worldwide, and are a heterogenous clinical entity with a high risk of metastatic complications and high in-hospital mortality [63]. Boguslawski et al [31] identified the 11-residue domain required for LukAB specificity to human CD11b, which is sufficient to make mice CD11b compatible with toxin binding; clustered regularly interspaced short palindromic repeats (CRISPR)-mediated gene editing was then used to replace this domain, generating humanized mice. Increased susceptibility to MRSA bloodstream infections was observed in humanized mice. Unlike previous studies, which mostly involved implanting human cells, this study used genetic engineering to produce humanized mice, which might facilitate the subsequent development of improved mouse models that more closely resemble human infections, and might be useful in the development of anti-S. aureus therapy.
LIMITATIONS AND THE FUTURE OF HUMANIZED MOUSE MODELS
Humanized mouse models have greatly helped to overcome the limitations of traditional mouse models in the investigation of human diseases. Unfortunately, no single model is sufficient to support the study of a wide range of human diseases, including infectious diseases, parasitic diseases, and cancer [33]. Apart from this, the cost of producing humanized mice is high and the small sizes of mice are restricted [38, 64]. The limited lifespan of mice is another barrier. The short experimental window for humanized mice inoculated with S. aureus may not be suitable for certain types of studies [39]. In addition, the effect of interactions between other S. aureus factors and their human receptors may not be measured using a model of mice expressing one human molecule. In addition, the use of CD34+ cells from different donors may lead to variations, and these mice are immunocompromised and not suitable for certain types of studies. There also exist known and unknown incompatibilities between humans and mice, which may affect the development and function of human immune cells [38]. For example, Hung et al [45] indicated that the human immune system in humanized mice got caught in the maelstrom of local infections and bacterial spreading without being able to produce enough immune cells to overcome this challenge. This might, at least in part, be due to the fact that some human immune cell lineages are underrepresented in huNSG mice. In addition, many of the tools available in mice, such as knockout and neutralizing antibodies, are not available in humanized mouse models [38]. NSG mice are most commonly used to generate humanized mice; however, they have some inherent defects including limited myeloid development and underdevelopment of functional cells, which have implications for certain studies [33, 65, 66].
Due to the above limitations, there is much room for improvement in humanized mouse models, which would benefit the study of S. aureus to a great extent. The susceptibility and sensitivity of modified humanized mouse models to S. aureus infections could also be further increased [10]. The huSGM3 mice described above are a new generation of humanized mice with improved myeloid cell reconstitution [46]. The more complex genetic background and human environmental exposure to microbes raise the question of generalizability from mice to humans. Perhaps this could be addressed in the future by using humanized sterile mouse models that represent the human gut [67]. In addition, more efforts are necessary in the future to fully generalize the function of neutrophils in mice. Based on the identification of human-specific receptors and recent advances in the CRISPR-CAS system [68], it is possible to develop a mouse model compatible with all leukocidins, which could lead to studies on the role of leukocidins as a group in S. aureus infections [32]. Instead of limiting ourselves to mouse models, perhaps it would be better to use more models of the immune and cardiovascular systems that are closer to those of humans, such as rabbits.
CONCLUSIONS
S. aureus is among the most common causes of morbidity and mortality throughout the world and can cause a variety of diseases such as skin infections, pneumonia, and sepsis in humans. Mouse models are the most commonly used animal models to study the interaction between S. aureus and hosts; however, the experimental results obtained from them cannot be translated to humans due to the limitations of traditional mice. In recent years, studies have been conducted of S. aureus skin infections, pneumonia, intraperitoneal infections, osteomyelitis, and muscle infections using humanized mice. These have demonstrated that humanized mice have increased susceptibility to S. aureus and exhibited more severe infections compared to controls. Several researchers have used humanized mouse models to study PVL and LukAB, both of which are human-specific virulence factors produced by S. aureus. Humanized mice have facilitated the study of human-specific bacterial factors (PVL and LukAB), allowed the testing of specific human therapies, and provided a unique framework to begin exploring vaccine development for S. aureus, not only as highly promising preclinical models but as useful tools for understanding the pathophysiology and developing therapies for human diseases. Despite the tremendous progress in the field, there remain some limitations, and more human-like models, such as the next generation of humanized mice and other animal models closer to humans, are needed.
Notes
Author contributions. H. Y. and G. D. conceptualized the study. P. Z., F. L., J. L., Y. J., and S. C. performed literature search. P. Z. wrote the manuscript. All authors approved the final manuscript.
Disclaimer. The funder had no role in the data collection, data analysis, preparation of manuscript, and decision to submit.
Data availability. The data that support the findings of this study are included in this article and available from the corresponding author upon reasonable request.
Financial support. This work was supported by the National Natural Science Foundation of China (grant numbers 82273696 and 81973105).
References
Author notes
Potential conflicts of interest. All authors: No potential conflicts of interest. All authors have submitted the ICMJE Form for Disclosure of Potential Conflicts of Interest. Conflicts that the editors consider relevant to the content of the manuscript have been disclosed.