-
PDF
- Split View
-
Views
-
Cite
Cite
Paulina Andrade, Parnal Narvekar, Magelda Montoya, Daniela Michlmayr, Angel Balmaseda, Josefina Coloma, Eva Harris, Primary and Secondary Dengue Virus Infections Elicit Similar Memory B-Cell Responses, but Breadth to Other Serotypes and Cross-Reactivity to Zika Virus Is Higher in Secondary Dengue, The Journal of Infectious Diseases, Volume 222, Issue 4, 15 August 2020, Pages 590–600, https://doi.org/10.1093/infdis/jiaa120
- Share Icon Share
Abstract
The 4 antigenically distinct serotypes of dengue virus (DENV) share extensive homology with each other and with the closely related Zika flavivirus (ZIKV). The development of polyclonal memory B cells (MBCs) to the 4 DENV serotypes and ZIKV during DENV infection is not fully understood.
In this study, we analyzed polyclonal MBCs at the single-cell level from peripheral blood mononuclear cells collected ~2 weeks or 6–7 months postprimary or postsecondary DENV infection from a pediatric hospital-based study in Nicaragua using a Multi-Color FluoroSpot assay.
Dengue virus elicits robust type-specific and cross-reactive MBC responses after primary and secondary DENV infection, with a significantly higher cross-reactive response in both. Reactivity to the infecting serotype dominated the total MBC response. Although the frequency and proportion of type-specific and cross-reactive MBCs were comparable between primary and secondary DENV infections, within the cross-reactive response, the breadth of MBC responses against different serotypes was greater after secondary DENV infection. Dengue virus infection also induced cross-reactive MBC responses recognizing ZIKV, particularly after secondary DENV infection.
Overall, our study sheds light on the polyclonal MBC response to DENV and ZIKV in naive and DENV-preimmune subjects, with important implications for natural infections and vaccine development.
Dengue virus (DENV), consisting of 4 distinct serotypes (DENV1–4), is a mosquito-borne flavivirus that circulates in 128 countries and causes up to ~390 million human infections annually [1, 2]. The majority of DENV infections are asymptomatic; however, ~25% of infections develop classical dengue fever, and a small percentage can progress to dengue hemorrhagic fever/dengue shock syndrome, a potentially fatal complication characterized by plasma leakage leading to shock [3]. Epidemiological studies indicate that severe disease is more likely to occur during a secondary infection with a different DENV serotype than the primary infection [4, 5]. Recent data from cohort studies have shown that specific prior DENV antibody titers are associated with severity in secondary infections [6, 7].
Immunological memory in B cells involves the long-term production of antibodies in serum by bone marrow-resident long-lived plasma cells (LLPCs) [8] and the generation of memory B cells (MBCs) that circulate in the blood and lymphoid tissues and are reactivated by subsequent antigen exposure [9]. Analysis of serum during natural DENV infections shows that primary DENV infections result in strong serotype-specific neutralizing responses and that secondary DENV infections induce broadly cross-reactive antibodies capable of binding and neutralizing multiple DENV serotypes [10, 11]. Memory B-cell responses after primary DENV have been found to be predominantly serotype-specific, whereas MBCs after secondary infection have been shown to be predominantly serotype cross-reactive [12]. Monoclonal antibodies (mAbs) derived from MBCs show that DENV-immune individuals have cross-reactive and type-specific MBCs many years after infection [13–15]. During secondary DENV infection, the activation of pre-existing MBCs contributes to an extensive plasmablast expansion [16–18] that results in a rapid increase in neutralizing antibody titers [19] and temporary cross-protection against all 4 serotypes but can also be associated with increased risk of severe dengue [20]. Although polyclonal MBC studies have broadly analyzed DENV MBC populations during primary and secondary infections, little is known about the potential of single MBCs to elicit type-specific and cross-reactive antibodies [12].
In this work, we analyzed the MBC response at a single-cell level in primary and secondary DENV infections from a Nicaraguan pediatric hospital-based study. Using a Multi-Color FluoroSpot assay [21, 22], we determined type specificity and cross-reactivity of early and late convalescent polyclonal MBC responses and the neutralization capacity of polyclonal MBC culture supernatants. Understanding the development of type-specific and cross-reactive polyclonal MBC responses will help better assess the role of antibody responses during dengue and inform vaccine development. The recent emergence of Zika virus (ZIKV) and its cocirculation in dengue-endemic areas has implications for the epidemiology of dengue and Zika, because the cross-reactive responses between these flaviviruses can potentially modulate long-term ZIKV and DENV responses [23–25]. Thus, we also analyzed DENV and ZIKV cross-reactive MBCs among primary versus secondary DENV infections before the introduction of ZIKV into Nicaragua.
METHODS
Ethics Statement
The Pediatric Dengue Hospital-based Study (2005–present) at the Hospital Infantil Manuel de Jesús Rivera, the National Pediatric Reference Hospital in Managua, Nicaragua, was reviewed and approved by the Institutional Review Boards of the University of California, Berkeley, and the Nicaraguan Ministry of Health. Parents or legal guardians of all subjects provided written informed consent, and subjects 6 years of age and older provided oral assent.
Study Design and Population
Children between 6 months and 14 years of age suspected of having dengue (<7 days of illness) were enrolled [26]. In this study, peripheral blood mononuclear cells (PBMCs) from reverse-transcription polymerase chain reaction (RT-PCR)-confirmed DENV-positive cases (n = 32) collected at early convalescence (days 13–21 postonset of symptoms, here referred to as ~2 weeks postinfection) and late convalescence (6–7 months) after primary or secondary symptomatic DENV1, DENV2, or DENV3 infections were analyzed (Table 1). Paired samples from early and late convalescence from the same individual were selected for analysis. Due to unforeseen circumstances, however, unpaired samples had to be used for secondary DENV3 infections. The PBMCs were collected between 2008 and 2016, before the Zika epidemic in Managua in 2016. Primary and secondary dengue cases were defined by a DENV inhibition enzyme-linked immunosorbent assay (ELISA) titer of <2560 or ≥2560, respectively, in early convalescent-phase samples as defined previously [27–29]. The PBMCs were prepared as previously described [30, 31].
Infectiona . | . | DENV1 . | DENV2 . | DENV3 . |
---|---|---|---|---|
Primary | Number, n | 4 | 5 | 5 |
Sex, n % female | 2 (50) | 2 (40) | 2 (66.6) | |
Age, mean (SD) | 10 (2) | 7 (5) | 7 (7) | |
Day of early convalescent sample collectionb, mean (SD) | 15 (1) | 16 (4) | 15 (2) | |
Month of late convalescent sample collectionc, mean (SD) | 7 (0.35) | 7 (0.6) | 7 (0.5) | |
Secondary | Number, n | 5 | 5 | 8d |
Sex, n % female | 3 (60) | 2 (40) | 1 (12.5) | |
Age, mean (SD) | 12 (4) | 12 (1) | 11 (3) | |
Day of early convalescent sample collectionb, mean (SD) | 15 (1) | 14 (1) | 14 (1) | |
Month of late convalescent sample collectionc, mean (SD) | 6 (0.4) | 6 (0.3) | 6 (0.3) |
Infectiona . | . | DENV1 . | DENV2 . | DENV3 . |
---|---|---|---|---|
Primary | Number, n | 4 | 5 | 5 |
Sex, n % female | 2 (50) | 2 (40) | 2 (66.6) | |
Age, mean (SD) | 10 (2) | 7 (5) | 7 (7) | |
Day of early convalescent sample collectionb, mean (SD) | 15 (1) | 16 (4) | 15 (2) | |
Month of late convalescent sample collectionc, mean (SD) | 7 (0.35) | 7 (0.6) | 7 (0.5) | |
Secondary | Number, n | 5 | 5 | 8d |
Sex, n % female | 3 (60) | 2 (40) | 1 (12.5) | |
Age, mean (SD) | 12 (4) | 12 (1) | 11 (3) | |
Day of early convalescent sample collectionb, mean (SD) | 15 (1) | 14 (1) | 14 (1) | |
Month of late convalescent sample collectionc, mean (SD) | 6 (0.4) | 6 (0.3) | 6 (0.3) |
aNo significant differences were found for age, sex and time of sample collection for primary and secondary DENV1, DENV2 and DENV3 infections or for primary versus secondary DENV1, DENV2, or DENV3 infection.
bDays postonset of symptoms.
cMonths postonset of symptoms.
dEarly and late convalescence samples are not from the same individual.
Infectiona . | . | DENV1 . | DENV2 . | DENV3 . |
---|---|---|---|---|
Primary | Number, n | 4 | 5 | 5 |
Sex, n % female | 2 (50) | 2 (40) | 2 (66.6) | |
Age, mean (SD) | 10 (2) | 7 (5) | 7 (7) | |
Day of early convalescent sample collectionb, mean (SD) | 15 (1) | 16 (4) | 15 (2) | |
Month of late convalescent sample collectionc, mean (SD) | 7 (0.35) | 7 (0.6) | 7 (0.5) | |
Secondary | Number, n | 5 | 5 | 8d |
Sex, n % female | 3 (60) | 2 (40) | 1 (12.5) | |
Age, mean (SD) | 12 (4) | 12 (1) | 11 (3) | |
Day of early convalescent sample collectionb, mean (SD) | 15 (1) | 14 (1) | 14 (1) | |
Month of late convalescent sample collectionc, mean (SD) | 6 (0.4) | 6 (0.3) | 6 (0.3) |
Infectiona . | . | DENV1 . | DENV2 . | DENV3 . |
---|---|---|---|---|
Primary | Number, n | 4 | 5 | 5 |
Sex, n % female | 2 (50) | 2 (40) | 2 (66.6) | |
Age, mean (SD) | 10 (2) | 7 (5) | 7 (7) | |
Day of early convalescent sample collectionb, mean (SD) | 15 (1) | 16 (4) | 15 (2) | |
Month of late convalescent sample collectionc, mean (SD) | 7 (0.35) | 7 (0.6) | 7 (0.5) | |
Secondary | Number, n | 5 | 5 | 8d |
Sex, n % female | 3 (60) | 2 (40) | 1 (12.5) | |
Age, mean (SD) | 12 (4) | 12 (1) | 11 (3) | |
Day of early convalescent sample collectionb, mean (SD) | 15 (1) | 14 (1) | 14 (1) | |
Month of late convalescent sample collectionc, mean (SD) | 6 (0.4) | 6 (0.3) | 6 (0.3) |
aNo significant differences were found for age, sex and time of sample collection for primary and secondary DENV1, DENV2 and DENV3 infections or for primary versus secondary DENV1, DENV2, or DENV3 infection.
bDays postonset of symptoms.
cMonths postonset of symptoms.
dEarly and late convalescence samples are not from the same individual.
Virus Propagation and Purification
Aedes albopictus C6/36 cells were grown to 85% confluence in minimal essential medium (Life Technologies) supplemented with 10% fetal bovine serum ([FBS] Corning), 1% GlutaMAX, 1% HEPES, and 1% penicillin/streptomycin. Cells were infected with ZIKV (Nica 2–16), DENV1 (DV1-N5575-10), DENV2 (DV2-N8872-12), DENV3 (DV3-N6629-10), or DENV4 (DV4-N703-99) for 5 days. The infectious supernatant was concentrated using a 100-kDa Amicon filter (Millipore), then layered on a discontinuous 20/55% OptiPrep (Sigma-Aldrich) density gradient, and centrifuged at 273 000 ×g at 4°C for 2 hours. Purified virus was collected from the density interface and stored at −80°C. Virus quality control was performed as described previously [31] and detailed in Supplementary Materials.
Monoclonal Antibodies and Conjugation to Qdots
Detection mAbs (anti-DENV1 E95, anti-DENV2 E96, anti-DENV3 5J7, anti-DENV4 E88, and anti-ZIKV ZKA64) were assessed by indirect ELISA as previously described [22] (see Supplementary Methods). Four mAbs (100–125 μg) were conjugated to dibenzocyclooctynol-modified Qdot nanocrystals (Qdot525, Qdot625, Qdot700, and Qdot800) using a Site-Click labeling kit (Life Technologies) according to the manufacturer’s instructions. One mAb was labeled using the EZ-Link sulfo NHS LC Biotinylation kit (Thermo Fisher Scientific) and detected with Qdot 565-labeled streptavidin.
Multi-Color FluoroSpot
The Multi-Color FluoroSpot was performed as previously described [22]. In brief, activated IPFL FluoroSpot plates (Cellular Technology Limited) were coated with 2 μg/well Fcγ fragment-specific antihuman immunoglobulin (Ig)G (Jackson ImmunoResearch) for 2 hours at 37°C. Plates were then blocked with complete Roswell Park Memorial Institute (RPMI) 1640 medium (Gibco) containing 10% FBS, 1% GlutaMax, 1% HEPES, and 1% penicillin/streptomycin overnight. The PBMCs were thawed in complete RPMI 1640 containing 1 μL/mL DNase I and activated with interleukin-2 (1000 U/mL) and R848 (2.5 μg/μL) for 5 days [21]. The activated PBMCs were added to each well in eight 2-fold serial dilutions and incubated for 48 hours at 37°C. Next, plates were washed and incubated with purified virions (referred to hereafter as antigen) (20 μg/mL in 1× phosphate-buffered saline) for 1 hour at 37°C and then blocked with human gamma-globulin (1:500). Finally, plates were incubated with Qdot-labeled mAbs (1:1000) for 1 hour at 37°C and visualized using a CTL ImmunoSpot S6 Micro-Analyzer (Cellular Technology Limited). To determine total IgG antibody-secreting cells (ASCs), activated PBMCs were plated in 1:2 serial dilutions. Immunoglobulin G-positive spots were visualized using a polyclonal phycoerythrin-labeled antihuman IgG detection mAb (H+L) (Life Technologies). Spots were then counted using the CTL analyzer.
Flow Cytometry-Based Neutralization Assay
A flow cytometry-based neutralization assay using reporter virus particles was performed with MBC supernatants as detailed in Supplementary Materials [32, 33].
Statistical Analysis
FluoroSpot results were analyzed using Prism 6 software (GraphPad Software) using a 2-tailed Wilcoxon test and Mann-Whitney test for paired and unpaired comparisons of non-parametric data, respectively. For multiple comparisons, FluoroSpot data were analyzed using a one-way and two-way analysis of variance with a Tukey’s multiple comparisons test.
RESULTS
Responses to the Infecting Serotype Dominate Type-Specific and Cross-Reactive Memory B-Cell Responses During Primary and Secondary Dengue Virus Infection
To study the development of the DENV MBC response, early and late convalescent PBMC samples collected ~2 weeks and 6–7 months postinfection, respectively, from 32 RT-PCR-confirmed primary and secondary DENV1, DENV2, and DENV3 cases were analyzed (Table 1). The Multi-Color FluoroSpot assay was used to determine the specificity of MBCs to all 4 DENV serotypes at the single-cell level [21]. The proportion of MBC responses that bound to DENV1–4 was calculated over total antigen response per well. Type-specific responses were defined as MBCs binding to one antigen and cross-reactive responses as MBCs binding to multiple DENV antigens.
As expected, during primary DENV infection, type-specific MBCs reacted only towards virions from the infecting serotype, and these responses were maintained over time (Figure 1A). During secondary DENV infection, type-specific MBCs additionally recognized virions from presumably previous infecting serotypes (Figure 1B). Analysis of serum from a subset of secondary patients showed that neutralizing antibody titers coincided in the majority of cases (9 of 11 [82%]) with the results from the Multi-Color FluoroSpot regarding prior heterotypic exposure. Of all type-specific MBC responses, an average proportion of 71% was due to the currently infecting serotype at ~2 weeks postinfection (Supplementary Figure 1). The responses towards the infecting or heterotypic serotype were maintained in secondary DENV infections into late convalescence (Figure 1B).
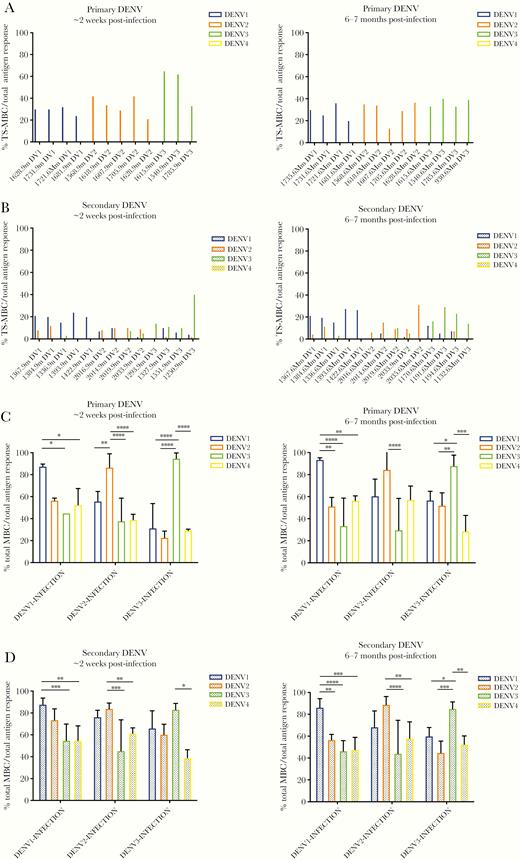
Analysis of serotype specificity of type-specific and cross-reactive memory B-cell (MBC) responses. Proportion of dengue virus (DENV) type-specific MBCs recognizing DENV1–4 after (A) primary and (B) secondary infection at ~2 weeks (left panel) and 6–7 months (right panel) postinfection. Proportion of total type-specific and cross-reactive responses to DENV1–4 after (C) primary and (D) secondary DENV infections at ~2 weeks (left panel) and 6–7 months (right panel) postinfection. The mean and standard deviation are shown for C and D. Significance was determined by a two-way analysis of variance multiple comparisons test: *, P < .05; **, P < .01; ***, P < .001; ****, P < .0001. Primary DENV1, n = 4; primary DENV2, n = 5; primary DENV3, n = 3 at ~2 weeks postinfection and primary DENV1, n = 4; primary DENV2, n = 5, and primary DENV3, n = 4 at 6–7 months postinfection. Secondary DENV1, n = 5; secondary DENV2, n = 4; and secondary DENV3, n = 4 at ~2 weeks postinfection and secondary DENV1, n = 5; secondary DENV2, n = 4; and secondary DENV3, n = 4 at 6–7 months postinfection.
Overall, analysis of cross-reactive MBCs revealed similar development of antigen-specific responses across the different serotypes in primary and secondary infections (Supplementary Figure 2B). When analyzing total MBCs, the response towards the infecting serotype was dominant after primary DENV infection (Figure 1C). Thus, at ~2 weeks post-DENV1 infection, 87% of the MBCs recognized DENV1 virions compared with DENV3 (45%) and DENV4 (52%). At 6–7 months postinfection, the responses towards the infecting DENV1 (94%) were even higher than MBC responses to the other serotypes (Figure 1C). Likewise, homologous DENV2 MBCs were significantly higher than MBCs recognizing DENV1, DENV3, and DENV4 ~2 weeks post-DENV2 infection, although by 6–7 months postinfection, the proportion of DENV2 MBCs remained significantly higher only to DENV3 virions. Likewise, DENV3 infections resulted in MBCs with significantly higher reactivity against DENV3 than against DENV1, DENV2, and DENV4 antigens at both ~2 weeks and 6–7 months postinfection (Figure 1C).
Likewise, the greatest reactivity of total MBC responses in secondary DENV infections was to the infecting serotype; this was most pronounced at late convalescence (Figure 1D). At ~2 weeks post-DENV1 secondary infection, the proportion of MBCs to the homologous antigen was significantly higher (87%) than those recognizing DENV3 (55%) and DENV4 (55%) antigens. At 6–7 months postinfection, this proportion (86%) was significantly higher than all heterologous serotypes (57%, 47%, and 48% DENV2–4, respectively). After DENV2 infection, cross-reactive responses towards the infecting serotype were significantly higher at early (84%) and late (89%) convalescence when compared with DENV3 (45% and 44%, respectively) and DENV4 (61% and 58%, respectively) antigen responses. Finally, DENV3 infections resulted in significantly higher DENV3-specific MBC responses compared to DENV4 antigen responses at early convalescence and compared to all heterologous serotypes by late convalescence (Figure 1D).
To analyze the functionality of DENV MBC-derived antibodies, we performed neutralization assays on the supernatants of activated MBCs from DENV1–3 infections. Primary infections resulted in MBC-derived antibodies that neutralized predominantly the infecting serotype, but MBC-derived antibodies from secondary infections neutralized all 4 serotypes (Supplementary Figure 3).
Primary and Secondary Dengue Virus Infections Induce More Cross-Reactive Than Type-Specific Memory B Cells
To analyze whether having experienced 1 versus more than 1 DENV infection affected the profile of MBC responses, the frequency (number of type-specific or cross-reactive MBCs over total IgG ASCs) and proportion of type-specific and cross-reactive responses were compared in primary versus secondary DENV infections. The frequency of cross-reactive responses was significantly higher than type-specific responses in both primary and secondary DENV infections at early (3.6% vs 1.7%, P < .05 and 4.3% vs 1.4%, P < .001, respectively) and late convalescence (3.2% vs 1.6%, P < .001 and 4% vs 1.5%, P < .0001, respectively) (Figure 2A and B). Likewise, the proportion of cross-reactive MBC responses at early and late convalescence was significantly greater than type-specific MBCs (acute, 32% vs 69% [P < .05]; convalescent, 33% vs 67% [P < .001]) (Supplementary Figure 4A). Similar but more pronounced responses were detected in secondary DENV infection samples, in which type-specific MBC responses (~2 weeks, 20%; 6–7 months, 28%) were lower than cross-reactive MBCs (~2 weeks, 80% [P < .01]; 6–7 months, 74% [P < .01]) (Supplementary Figure 4B).
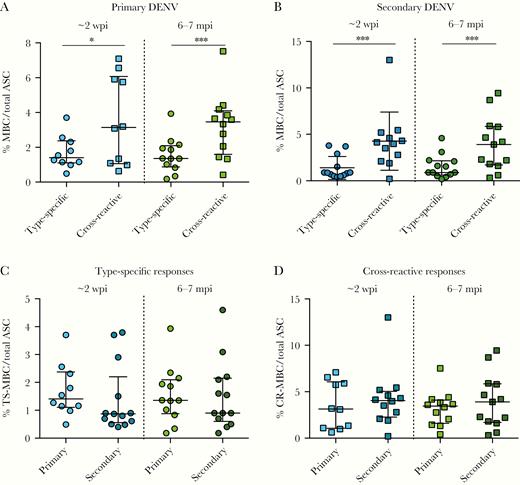
Development of type-specific and cross-reactive memory B-cell (MBC) responses after dengue virus (DENV) infection. Frequency of DENV type-specific and DENV cross-reactive immunoglobulin G-secreting MBCs over total antibody-secreting cells (ASCs) in (A) primary and (B) secondary DENV infections at ~2 weeks (left) and 6–7 months (right) postinfection. The frequency of (C) DENV type-specific and (D) DENV cross-reactive MBC responses in primary and secondary infections at ~2 weeks and 6–7 months postinfection. The mean and standard deviation are shown for all graphs. Significance was determined by a Wilcoxon test for A and B and a Mann-Whitney for test for C and D: *, P < .05; ***, P < .001. At ~2 weeks postinfection, primary DENV, n = 10; secondary DENV, n = 13. At 6–7 months postinfection, primary DENV, n = 12; secondary DENV, n = 13. mpi, months postinfection; wpi, weeks postinfection.
Our results also demonstrate that the mean frequency of type-specific MBCs was similar for primary and secondary DENV infections at ~2 weeks and at 6–7 months postinfection (Figure 2C). Cross-reactive MBCs presented similar frequencies during early convalescence of primary and secondary DENV infections as well as late convalescence (Figure 2D). The frequency of type-specific and cross-reactive responses was maintained over time in both primary and secondary DENV infections (Figure 2C and D). A similar pattern was observed when the proportion of type-specific and cross-reactive responses in primary and secondary DENV infections was analyzed (Supplementary Figure 4B).
The Breadth of Cross-Reactive Memory B Cells Increases After Secondary Dengue Virus Infection
To understand whether the quality of cross-reactive responses changes after experiencing more than 1 DENV infection, the proportion of cross-reactivity developed towards 2, 3, and 4 DENV serotypes was analyzed over time in primary and secondary infections. Primary DENV infections developed similar MBC cross-reactivity across different numbers of serotypes in early (Figure 3A) and late (Figure 3B) convalescence. However, in secondary DENV infections, cross-reactivity towards 4 serotypes was significantly higher when compared with the cross-reactivity towards 2 or 3 serotypes (44% vs 26%, P < .001 and 30%, P < .01, respectively) at ~2 weeks (Figure 3A) and 6–7 months (46% vs 29%, P < .01; 26%, P < .001) postinfection (Figure 3B). Finally, the proportion of cross-reactive MBC responses to all 4 serotypes was significantly higher in secondary compared with primary DENV infections at early and late convalescence (Figure 3A and B).
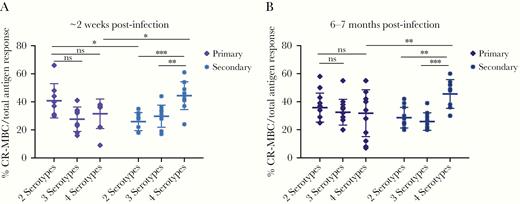
Comparison of the proportion of cross-reactive memory B-cell (CR-MBC) responses to different numbers of dengue virus (DENV) serotypes. Samples were collected after primary and secondary DENV infection at (A) ~2 weeks and (B) 6–7 months postinfection and stratified by the number of DENV serotypes. The mean and standard deviation are shown for both graphs. Significance was determined by a two-way analysis of variance multiple comparisons test: *, P < .05; **, P< .01; ***, P < .001. At ~2 weeks postinfection, primary DENV, n = 10; secondary DENV, n = 13. At 6–7 months postinfection, n = 13 for both groups. ns, not significant.
Secondary Dengue Virus Infection Generates Higher Cross-Reactive Memory B Cells Towards Zika Virus Than Primary Infection
To expand analysis of the breadth of cross-reactivity, MBC responses that recognized ZIKV were determined. Proportion and frequency analysis showed that ZIKV was recognized after both primary and secondary DENV infections (Supplementary Figure 5) in samples naive to ZIKV. The proportion of DENV/ZIKV cross-reactive responses compared with DENV-serotype cross-reactive responses was analyzed among total cross-reactive MBCs (Figure 4). In primary DENV infections, the proportion of DENV/ZIKV cross-reactivity was lower than the proportion of MBCs cross-reacting only to DENV at ~2 weeks and 6–7 months postinfection (Figure 4A). For secondary DENV, the proportion of DENV/ZIKV cross-reactive MBCs was only lower at ~2 weeks postinfection (Figure 4B). We then compared DENV/ZIKV cross-reactivity in primary versus secondary DENV infection and found that the frequency and proportion of DENV/ZIKV cross-reactivity increases after >1 DENV infection (Figure 4C and D). At early convalescence, the proportion and frequency of DENV/ZIKV MBC cross-reactivity in secondary DENV infections was 27.5% and 2.3%, respectively, and was higher than in primary DENV infections (13.2%, P < .05; 0.8%, P < .01). At late convalescence, the proportion of DENV/ZIKV cross-reactivity was also higher in secondary when compared with primary DENV infection (41% vs 23%; P < .01) (Figure 4C). Dengue virus/ZIKV cross-reactive responses were maintained from ~2 weeks until 6–7 months post-DENV infection. When the proportion of DENV/ZIKV cross-reactive responses was analyzed in relation to the infecting serotypes, secondary DENV3 developed a higher proportion of DENV/ZIKV cross-reactive MBCs than DENV1 and DENV2 infections (45% vs 23% [P < .05] and 20% [P < .05], respectively) at ~2 weeks postinfection (Supplementary Figure 6). Finally, to analyze whether DENV/ZIKV cross-reactive MBC antibodies could neutralize ZIKV, we performed neutralization assays on supernatants from activated MBCs. We found that MBC-derived antibodies developed after primary and secondary DENV infection have the capacity to neutralize ZIKV, with secondary infections trending higher than primary infections (P = .067) (Supplementary Figure 7).
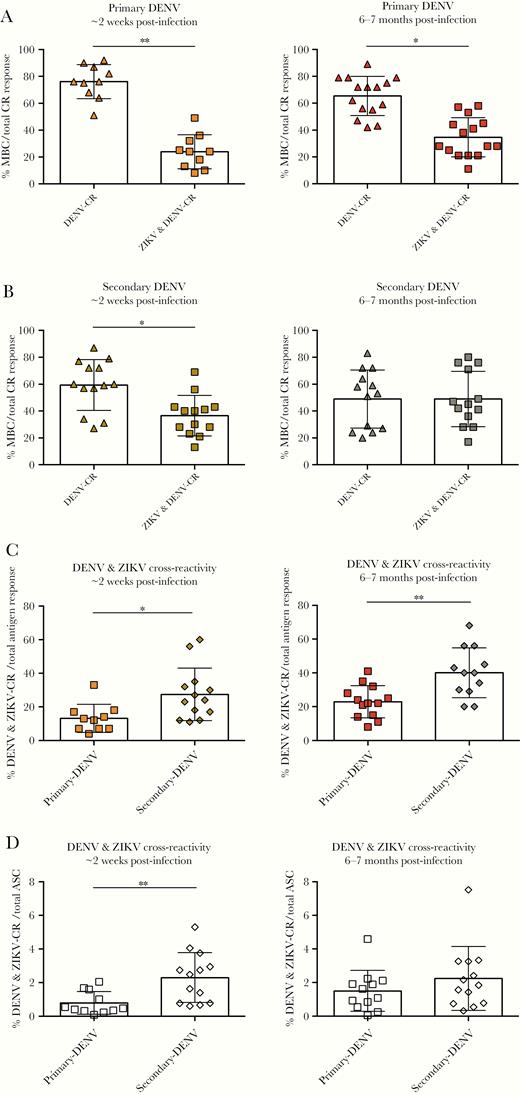
Dengue virus (DENV) and Zika virus (ZIKV) cross-reactive (CR) memory B-cell (MBC) responses developed after primary or secondary DENV infections. Proportion of DENV and DENV/ZIKV cross-reactive MBCs over total CR antigen responses after (A) primary and (B) secondary DENV at ~2 weeks (left panel) and 6–7 months (right panel) postinfection. Comparison of primary and secondary DENV/ZIKV cross-reactive immunoglobulin G-secreting MBCs over (C) total DENV-specific antigen response and (D) total antibody-secreting cells (ASCs) at ~2 weeks (left panel) and 6–7 months (right panel) postinfection. The mean and standard deviation are shown for all graphs. Significance was determined by a Mann-Whitney test; *, P < .05, **, P < .01. At ~2 weeks postinfection, primary DENV, n = 10; secondary DENV, n = 13. At 6–7 months postinfection, n = 13 for both groups.
DISCUSSION
In this study, we present a comprehensive analysis of the polyclonal MBC response in PBMCs from 32 children after primary and secondary DENV infections from our long-standing hospital-based study in Nicaragua. We used the Multi-Color FluoroSpot assay to determine the MBC response to DENV1–4 and ZIKV at early and late convalescence. One major strength of the FluoroSpot assay is that it allows the characterization of MBC antibody specificity at a single-cell level rather than at the population level like the traditional enzyme-linked immunospot (ELISPOT) assay. We found that the anti-DENV polyclonal MBC response had strong type-specific and cross-reactive responses towards the infecting serotype even during secondary infection. Our results also indicated that anti-DENV MBCs were predominantly cross-reactive until at least 6–7 months postinfection in both primary and secondary DENV cases. Furthermore, we showed that the frequency and proportion of type-specific and cross-reactive MBC responses are similar between primary and secondary DENV cases, but that the breadth of MBC response is greater after secondary DENV infection. We noted that MBCs were substantially cross-reactive towards ZIKV, particularly after secondary DENV infection, despite the patients being ZIKV-naive. Our study sheds light on the polyclonal MBC response after DENV infection and the dynamics of immune memory after primary and secondary infection.
We found that the MBC response was higher towards the current infecting serotype, with more significant differences at 6–7 months postinfection. An important proportion of the response to the infecting serotype was due to type-specific responses in both primary and secondary DENV infection. In contrast, previous studies of acute-phase B-cell responses after DENV infection demonstrated that cross-reactive plasmablasts were more reactive towards the previous infecting serotype [30, 34, 35]. Passage through the germinal center and the process of affinity maturation may result in selection of MBCs with antibodies directed to the next (current) infecting serotype, which we noted was more pronounced in later convalescence. Memory B cells retained similar amounts of type-specific and cross-reactive responses during primary and secondary infections, but cross-reactivity was higher than type-specificity at early and late convalescence in both primary and secondary dengue. Another study of MBCs found that the serotype-specific response was higher in primary compared with secondary DENV infections and that the frequency of MBC responses was not maintained between early convalescent DENV infection and 6 months postinfection in a pediatric cohort study in Thailand [12]. This study used a traditional ELISPOT assay, which characterizes the MBC response at a population level rather than the single-cell level as in the Multi-Color FluoroSpot assay used here, and used recombinant envelope protein rather than virions. Most other studies characterizing the B-cell response during and after DENV infection examined either the polyclonal response in serum [36] or studied mAbs isolated from PBMCs [14, 15, 37, 38]. These studies revealed that the majority of human mAbs derived from primary and secondary DENV infection are cross-reactive, similar to our results obtained from MBCs [14, 15, 37, 38]. It is interesting to note that although we found that the DENV cross-reactive MBC response was maintained at 6–7 months post-DENV infection, our previous study revealed that the DENV/ZIKV cross-reactive MBC response decreased over time post-ZIKV infection [31]. Overall, DENV induces a robust MBC cross-reactive response after primary and secondary DENV infection that is maintained over time.
Our study revealed that the breadth of DENV cross-reactive MBCs (ie, recognition of 4 DENV serotypes) was greater after secondary DENV infection compared with primary infection. We also found that DENV infection induces an MBC response against ZIKV, despite all patients being ZIKV-naive before ZIKV introduction into the Americas. It is interesting to note that we found a significantly higher proportion of DENV/ZIKV cross-reactive MBCs in secondary DENV3 infections, similar to our previous report [31], possibly suggesting a unique shared epitope. Dengue virus/ZIKV cross-reactive MBCs were expanded after secondary DENV infection compared with primary infection, suggesting that the breadth of the MBC response increases with subsequent DENV infections. Furthermore, the DENV/ZIKV cross-reactive MBC response was maintained until late convalescence post-DENV infection. In contrast, we previously found that after ZIKV infection, the DENV/ZIKV cross-reactive MBC response declined by late convalescence [31]. Other studies also found that plasmablast-derived antibodies and sera from acute and convalescent secondary dengue patients were cross-reactive with ZIKV [39, 40]. The induction of DENV/ZIKV cross-reactive MBCs after DENV infection may be explained by the high degree of genomic and structural similarities between ZIKV and DENV [41, 42]. What the precise roles of these DENV/ZIKV cross-reactive antibodies are and how they impact disease and shape immunity to subsequent ZIKV infection is still unclear. However, recent studies demonstrated that prior DENV infection and anti-DENV antibodies are protective against symptomatic Zika [25, 43]. Thus far, prior DENV infection has not been shown to be a risk factor for severe Zika in humans, although the role of DENV/ZIKV cross-reactive antibodies still needs to be definitively assessed in prospective cohorts of pregnant women.
One limitation of our hospital-based study is that information is not available about the DENV preinfection history of our patients. To reconstruct the history of prior DENV exposure in secondary cases, we performed neutralization analysis of serum samples and confirmed, in the majority of cases, that prior heterotypic exposure was in accordance with the Multi-Color FluoroSpot results. Another limitation was that when we performed DENV neutralization assays on MBC supernatants, the antibody concentration in a number of samples was below the limit of detection of the assay. Therefore, we could not draw conclusions about relative antibody titers in cross-reactive responses and could only conclude that there were functional MBC-derived antibodies in our samples and that these antibodies neutralized predominantly the infecting serotype in primary DENV infections.
CONCLUSIONS
In summary, we provide a novel single-cell analysis of the polyclonal MBC response against DENV. We found that the MBC response to the currently infecting serotype is dominant in secondary infections and contains a strong type-specific response regardless of prior DENV exposure. We also showed that responses to prior heterotypic exposures are maintained in the type-specific pool of MBCs. We found that the overall proportion of type-specific versus cross-reactive MBC responses is comparable between primary and secondary DENV infection, with serotype cross-reactive responses being dominant over type-specific MBCs. However, we found that secondary DENV infections generate a broader response, recognizing more DENV serotypes and the related ZIKV flavivirus, than primary infections. This may have important implications for understanding the modulation of subsequent infection with ZIKV and other flaviviruses and how MBCs might evolve broader responses than LLPCs to protect against future infections with related viruses. Future studies of the polyclonal MBC response could help reveal the role of MBCs on outcome of DENV infection and disease and inform markers of protection for future vaccine studies.
Supplementary Data
Supplementary materials are available at The Journal of Infectious Diseases online. Consisting of data provided by the authors to benefit the reader, the posted materials are not copyedited and are the sole responsibility of the authors, so questions or comments should be addressed to the corresponding author.
Notes
Acknowledgments. We thank Dr. Michael Diamond (Washington University in St. Louis) for his donation of E95, E96, and E88 monoclonal antibodies (mAbs), Dr. Davide Corti (Humabs Biomed, subsidiary of Vir Biotechnology, Inc., Bellinzona) for the donation of ZKA64 mAb, Drs. Kim Dowd and Theodore Pierson (National Institutes of Health) for ZIKV Reporter Virus Particles, and Dr. Ralph Baric (University of North Carolina Chapel Hill) for his donation of recombinant 5J7 mAb and Aedes albopictus C6/36 cells. We also thank our study team at the Hospital Infantil Manuel de Jesús Rivera and the Laboratorio Nacional de Virología at the Centro Nacional de Diagnóstico y Referencia, Ministry of Health, as well as the Sustainable Sciences Institute, in Nicaragua for their high-quality work and dedication. We are also grateful to the study participants and their families.
Financial support. This work was funded by Grants P01AI106695 (to E. H.), U19AI118610 (to E. H.), and R01AI099631 (to A. B.) from the National Institute of Allergy and Infectious Diseases, National Institutes of Health.
Potential conflicts of interest. All authors: No reported conflicts of interest. All authors have submitted the ICMJE Form for Disclosure of Potential Conflicts of Interest.