-
PDF
- Split View
-
Views
-
Cite
Cite
Satoru Watanabe, Nicole Wei Wen Tan, Kitti Wing Ki Chan, Subhash G Vasudevan, Dengue Virus and Zika Virus Serological Cross-reactivity and Their Impact on Pathogenesis in Mice, The Journal of Infectious Diseases, Volume 219, Issue 2, 15 January 2019, Pages 223–233, https://doi.org/10.1093/infdis/jiy482
- Share Icon Share
Abstract
Preexisting immunity to Zika virus (ZIKV) or dengue virus (DENV) may alter the course of their infection, and here we use robust mouse models to examine pathological outcomes following passive immunization, sequential cross-infection, or vaccination with inactivated virus. DENV infection was enhanced (through antibody-dependent enhancement [ADE]) or was suppressed by both DENV and ZIKV immunity. Notably, inactivated ZIKV vaccination enhanced dengue disease severity, although it was highly protective against ZIKV infection. On the other hand, ADE was not observed upon ZIKV infection in mice that were passively immunized or preinfected with DENV. Surprisingly, however, we found that vaccination with inactivated DENV enhanced ZIKV infection, mainly in the mesenteric lymph node, indicating the potential for DENV immunity to cause ADE in vivo. Collectively, our data call for greater attention to detail in the design of ZIKV or DENV vaccines.
Since 2015, Zika virus (ZIKV) has spread rapidly into regions where other flaviviruses, such as dengue virus (DENV), are endemic. ZIKV and DENV are phylogenetically closely related and transmitted by Aedes aegypti mosquitoes. Although ZIKV infection is primarily associated with mild self-limiting illness, there is now compelling evidence of its association with severe neurological complications, such as microcephaly in infants and Guillain-Barré syndrome in adults [1], leading to urgent calls for the development of antivirals or vaccines against ZIKV.
DENV infection with any of the 4 related serotypes (DENV1–4) causes a broad spectrum of clinical manifestations ranging from self-limiting febrile illness (dengue fever) to the life-threatening severe diseases—dengue hemorrhagic fever or dengue shock syndrome [2–4]. Severe dengue diseases are usually associated with secondary heterotypic infections or primary infection in infants born to DENV-immune mothers. Preexisting cross-reactive antibodies (Abs) among 4 DENV serotypes are believed to enhance DENV infection through antibody-dependent enhancement (ADE) of infection [5–7]. Since the envelope (E) proteins of DENV and ZIKV share >50% sequence homology [8], there is a major concern that the more severe disease manifestations may result from prior cross-reactive immunity between DENV and ZIKV, either from natural infection or vaccination.
Several studies have examined the potential for ADE by serological cross-reaction between ZIKV and DENV (Supplementary Table 1). Briefly, mouse ZIKV-immune serum enhanced DENV infection in vitro [9]. Also, human monoclonal anti-E Abs isolated from ZIKV-infected donors enhanced DENV infection in vitro and exacerbated disease severity in mice [10]. Furthermore, the prior infection of nonhuman primates with ZIKV was found to increase the viremia level during DENV infection [11]. Collectively, these results suggest that there is potentially a considerable risk of ADE of DENV infection in patients with preexisting ZIKV immunity. Several studies have also shown that human DENV-immune serum/plasma or isolated cross-reactive monoclonal Abs can enhance ZIKV infection in vitro [12–14]. Additionally, human DENV-immune plasma was shown to lethally enhance ZIKV infection in mice [14]. However, opposing data that mouse DENV-immune serum did not cause ADE of ZIKV infection in mice [15] and that prior infection with DENV did not affect ZIKV pathogenesis in monkeys [16, 17] have been reported.
In this study, we investigated the impact of DENV/ZIKV serological cross-reactivity either on ZIKV or DENV infection in mice to understand the interplay between immunity and pathogenesis in vivo. We show that ADE of ZIKV infection is not evident in mice inoculated with DENV-immune serum or preinfected with DENV. However, DENV pathogenesis is readily influenced by ZIKV immunity, either through ADE or protective immune responses. Inactivated ZIKV vaccination was highly protective against ZIKV challenge, but it caused severe disease in DENV-infected mice. Notably, immunization with inactivated DENV can cause enhanced ZIKV infection. Together, our data call for a cautious approach to the design and development of safe ZIKV and/or DENV vaccines.
METHODS
Cells and Viruses
BHK-21 cells (baby hamster kidney fibroblast cells; ATCC), THP-1 (human monocytic cells; ATCC), and C6/36 (Aedes albopictus cells; ATCC) were cultured as described previously [18]. Mouse-adapted DENV2 strain S221 (DENV2-S) [19] was obtained from Dr Sujan Shresta (La Jolla, CA). ZIKV H/PF/2013 (French Polynesia; ZIKV-FP) and MR766 (Uganda; ZIKV-U) strains were obtained from the European Virus Archive. ZIKV Paraiba 01/2015 (Brazil; ZIKV-B) was a gift from the Evandro Chagas Institute. DENV1-2402 (DENV1-E; GenBank accession EU081230.1) and DENV2-3295 (DENV2-E; GenBank accession EU081177.1) were obtained from the Early Dengue Infection and Outcome (EDEN) study in Singapore [20]. All virus strains were grown in C6/36 cells, and the supernatants were stored at −80°C after filtration through a 0.45-μm membrane. Virus titer was determined by standard plaque assay on BHK-21 cells.
In Vitro Enhancement or Neutralization Cell-Based Assay
Testing of the enhancing or neutralizing activity of serum was conducted as described previously [18]. Briefly, DENV2-S (2 × 104 plaque-forming units [PFU]) or ZIKV-B (2 × 104 PFU) were mixed with serially diluted mouse or human DENV-immune or ZIKV-immune serum and infected in THP-1 or BHK-21 cells (1 × 105 cells). Virus titer in the supernatants of THP-1 cells (48 hours after infection) and BHK-21 cells (24 hours after infection) were measured by a standard plaque assay, using BHK-21 cells.
Ethics Statement
All animal experiments (protocol 2016/SHS/1197) were approved by the Institutional Animal Care and Use Committee at Singapore Health Services and conformed to the National Institutes of Health guidelines and public law. The human serum used in this study was collected in accordance with National University of Singapore Institutional Review Board approval B-12-227.
Mice
Sv/129 mice deficient in type I interferon (IFN) receptor (A129) and type I/II IFN receptors (AG129; B&K Universal; Grimston, UK) were housed in the biosafety level 2 animal facility at Duke–National University of Singapore. Mice aged 7–12 weeks were used for all experiments.
DENV or ZIKV Infection in the Presence of Immune Serum in Mice
To obtain mouse DENV2-immune serum, AG129 or A129 mice were nonlethally infected intraperitoneally with DENV2-E (1 × 107 PFU). For ZIKV-immune serum, A129 mice were nonlethally infected intraperitoneally with ZIKV-B (1 × 105 PFU). Blood samples were collected twice weekly from the facial vein 4–8 weeks after infection. Pooled DENV-immune or ZIKV-immune sera were heat inactivated at 56°C for 30 minutes. Human serum samples were obtained from 2 DENV-seropositive healthy volunteers and heat inactivated at 56°C for 30 minutes.
AG129 mice were injected intraperitoneally with mouse immune serum diluted with phosphate-buffered saline (PBS; total volume, 500 μL) or human serum diluted with PBS (total volume, 200 μL) 1 day before infection. The mice were inoculated intravenously with DENV2-S (2 × 104 PFU) or ZIKV-B (2 × 104 PFU).
DENV or ZIKV Sequential Infection in Mice
A129 mice were inoculated intraperitoneally with DENV2-E (1 × 107 PFU) or ZIKV-B (1 × 105 PFU). At 8 week after infection, DENV2-E–infected mice were challenged intravenously with ZIKV-FP (2 × 104 PFU), and ZIKV-B–infected mice were challenged intravenously with DENV2-S (2 × 107 PFU).
Immunization of Mice with UV-Inactivated Virus and Virus Infection
ZIKV-FP, ZIKV-B, DENV1-E, and DENV2-E strains were inactivated by exposure to long-wave UV light for 45 minutes on ice. Complete inactivation following UV treatment was confirmed by a standard plaque assay. Viral genome copy numbers of inactivated virus were measured by real-time reverse transcription–polymerase chain reaction (RT-PCR) analysis (described below), and the viruses were diluted in PBS to adjust the genome copy numbers to 5 × 1010 copies/mL. A total of 50 μL of inactivated virus or PBS were mixed with 50 μL of complete Freund’s adjuvant (CFA; InvivoGen) and injected intraperitoneally into AG129 mice. At weeks 2 and 5 following primary immunization, all mouse groups were boosted intraperitoneally with 50 μL of inactivated virus or PBS with 50 μL of incomplete Freund’s adjuvant (IFA; InvivoGen). At week 8 after primary immunization, the mice were challenged intravenously with DENV2-S (2 × 104 PFU) or ZIKV-B (2 × 104 PFU).
Quantification of Viral Genome Copies by Quantitative Real-Time RT-PCR
Serum viral RNA was extracted using QIAamp Viral RNA Mini Kit (Qiagen) according to the manufacturer’s instructions. Real-time RT-PCR was performed in Bio-Rad Real-time thermal cycler CFX96 by the use of qScript One-Step qRT-PCR kit (Quanta). Primers and TaqMan probes targeting for DENV1 or DENV2 were obtained from Center for Disease Control and Prevention, and published primers were used for ZIKV detection (Supplementary Table 2) [21]. In vitro–transcribed RNA products of sets of the primers were used to generate the standard curve for quantification of viral genome copy.
Measurement of Viral Load in Mouse Tissues
Tissue viral load was measured as described previously [18]. Briefly, mice were perfused with PBS after blood specimen collection, and tissue samples were collected and snap frozen in liquid nitrogen. Frozen tissues were homogenized by TissueLyser (Qiagen) with PBS, and the supernatants after centrifugation were used to measure tissue viral load. RNA was extracted with Trizol (Thermo-Fisher Scientific), and 80 ng of RNA was used to measure viral RNA levels by real-time PCR.
Statistical Analysis
Significant differences between data groups were determined by the 2-tailed Student t test. For mouse survival, statistical analysis was performed by the log-rank test, using GraphPad Prism software. A P value of <.05 was considered statistically significant.
RESULTS
Infectivity Testing of ZIKV Strains in IFN Receptor–Deficient Mice
First, to characterize ZIKV infection in mice, AG129 mice (lacking the IFN-α/β and IFN-γ receptors) and A129 mice (lacking the IFN-α/β receptor) were tested for their susceptibility to ZIKV strains ZIKV-FP, ZIKV-B, and ZIKV-U. Infection with ZIKV-FP (105 and 103 PFU) or ZIKV-B (106 and 104 PFU) caused 100% mortality by day 10 after infection in AG129 mice, accompanied with high levels of viremia that peaked on day 2 after infection, while ZIKV-U (105 and 103 PFU) induced delayed mortality with lower viremia levels (Figure 1A). ZIKV-FP infection was also lethal in A129 mice, whereas ZIKV-B or ZIKV-U did not kill A129 mice, although ZIKV-B could induce a high level of viremia comparable to that induced by ZIKV-FP (Figure 1B). Neurological disorders were commonly observed in mice lethally infected with ZIKV. Together, ZIKV-FP and ZIKV-B are highly infectious in both AG129 and A129 mice, compared with DENV, which has low infectivity in A129 mice [22, 23]. Our results are not completely consistent with previous reports that showed high infectivity in IFN-deficient mice by ZIKV-U [24–26]. This discrepancy may be due to different virus sources or to changes acquired during laboratory-based passage. Indeed, a recent report showed that passage-acquired mutations can affect the pathogenesis of ZIKV in mice [27]. Determining the susceptibilities of the AG129 and A129 mice to the ZIKV strains were important for scheduling subsequent experiments, and we used the same virus batches for all experiments in this study.
![Zika virus (ZIKV) infection in AG129 and A129 mice. AG129 (A) mice or A129 (B) mice were inoculated intraperitoneally with ZIKV H/PF/2013 (ZIKV-FP; 105 and 103 plaque-forming units [PFU]), Brazilian (ZIKV-B; 106 and 104 PFU), or MR766 (ZIKV-U; 105 and 103 PFU). The mouse survival rate was monitored through day 30 after infection. Blood samples were collected on days 1–6 after infection, and mixed serum specimens from each group were subjected to real-time reverse transcription–polymerase chain reaction analysis to obtain the average viral genome copy numbers. The number of mice per group was 5–6.](https://oup.silverchair-cdn.com/oup/backfile/Content_public/Journal/jid/219/2/10.1093_infdis_jiy482/1/m_jiy48201.jpeg?Expires=1749834846&Signature=pWRqO8OmmR9ZNro4ZpR6j9KYB7Ns08SMItUKItEWvvlJjkr~iRWNSlz2Yq4P3TVXEfuoNnusOyilOJ7jIlPbGlnjiJ7xxLYk8oYX1bzlwICGJQgLTWPAh38~TksT8kYjwSlDOXDMNNBmE9uEiU5W0VnEYW4FVAbiuiWwElVDwdgX3qPlGswozFA5RgXmVVhIelpILqW1u2Ipn6x-o6xP4xY3bJer95g5cWU1qhbL-TJc1IMsbdyVI0xbIYmPpPLPEIXHtq9~6uoTrm~m1aeURig0FfcIiCTh5fCwD3zIvIr9P6WZXm1K3~r8NoqVgT4d0SIYGvR5Xyf68WmoqXISdg__&Key-Pair-Id=APKAIE5G5CRDK6RD3PGA)
Zika virus (ZIKV) infection in AG129 and A129 mice. AG129 (A) mice or A129 (B) mice were inoculated intraperitoneally with ZIKV H/PF/2013 (ZIKV-FP; 105 and 103 plaque-forming units [PFU]), Brazilian (ZIKV-B; 106 and 104 PFU), or MR766 (ZIKV-U; 105 and 103 PFU). The mouse survival rate was monitored through day 30 after infection. Blood samples were collected on days 1–6 after infection, and mixed serum specimens from each group were subjected to real-time reverse transcription–polymerase chain reaction analysis to obtain the average viral genome copy numbers. The number of mice per group was 5–6.
DENV/ZIKV Infection Following Passive Transfer of ZIKV/DENV-Immune Serum in AG129 Mice
First, to investigate the impact of humoral cross-reactivity between DENV and ZIKV on DENV/ZIKV pathogenesis, we collected immune serum 4–8 weeks after infection from AG129 mice nonlethally infected with DENV2-E [28] or A129 mice infected with ZIKV-B. THP-1 cells were used to test the potential for ADE in vitro, and BHK-21 cells were used to score for the neutralization capacity [18]. DENV2-S infection was enhanced in the presence of DENV2-E–immune or ZIKV-B–immune serum in THP-1 cells (Figure 2A). Higher dilution was required for DENV2-E serum consistent with its high neutralizing activity against DENV2-S, while low dilutions of ZIKV-immune serum enhanced DENV2 infection reflecting the lack of neutralizing activity in BHK-21 (Figure 2A). Similarly, ZIKV-B was also found to be enhanced by DENV2-immune serum at lower serum dilutions and ZIKV-immune serum at higher dilutions (Figure 2B). We confirmed that the nonimmune mouse serum did not enhance DENV2-S or ZIKV-B infection at any dilutions (data not shown). Our data are consistent with previous studies [9, 14] and show that serological cross-reaction between DENV or ZIKV can readily induce ADE of infection in vitro.
![Effect of serological cross-reactivity on dengue virus (DENV) or Zika virus (ZIKV) infection in vitro and in vivo. A and B, DENV-immune serum (DV serum) was collected from AG129 mice nonlethally infected with DENV2 (strain DENV2-E; 1 × 107 plaque-forming units [PFU]). ZIKV-immune serum (ZK serum) was collected from A129 mice nonlethally infected with ZIKV-B (1 × 105 PFU). DENV2 (strain S221 [DENV2-S]; 2 × 104 PFU; A) or ZIKV-B (2 × 104 PFU; B) were mixed with serially diluted (4-400 000-fold) mouse DENV-immune or ZIKV-immune serum and infected in THP-1 or BHK-21 cells (1 × 105 cells). Virus titer in the supernatants of THP-1 cells (48 hours after infection) and BHK-21 cells (24 hours after infection) were measured by a standard plaque assay, using BHK-21 cells. Error bars indicate standard deviations from duplicate samples. C and D, AG129 mice were injected intraperitoneally with 0 μL (virus control [VC]), 5 μL, 50 μL, or 500 μL of DENV-immune or ZIKV-immune serum 1 day before infection and inoculated intravenously with 2 × 104 PFU of DENV2-S (C) or ZIKV-B (D). The mouse survival rate was monitored through day 10 after infection for DENV2 infection or through day 25 after infection for ZIKV infection. Blood samples were collected until day 8 after infection, and mixed serum specimens from each group were subjected to real-time reverse transcription–polymerase chain reaction analysis to obtain the average viral genome copy numbers. The number of mice per group was 6.](https://oup.silverchair-cdn.com/oup/backfile/Content_public/Journal/jid/219/2/10.1093_infdis_jiy482/1/m_jiy48202.jpeg?Expires=1749834846&Signature=FPgXpQ1u-RDXYLl1EuoWIujJuVfT-LmcyiibuQ2loBMgtCqN8AKN-c1co27XSBz85cmqajY42LkWZ39rBgY17sy2Or9sIsn68EgV1mwFtQcDlU4c7IWmmWrcqDn21W6XEeqf2hg-TstplQoPLVDcvcIZyqs5fd9jYuTimsJSZidWZdLH75baUkrRvkm3v3L8fSVYdzsnjBiu2Ib0vIBG94ePvtxwOs-eodIyiPUePiHyAH2C9iBeyZ~sH6hxMhIefKn7vSSFiSxa0W3zCAdzaobjy74OW3nVXRZ8I0x2W8M0BMJ6e59d8bAb925O2h-JaNbU9rG1zHl712wKHP1VCg__&Key-Pair-Id=APKAIE5G5CRDK6RD3PGA)
Effect of serological cross-reactivity on dengue virus (DENV) or Zika virus (ZIKV) infection in vitro and in vivo. A and B, DENV-immune serum (DV serum) was collected from AG129 mice nonlethally infected with DENV2 (strain DENV2-E; 1 × 107 plaque-forming units [PFU]). ZIKV-immune serum (ZK serum) was collected from A129 mice nonlethally infected with ZIKV-B (1 × 105 PFU). DENV2 (strain S221 [DENV2-S]; 2 × 104 PFU; A) or ZIKV-B (2 × 104 PFU; B) were mixed with serially diluted (4-400 000-fold) mouse DENV-immune or ZIKV-immune serum and infected in THP-1 or BHK-21 cells (1 × 105 cells). Virus titer in the supernatants of THP-1 cells (48 hours after infection) and BHK-21 cells (24 hours after infection) were measured by a standard plaque assay, using BHK-21 cells. Error bars indicate standard deviations from duplicate samples. C and D, AG129 mice were injected intraperitoneally with 0 μL (virus control [VC]), 5 μL, 50 μL, or 500 μL of DENV-immune or ZIKV-immune serum 1 day before infection and inoculated intravenously with 2 × 104 PFU of DENV2-S (C) or ZIKV-B (D). The mouse survival rate was monitored through day 10 after infection for DENV2 infection or through day 25 after infection for ZIKV infection. Blood samples were collected until day 8 after infection, and mixed serum specimens from each group were subjected to real-time reverse transcription–polymerase chain reaction analysis to obtain the average viral genome copy numbers. The number of mice per group was 6.
Since ADE of DENV infection in AG129 mice has been thoroughly characterized [18, 23, 29, 30], we used this model for in vivo studies, where possible. AG129 mice were passively inoculated with 0 μL (virus control [VC]) or 5–500 μL of DENV2-E–immune or ZIKV-B–immune serum, followed by infection with DENV2-S or ZIKV-B. DENV2-S infection without serum transfer did not cause lethal infection, while mice that received 5 μL of DENV2-immune serum died of infection accompanied by enhanced peak viremia levels (Figure 2C). Mice receiving 50 μL of DENV2-immune serum also died of DENV2-S infection despite a lower viremia level than those infected with VC (Figure 2C). This phenomenon may be explained by our previous studies, which showed that tissue-dependent ADE can be induced even when the viremia level is suppressed and that increased viral loads and inflammatory cytokine levels in the small intestine correlated with disease severity in the mice [18, 31]. Inoculation with 500 μL of DENV2-immune serum was protective, with remarkable viremia suppression, suggesting that neutralizing activity overcomes ADE at this dose (Figure 2C). Notably, ZIKV-immune serum promoted the lethality of DENV2-S infection, with 100% mortality for high doses (50–500 μL) and 50% mortality for low doses (5 μL; Figure 2C), demonstrating the potential for ZIKV seroimmunity to cause ADE of DENV infection in vivo.
In contrast to DENV infection, inoculation of ZIKV-immune serum prolonged mouse survival (5 μL and 50 μL) or completely protected mice from mortality (500 μL) and was accompanied by remarkable viremia suppression when mice were infected with ZIKV-B (Figure 2D). Interestingly, however, inoculation with 5–500 μL of DENV2-immune serum did not alter the kinetics of the viremia level or the severity of disease progression, as measured by 100% lethality by day 10 after infection with ZIKV-B (Figure 2D). We confirmed similar findings among mice inoculated with serum collected from A129 mice infected with DENV2-E (Supplementary Figure 1).
Next, we tested the potential for ADE of ZIKV infection following inoculation of human serum collected from 2 healthy DENV-seropositive donors, who were suggested to have experienced DENV4 infection (serum 1) or DENV1 infection (serum 2), to evaluate a previous study, which reported that passive transfer of 2–50 μL of pooled convalescent-phase plasma from DENV-infected human donors enhanced ZIKV infection and promoted disease severity in Stat2 KO mice [14]. Human DENV-immune serum enhanced ZIKV infection at low dilutions in THP-1 cells (Figure 3A), similar to mouse DENV-immune serum (Figure 2B). However, no significant change in the survival rate or enhancement of the viremia level were observed in mice that received 20 μL or 200 μL of human DENV-immune serum (Figure 3B). Collectively, these results indicate that ADE of DENV infection is readily induced in mice by either DENV or ZIKV humoral immunity but that ZIKV is not similarly induced.
![Test of human dengue virus (DENV)–seropositive serum for enhancement or neutralization of Zika virus (ZIKV) infection in vitro and in vivo. A, Serum specimens collected from 2 DENV-seropositive donors (DV serum 1 and 2) were serially diluted with culture medium and mixed with ZIKV (Brazil; 2 × 104 plaque-forming units [PFU]) followed by infection in THP-1 or BHK-21 cells (1 × 105 cells). Virus titers in the supernatants of THP-1 cells (48 hours after infection) and BHK-21 cells (24 hours after infection) were measured by a standard plaque assay, using BHK-21 cells. DENV-seronegative control serum served as a negative control (NC). Error bars indicate standard deviations from duplicate samples. B, AG129 mice were injected intraperitoneally with 0 μL (virus control [VC]), 20 μL, or 200 μL of human DENV-immune serum 1 day before infection and inoculated intravenously with 2 × 104 PFU of ZIKV-B. The mouse survival rate was monitored until mice died of ZIKV infection. Blood samples were collected until day 8 after infection, and mixed serum specimens from each group were subjected to real-time reverse transcription–polymerase chain reaction analysis to obtain the average viral genome copy numbers. The number of mice per group was 6.](https://oup.silverchair-cdn.com/oup/backfile/Content_public/Journal/jid/219/2/10.1093_infdis_jiy482/1/m_jiy48203.jpeg?Expires=1749834846&Signature=3wH6WKKDYwI1gm0H05vfLHDNZo5WRTc6kPxthoIWYgmjLq9CNCHB1uNEWPFd2ELn9TqpGwuMNDRlKetUwIMsV1VD6o5TYYVCI5~n95sFb56WaOPjDgpgD0UmGK~23oSi1a0k7uWyaZmR3mhLHe4HCO7c5hKJUaMDoRe0cgIGbfocMEDSAzyhXfFt~QzglenIaCiNzzdqa7RS0Msq3Ct4NMR89J301kcyB60nm25UCoHp3fV~npxWvlEMPnrpptwm1oMhc~fsyGgxuAcYCBu7QsO~gcCFyUIeZo7iDA15mTMN8HSBNVaz3xPN18Z2Q8zI0Yz5CAjfVz90I-tk0wCWMw__&Key-Pair-Id=APKAIE5G5CRDK6RD3PGA)
Test of human dengue virus (DENV)–seropositive serum for enhancement or neutralization of Zika virus (ZIKV) infection in vitro and in vivo. A, Serum specimens collected from 2 DENV-seropositive donors (DV serum 1 and 2) were serially diluted with culture medium and mixed with ZIKV (Brazil; 2 × 104 plaque-forming units [PFU]) followed by infection in THP-1 or BHK-21 cells (1 × 105 cells). Virus titers in the supernatants of THP-1 cells (48 hours after infection) and BHK-21 cells (24 hours after infection) were measured by a standard plaque assay, using BHK-21 cells. DENV-seronegative control serum served as a negative control (NC). Error bars indicate standard deviations from duplicate samples. B, AG129 mice were injected intraperitoneally with 0 μL (virus control [VC]), 20 μL, or 200 μL of human DENV-immune serum 1 day before infection and inoculated intravenously with 2 × 104 PFU of ZIKV-B. The mouse survival rate was monitored until mice died of ZIKV infection. Blood samples were collected until day 8 after infection, and mixed serum specimens from each group were subjected to real-time reverse transcription–polymerase chain reaction analysis to obtain the average viral genome copy numbers. The number of mice per group was 6.
Sequential Cross-Infection Between DENV and ZIKV in Mice Showed Different Levels of Impact on DENV and ZIKV Pathogenesis
The risk arising from sequential infection involving DENV and ZIKV is a critical concern, especially in the regions where both viruses are endemic. Therefore, we next examined the influence of DENV or ZIKV preinfection on the pathogenesis of subsequent DENV/ZIKV infection. Since ZIKV strains are lethal in AG129 mice (Figure 1A), A129 mice infected with ZIKV-B were used for challenge with DENV2 8 weeks after infection. Inoculation with a high titer of DENV2-S (2 × 107 PFU) was sublethal (60% mortality) in naive A129 mice, whereas mice preinfected with ZIKV-B did not die of DENV2-S infection (Figure 4A) and displayed a remarkable reduction in the viremia level (by 128-, 382-, and 364-fold on days 1, 2, and 3, respectively; Figure 4A). For consistency, A129 mice were also used for the sequential infection of ZIKV at 8 weeks after DENV2-E infection. All naive mice inoculated with ZIKV-FP succumbed to infection, while mice preinfected with DENV2-E were protected from subsequent ZIKV infection (Figure 4B). However, viremia levels decreased by only 2.0, 2.8, 3.2, 48.5, 68.6, and 22.0-fold on days 1, 2, 3, 4, 6, and 8, respectively (Figure 4B). Taken together, DENV immunity has less impact on ZIKV pathogenesis than the influence of ZIKV immunity on DENV pathogenesis in mice.
![Sequential dengue virus (DENV) or Zika virus (ZIKV) infection in DENV/ZIKV-infected mice. A, A129 mice were nonlethally infected intraperitoneally with ZIKV-B (1 × 105 plaque-forming units [PFU]) and challenged intravenously with a sublethal dose of DENV2-S (2 × 107 PFU) 8 weeks after infection. B, A129 mice were nonlethally infected intraperitoneally with DENV2-E (1 × 107 PFU) and challenged intravenously with a lethal dose of ZIKV-FP (2 × 104 PFU) 8 weeks after infection. The mouse survival rate was monitored through day 10 after infection for DENV2 infection or through day 20 after infection for ZIKV infection. Blood samples were collected through day 8 after infection, and mixed serum specimens from each group were subjected to real-time reverse transcription–polymerase chain reaction analysis to obtain the average viral genome copy numbers. The number of mice per group was 5.](https://oup.silverchair-cdn.com/oup/backfile/Content_public/Journal/jid/219/2/10.1093_infdis_jiy482/1/m_jiy48204.jpeg?Expires=1749834846&Signature=0oagU58F0MX9jX2GE8~iLa7uzrig7omd12ktPJRZRu1dvRzAC7gEzxQiuQwx9tdmiuXQzeudcUhHQCb692obqLU8wTo3-uQ9kunXSEW9qC6hlt2qDcib4lEchoqP856dpgoz4-am5-a3FusDLzuhllCpRD5pw7~9SZlKZ9lqfGrS~65I6uJ4YSuUn9Xr9qkYYIUvWYjoDn6dI7EiklWQtxfKxsGPe0Fim~YhJ7XZCLN7KpwSxTiCRWmF4TwYj~AS2UEWuMRpKQAN-Xb6UwmzMx9-rk73OE7eggPYhUQZj2h4sAhS7iXTe4wumGxDFYgluIXQmmORK-VqYR1bjhVnCA__&Key-Pair-Id=APKAIE5G5CRDK6RD3PGA)
Sequential dengue virus (DENV) or Zika virus (ZIKV) infection in DENV/ZIKV-infected mice. A, A129 mice were nonlethally infected intraperitoneally with ZIKV-B (1 × 105 plaque-forming units [PFU]) and challenged intravenously with a sublethal dose of DENV2-S (2 × 107 PFU) 8 weeks after infection. B, A129 mice were nonlethally infected intraperitoneally with DENV2-E (1 × 107 PFU) and challenged intravenously with a lethal dose of ZIKV-FP (2 × 104 PFU) 8 weeks after infection. The mouse survival rate was monitored through day 10 after infection for DENV2 infection or through day 20 after infection for ZIKV infection. Blood samples were collected through day 8 after infection, and mixed serum specimens from each group were subjected to real-time reverse transcription–polymerase chain reaction analysis to obtain the average viral genome copy numbers. The number of mice per group was 5.
Vaccination With Inactivated DENV or ZIKV Influenced Not Only DENV Infection but Also ZIKV Infection in Mice
Vaccination with purified inactivated virus, which can elicit immunity mainly against E and (pr)M proteins, is an approach that is being examined as a potential Zika vaccine strategy [24, 32, 33], and one candidate is being investigated in a phase I clinical study [34]. In addition, tetravalent dengue inactivated vaccine was also examined recently in phase 1 clinical studies [35, 36]. In this background, we examined the impact of inactivated viral vaccination on DENV or ZIKV pathogenesis in AG129 mice. Mice were immunized with UV-inactivated ZIKV-FP, ZIKV-B, DENV1-E, or DENV2-E; administered 2 times booster inoculations 2 and 5 weeks after immunization; and challenged with DENV2-S or ZIKV-B at 8 weeks (Figure 5A). Notably, both ZIKV and DENV vaccinations promoted lethality of DENV2 infection, with 67%, 50%, 100%, and 67% mortality for ZIKV-FP, ZIKV-B, DENV1-E, and DENV2-E vaccine recipients, respectively (Figure 5B). Lower levels of viremia were observed in the immunized mice, compared with those that received VC (Figure 5B), suggesting that the immunized mice had acquired immune responses against DENV2 but that the neutralizing activity could not overcome ADE-induced disease progression. In contrast, inactivated ZIKV immunizations successfully prolonged mouse survival, accompanied by a remarkable reduction in the viremia level, when the mice were challenged with ZIKV-B (Figure 5C). This is consistent with the previous study that showed that inactivated ZIKV vaccination was protective against homotypic and heterotypic ZIKV challenge in AG129 mice [24]. Notably, when ZIKV was challenged in DENV-immunized mice, the viremia level was found to be increased on days 3 and 4 after infection (Figure 5C), with a 7.1-fold higher level in mice vaccinated with DENV1 (P = .004) and a 7.7-fold higher level in those vaccinated with DENV2 (P = .003) on day 3 after infection (Figure 5D), although the mouse survival rate was not significantly affected (Figure 5C). Thus, ZIKV infection may be potentially enhanced by DENV immunity under defined conditions in vivo.
![Effect of vaccination with inactivated dengue virus (DENV) or Zika virus (ZIKV) on DENV/ZIKV pathogenesis in mice. A, ZIKV-FP, ZIKV-B, DENV1 (strain EDEN1), and DENV2-E were UV inactivated and inoculated intraperitoneally into AG129 mice with adjuvant. After boosting 2 times, mice were challenged intravenously with DENV2-S (2 × 104 plaque-forming units [PFU]) or ZIKV-B (2 × 104 PFU). Virus-infected control mice (VC) received phosphate-buffered saline (PBS) with adjuvant. B and C, The mouse survival rate was monitored through day 10 after infection for DENV2 infection or through day 30 after infection for ZIKV infection. Blood samples were collected until day 8 after infection, and mixed serum specimens from each group were subjected to real-time reverse transcription–polymerase chain reaction analysis to obtain the average viral genome copy numbers. The number of mice per group was 6. D, The viral copy number in serum of ZIKV-infected mice on day 3 after infection was measured individually. The graphs show the average results with standard deviations. CFA, complete Freund’s adjuvant; IFA, incomplete Freund’s adjuvant; ip, intraperitoneally; iv, intravenously. *P < .05.](https://oup.silverchair-cdn.com/oup/backfile/Content_public/Journal/jid/219/2/10.1093_infdis_jiy482/1/m_jiy48205.jpeg?Expires=1749834846&Signature=1pDUao14AI4XliFD37bU2x-UCK-h7Y4EdzN5M4cDIrx2gbTqEbH2xhWPaJsm9N7AXXUj5a18QcGo~n~CEwpuAvEycJBdGXNqFCjlaGT-V75mlQUEVhav7mi-0Y1JJVpl4l9X4DlHbEgAFH2royDXb22Ahs48dMNBH6E7cY~pA8PWlEjTp~5LUM1uNxjV-l1-upCZPv2I9L-C3siwPH0EwIiEL2J8bEXsF3-c1lCyeGNI~cAhY5jX0K9T287H7wYe8AijkYTlOteRJ-zatfN-BBFbJb1EkkUWuObaiWb6OWr5kEsV~1vCLJGWFyKYKofChr57mOl8KXddA0yFhwbS9w__&Key-Pair-Id=APKAIE5G5CRDK6RD3PGA)
Effect of vaccination with inactivated dengue virus (DENV) or Zika virus (ZIKV) on DENV/ZIKV pathogenesis in mice. A, ZIKV-FP, ZIKV-B, DENV1 (strain EDEN1), and DENV2-E were UV inactivated and inoculated intraperitoneally into AG129 mice with adjuvant. After boosting 2 times, mice were challenged intravenously with DENV2-S (2 × 104 plaque-forming units [PFU]) or ZIKV-B (2 × 104 PFU). Virus-infected control mice (VC) received phosphate-buffered saline (PBS) with adjuvant. B and C, The mouse survival rate was monitored through day 10 after infection for DENV2 infection or through day 30 after infection for ZIKV infection. Blood samples were collected until day 8 after infection, and mixed serum specimens from each group were subjected to real-time reverse transcription–polymerase chain reaction analysis to obtain the average viral genome copy numbers. The number of mice per group was 6. D, The viral copy number in serum of ZIKV-infected mice on day 3 after infection was measured individually. The graphs show the average results with standard deviations. CFA, complete Freund’s adjuvant; IFA, incomplete Freund’s adjuvant; ip, intraperitoneally; iv, intravenously. *P < .05.
To investigate the detailed pathogenic events under ADE of ZIKV infection, tissue specimens were harvested from mice that received inactivated DENV2 or VC on days 2, 3, and 6 after infection. A significant increase in viral load was detected in liver (1.9-fold; P = .01), small intestine (2.6-fold; P = .03), and mesenteric lymph node (LN; 7.2-fold; P = .008) specimens on day 2 after infection (Figure 6A) and in liver (2.4-fold; P = .03) and mesenteric LN (9.6-fold; P = .0006) specimens on day 3 after infection (Figure 6B). Specimens of other tissues, such as spleen, kidney, uterus, brain, large intestine, and eye, did not show significant increases in virus levels at any time points (Figure 6A–C). This suggests that the mesenteric LN is one of the main target sites for ADE of ZIKV infection in the presence of DENV immunity in these mice.
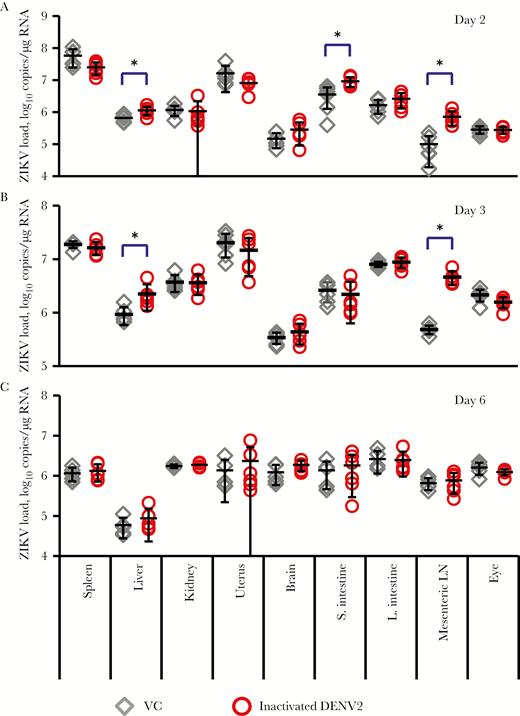
Comparison of tissue viral load of Zika virus (ZIKV) infection between control and inactivated dengue virus type 2 (DENV2)–immunized mice. AG129 mice were immunized with UV-inactivated DENV2-E and challenged with ZIKV as described in Figure 5. Mice were euthanized on days 2, 3, or 6 after infection, and spleen, liver, kidney, uterus, brain, small intestine (S. intestine), large intestine (L. intestine), mesenteric lymph node (LN), and eye specimens were collected to measure viral load. The average ZIKV loads (±SD) in various tissues on days 2 (A), 3 (B), and 6 (C) after infection were plotted. The number of mice per group was 6. VC, virus-infected control mice. *P < .05.
DISCUSSION
The close phylogenetic relationship between DENV and ZIKV raised the concern for ADE of infection through serological cross-reactivity. Indeed, it has been widely reported that their cross-reactivity can enhance DENV/ZIKV infection in FcγR-bearing cells [9, 10, 12–14]. Similarly, we show that DENV infection and ZIKV infection are enhanced by DENV-immune or ZIKV-immune serum in vitro. However, our in vivo results clearly show that DENV and ZIKV immunity can have distinct roles in viral pathogenesis as summarized in Table 1.
Effect of Serological Cross-reactivity Between Dengue Virus Type 2 (DENV2) Infection and Zika Virus (ZIKV) on DENV/ZIKV Infection in Mice
Variable . | DENV2 Infection . | ZIKV Infection . |
---|---|---|
Mouse ZIKV-immune serum (5–500 μL) | Enhancement of infection/disease severity | Protective (high level of viremia reduction) |
Mouse DENV2-immune serum (5–500 μL) | Enhancement or suppression of infection/disease severity | No significant effect |
Human DENV-immune serum (20–200 μL) | … | No significant effect |
Prior ZIKV infection | Protective (high level of viremia reduction) | … |
Prior DENV infection | … | Protective (mild level of viremia reduction) |
Inactivated ZIKV vaccination | Enhancement of disease severity | Protective (high level of viremia reduction) |
Inactivated DENV vaccination | Enhancement of disease severity | Enhancement of infection (viremia and mesenteric lymph nodes) |
Variable . | DENV2 Infection . | ZIKV Infection . |
---|---|---|
Mouse ZIKV-immune serum (5–500 μL) | Enhancement of infection/disease severity | Protective (high level of viremia reduction) |
Mouse DENV2-immune serum (5–500 μL) | Enhancement or suppression of infection/disease severity | No significant effect |
Human DENV-immune serum (20–200 μL) | … | No significant effect |
Prior ZIKV infection | Protective (high level of viremia reduction) | … |
Prior DENV infection | … | Protective (mild level of viremia reduction) |
Inactivated ZIKV vaccination | Enhancement of disease severity | Protective (high level of viremia reduction) |
Inactivated DENV vaccination | Enhancement of disease severity | Enhancement of infection (viremia and mesenteric lymph nodes) |
Effect of Serological Cross-reactivity Between Dengue Virus Type 2 (DENV2) Infection and Zika Virus (ZIKV) on DENV/ZIKV Infection in Mice
Variable . | DENV2 Infection . | ZIKV Infection . |
---|---|---|
Mouse ZIKV-immune serum (5–500 μL) | Enhancement of infection/disease severity | Protective (high level of viremia reduction) |
Mouse DENV2-immune serum (5–500 μL) | Enhancement or suppression of infection/disease severity | No significant effect |
Human DENV-immune serum (20–200 μL) | … | No significant effect |
Prior ZIKV infection | Protective (high level of viremia reduction) | … |
Prior DENV infection | … | Protective (mild level of viremia reduction) |
Inactivated ZIKV vaccination | Enhancement of disease severity | Protective (high level of viremia reduction) |
Inactivated DENV vaccination | Enhancement of disease severity | Enhancement of infection (viremia and mesenteric lymph nodes) |
Variable . | DENV2 Infection . | ZIKV Infection . |
---|---|---|
Mouse ZIKV-immune serum (5–500 μL) | Enhancement of infection/disease severity | Protective (high level of viremia reduction) |
Mouse DENV2-immune serum (5–500 μL) | Enhancement or suppression of infection/disease severity | No significant effect |
Human DENV-immune serum (20–200 μL) | … | No significant effect |
Prior ZIKV infection | Protective (high level of viremia reduction) | … |
Prior DENV infection | … | Protective (mild level of viremia reduction) |
Inactivated ZIKV vaccination | Enhancement of disease severity | Protective (high level of viremia reduction) |
Inactivated DENV vaccination | Enhancement of disease severity | Enhancement of infection (viremia and mesenteric lymph nodes) |
Absence of ADE of ZIKV infection following passive transfer of mouse DENV-immune serum in this study is consistent with the previous report that used Ifnar1−/− mice [15] but contradicted the observation in Stat2−/− mice that show enhancement of viremia and tissue viral load in the presence of convalescent plasma from DENV-infected patients [14]. Notably, human DENV-positive serum specimens also failed to induce ADE of ZIKV infection in AG129 mice. This contradiction may arise from the differences of human samples or mouse strains used in the experiments. Nevertheless, immunization with inactivated DENV enhanced ZIKV infection in our study, suggesting that induction of ADE of ZIKV infection may require defined preconditions in vivo. At the tissue level, the mesenteric LN seems to be the major target organ for ADE of ZIKV infection that may be responsible for the observed enhancement of viremia. Whether the enhancement of viremia increases the risk of maternal-fetal ZIKV transmission or other neurological disorders should be addressed in future studies.
Interestingly, sequential DENV infection in mice with prior ZIKV infection did not result in mortality with a 2-log reduction in the viremia level, suggesting the potential of ZIKV immunity to prevent the progression toward a severe form of dengue. However, an acknowledged limitation is that, unlike human sequential heterotypic DENV infection, which is often associated with ADE, no mouse model so far can reproduce an enhanced viral load or mortality by a sequential heterotypic DENV infection [37], in part because of the cross-reactive T-cell responses [38, 39]. Therefore, complete protection from mortality observed in mice subjected to ZIKV-DENV sequential infection does not indicate no risk for exacerbation of dengue disease in humans. Indeed, in a recent report, ZIKV-DENV sequential infection resulted in increased viremia levels in Rhesus monkeys [11]. On the other hand, sequential DENV-ZIKV infection of mice did not show a remarkable reduction in viremia levels, although it was also protective. Recent studies also showed that prior DENV infection has minimal effect on ZIKV pathogenesis in Rhesus monkeys [16, 17]. The complete protection from ZIKV challenge in mice preinfected with DENV may be accounted for by findings in a recent report, which suggested that cross-reactive CD8+ T-cell immunity, rather than humoral immunity, confers protection against subsequent ZIKV infection in mice [15].
Currently, ZIKV vaccine candidates are being actively developed, and their efficacies are being evaluated by ZIKV challenge in animal models [34]. Several of them, including an inactivated ZIKV vaccine candidate, are under evaluation in early phase clinical trials [34]. However, a specific concern is that these vaccine candidates have not been robustly tested for the risk of ADE of DENV infection preclinically in animal models. Importantly, our results show that inactivated ZIKV vaccination is indeed highly effective against ZIKV challenge but can promote disease progression in mice challenged with DENV. Additionally, inactivated DENV vaccine enhanced dengue disease severity in mice, and notably, it could also enhance ZIKV infection. A previous study reported that no measurable CD8+ T-cell response was generated by inactivated DENV2 vaccination in AG129 mice, resulting in an increase in mortality by following DENV2 challenge [40], as observed in our study. Therefore, the immune condition that involves memory B-cell activity, which can continuously produce Abs, but not active T-cell immunity may enable to induce the enhancement of ZIKV infection in inactivated DENV-vaccinated mice.
In conclusion, both DENV and ZIKV infection can potentially be enhanced by preexisting serological cross-reactivity in vivo. Therefore, careful attention is needed for the design and development of safe ZIKV or DENV vaccines [41].
Supplementary Data
Supplementary materials are available at The Journal of Infectious Diseases online. Consisting of data provided by the authors to benefit the reader, the posted materials are not copyedited and are the sole responsibility of the authors, so questions or comments should be addressed to the corresponding author.
Notes
Potential conflicts of interest. All authors: No reported conflicts of interest. All authors have submitted the ICMJE Form for Disclosure of Potential Conflicts of Interest. Conflicts that the editors consider relevant to the content of the manuscript have been disclosed.
Financial support. This work was supported by the National Medical Research Council (Open Fund Young Individual Research Grant 0003/2016 to S. W. and Cooperative Basic Research Grant/0103/2016 to S. G. V.).