-
PDF
- Split View
-
Views
-
Cite
Cite
Mitchell J Waldran, Adam D Wegman, Lauren E Bahr, Nathan H Roy, Jeffrey R Currier, Adam T Waickman, Soluble NS1 Antagonizes IgG- and IgA- Mediated Monocytic Phagocytosis of DENV Infected Cells, The Journal of Infectious Diseases, Volume 228, Issue 1, 1 July 2023, Pages 70–79, https://doi.org/10.1093/infdis/jiad122
- Share Icon Share
Abstract
Dengue virus (DENV) is endemic in >100 countries, infecting an estimated 400 million individuals every year. Infection with DENV raises an antibody response primarily targeting viral structural proteins. However, DENV encodes several immunogenic nonstructural (NS) proteins, one of which, NS1, is expressed on the membrane of DENV-infected cells. IgG and IgA isotype antibodies that bind NS1 are abundant in serum following DENV infection. Our study aimed to determine if NS1-binding IgG and IgA isotype antibodies contribute to the clearance of DENV-infected cells by antibody-mediated cellular phagocytosis. We observed that both IgG and IgA isotype antibodies can facilitate monocytic uptake of DENV NS1-expressing cells in an FcγRI- and FcαRI-dependent fashion. Interestingly, this process was antagonized by the presence of soluble NS1, suggesting that the production of soluble NS1 by infected cells may serve as immunological chaff, antagonizing opsonization and clearance of DENV-infected cells.
Dengue virus (DENV) infects an estimated 400 million individuals annually, resulting in 100 million clinically apparent infections and at least 40 000 deaths [1, 2]. Consisting of 4 immunologically and genetically distinct serotypes (DENV-1, -2, -3, and -4), DENV is transmitted by the bite of infected mosquitos belonging to the genus Aedes [1, 3, 4]. Primary DENV infections are typically mild, causing fever, rashes, nausea, vomiting, and myalgias and arthralgias [1, 4, 5]. However, a fraction of heterologous secondary infections progress to severe dengue, characterized by plasma leakage or bleeding leading to shock and risk of death [6–8]. The leading mechanistic explanation for the increased disease risk associated with post–primary dengue is a process known as antibody-dependent enhancement, wherein DENV-reactive antibodies generated by a primary DENV infection facilitate the infection of cells that are normally poorly susceptible to the virus via Fc-gamma receptor (FcγR)–mediated endocytosis [9–11].
Antibodies directed against the structural components of the DENV virion—namely the E and prM proteins—are responsible for both neutralizing and enhancing the infectivity of the virus depending on the abundance of antibody and the epitope engaged by an antibody [12]. However, these are not the only antigenic viral proteins expressed by DENV-infected cells. Nonstructural protein 1 (NS1) is a 55 kDa glycoprotein that is thought to play a role in several steps of DENV assembly. Initially synthesized as a monomer, intracellular NS1 rapidly forms a membrane-associated homodimer in the endoplasmic reticulum and serves as core component of the viral replication complex [13, 14]. Dimeric NS1 can also be found on the membrane of infected cells (mNS1) [15–18] and is secreted by infected cells into the extracellular space in higher-order oligomeric forms [19, 20]. Once in circulation, soluble NS1 (sNS1) is thought to be a mechanistic trigger of vascular leakage symptoms in severe dengue by binding and destabilizing vascular endothelial cells [21, 22]. Accordingly, reducing the immunostimulatory capability of sNS1 is a leading avenue of anti-dengue therapeutic research.
Antibodies capable of binding both sNS1 and mNS1 can be found in circulation shortly after the appearance of antibodies directed against the DENV virion, with iIgM, IgA, and IgG all represented [23–25]. As with DENV virion-binding antibodies, NS1-binding IgM and IgA appear transiently after infection, while NS1-specific IgG persists in circulation [23]. How these different antibody isotypes functionally contribute to the resolution of an acute DENV infection and provide protection from subsequent infection is unclear.
Antibodies of all isotypes are in theory capable of binding and sequestering sNS1—thereby reducing the immunopathogenic properties of the protein—while IgM and IgG isotype antibodies can facilitate lysis of DENV-infected cells via complement fixation [26]. However, IgG and IgA isotype antibodies are additionally capable of interacting with their cognate Fc receptors (FcRs) on innate immune cells, thereby facilitating an array of FcR-dependent effector function [27]. This includes antibody-dependent cellular cytotoxicity (ADCC)—facilitated by FcγR expressing natural killer (NK) cells—as well as antibody-dependent cellular phagocytosis (ADCP). Of note, multiple professional phagocytes, including monocytes, macrophages, and dendritic cells, express both FcγRs and Fc-alpha receptors (FcαRs), meaning they are potentially capable of utilizing both IgG and IgA isotype antibodies for the phagocytic clearance of opsonized viral antigen and virally infected cells [26]. However, what role—if any—opsonizing IgG and IgA isotype anti-mNS1 antibodies play in the phagocytic clearance of DENV-infected cells remains unclear.
To fill this knowledge gap, we developed an in vitro model of NS1-directed phagocytosis and a pair of idiotope-matched NS1-reactive IgG and IgA isotype monoclonal antibodies (mAbs). Using this system, we observed that both IgG and IgA isotype antibodies are capable of facilitating monocytic uptake of DENV NS1 expressing cellular material in an additive fashion. This process was dependent on the expression FcγRI (CD64) and FcαR (CD89) for IgG- and IgA-mediated membrane uptake, respectively. Furthermore, this process was antagonized by the presence of sNS1, suggesting that the production of sNS1 by infected cells may serve as an immunological smoke screen, limiting opsonization and clearance of infected cells by opsonizing antibodies.
MATERIALS AND METHODS
Monoclonal Antibodies and Serum
The variable regions of the previously described DENV-2–reactive murine monoclonal antibody 1G5.3 (Supplementary Figure 1A–C) [28] were codon optimized, synthesized in vitro, and subcloned into an expression vector containing the human IgG1 or IgA1 Fc region by a commercial partner (Genscript). Following transfection into Chinese Hamster Ovary (CHO) cells, the cell culture IgG supernatant was purified using MabSelect SuReTM LX and the IgA supernatant was purified using CaptureSelectTM IgA affinity matrix. Antibody purity was confirmed by sodium dodecyl sulphate–polyacrylamide gel electrophoresis and the final concentration determined by 280 nm absorption. DENV immune serum was obtained from a commercial source (SeraCare, Milford, Massachusetts).
Cell Lines and Primary Human Peripheral Blood Mononuclear Cell Isolation
A DENV-2 NS1-expressing cell line was generated by electroporating CEM.NKR cells with a linearized pCDNA3.1 plasmid containing the codon-optimized sequence shown in Supplementary Figure 2A derived from the E, NS1, and NS2A sequence of the DENV-2 strain 16681 (GenBank NC_001474), followed by G418 selection. Stable clones were generated by single-cell sorting of NS1 expressing cells using a BD FACSAria Fusion instrument into a 96-well plate. A DC-SIGN expressing cell line was generated by transfecting CEM.NKR cells with a linearized pCDNA3.1 plasmid containing the codon-optimized sequence of DC-SIGN (GenBank NM_021155.4), followed by the same selection and subcloning process as above. Surface expression of DENV-2 NS1 and DC-SIGN was confirmed by flow cytometry prior to each assay. All cells were maintained in RPMI media (Corning, 10–040-CV) supplemented with 10% fetal bovine serum (FBS) (Corning, 35-016-CV), 1% penicillin/streptomycin, and 1% L-glutamine. Peripheral blood mononuclear cells (PBMCs) were collected and isolated from normal healthy volunteers using BD Vacutainer CPT tubes containing sodium heparin. The State University of New York Upstate Medical University institutional review guidelines for the use of human subjects were followed for all experimental protocols in the study.
Viruses
DENV-2 (strain S16803) was propagated in Vero cells. Virus was titrated on DC-SIGN expressing CEM.NKR cells overnight prior to use in phagocytosis assays.
NS1 Enzyme-Linked Immunosorbent Assay
Ninety-six-well NUNC MaxSorbflat-bottom plates (Thermo Scientific) were coated with 50 µL of a 2 µg/mL solution of purified DENV-2 NS1 (Native Antigen, DENV2-NS1–100) or DENV-1 NS1 (Native Antigen, DENV1-NS1–100) diluted in carbonate/bicarbonate buffer. After washing and blocking, the monoclonal antibodies were prepared at 10 µg/mL top concentration followed by 4-fold serial dilutions. Antibody binding was detected using peroxidase-labeled anti-human IgG (Southern Biotech 2044-05) or IgA (Biolegend 411002), followed by detection with TMB Microwell Peroxidase Substrate (KPL, cat. #50-76-00).
Antibody-Dependent Phagocytosis Assay
CEM.NKR and DENV-2 NS1-expressing CEM.NKR cells were stained with PKH26 (MiliporeSigma, cat #MIDI26) at a final concentration of 3.0 × 10−6 M in Diluent C for 5 minutes at room temperature. Cells were then washed twice with phosphate-buffered saline + 2% FBS prior to opsonization at 4°C with sera (1:500 dilution) or anti-NS1 mAbs (1 µg/mL) for 30 minutes. After opsonization, 2 × 104 of the labeled CEM.NKR cells were added to a well of a polypropylene round-bottom 96-well plate (Corning, CLS3879), and mixed with 2 × 105 of purified PBMCs at 37°C. The cells were incubated for 3 hours at 37°C. The cells were then washed twice, stained with antihuman CD14 (Biolegend 301818), and analyzed on a BD LSRII flow cytometer. Purified soluble DENV-1 NS1 protein (Native Antigen DENV1-NS1-100) and DENV2-NS1 (Native Antigen DENV2-NS1-100) was added during the opsonization step as a competitive inhibitor for experiments at a final concentration of 1 µg/mL.
FcR Blocking Assay
To assess the contribution of individual FcRs to NS1-directed ADCP, PBMCs were incubated with either anti-CD89 (Bio-Rad MCA1824, clone MIP8a), anti-CD32 (Stemcell Technologies 60012, clone IV.3), or anti-CD64 (Biolegend 305002, clone 10.1) prior incubation with opsonized CEM.NKR target cells. The PBMCs were incubated for 5 minutes on ice before being combined with the target cells, and the blocking antibodies were maintained during the phagocytosis assay.
Microscopy
Monocytes were purified from fresh PBMCs using a Mojosort Human Pan Monocyte Isolation kit (catalog number 480059, lot number B375603). PKH26-labeled CEM.NKR cells and CFSE (BD Horizon, 565082)–labeled monocytes were co-incubated in a 8-well microscope plate (ibidi, cat. #80806). Cells were allowed to settle for 15 minutes before being imaged on a Marianas system (3i) enclosed in an environmental chamber (Okolab) consisting of a Ziess Axio Observer 7 equipped with a X-Cite mini + light source (Excelitas) and a Prime BSI Express CMOS camera (Photometrics). Images were taken every 30 seconds for 2 hours at ×10 magnification. Acquisition is done using SlideBook 6 (3i) and exported into Fiji for analysis and presentation [29].
Quantification of Surface FcR Abundance
Phycoerythrin (PE)–conjugated calibration beads (Bangs Laboratories) were analyzed at the same time as live cells stained with saturating concentrations of PE-conjugated antibodies. Linear regression calculation was performed using the isotype-subtracted mean fluorescence intensity (MFI) values from the PE calibration beads and known number of PE molecules per bead. The resulting equation was used to calculate the number of cytokine receptors present on live cells, using the isotype-subtracted PE MFI values from each FcR analyzed. The anti-FcR antibodies used were anti-CD32 (Biolegend 303205), anti-CD64 (Biolegend 305007), anti-CD89 (Biolegend 354103), PE mouse IgG1 isotype control (Biolegend 400114), PE mouse IgG2a (Biolegend 400214), and PE mouse IgG2b (Biolegend 400314).
Statistical Analysis
All statistical analyses were performed using GraphPad Prism Software (GraphPad Software, La Jolla, California). A P value <.05 was considered significant. Analysis of variance was used for the comparison of multiple conditions.
RESULTS
Development of an IgG and IgA Opsonization Assay
The objective of this study was to assess the contribution of NS1-reactive IgG and IgA isotype antibodies to the phagocytic uptake of material from DENV-infected cells. To this end, we synthesized the previously described DENV-2–specific mAb 1G5.3 [28] with either a human IgG1 or an IgA1 Fc domain (Figure 1A, Supplementary Figure 1A–C). To confirm that the isotype conversions did not impact the antigen-binding capability of the synthesized mAbs, we tested the DENV-2 and DENV-1 sNS1 binding activity of the antibodies by ELISA (Figure 1B, Supplementary Figure 1D). This analysis showed that the conversion of 1G5.3 to either a human IgG1 or IgA1 isotype did not impact their antigen binding ability, with both mAbs exhibiting a half maximal effective concentration (EC50) of approximately 7 ng/mL toward DENV-2 NS1 and no appreciable binding of DENV-1 NS1.
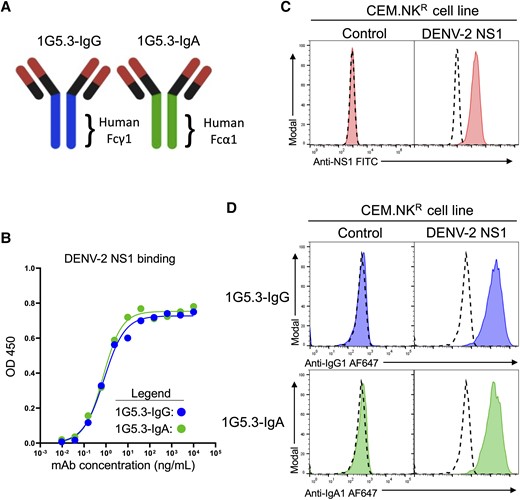
Generation and characterization of a nonstructural protein 1 (NS1)–dependent IgG and IgA opsonization assay. A, Schematic representation of the 1G5.3-IgG and 1G5.3-IgA monoclonal antibodies (mAbs) utilized in this study. B, Assessment of NS1 binding activity of 1G5.3-IgG and 1G5.3-IgA by enzyme-linked immunosorbent assay at optical density (OD) 450. C, Characterization of a dengue virus serotype 2 (DENV-2) mNS1-expressing CEM.NKR cell line by staining with the pan-DENV NS1-reactive antibody clone FE8. Dashed histograms indicate staining without primary antibody of the indicated cell type. D, Opsonization of DENV-2 NS1-expressing CEM.NKR cells 1G5.3-IgG and 1G5.3-IgA. Dashed histograms indicate staining without primary antibody on the indicated cell line.
To test the ability of these antibodies to bind mNS1 and opsonize mNS1 expressing cells, we synthesized a DENV-2 NS1 expression construct that was stably expressed in the CEM.NKR human T lymphoblastoid cell line (Figure 1C, Supplementary Figure 2A). We confirmed that both 1G5.3-IgG and 1G5.3-IgA exhibited opsonizing activity against the DENV-2 mNS1-expressing CEM.NKR cell line, while exhibiting no binding to the parental line (Figure 1D).
Primary Human Monocytes Are Capable of IgG- and IgA-Mediated Phagocytosis of DENV mNS1-Expressing Target Cells
Having developed both the antibodies and cell lines required to test the ability of IgG and IgA isotype antibodies to facilitate the phagocytic clearance of DENV-infected cells, first we assessed the ability of purified monocytes to phagocytize IgG-opsonized DENV-2 NS1-expressing CEM.NKR cells using time-lapse microscopy. In this assay, NS1-expressing CEM.NKR target cells were labeled with the lipophilic dye PKH26 prior to opsonization with 1G5.3-IgG. Target cells were then mixed with bead-purified primary human monocytes labeled with CFSE and imaged over the course of 2 hours. During the course of this assay, the CFSE-labeled monocytes were observed to interact with and engulf portions of the PKH26-labeled target, resulting in punctate accumulations of labeled membrane within the monocytes (Figure 2A, Mov1). To allow for higher-throughput analysis of monocyte phagocytosis, we next optimized a flow-based ADCP assay (Supplementary Figure 2B). As with the microscopy assay, CEM.NKR target cells were first labeled with PKH26 prior to opsonization with either serum or mAbs. Target cells were then co-incubated with freshly isolated PBMCs from normal healthy donors for 3 hours, after which the phagocytic accumulation of PKH26-labeled target cell membrane within CD14+ monocytes was assessed by flow cytometry.
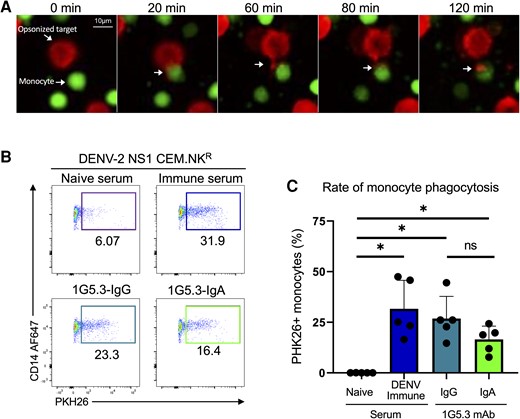
Generation and characterization of a nonstructural protein 1 (NS1)–dependent antibody phagocytosis assay. A, Time-lapse microscopy of CFSE-stained monocytes (green) interacting with PKH26-stained dengue virus serotype 2 NS1-expressing CEM.NKR cells opsonized with 1G5.3-IgG (red). B, Representative flow plots from the antibody-dependent phagocytosis assay. C, Percentage of monocytes that were positive for the target cell membrane dye, separated by antibody used to opsonize target. Data are expressed as background subtracted from a no serum condition, n = 5 individual experiments. Error bars are mean ± standard error of the mean. *P < .05, paired 1-way analysis of variance. Abbreviations: DENV-2, dengue virus serotype 2; IgA, immunoglobulin A; IgG, immunoglobulin G; mAb, monoclonal antibody; ns, not significant; NS1, nonstructural protein 1.
Minimal monocytic phagocytosis was observed when the target cells were opsonized with DENV-naive serum (Figure 2B and 2C). However, a significant increase in phagocytic activity was observed when the mNS1-expressing target cells were opsonized with either DENV-immune sera or with either 1G5.3-IgG or 1G5.3-IgA NS1-reactive mAbs (Figure 2B and 2C). No phagocytic activity was observed in assays using the parental CEM.NKR cell line, which lacks DENV-2 NS1 expression as the target cell in conjunction with any of the opsonizing antibodies described above (Supplementary Figure 2C).
FcγRI and FcαR Mediate Antibody-Dependent Phagocytosis of mNS1-Expressing Cells
Primary human CD14+ monocytes express FcγRIIA (CD32), FcγRI (CD64), and FcαR (CD89), with each FcγR twice as abundant as the single FcαR (Figure 3A). To determine the individual contribution of the FcRs on antibody-mediated monocytic phagocytosis, we utilized a panel of FcR blocking antibodies to inhibit Fc/FcR interactions during phagocytosis. Pretreatment with an anti-FcγRIIa blocking antibody did not reduce IgG1-medated monocytic phagocytosis (Figure 3B). However, pretreatment with anti-FcγRI significantly antagonized IgG-dependent phagocytic activity in this assay. Neither FcγR-blocking antibody reduced IgA-mediated phagocytosis under the same assay conditions. However, treatment with the FcαR-blocking antibody significantly reduced IgA1-medated monocytic phagocytosis without impacting IgG activity (Figure 3C).
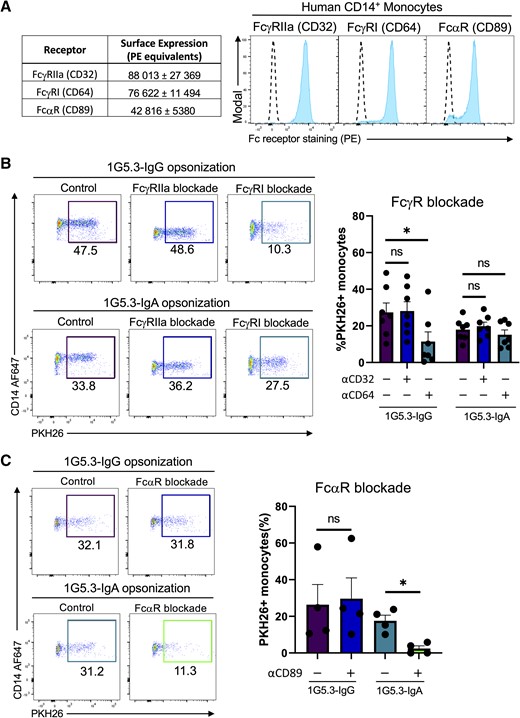
Contribution of FcγR and FcαR to IgG– and IgA–mediated phagocytosis. A, Surface abundance of FcRs on CD14+ monocytes mean ± standard error of the mean (SEM) (left) and representative flow histograms (right). Quantification of FcR abundance was performed utilizing flow cytometric analysis of a standard curve of calibration beads conjugated with known quantifies of phycoerythrin (PE) molecules run in parallel with peripheral blood mononuclear cells stained with saturating concentrations of PE-conjugated anti-FcR antibodies. Dashed line indicates staining of the indicated cell line with a corresponding isotype control. B, Percentage of monocytes positive for target cell membrane indicating antibody-dependent phagocytosis with either anti-FcγRIIa or anti-FcγRI antibodies. Data are expressed as background subtracted from a no serum condition, n = 7 individual experiments. C, Percentage of monocytes positive for target cell membrane indicating antibody-dependent phagocytosis with anti-FcαR antibodies. Data are expressed as background subtracted from a no serum condition, n = 4 individual experiments. All error bars are mean ± SEM. *P < .05, paired 1-way analysis of variance; ns, not significant.
No Evidence of Synergy Between IgG and IgA Antibodies in Mediating Monocytic Phagocytosis
Having established that both IgG and IgA isotype antibodies can independently mediate monocytic phagocytosis of mNS1-expressing cells, we next endeavored to assess any potential synergistic activity of NS1-specific IgG and IgA in this process. It has been previously observed that IgA is able to enhance IgG-mediated ADCC by monocytes and polymorphonuclear leukocytes, and we hypothesized a similar effect might be observed in the setting of antibody-dependent monocytic phagocytosis [30]. To this end we titrated both 1G5.3-IgG and 1G5.3-IgA independently or mixed at a 1:1 ratio to determine if the mix of antibodies exhibited any differential activity when used together. Both 1G5.3-IgG and 1G5.3-IgA facilitated nearly identical levels of phagocytic activity across more than 3 logs of antibody concentration, as did the 1:1 mixture of the 2 antibodies used at the same opsonizing concentration (Figure 4). These results suggest that IgG and IgA can act in an additive fashion to facilitate monocytic phagocytic of antigen-expressing cells, but there is no evidence of synergistic activity.
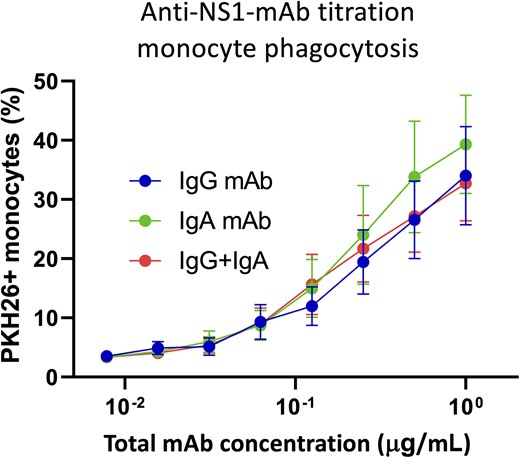
Assessment of IgG and IgA synergism in antibody-mediated phagocytosis. Titration curve for monocytic phagocytosis using either 1G5.3-IgG (blue), 1G5.3-Iga (green), or a 1:1 mix of 1G5.3-IgG and 1G5.3-IgA at the indicated total antibody concentration. n = 3 individual experiments. Abbreviations: IgA, immunoglobulin A; IgG, immunoglobulin G; mAb, monoclonal antibody; NS1, nonstructural protein 1.
IgA and IgG Facilitate Antibody-Dependent Monocytic Uptake of DENV-2–Infected Cells
The analysis presented in the study thus far has utilized a transgenic expression system to uniformly express mNS1 on a target cell. While this represents a well-controlled and highly reproducible experimental system, we wanted to confirm that the results obtained thus far were still observed in cells expressing mNS1 as the result of DENV infection. To this end, we infected DC-SIGN expressing CEM.NKR cells with DENV-2 for use as target cells in our ADCP assay (Figure 5A, Supplementary Figure 3B). Approximately 25% of the target cells expressed mNS1 in these conditions at levels consistent with those observed in the DENV-2 NS1-expressing CEM.NKR cell line (Figure 5B). Having confirmed mNS1 expression on these DENV-2–infected cells, they were used in the ADCP assay described above. As was observed with the mNS1-expressing CEM.NKR cell line, DENV-immune serum, 1G5.3-IgG, and 1G5.3-IgA were observed to facilitated monocytic phagocytosis of DENV-2–infected cells (Figure 5C and 5D).
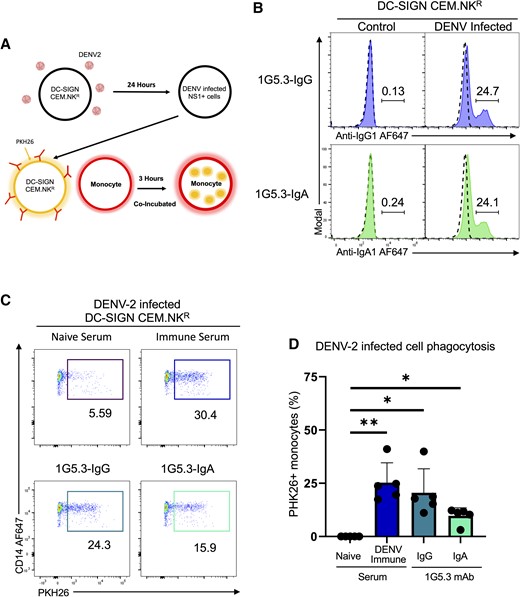
IgA and IgG facilitate antibody-dependent monocytic uptake of dengue virus serotype 2 (DENV-2)–infected cells. A, Model depicting the antibody-dependent phagocytosis assay including the infection of DC-SIGN–expressing CEM.NKR cells. B, Opsonization of DENV-2–infected DC-SIGN–expressing CEM.NKR cells with nonstructural protein 1–reactive 1G5.3-IgG and 1G5.3-IgA monoclonal antibodies (mAbs), with percentage monocytes positive for target cell membrane indicating antibody-dependent phagocytosis. Dashed line in histograms indicates staining with DENV-naive serum. C, Representative flow plot for antibody-dependent phagocytosis assay using DENV-2–infected DC-SIGN–expressing cells as targets. D, Percentage of monocytes positive for target cell membrane indicating antibody-dependent phagocytosis with DENV-2–infected cells as targets. Data are expressed as background subtracted from a no serum condition, n = 5 individual experiments. Error bars are mean ± standard error of the mean. *P < .05, **P < .01, paired 1-way analysis of variance.
Soluble NS1 Is Capable of Abrogating Monocyte Antibody-Dependent Phagocytosis
While our study thus far has focused on the immunologic contribution of mNS1 in dengue, NS1 is best known for its sNS1 form, which can be found at microgram per milliliter quantities in serum during acute DENV infection [31–33]. It is currently unclear why flaviviruses have evolved to secrete such high levels of NS1 into the extracellular space. While sNS1 has been described as a potential pathogenic factor capable of triggering multiple immunopathogenic programs, there is no clear advantage to the virus from an evolutionary standpoint. However, it has been proposed that sNS1 acts to aid in immune avoidance by depleting complement in the vicinity of DENV-infected cells [34]. In light of the results obtained in our study this far, we hypothesized that another reason why DENV-infected cells may produce such abundant sNS1 was to act as immunologic “chaff,” protecting DENV-infected cells from opsonizing and subsequent clearance by NK cells and phagocytes.
To test this hypothesis, we modified our ADCP assay to include the addition of purified recombinant NS1 during the opsonization and co-incubation steps. The CEM.NKR cell line used in this study produces very little sNS1 following either transfection with the NS1 expression construct or following infection with DENV, so exogenous recombinant sNS1 at a concentration similar to what is observed in serum during acute DENV infection (1 μg/mL) was spiked into the assay cultures. Given the highly preferential DENV-2 NS1 binding activity of the 1G5.3 mAbs used in this study, DENV-1 NS1 was used as a control to account for any immunomodulatory effects of NS1 itself outside of its role as an antibody target. Using this modified assay, we observed that the addition of purified DENV-2 NS1 was able to abrogate the monocytic antibody-dependent phagocytosis of DENV-2 NS1-expressing CEM.NKR cells (Figure 6). This process was specific to the anti-NS1 antibodies as the addition of serotype-mismatched DENV-1 NS1—which neither 1G5.3-IgG or 1G5.3-IgA binds—had no effect on the level of antibody-dependent phagocytosis (Figure 6).
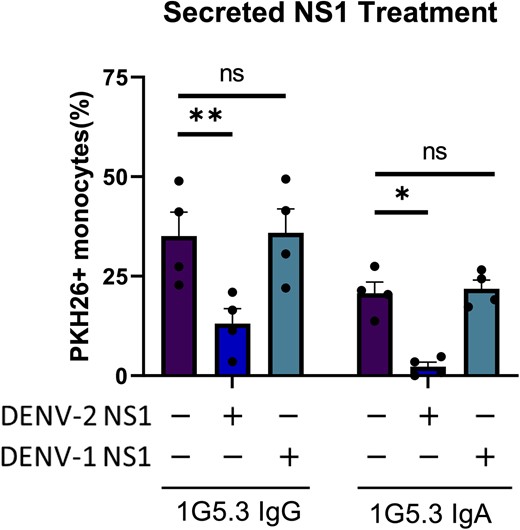
Soluble nonstructural protein 1 (NS1) is capable of antagonizing monocytic antibody-dependent phagocytosis. Phagocytosis assay with purified secreted dengue virus (DENV)–1 or DENV-2 NS1 added during the opsonization step. Percentage monocytes positive for target cell membrane indicates phagocytosis, n = 4 individual experiments. Error bars are mean ± standard error of the mean. *P < .05, **P < .01, paired 1-way analysis of variance. Abbreviations: DENV, dengue virus; IgA, immunoglobulin A; IgG, immunoglobulin G; ns, not significant; NS1, nonstructural protein 1.
In light of these results, we propose the following model: NS1 expressed on the cell surface of DENV-infected cells can be opsonized by NS1-reactive IgG and IgA isotype antibodies, which can then facilitate monocytic phagocytosis (Supplementary Figure 4). However, NS1-directed antibody-mediated phagocytosis can be abrogated by sNS1 binding NS1-reactive antibodies, leading to immune evasion of the infected cells and increased viral replication and dissemination within the host.
DISCUSSION
In this study, we defined a role for NS1-reactive IgG and IgA antibodies in mediating antibody-dependent monocytic phagocytosis of DENV mNS1-expressing cells. Our analysis revealed that both IgG and IgA isotype antibodies can facilitate phagocytic uptake of DENV-infected material via FcγRI (CD64) and FcαR (CD89) on monocytes, and that these 2 antibody isotypes can work together in an additive—but not synergistic—fashion when combined. Notably, we observed that the addition of sNS1 dramatically antagonized the ability of both NS1-reactive IgG and IgA to facilitate monocytic uptake of NS1-expressing cellular material, highlighting a potential mechanism of DENV immune evasion.
We posit that sNS1 plays a role in DENV immune evasion by sequestering anti-NS1 antibodies to prevent opsonization of DENV-infected cells, thereby shielding them from clearance via monocytic antibody-dependent phagocytosis, ADCC, or other any other Fc receptor–mediated effector mechanisms. This would not be a unique immune-avoidance mechanism, as there are numerous examples of secreted viral proteins aiding in viral persistence in the face of antigen-specific adaptive immunity. For example, cells infected with cowpox virus secrete the CPXV14 protein that antagonizes antibody-mediated immune activation via FcγR [35]. Vaccinia virus also encodes a secretory protein, VCP, that binds C3b and C4b to inhibit complement activation [36]. More closely related to DENV, West Nile virus NS1 has been shown to inhibit complement activation by binding to regulatory protein factor H [37]. This is similar to how DENV sNS1 has been suggested to block complement activity in the proximity of DENV-infected cells by either blocking the formation of the membrane attack complex and/or C9 polymerization [34, 38].
While our study focuses on DENV, NS1-reactive antibodies have been observed in circulation after infection with numerous other flavivirus including Zika virus (ZIKV) [39–42]. Notably, ZIKV NS1-reactive antibodies have been shown to be capable of facilitating NK cell degranulation and lysis of NS1-expressing cells using a system similar to that presented in our study [39]. NS1-reactive antibody therapies and NS1-containing vaccine products have all exhibited promise in preclinical testing, with these products exhibiting the ability to facilitate both ADCC as well as complement fixation on flavivirus-infected cells [43–46]. However, while this vaccination strategy may reduce DENV pathogenesis by sequestering sNS1 in serum and reducing its ability to interact with endothelial cells, our data suggest that this same property would limit the ability of these NS1-reactive antibodies to bind and clear DENV-infected cells [47].
There are a few limitations that should be considered while interpreting the results of this study. Most of the data were generated using a cell line that was modified to stably express NS1 on the cell surface or infected cell cultures with a high rate of infection. The consequence of this is that the local NS1 antigen burden in this assay may be significantly higher than what is observed during natural infection and may exaggerate the efficacy of antibody-mediate phagocytosis. In addition, the timing of sNS1 production and the production of NS1-reactive antibodies means that sNS1 blockade of antibody-mediated phagocytosis may only play a role during secondary DENV infections, leaving unaddressed the potential immune-avoidance role of NS1 during primary DENV infection.
In closing, we suggest that our study highlights several unappreciated facets of anti-DENV immunity. Not only have we demonstrated a role for NS1-reactive IgA in the setting of acute flavivirus infection, but have identified a putative method of flavivirus immune evasion via sNS1. These results will inform not only future countermeasure development, but may also represent a new correlate of risk or protection from infection.
Supplementary Data
Supplementary materials are available at The Journal of Infectious Diseases online. Consisting of data provided by the authors to benefit the reader, the posted materials are not copyedited and are the sole responsibility of the authors, so questions or comments should be addressed to the corresponding author.
Notes
Author contributions. Conceptualization: M. J. W., J. R. C., A. T. W. Formal analysis: M. J. W., A. T. W. Funding acquisition: A. T. W. Investigation: M. J. W., A. D. W., L. E. B. Resources: J. R. C., A. T. W. Writing–original draft: M. J. W., A. T. W. Writing–review and editing: all authors.
Acknowledgments. We gratefully acknowledge Lisa Phelps of the State University of New York Upstate Medical University Flow Cytometry Core for technical assistance, and Dr Joel Wilmore for his critical review of the manuscript.
Disclaimer. The opinions or assertions contained herein are the private views of the authors and are not to be construed as reflecting the official views of the US Army or the US Department of Defense. The material has been reviewed by the Walter Reed Army Institute of Research, and there is no objection to its presentation and/or publication.
Financial support. This work was supported by the Military Infectious Disease Research Program and the State of New York.
References
Author notes
Potential conflicts of interest. A. T. W. is a co-founder of Azimuth Biologics, Inc. All other authors report no potential conflicts.
All authors have submitted the ICMJE Form for Disclosure of Potential Conflicts of Interest. Conflicts that the editors consider relevant to the content of the manuscript have been disclosed.