-
PDF
- Split View
-
Views
-
Cite
Cite
Beckie N Tagbo, Harish Verma, Zubairu M Mahmud, Kolade Ernest, Roosevelt O Nnani, Chinedu Chukwubike, Kehinde T Craig, Abdullahi Hamisu, William C Weldon, Steven M Oberste, Visalakshi Jeyaseelan, Fiona Braka, Pascal Mkanda, Dorothy Esangbedo, Adebiyi Olowu, Eric Nwaze, Roland W Sutter, Randomized Controlled Clinical Trial of Bivalent Oral Poliovirus Vaccine and Inactivated Poliovirus Vaccine in Nigerian Children, The Journal of Infectious Diseases, Volume 226, Issue 2, 15 July 2022, Pages 299–307, https://doi.org/10.1093/infdis/jiaa726
- Share Icon Share
Abstract
We conducted a trial in Nigeria to assess the immunogenicity of the new bivalent oral poliovirus vaccine + inactivated poliovirus vaccine (bOPV+IPV) immunization schedule and gains in type 2 immunity with addition of second dose of IPV. The trial was conducted in August 2016–March 2017, well past the trivalent OPV-bOPV switch in April 2016.
This was an open-label, 2-arm, noninferiority, multicenter, randomized, controlled trial. We enrolled 572 infants aged ≤14 days and randomized them into 2 arms. Arm A received bOPV at birth, 6, and 10 weeks, bOPV+IPV at week 14, and IPV at week 18. Arm B received IPV each at 6, 10, and 14 weeks and bOPV at 18 weeks of age.
Seroconversion rates for poliovirus types 1 and 3, respectively, were 98.9% (95% confidence interval [CI], 96.7–99.8) and 98.1% (95% CI, 88.2–94.8) in Arm A and 89.6% (95% CI, 85.4–93.0) and 98.5% (95% CI, 96.3–99.6) in Arm B. Type 2 seroconversion with 1 dose IPV in Arm A was 72.0% (95% CI, 66.2–77.3), which increased significantly with addition of second dose to 95.9% (95% CI, 92.8–97.9).
This first trial on the new Expanded Program on Immunization (EPI) schedule in a sub-Saharan African country demonstrated excellent immunogenicity against poliovirus types 1 and 3 and substantial/enhanced immunogenicity against poliovirus type 2 after 1 to 2 doses of IPV, respectively.
The Global Polio Eradication Initiative (GPEI) has made significant progress since 1988 when the World Health Assembly resolved to eradicate poliomyelitis by the year 2000 [1]. Type 2 poliomyelitis associated with wild poliovirus type 2 (WPV2) and type 3 (WPV3) were certified to be eradicated in 2015 [2] and 2019 [3], respectively. Nigeria has not detected any WPV1 transmission since August 2016 [4, 5], but it continues to circulate in Pakistan and Afghanistan [6]. Although, oral poliovirus vaccine (OPV) usage resulted in a significant reduction in the number of polio-affected countries from >125 in 1988 to only 3 in 2018 [4], it is associated with the emergence of vaccine-derived poliovirus (VDPV) [7] and a low risk of vaccine-associated paralytic polio [8]. The Polio Eradication Endgame and Strategic Plan 2013–2018 recommended a sequential removal of all Sabin strains, starting with type 2, in all OPV-using countries with the introduction of ≥1 dose of inactivated poliovirus vaccine (IPV) in routine immunization (RI) [9]. In April 2016, Sabin type 2 component was withdrawn and the world switched from trivalent OPV (tOPV) to the bivalent OPV (bOPV) [10]. Nigeria, like every other OPV-using country, switched from tOPV to bOPV in 2016 with the introduction of IPV in RI, and the current RI schedule for polio is bOPV at birth, 6, 10, and 14 weeks with IPV at 14 weeks [11]. It was considered important to obtain immunogenicity data from Nigeria, Africa’s last WPV-endemic country, to assess the efficacy of the current Expanded Program on Immunization (EPI) schedule after the switch as well as assess the need for an additional dose of IPV. Therefore, the main objective of the trial was to assess immunogenicity of the new EPI schedule introduced in Nigeria. It is the only recent trial in sub-Saharan Africa that reported immunogenicity of combined use of bOPV and IPV in RI, although similar data were available from a historical collaborative study conducted in Oman, the Gambia, and Thailand [12] and recent studies conducted in Bangladesh [13] and India [14]. In addition, this trial was designed to evaluate the immunogenicity of administering a different number of doses of IPV only, considering that all OPVs are likely to be withdrawn globally in future.
METHODS
The design was an open-label, 2 arm, prospective, multicenter, randomized, noninferiority trial conducted between August 2016 and March 2017 at 4 sites, 2 each in Enugu and Kwara states of Nigeria. These states were selected because they were free from repeated subnational supplementary polio immunization activities. The study arms and the study procedures are as shown in the consort flow diagram (Figure 1). Arm A received bOPV at birth and 6 and 10 weeks, bOPV+IPV at week 14, and IPV at week 18. Arm B received IPV each at 6, 10, and 14 weeks of age and bOPV at 18 weeks. Another dose of bOPV was given at 22 weeks to ensure mucosal immunity to poliovirus types 1 and 3. Infants aged ≤14 days, weighing ≥2.5 kilograms, and who had not received any poliovirus vaccine since birth were eligible. The protocol followed good clinical practice standards, ethical approvals were obtained from the World Health Organization (WHO) Ethical Committee, and Ethics Committees of the study institutions were involved in the trial. The Nigerian government approval was provided by the National Agency for Food and Drugs Administration and Control (NAFDAC). The trial was registered with the Australian New Zealand Clinical Trials Registry as ANZCTR 12616001272482 (http://www.anzctr.org.au).
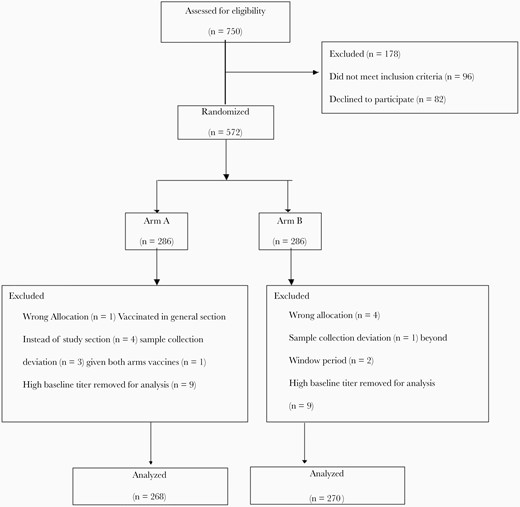
Consort flow chart of participants included and excluded in per-protocol analysis for the primary outcome.
Randomization and Masking
Eligible children were randomly allocated to 2 study arms. This was based on computer-generated, centrally labeled, permuted block randomization with block sizes of 2, 4, or 6 1:1 allocation in both arms. The parent or the study investigator had no discretion to opt for a particular study arm. The random numbers were generated using SAS 9.1.3 [15]. This study was open-label because the participants and the study investigators were not masked to the vaccines administered. However, the outcome assessors who were the laboratory investigators at the Centers for Disease Control and Prevention ([CDC] Atlanta, GA) were masked to the vaccine allocation.
Study Procedures
The mother and child health (MCH) centers at the selected institutions served as the study sites. Mothers of newborn babies in the catchment areas were counseled about the usefulness of vaccination for their babies. On arrival at the MCH centers, after the usual registration, weight and length measurements, an immunization card was made. In addition, the nurse at the counter screened the infants for study participation. Those within 14 days of age were connected with the study team for consent. If the caretaker provided approval for the child’s participation, written informed consent was obtained, and the child was enrolled into 1 of 2 study arms as per random allocation. Venous blood sample (2 mL) was collected under sterile conditions from each participant. Subsequently, each participant was vaccinated by a trained nurse who took the necessary aseptic precautions. A paper questionnaire was also filled out with necessary information. Subsequent visits and procedures took place at 6, 10, 14, 18, and 22 weeks. The standard composition bOPV (not less than 106 CCID50 [culture infectious dose 50% assay] for type 1; not less than 105.8 CCID50 for type 3) from Biofarma (Bandung, Indonesia) and IPV from Sanofi Pasteur (Lyon, France) (type 1 [Mahoney], type 2 [MEF-1], and type 3 [Saukett] 0.5 mL contained 40 D-antigen units [DU] for type 1, 8 DU for type 2, and 32 DU for type 3) were used. The vaccines used in the trial were the same as those routinely used in Nigeria’s immunization program and were WHO-prequalified, UNICEF-supplied, and nationally licensed. The conventional doses of bOPV (2 drops) orally and IPV (0.5 mL intramuscularly) were used. Each subject was vaccinated from a separate vaccine vial at each visit. The remainder of each vial was labeled with the subject’s identification number and stored back in cold chain for vaccine accountability. All vaccines, used and unused, were stored in strict cold chain conditions. Serum separation was done from the blood samples collected at baseline, 14, 18, and 22 weeks. Sera were stored in 2-mL cryovials at ≤−20°C until shipment on dry ice by a professional courier agency. They were sent to the CDC for microneutralization assay of antibody titers to the 3 poliovirus serotypes [16]. Adverse events (AEs) and serious AEs (SAEs) were captured via observation for 30 minutes after each vaccination and after telephonic and/or home visit follow up on days 1, 3, and 7. In addition, at the time of each subsequent visit, the physician enquired about any events in-between the visits and performed a physical examination to detect and record any findings. An elaborate plan for early reporting, timely assessment, investigations, and treatment was put in place for all AEs. Study subjects could withdraw at any time and were not replaced. The reason for withdrawal was recorded.
Outcomes
The primary endpoint was cumulative seroconversion rate at week 18. Seropositivity was defined as antibody titer ≥1:8 (log2 titer of ≥3). Criteria for seroconversion included the subjects who became seropositive from seronegative after vaccination or a ≥4-fold rise in antibody titer over the expected decline in maternal antibodies at that point of time [17]. A separate analysis was performed for poliovirus types 1, 2, and 3 titers. Secondary outcome was gains in seroconversion with an additional dose of IPV in Arm A.
Sample Size
We aimed to show that the cumulative seroconversion rate of the 4-dose bOPV + 1-dose IPV schedule in Arm A was noninferior to the 3-dose IPV schedule in Arm B against poliovirus types 1 and 3. Seroconversion rates for type 1 and type 3 after 3 doses of IPV administered at 6, 10, and 14 weeks were taken from studies in Cuba (94% and 85.8%) [18] and Puerto Rico (100% and 96.9%) [19]. Assuming a conservative seroconversion rate of 85% in both schedules, with a noninferiority margin of 10%, significance level of α = 0.025, and power of 0.90, the required sample size was determined to be 218 in each arm. Anticipating approximately 15% loss to follow up or insufficient blood samples, the total number of children to be enrolled per arm was determined as 260. In total, the sample size required was 520.
Analyses
Baseline distribution of the subject characteristics have been summarized as percentages for categorical variables and mean with standard deviation for continuous variables. Length and weight were measured to assess nutritional status and determine stunting and wasting by comparing the child’s measurements to the WHO Child Growth Standards reference population based on the WHO Multicenter Growth Reference Study. Wasting and stunting, based on the Z-scores, were categorized as no (≥ −2) and yes (< −2) [20]. All children who had antibody titer values above the highest reported titer of ≥1448 at baseline in both groups were excluded from per-protocol (PP) analysis. Seroconversion rates, expressed as percentage, were presented in both arms with Wilson’s score 95% confidence intervals (CIs) for all 3 poliovirus types. Comparison of seroconversion rates was made using Fisher’s exact test for proportions. Noninferiority analysis was conducted on PP and intention-to-treat (ITT) population. The median antibody titers were calculated using a bootstrap method with 95% CIs obtained from 10 000 bootstrap samples [21]. The median titers were compared using Wilcoxon rank-sum test. The other secondary outcome was assessed using Cochrane-Armitage test. The AEs and SAEs were expressed as percentages. R 3.4.3 [22] and SAS 9.1.3 were used for statistical analyses.
RESULTS
There were 750 children screened for eligibility, 96 of whom did not meet the inclusion criteria, and parents of 82 infants refused to participate. A total of 572 eligible children were randomized to 1 of the 2 arms (Figure 1). There were no significant differences in sociodemographic characteristics and nutritional status of participants between the 2 study arm groups (Table 1). In total, 34 participants (6%) were excluded from the final analysis. Finally, 268 participants in Arm A and 270 participants in Arm B were included in the final PP analysis (Figure 1). The ITT population consisted of all children randomized to the 2 vaccine arms. Baseline type 1 and type 3 poliovirus seroprevalences were slightly different between the study arms (Table 1).
Study characteristics . | Arm A . | . | Arm B . | . |
---|---|---|---|---|
. | n . | % . | n . | % . |
States | ||||
Enugu vs kwara | 143 | 50.0 | 143 | 50.0 |
Mother’s highest education | ||||
Primary | 43 | 15.0 | 40 | 14.0 |
Secondary | 121 | 42.3 | 127 | 44.4 |
Tertiary | 122 | 42.7 | 119 | 41.6 |
Father’s highest education | ||||
Primary | 32 | 11.2 | 24 | 8.4 |
Secondary | 121 | 42.3 | 129 | 45.1 |
Tertiary | 133 | 46.5 | 133 | 46.5 |
Gender | ||||
Female vs Male | 134 | 46.9 | 132 | 46.2 |
Breast Feeding (BF) | ||||
BF + supplementary milk | 7 | 2.4 | 10 | 3.5 |
BF + water | 46 | 16.1 | 57 | 19.9 |
Exclusive BF | 133 | 81.5 | 218 | 76.2 |
Wasting | ||||
No vs Yes | 280 | 98.2 | 275 | 96.5 |
Stunting | ||||
No vs Yes | 181 | 63.2 | 188 | 65.7 |
Poliovirus type 1 seroprevalence | ||||
Yes vs No | 215 | 78.8 | 241 | 85.3 |
Poliovirus type 2 seroprevalence | ||||
Yes vs No | 216 | 79.1 | 218 | 78.1 |
Poliovirus type 3 seroprevalence | ||||
Yes vs No | 133 | 48.7 | 158 | 55.2 |
Mothers’ age (in years) (Mean and SD) | 28.9 | 4.8 | 28.8 | 4.8 |
Father’s age (in years) (Mean and SD) | 35.4 | 6.1 | 35.3 | 6.0 |
Poliovirus type 1 titer among seropositive at baseline | ||||
Median (95% bootstrap CI) | 91 | 74–111 | 111 | 74–147 |
Poliovirus type 2 titer among seropositive at baseline | ||||
Median (95% bootstrap CI) | 69 | 58–91 | 56 | 45–56 |
Poliovirus type 3 titer among seropositive at baseline | ||||
Median (95% bootstrap CI) | 28 | 23–45 | 36 | 28–45 |
Study characteristics . | Arm A . | . | Arm B . | . |
---|---|---|---|---|
. | n . | % . | n . | % . |
States | ||||
Enugu vs kwara | 143 | 50.0 | 143 | 50.0 |
Mother’s highest education | ||||
Primary | 43 | 15.0 | 40 | 14.0 |
Secondary | 121 | 42.3 | 127 | 44.4 |
Tertiary | 122 | 42.7 | 119 | 41.6 |
Father’s highest education | ||||
Primary | 32 | 11.2 | 24 | 8.4 |
Secondary | 121 | 42.3 | 129 | 45.1 |
Tertiary | 133 | 46.5 | 133 | 46.5 |
Gender | ||||
Female vs Male | 134 | 46.9 | 132 | 46.2 |
Breast Feeding (BF) | ||||
BF + supplementary milk | 7 | 2.4 | 10 | 3.5 |
BF + water | 46 | 16.1 | 57 | 19.9 |
Exclusive BF | 133 | 81.5 | 218 | 76.2 |
Wasting | ||||
No vs Yes | 280 | 98.2 | 275 | 96.5 |
Stunting | ||||
No vs Yes | 181 | 63.2 | 188 | 65.7 |
Poliovirus type 1 seroprevalence | ||||
Yes vs No | 215 | 78.8 | 241 | 85.3 |
Poliovirus type 2 seroprevalence | ||||
Yes vs No | 216 | 79.1 | 218 | 78.1 |
Poliovirus type 3 seroprevalence | ||||
Yes vs No | 133 | 48.7 | 158 | 55.2 |
Mothers’ age (in years) (Mean and SD) | 28.9 | 4.8 | 28.8 | 4.8 |
Father’s age (in years) (Mean and SD) | 35.4 | 6.1 | 35.3 | 6.0 |
Poliovirus type 1 titer among seropositive at baseline | ||||
Median (95% bootstrap CI) | 91 | 74–111 | 111 | 74–147 |
Poliovirus type 2 titer among seropositive at baseline | ||||
Median (95% bootstrap CI) | 69 | 58–91 | 56 | 45–56 |
Poliovirus type 3 titer among seropositive at baseline | ||||
Median (95% bootstrap CI) | 28 | 23–45 | 36 | 28–45 |
Study characteristics . | Arm A . | . | Arm B . | . |
---|---|---|---|---|
. | n . | % . | n . | % . |
States | ||||
Enugu vs kwara | 143 | 50.0 | 143 | 50.0 |
Mother’s highest education | ||||
Primary | 43 | 15.0 | 40 | 14.0 |
Secondary | 121 | 42.3 | 127 | 44.4 |
Tertiary | 122 | 42.7 | 119 | 41.6 |
Father’s highest education | ||||
Primary | 32 | 11.2 | 24 | 8.4 |
Secondary | 121 | 42.3 | 129 | 45.1 |
Tertiary | 133 | 46.5 | 133 | 46.5 |
Gender | ||||
Female vs Male | 134 | 46.9 | 132 | 46.2 |
Breast Feeding (BF) | ||||
BF + supplementary milk | 7 | 2.4 | 10 | 3.5 |
BF + water | 46 | 16.1 | 57 | 19.9 |
Exclusive BF | 133 | 81.5 | 218 | 76.2 |
Wasting | ||||
No vs Yes | 280 | 98.2 | 275 | 96.5 |
Stunting | ||||
No vs Yes | 181 | 63.2 | 188 | 65.7 |
Poliovirus type 1 seroprevalence | ||||
Yes vs No | 215 | 78.8 | 241 | 85.3 |
Poliovirus type 2 seroprevalence | ||||
Yes vs No | 216 | 79.1 | 218 | 78.1 |
Poliovirus type 3 seroprevalence | ||||
Yes vs No | 133 | 48.7 | 158 | 55.2 |
Mothers’ age (in years) (Mean and SD) | 28.9 | 4.8 | 28.8 | 4.8 |
Father’s age (in years) (Mean and SD) | 35.4 | 6.1 | 35.3 | 6.0 |
Poliovirus type 1 titer among seropositive at baseline | ||||
Median (95% bootstrap CI) | 91 | 74–111 | 111 | 74–147 |
Poliovirus type 2 titer among seropositive at baseline | ||||
Median (95% bootstrap CI) | 69 | 58–91 | 56 | 45–56 |
Poliovirus type 3 titer among seropositive at baseline | ||||
Median (95% bootstrap CI) | 28 | 23–45 | 36 | 28–45 |
Study characteristics . | Arm A . | . | Arm B . | . |
---|---|---|---|---|
. | n . | % . | n . | % . |
States | ||||
Enugu vs kwara | 143 | 50.0 | 143 | 50.0 |
Mother’s highest education | ||||
Primary | 43 | 15.0 | 40 | 14.0 |
Secondary | 121 | 42.3 | 127 | 44.4 |
Tertiary | 122 | 42.7 | 119 | 41.6 |
Father’s highest education | ||||
Primary | 32 | 11.2 | 24 | 8.4 |
Secondary | 121 | 42.3 | 129 | 45.1 |
Tertiary | 133 | 46.5 | 133 | 46.5 |
Gender | ||||
Female vs Male | 134 | 46.9 | 132 | 46.2 |
Breast Feeding (BF) | ||||
BF + supplementary milk | 7 | 2.4 | 10 | 3.5 |
BF + water | 46 | 16.1 | 57 | 19.9 |
Exclusive BF | 133 | 81.5 | 218 | 76.2 |
Wasting | ||||
No vs Yes | 280 | 98.2 | 275 | 96.5 |
Stunting | ||||
No vs Yes | 181 | 63.2 | 188 | 65.7 |
Poliovirus type 1 seroprevalence | ||||
Yes vs No | 215 | 78.8 | 241 | 85.3 |
Poliovirus type 2 seroprevalence | ||||
Yes vs No | 216 | 79.1 | 218 | 78.1 |
Poliovirus type 3 seroprevalence | ||||
Yes vs No | 133 | 48.7 | 158 | 55.2 |
Mothers’ age (in years) (Mean and SD) | 28.9 | 4.8 | 28.8 | 4.8 |
Father’s age (in years) (Mean and SD) | 35.4 | 6.1 | 35.3 | 6.0 |
Poliovirus type 1 titer among seropositive at baseline | ||||
Median (95% bootstrap CI) | 91 | 74–111 | 111 | 74–147 |
Poliovirus type 2 titer among seropositive at baseline | ||||
Median (95% bootstrap CI) | 69 | 58–91 | 56 | 45–56 |
Poliovirus type 3 titer among seropositive at baseline | ||||
Median (95% bootstrap CI) | 28 | 23–45 | 36 | 28–45 |
Primary Outcome
At week 18, the cumulative seroconversion to poliovirus type 1 in Arms A and B were 98.9% (95% CI, 96.8–99.8) and 89.6% (95% CI, 85.4–93.0), respectively. The difference in seroconversion rate between the 2 arms in the PP population was 9.3% (95% CI, 5.5–13.1). The difference in the proportions in ITT population was 8.1% (95% CI, 2.9–13.3) (Figure 2). The cumulative seroconversion to poliovirus type 3 in Arms A and B were 98.1 (95% CI, 95.7–99.4) and 98.5% (95% CI, 96.3–99.6), respectively. The difference in the seroconversion rate between the 2 arms was −0.4% (95% CI, −2.5 to 1.8) (ITT: −1.0) (95% CI, −5.3 to 3.3) (Figure 2).
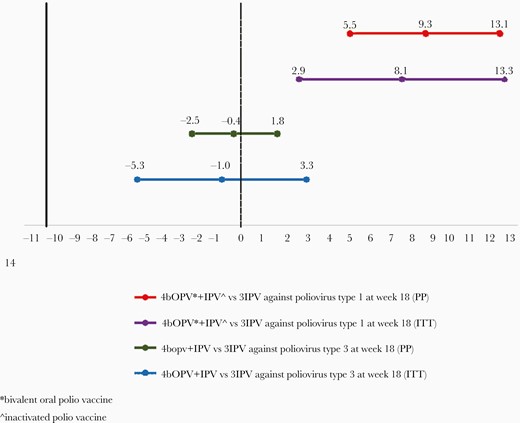
Seroconversion rates at week 18 comparing Arm A vs Arm B for poliovirus 552 types 1 and 3, respectively.
Secondary Outcomes
The cumulative seroconversion rates at week 18 for poliovirus type 2 in the 2 arms were 72.0% (95% CI, 66.2–77.3) and 93.7% (95% CI, 90.1–96.3), respectively. Addition of a second dose of IPV in Arm A at week 18 increased seroconversion from 72.0% to 95.9% (95% CI, 92.8–97.9; P < .001). The second dose of IPV also closed the immunity gaps for serotypes 1 and 3 in Arm A. The median reciprocal antibody titers by the study arms are also presented (see Table 2). The comparison of the median titers at week 18 in Arm A for poliovirus types 1 and 3 were ≥1448 (95% CI, ≥1448 to ≥1448) and ≥1448 (95% CI, ≥1448 to ≥1448), whereas in Arm B the median titers were 455 (95% CI, 362–576; P < .0001) and 1152 (95% CI, 1152 to ≥1448; P < .001). The median titers comparisons for poliovirus type 2 at week 18 in Arms A and B were 28 (95% CI, 23–36) and 181 (95% CI, 181–228), respectively (P < .001).
Seroconversion and median titers with 95% bootstrap CIs in per-protocol population
Response . | Arm A . | . | . | . | . | Arm B . | . | . | . | . |
---|---|---|---|---|---|---|---|---|---|---|
. | Seroconversion . | . | . | Reciprocal titers . | . | Seroconversion . | . | . | Reciprocal titers . | . |
Poliovirus type 1 . | N . | N% . | 95% CI . | Median . | CI . | n . | N% . | 95% CI . | Median . | CI . |
At week 14 | 243 | 26890.7 | 86.5–93.9 | ≥1448 | ≥1448 to ≥1448 | 175 | 27064.8 | 58.8–70.5 | 288 | 228–455 |
At week 18 | 22 | 2588.0 | 68.8–97.5 | ≥1448 | 576 to ≥1448 | 67 | 9571.0 | 61.0–80.1 | 144 | 91–228 |
Cumulativea | 265 | 26898.9 | 96.8–998 | ≥1448 | ≥1448 to -≥1448 | 242 | 27089.6 | 85.4–93.0 | 455 | 362–576 |
At week 22 | 3 | 3100.0 | 20 | 2876.9 | 56.4–91.0 | 1300 | 910 to ≥1448 | |||
Cumulativeb | 268 | 268100.0 | 268 | 268100.0 | ≥1448 | ≥1448 to ≥1448 | ||||
Poliovirus type 2 | n | N% | 95% CI | Median | CI | n | N% | 95% CI | Median | CI |
At week 14 | 25 | 2689.3 | 6.1–13.5 | 23 | 18–57 | 180 | 27066.7 | 60.7–72.3 | 114 | 91–144 |
At week 18 | 168 | 24369.4 | 63.2–75.2 | 28 | 23–36 | 73 | 9081.1 | 71.5–88.6 | 91 | 57–144 |
Cumulativea | 193 | 26872.0 | 66.2–77.3 | 28 | 23–36 | 253 | 27093.7 | 90.1–96.3 | 181 | 181–228 |
At week 22 | 64 | 7585.3 | 75.3–92.4 | 114 | 57–114 | 2 | 1711.8 | 1.5–36.4 | 34 | 23–45 |
Cumulativeb | 257 | 26895.9 | 92.8–97.9 | 114 | 91–114 | 255 | 27094.4 | 91.0–96.9 | 144 | 114–181 |
Poliovirus type 3 | n | N% | 95% CI | Median | CI | n | N% | 95% CI | Median | CI |
At week 14 | 241 | 26889.9 | 85.7–93.3 | 724 | 724–910 | 247 | 27091.5 | 87.5–94.5 | 1152 | 1152 to ≥1448 |
At week 18 | 22 | 2781.5 | 61.9–93.7 | 455 | 228 to ≥1448 | 19 | 2382.6 | 61.2–95.0 | 724 | 228–1029 |
Cumulativea | 263 | 26898.1 | 95.7–99.4 | ≥1448 228 | ≥1448 to ≥1448 | 266 | 27098.5 | 96.3-99.6 | 1152 | 1152 to ≥1448 |
At week 22 | 5 | 5100.0 | 98.6–100.0 | ≥1448 | 144–576 | 4 | 4100.0 | 98.6–100.0 | ≥1448 | 724 to ≥1448 |
Cumulativeb | 268 | 268100.0 | 98.6–100.0 | ≥1448 to ≥1448 | 270 | 270100.0 | 98.6–100.0 | ≥1448 | ≥1448 to ≥1448 |
Response . | Arm A . | . | . | . | . | Arm B . | . | . | . | . |
---|---|---|---|---|---|---|---|---|---|---|
. | Seroconversion . | . | . | Reciprocal titers . | . | Seroconversion . | . | . | Reciprocal titers . | . |
Poliovirus type 1 . | N . | N% . | 95% CI . | Median . | CI . | n . | N% . | 95% CI . | Median . | CI . |
At week 14 | 243 | 26890.7 | 86.5–93.9 | ≥1448 | ≥1448 to ≥1448 | 175 | 27064.8 | 58.8–70.5 | 288 | 228–455 |
At week 18 | 22 | 2588.0 | 68.8–97.5 | ≥1448 | 576 to ≥1448 | 67 | 9571.0 | 61.0–80.1 | 144 | 91–228 |
Cumulativea | 265 | 26898.9 | 96.8–998 | ≥1448 | ≥1448 to -≥1448 | 242 | 27089.6 | 85.4–93.0 | 455 | 362–576 |
At week 22 | 3 | 3100.0 | 20 | 2876.9 | 56.4–91.0 | 1300 | 910 to ≥1448 | |||
Cumulativeb | 268 | 268100.0 | 268 | 268100.0 | ≥1448 | ≥1448 to ≥1448 | ||||
Poliovirus type 2 | n | N% | 95% CI | Median | CI | n | N% | 95% CI | Median | CI |
At week 14 | 25 | 2689.3 | 6.1–13.5 | 23 | 18–57 | 180 | 27066.7 | 60.7–72.3 | 114 | 91–144 |
At week 18 | 168 | 24369.4 | 63.2–75.2 | 28 | 23–36 | 73 | 9081.1 | 71.5–88.6 | 91 | 57–144 |
Cumulativea | 193 | 26872.0 | 66.2–77.3 | 28 | 23–36 | 253 | 27093.7 | 90.1–96.3 | 181 | 181–228 |
At week 22 | 64 | 7585.3 | 75.3–92.4 | 114 | 57–114 | 2 | 1711.8 | 1.5–36.4 | 34 | 23–45 |
Cumulativeb | 257 | 26895.9 | 92.8–97.9 | 114 | 91–114 | 255 | 27094.4 | 91.0–96.9 | 144 | 114–181 |
Poliovirus type 3 | n | N% | 95% CI | Median | CI | n | N% | 95% CI | Median | CI |
At week 14 | 241 | 26889.9 | 85.7–93.3 | 724 | 724–910 | 247 | 27091.5 | 87.5–94.5 | 1152 | 1152 to ≥1448 |
At week 18 | 22 | 2781.5 | 61.9–93.7 | 455 | 228 to ≥1448 | 19 | 2382.6 | 61.2–95.0 | 724 | 228–1029 |
Cumulativea | 263 | 26898.1 | 95.7–99.4 | ≥1448 228 | ≥1448 to ≥1448 | 266 | 27098.5 | 96.3-99.6 | 1152 | 1152 to ≥1448 |
At week 22 | 5 | 5100.0 | 98.6–100.0 | ≥1448 | 144–576 | 4 | 4100.0 | 98.6–100.0 | ≥1448 | 724 to ≥1448 |
Cumulativeb | 268 | 268100.0 | 98.6–100.0 | ≥1448 to ≥1448 | 270 | 270100.0 | 98.6–100.0 | ≥1448 | ≥1448 to ≥1448 |
14, 18 and 22 weeks’ response includes impact of the following vaccine schedules: 14 weeks: Arm A (3bOPV) vs Arm B (2IPV).
a18 weeks: Arm A (4bOPV + IPV) vs Arm B (3IPV).
b22 weeks: Arm A (4bOPV + 2IPV) vs Arm B (3IPV + bOPV).
Seroconversion and median titers with 95% bootstrap CIs in per-protocol population
Response . | Arm A . | . | . | . | . | Arm B . | . | . | . | . |
---|---|---|---|---|---|---|---|---|---|---|
. | Seroconversion . | . | . | Reciprocal titers . | . | Seroconversion . | . | . | Reciprocal titers . | . |
Poliovirus type 1 . | N . | N% . | 95% CI . | Median . | CI . | n . | N% . | 95% CI . | Median . | CI . |
At week 14 | 243 | 26890.7 | 86.5–93.9 | ≥1448 | ≥1448 to ≥1448 | 175 | 27064.8 | 58.8–70.5 | 288 | 228–455 |
At week 18 | 22 | 2588.0 | 68.8–97.5 | ≥1448 | 576 to ≥1448 | 67 | 9571.0 | 61.0–80.1 | 144 | 91–228 |
Cumulativea | 265 | 26898.9 | 96.8–998 | ≥1448 | ≥1448 to -≥1448 | 242 | 27089.6 | 85.4–93.0 | 455 | 362–576 |
At week 22 | 3 | 3100.0 | 20 | 2876.9 | 56.4–91.0 | 1300 | 910 to ≥1448 | |||
Cumulativeb | 268 | 268100.0 | 268 | 268100.0 | ≥1448 | ≥1448 to ≥1448 | ||||
Poliovirus type 2 | n | N% | 95% CI | Median | CI | n | N% | 95% CI | Median | CI |
At week 14 | 25 | 2689.3 | 6.1–13.5 | 23 | 18–57 | 180 | 27066.7 | 60.7–72.3 | 114 | 91–144 |
At week 18 | 168 | 24369.4 | 63.2–75.2 | 28 | 23–36 | 73 | 9081.1 | 71.5–88.6 | 91 | 57–144 |
Cumulativea | 193 | 26872.0 | 66.2–77.3 | 28 | 23–36 | 253 | 27093.7 | 90.1–96.3 | 181 | 181–228 |
At week 22 | 64 | 7585.3 | 75.3–92.4 | 114 | 57–114 | 2 | 1711.8 | 1.5–36.4 | 34 | 23–45 |
Cumulativeb | 257 | 26895.9 | 92.8–97.9 | 114 | 91–114 | 255 | 27094.4 | 91.0–96.9 | 144 | 114–181 |
Poliovirus type 3 | n | N% | 95% CI | Median | CI | n | N% | 95% CI | Median | CI |
At week 14 | 241 | 26889.9 | 85.7–93.3 | 724 | 724–910 | 247 | 27091.5 | 87.5–94.5 | 1152 | 1152 to ≥1448 |
At week 18 | 22 | 2781.5 | 61.9–93.7 | 455 | 228 to ≥1448 | 19 | 2382.6 | 61.2–95.0 | 724 | 228–1029 |
Cumulativea | 263 | 26898.1 | 95.7–99.4 | ≥1448 228 | ≥1448 to ≥1448 | 266 | 27098.5 | 96.3-99.6 | 1152 | 1152 to ≥1448 |
At week 22 | 5 | 5100.0 | 98.6–100.0 | ≥1448 | 144–576 | 4 | 4100.0 | 98.6–100.0 | ≥1448 | 724 to ≥1448 |
Cumulativeb | 268 | 268100.0 | 98.6–100.0 | ≥1448 to ≥1448 | 270 | 270100.0 | 98.6–100.0 | ≥1448 | ≥1448 to ≥1448 |
Response . | Arm A . | . | . | . | . | Arm B . | . | . | . | . |
---|---|---|---|---|---|---|---|---|---|---|
. | Seroconversion . | . | . | Reciprocal titers . | . | Seroconversion . | . | . | Reciprocal titers . | . |
Poliovirus type 1 . | N . | N% . | 95% CI . | Median . | CI . | n . | N% . | 95% CI . | Median . | CI . |
At week 14 | 243 | 26890.7 | 86.5–93.9 | ≥1448 | ≥1448 to ≥1448 | 175 | 27064.8 | 58.8–70.5 | 288 | 228–455 |
At week 18 | 22 | 2588.0 | 68.8–97.5 | ≥1448 | 576 to ≥1448 | 67 | 9571.0 | 61.0–80.1 | 144 | 91–228 |
Cumulativea | 265 | 26898.9 | 96.8–998 | ≥1448 | ≥1448 to -≥1448 | 242 | 27089.6 | 85.4–93.0 | 455 | 362–576 |
At week 22 | 3 | 3100.0 | 20 | 2876.9 | 56.4–91.0 | 1300 | 910 to ≥1448 | |||
Cumulativeb | 268 | 268100.0 | 268 | 268100.0 | ≥1448 | ≥1448 to ≥1448 | ||||
Poliovirus type 2 | n | N% | 95% CI | Median | CI | n | N% | 95% CI | Median | CI |
At week 14 | 25 | 2689.3 | 6.1–13.5 | 23 | 18–57 | 180 | 27066.7 | 60.7–72.3 | 114 | 91–144 |
At week 18 | 168 | 24369.4 | 63.2–75.2 | 28 | 23–36 | 73 | 9081.1 | 71.5–88.6 | 91 | 57–144 |
Cumulativea | 193 | 26872.0 | 66.2–77.3 | 28 | 23–36 | 253 | 27093.7 | 90.1–96.3 | 181 | 181–228 |
At week 22 | 64 | 7585.3 | 75.3–92.4 | 114 | 57–114 | 2 | 1711.8 | 1.5–36.4 | 34 | 23–45 |
Cumulativeb | 257 | 26895.9 | 92.8–97.9 | 114 | 91–114 | 255 | 27094.4 | 91.0–96.9 | 144 | 114–181 |
Poliovirus type 3 | n | N% | 95% CI | Median | CI | n | N% | 95% CI | Median | CI |
At week 14 | 241 | 26889.9 | 85.7–93.3 | 724 | 724–910 | 247 | 27091.5 | 87.5–94.5 | 1152 | 1152 to ≥1448 |
At week 18 | 22 | 2781.5 | 61.9–93.7 | 455 | 228 to ≥1448 | 19 | 2382.6 | 61.2–95.0 | 724 | 228–1029 |
Cumulativea | 263 | 26898.1 | 95.7–99.4 | ≥1448 228 | ≥1448 to ≥1448 | 266 | 27098.5 | 96.3-99.6 | 1152 | 1152 to ≥1448 |
At week 22 | 5 | 5100.0 | 98.6–100.0 | ≥1448 | 144–576 | 4 | 4100.0 | 98.6–100.0 | ≥1448 | 724 to ≥1448 |
Cumulativeb | 268 | 268100.0 | 98.6–100.0 | ≥1448 to ≥1448 | 270 | 270100.0 | 98.6–100.0 | ≥1448 | ≥1448 to ≥1448 |
14, 18 and 22 weeks’ response includes impact of the following vaccine schedules: 14 weeks: Arm A (3bOPV) vs Arm B (2IPV).
a18 weeks: Arm A (4bOPV + IPV) vs Arm B (3IPV).
b22 weeks: Arm A (4bOPV + 2IPV) vs Arm B (3IPV + bOPV).
We analyzed the influence of maternal antibodies on vaccine response to IPV in the 2 arms. Two IPV doses at early age of 6 and 10 weeks in Arm B provided lower type 2 seroconversion of 66.7% (95% CI, 60.7–72.3), whereas delayed 2 doses at 14 and 18 weeks in Arm A provided much higher type 2 seroconversion of 85.3% (95% CI, 75.3–92.4). We further analyzed seroconversion obtained with respect to level of maternal antibodies in the study subjects. The level of maternal antibodies was categorized into high (≥64 baseline reciprocal antibody titer) and low (<64). Besides the age of IPV administration, the immune response varied according to the level of maternal antibodies within early and late vaccination. For example, type 2 seroconversion in 2 early IPV-dose subgroups of Arm B was as low as 23.7% in the high maternal antibody category and much higher at 89.3% in the low maternal antibody category (Figure 3).
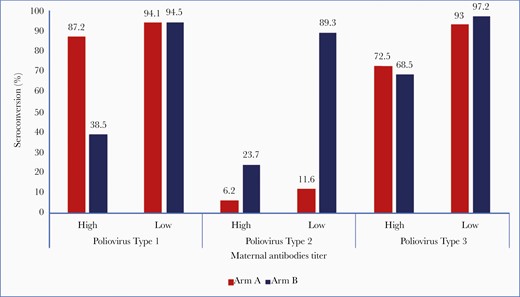
Influence of maternal antibodies on seroconversion at 14 weeks in Arms A and B.
The effect of maternal antibodies has more to do with IPV than OPV and was also demonstrated by types 1 and 3 responses. For serotype 1, 47.3% of participants in Arm A (bOPV arm) and 51.5% in Arm B (IPV arm) had high maternal antibody titers and for serotype 3, at 14.4% and 20.1%, respectively. In the subgroup with high maternal antibody titers for serotype 1, seroconversion was much lower at 34.3% in Arm B (IPV Arm) compared to 86.3% in Arm A (bOPV). However, in the low maternal antibody group, there was comparable seroconversion of 94% in both the arms. Seroconversion achieved against poliovirus type 3 was 71.1% and 93% in the high and low maternal antibody titers subgroups in Arm A and 68.5%, 97.2%, respectively in Arm B (Figure 3).
In total, 1371 subjects had AEs (663 in Arm A and 708 in Arm B). The most common AEs reported were cough, catarrh, fever, rash, and diarrhea. The distribution of AEs is shown in Supplementary Table S1. A total of 12 severe AEs were reported in the trial. These are shown in detail in Supplementary Table S2. Detailed clinical evaluation and laboratory investigations were undertaken for SAEs to the extent possible, reviewed by the principal investigator and an independent Data and Safety Monitoring Board at WHO, and assessed to be not causally related.
DISCUSSION
Our findings demonstrated for the first time in an African population a high immunogenicity of the 4bOPV+1IPV EPI polio vaccine schedule. The schedule achieved >95% seroconversion against poliovirus types 1 and 3. Seroconversion of 72% against poliovirus type 2 was quite high for 1 dose of IPV. These EPI schedule data in Nigeria are comparable to those obtained from similar studies in India [14], Latin America [23], Chile [24], and Pakistan [25]. The median antibody titers were significantly higher following the 4bOPV+1IPV EPI schedule compared with the IPV-only schedule, except for serotype 2. This is not surprising given that the EPI schedule contained 5 doses of poliovirus vaccine compared with 3 doses in the IPV-only schedule. Furthermore, with IPV administered in this early schedule, it is not unexpected that the immunogenicity is negatively affected by high maternal antibodies [12, 26, 27]. The relevance of the higher median titer for antibody persistence remains unknown. However, it appears that the initial seroconversion signals an immune response that can be boosted later in life, even if the preboosting antibody titer had decreased to nondetectable levels (reciprocal titer <8) [28]. The addition of a second dose of IPV to the EPI schedule provided a second opportunity to induce or boost immunity against poliovirus type 2. This second dose significantly increased the seroconversion rate to poliovirus type 2 from 72.0% to 95.9%. In this trial, the seroconversion rate to type 2 with 1 or 2 doses of IPV is consistent with rates in other trials [14, 29]. In addition, the second dose of IPV completely closed the remaining immunity gaps to poliovirus types 1 and 3. Our study also allowed for a comparison of 2 different 2-dose IPV effects against poliovirus type 2. The seroconversion rate against poliovirus type 2 administered at 14 and 18 weeks (Arm A) was 95.9%, whereas the rate for IPV given at 6 and 10 weeks (Arm B) was 66.7%. These results again confirm that IPV administered at an earlier age is less immunogenic than IPV administered at a later age due to interference by maternally derived antibodies [12, 25, 26, 29–31]. The interference by maternal antibodies was directly assessed in our trial as well. We demonstrated significantly lower seroconversion rates with higher maternal antibody titers to all 3 serotypes at week 14 in Arm B, but only to type 3 in Arm A. We were surprised to find that the effect of the maternal antibody to type 3 in Arm A was still demonstrable at 14 weeks of age after 3 doses of bOPV. Furthermore, the addition of a single dose of bOPV at 18 weeks to the 3-dose IPV schedule essentially closed the remaining immunity gaps to poliovirus type 1 (seroconversion rates increased from 89.6% to 97.0%) and 3 (seroconversion rates increased from 98.5% to 100%). Because bOPV does not contain type 2 antigen, there was no effect on serotype 2 conversion rates (93.7% to 94.4%). The study clearly demonstrated that polio vaccines currently in use, bOPV and IPV, work well together for boosting immunity whether bOPV is followed by IPV or vice versa. There were some limitations of the trial. The trial design was more of a programmatic kind as to how immunogenic is the current EPI schedule, what could be the gains with addition of second dose of IPV to the schedule (Arm A) and its comparisons made with IPV only (Arm B) in the same 6-, 10-, and 14-week schedule. The limitations are that the 2 arms do not receive corresponding comparable vaccines or doses, and the comparisons are not that straightforward. Oral poliovirus vaccine and IPV immunogenicity and titers are known to be different with different number of doses and age of administration as obtained or confirmed in this study also. Moreover, the IPV-only schedule for the future may comprise a lower number of doses given farther apart in ages ranging from 14 weeks to 9 months. The immunogenicity data of IPV in the 6, 10- and 14-weeks schedule provides support for potential IPV standalone or IPV containing Hexavalent vaccine schedule in future.
One unexpected finding was a low proportion (9.3%) of type 2 seroconversion in Arm A at 14 weeks without any type 2 vaccination. This could be the result of type 2 exposure in the community due to continued use of tOPV after the switch or the undetected circulation of vaccine-derived poliovirus type 2 (cVDPV2). We investigated the possibility of study subjects receiving additional vaccines outside the study, but we did not find any evidence. The few who received the wrong vaccine in error were removed from analysis. Another explanation for low-grade type 2 seroconversion in some bOPV recipients at 14 weeks could be undetected cVDPV2. Although only 1 case of cVDPV2 and 2 positive tests in healthy contacts were detected in the Nigeria Sokoto area in 2016, there were none reported from the study area during the study period. Because large-scale cVDPV2 outbreaks happened in subsequent years (a total of 131 VDPV2 isolates in 2018 and 100 in 2019 in Nigeria), mostly in northern states of Nigeria, but many in the study area of Kwara province, undetected circulation during the study period cannot be entirely ruled out. IPV not providing mucosal immunity per se and the period after tOPV bOPV switch, reduced mucosal immunity against serotype 2 could have facilitated the ongoing low grade VDPV2 transmission in these populations. Another potential explanation could be cross-immunity to serotype 2 from bOPV, but this level of cross-immunity is unlikely.
The high number of AEs conform to the high burden of infectious diseases in the local population and the common prevailing infections peculiar to the study age group participants. Therefore, this underscores the need for sustained high immunization coverage and other infection prevention strategies in the country.
Supplementary Data
Supplementary materials are available at The Journal of Infectious Diseases online. Consisting of data provided by the authors to benefit the reader, the posted materials are not copyedited and are the sole responsibility of the authors, so questions or comments should be addressed to the corresponding author.
Supplementary Table S1. Number of adverse events by study arms.
Supplementary Table S2. Severe adverse events (SAEs) by the study arms.
Notes
Acknowledgments. We acknowledge the collaboration between National Primary Health Care Development Agency (NPHCDA), Pediatrics Association of Nigeria (PAN), and World Health Organization (WHO), which all worked closely together for conceptualization, planning, and implementation of this trial. Chinenye Ekpemauzor and Abdulkadir Bello from NPHCDA played a critical role in vaccine supplies and cold chain logistics. The zonal NPHCDA of Enugu and Kwara state primary healthcare development agencies provided facilities at the study sites. Research physicians, project managers, and their teams at the 4 sites (Institute of Child Health, University of Nigeria Teaching Hospital, Enugu; Uwani Cottage Hospital Enugu; Department of Paediatrics and Child Health, University of Ilorin Teaching Hospital; and Children’s Specialist Hospital, Ilorin) carried out study procedures immaculately. The Heads of the study sites and their staff were highly supportive on administrative and other issues. We thank the laboratory team at the Centers for Disease Control and Prevention (Atlanta, GA) for carrying out neutralization assays of the sera of the study participants. Finally, we acknowledge the parents, guardians, and the participating infants of the study for their courage, timely visits, and bearing with the study procedures.
Disclaimer. The authors alone are responsible for the views expressed in this article and they do not necessarily represent the views, decisions, or policies of the institutions with which they are affiliated. The funders had no involvement in the design, execution, or writing of the manuscript.
Financial support. This work was supported by a grant from the Rotary International (PP14BMF0003), funded through the Polio Research Committee on Technical Merit and Programmatic Relevance.
Potential conflicts of interest. B. N. T. received grant and technical support from WHO for the study. D. E. reports grants from WHO, during the conduct of the study, and is a member of the National Immunisation Technical Advisory Group and the National Expert Committee on Adverse Event Following Immunisation. All authors have submitted the ICMJE Form for Disclosure of Potential Conflicts of Interest. Conflicts that the editors consider relevant to the content of the manuscript have been disclosed.