-
PDF
- Split View
-
Views
-
Cite
Cite
Arnaud G L’huillier, Victor H Ferreira, Cedric Hirzel, Yoichiro Natori, Jaclyn Slomovic, Terrance Ku, Katja Hoschler, Matthew Ierullo, Nazia Selzner, Jeffrey Schiff, Lianne G Singer, Atul Humar, Deepali Kumar, Cell-Mediated Immune Responses After Influenza Vaccination of Solid Organ Transplant Recipients: Secondary Outcomes Analyses of a Randomized Controlled Trial, The Journal of Infectious Diseases, Volume 221, Issue 1, 1 January 2020, Pages 53–62, https://doi.org/10.1093/infdis/jiz471
- Share Icon Share
Abstract
Despite annual immunization, solid organ transplant (SOT) patients remain at increased risk for severe influenza infection because of suboptimal vaccine immunogenicity. We aimed to compare the CD4+ and CD8+ T-cell responses of the high-dose (HD) and the standard-dose (SD) trivalent inactivated vaccine.
We collected peripheral blood mononuclear cells pre- and postimmunization from 60 patients enrolled in a randomized trial of HD versus SD vaccine (30 HD; 30 SD) during the 2016–2017 influenza season.
The HD vaccine elicited significantly greater monofunctional and polyfunctional CD4+ and CD8+ T-cell responses against influenza A/H1N1, A/H3N2, and B. For example, median vaccine-elicited influenza-specific polyfunctional CD4+ T cells were higher in recipients of the HD than SD vaccine after stimulation with influenza A/H1N1 (1193 vs 0 per 106 CD4+ T cells; P = .003), A/H3N2 (1154 vs 51; P = .008), and B (1102 vs 0; P = .001). Likewise, vaccine-elicited influenza-specific polyfunctional CD8+ T cells were higher in recipients of the HD than SD vaccine after stimulation with influenza B (367 vs 0; P = .002).
Our study provides novel evidence that HD vaccine elicits greater cellular responses compared with the SD vaccine in SOT recipients, which provides support to preferentially consider use of HD vaccination in the SOT setting.
Solid organ transplant (SOT) patients are at increased risk for infections, including influenza infection, which is responsible for significant morbidity and mortality [1, 2]. Influenza vaccine is recommended for all transplant recipients [3]. Despite yearly immunization, a significant proportion of transplant recipients develop influenza [1], which is likely related to the discordance between circulating influenza strains and those included in annual vaccine formulations, as well as suboptimal immunogenicity of vaccines in this population [4–6]. Despite suboptimal vaccine immunogenicity, previously immunized transplant recipients who develop influenza have been shown to have lower viral loads and reduced severity of disease compared with those who were not immunized [1].
To improve immunogenicity in SOT patients, several approaches have been evaluated such as changing the method of delivery or using adjuvants or booster doses, but none have shown clinically significant improvement in humoral immunogenicity [4, 6–8]. In healthy adults ≥65 years old who are known to be poor vaccine responders, the use of a high-dose (HD) influenza vaccine containing 60 µg of hemagglutinin (HA) per strain is recommended [9], because it has greater efficacy and compared with the standard-dose (SD) vaccine, which contains 15 µg of HA per strain [10–13]. In a recent study, a randomized controlled trial performed by our group comparing HD with SD influenza vaccine showed that the HD vaccine significantly increased seroconversion (SC) rates and resulted in significantly greater geometric mean titers (GMTs) in SOT patients compared with the SD vaccine [14]. Although humoral immunity is the most frequently studied aspect of the immune system after influenza vaccination, data suggest that T cell-mediated immunity (CMI) may be a better correlate of protection in patients with poor immune responses [15, 16]. There are no prior studies in transplantation that have studied cell-mediated immune responses between SD and HD influenza vaccines. Therefore, the aim of this study was to compare the CD4+ and CD8+ influenza-specific T-cell responses between patients who received the HD and the SD influenza vaccine enrolled in the previously conducted randomized trial.
METHODS
Patient Population and Study Design
A subset of patients enrolled in the HD versus SD influenza vaccine randomized controlled trial agreed to provide additional blood samples for the CMI study [14]. In brief, adult organ transplant recipients (kidney, liver, heart, lung, pancreas, or multiorgan), who were stable outpatients and at least 3 months posttransplant with a functioning graft, were enrolled between October 2016 and January 2017. Patients were randomized 1:1 to receive either HD vaccine containing 60 µg of antigen per strain or SD vaccine containing 15 µg of antigen per strain. Both the HD (FluzoneHD; Sanofi, Laval, Canada) and SD (Fluviral; GSK, Mississauga, Canada) vaccines were trivalent and contained the following influenza antigens in 0.5-mL volume: A/California/7/2009 (H1N1)pdm09, A/Hong Kong/4801/2014 (H3N2), and B/Brisbane/60/2008. During the informed consent process, consecutive patients had the option of also consenting for the CMI substudy and providing whole blood for CMI testing. All patients were given this option until the a priori sample size of 60 was reached. The randomization and blinding process has been previously described. The study was approved by the institutional research ethics board, as well as Health Canada for the off-label use of the HD vaccine. The study was registered under clinicaltrials.gov (NCT03139565).
Peripheral Blood Mononuclear Cells Stimulation and Flow Cytometry
Blood was obtained before and 4 weeks after vaccination. Peripheral blood mononuclear cells (PBMCs) were extracted from whole blood within 24 hours of collection using Ficoll gradient centrifugation (GE Healthcare Life Science, Issaquah, WA) and cryopreserved in fetal bovine serum (Gibco; Thermo Fisher Scientific, Waltham, MA) with 10% dimethyl sulfoxide (Fisher BioReagents; Thermo Fisher Scientific). To stimulate influenza-associated responses, 5 × 105 PBMCs pre- and postvaccination were stimulated immediately after thawing separately with 1 µg/mL each of 3 vaccine antigens or with media alone for 16 hours. Vaccine antigens corresponding to A/California/7/2009 (H1N1)pdm09, A/Hong Kong/4801/2014 (H3N2), and B/Brisbane/60/2008 were obtained from the National Institute for Biological Standards and Control ([Hertfordshire, UK] respective codes 16/106, 16/286, and 16/118) prepared from beta-propiolactone, formaldehyde, and formalin-inactivated virus, respectively. Antigens were reconstituted according to the manufacturer’s instructions. The BD FastImmune antihuman CD28/CD49d costimulatory reagent (BD Biosciences, Mississauga, ON, Canada) and the protein transport inhibitor cocktail of Brefeldin A and Monensin (eBioscience; ThermoFisher Scientific) were added at the same time as the antigens. After stimulation, cells were centrifuged and stained with the Zombie NIR viability dye (BioLegend, San Diego, CA). After Fc receptor blocking using Human BD Fc Block (BD Biosciences), cells were incubated with a cell-surface cocktail consisting of mouse antihuman CD45 (clone HI30)-PerCP/Cy5.5 (BioLegend), mouse antihuman CD3 (clone OKT3)-PE/Cy7 (BioLegend), mouse antihuman CD4 (clone SK3)-pacific blue (BioLegend), and mouse antihuman CD8 (clone RPA-T8)-V500 (BD Biosciences). After incubation with fixation buffer (BioLegend), cells were treated with an intracellular cytokine antibody cocktail prepared in permeabilization wash buffer (BioLegend). The intracellular cocktail consisted of mouse antihuman interferon (IFN)-γ (clone 4S.B3)-FITC (BioLegend), mouse antihuman tumour-necrosis factor (TNF)-α (clone MAb11)-BV605 (BioLegend), rat antihuman interleukin (IL)-2 (clone MQ1-17H12)-BV650 (BioLegend), and rat antihuman IL-4 (clone MP4-25D2)-PE-dazzle (BioLegend). Flow cytometry was performed on a BD LSR II at The SickKids-UHN Flow and Mass Cytometry Facility (Toronto, ON, Canada) with a target event count of 100 000 live, CD45+ cells. The data acquired were analyzed using FlowJo software, version 10 (FlowJo LLC, Ashland, OR). To account for background cytokine production, the frequency of cytokine producing obtained in the unstimulated specimen was subtracted from the frequency obtained in the stimulated specimen. To measure vaccine-associated T-cell responses, the prevaccination frequency of cytokine-producing cells was subtracted from the frequency postvaccination. In the absence of vaccine-associated response, specimens were given a value of 1 cytokine-producing cell per 106 CD4+ or CD8+ T cells. Cells producing at least 2 cytokines among TNF-α, IFN-γ, and IL-2 were considered polyfunctional cells and were determined using Boolean gating. We did not consider IL-4 as part of polyfunctionality based on previous published data that focus primarily on TNF-α, IFN-γ, and IL-2, which are a typical Th1 subset [17, 18]. However, given the importance of IL-4 as a hallmark Th2 cytokine, IL-4 was evaluated as an individual cytokine along with TNF-α, IFN-γ, and IL-2. A representative gating strategy for the identification of cytokine-producing CD4+ and CD8+ T cells is presented in Supplementary Figure 1.
Outcomes and Statistical Analysis
The primary outcome was the proportion of influenza-specific polyfunctional T cells elicited by vaccine antigens. Seroconversion was defined as a ≥4-fold increase in antibody titers compared with prevaccination as well as reaching a seroprotective titer. To evaluate clinical factors associated with a polyfunctional T-cell vaccine response, we defined vaccine responders as patients with the presence of vaccine-elicited antigen-specific polyfunctional cells for at least 2 influenza antigens. Analyses were performed in immunized patients for which both baseline and postvaccine specimens were available, per-protocol. For group comparisons, χ 2 or Fisher’s exact test were used for dichotomous variables, whereas Mann-Whitney U test was used for continuous variables. To determine whether CMI and GMTs postvaccination were correlated, we used Spearman’s coefficient. To further evaluate the relationship between humoral and cellular immunity, we compared the frequencies of vaccine-elicited CD4+ and CD8+ T cells between seroconverters and non-seroconverters using the Mann-Whitney U test. P < .05 was considered statistically significant, except for the evaluation of cytokine-specific responses where P < .02 was considered statistically significant to account for multiple comparisons. Statistics were performed using SPSS version 23.0 (IBM Corp., Armonk, NY). Figures were made using GraphPad, version 7.0 (La Jolla, CA). Heatmaps were generated using the Heatmapper web server [19].
RESULTS
Patient Population
We enrolled 60 patients into the CMI substudy (30 HD; 30 SD). Baseline characteristics are shown in Table 1 and were similar between the 2 groups except for a greater proportion of male patients in the SD group (P = .02). Immunosuppression and type of transplant were similar between the 2 groups. No patient had been diagnosed with rejection in the previous 6 months. Of the 60 enrolled patients with prevaccination PBMCs, 5 in the SD group did not provide a postvaccination sample. Therefore, 25 patients in the SD group and 30 in the HD group were analyzed. The proportion of prevaccine and postvaccine CD4+ and CD8+ T cells among live CD45+ cells did not significantly differ between HD and SD group (data not shown). During the 6-month follow-up period, there were no cases of documented influenza or acute rejection. Hospitalization rates, all unrelated to vaccination, were 5 of 25 (20%) in the SD group and 4 of 30 (13.3%) in the HD group (P = .721).
. | All (n = 55) . | Standard-Dose Vaccine (n = 25) . | High-Dose Vaccine (n = 30) . | P Value . |
---|---|---|---|---|
Median age, years (IQR) | 57 (46–63) | 59 (50–63) | 57 (43–63) | .41 |
Male sex | 39 (70.9%) | 22 (88.0%) | 17 (56.7%) | .02 |
Median time from transplantation to vaccination, months (IQR) | 58 (14–90) | 42 (12–90) | 64 (16–94) | .85 |
Within 1 year of transplantation | 12 (21.8%) | 6 (24.0%) | 6 (20.0%) | .72 |
Previous year vaccination | 40 (72.7%) | 19 (76.0%) | 21 (70.0%) | .62 |
History of documented influenzab | 1 (1.8%) | 0 (0%) | 1 (3.3%) | .99 |
Antithymocyte globulin within 6 months prior | 1 (1.8%) | 1 (4.0%) | 0 (0%) | .46 |
Type of Transplant | ||||
Kidney | 22 (40.0%) | 6 (24.0%) | 16 (53.3%) | .29 |
Liver | 9 (16.4%) | 5 (20.0%) | 4 (13.3%) | |
Lung | 8 (14.5%) | 5 (20.0%) | 3 (10.0%) | |
Heart | 7 (12.7%) | 4 (16.0%) | 3 (10.0%) | |
Combined | 9 (16.4%) | 5 (20%) | 4 (13.3%) | |
Immunosuppression | ||||
Prednisone | 44 (80.0%) | 19 (76.0%) | 25 (83.3%) | .50 |
Tacrolimus | 40 (72.7%) | 16 (64.0%) | 24 (80.0%) | .19 |
Cyclosporine | 13 (23.6%) | 9 (36.0%) | 4 (13.3%) | .06 |
MMF/MPS | 38 (69.1%) | 18 (72.0%) | 20 (66.7%) | .67 |
Azathioprine | 4 (7.2%) | 3 (12.0%) | 1 (3.3%) | .32 |
Sirolimus | 2 (3.6%) | 1 (4.0%) | 1 (3.3%) | .99 |
. | All (n = 55) . | Standard-Dose Vaccine (n = 25) . | High-Dose Vaccine (n = 30) . | P Value . |
---|---|---|---|---|
Median age, years (IQR) | 57 (46–63) | 59 (50–63) | 57 (43–63) | .41 |
Male sex | 39 (70.9%) | 22 (88.0%) | 17 (56.7%) | .02 |
Median time from transplantation to vaccination, months (IQR) | 58 (14–90) | 42 (12–90) | 64 (16–94) | .85 |
Within 1 year of transplantation | 12 (21.8%) | 6 (24.0%) | 6 (20.0%) | .72 |
Previous year vaccination | 40 (72.7%) | 19 (76.0%) | 21 (70.0%) | .62 |
History of documented influenzab | 1 (1.8%) | 0 (0%) | 1 (3.3%) | .99 |
Antithymocyte globulin within 6 months prior | 1 (1.8%) | 1 (4.0%) | 0 (0%) | .46 |
Type of Transplant | ||||
Kidney | 22 (40.0%) | 6 (24.0%) | 16 (53.3%) | .29 |
Liver | 9 (16.4%) | 5 (20.0%) | 4 (13.3%) | |
Lung | 8 (14.5%) | 5 (20.0%) | 3 (10.0%) | |
Heart | 7 (12.7%) | 4 (16.0%) | 3 (10.0%) | |
Combined | 9 (16.4%) | 5 (20%) | 4 (13.3%) | |
Immunosuppression | ||||
Prednisone | 44 (80.0%) | 19 (76.0%) | 25 (83.3%) | .50 |
Tacrolimus | 40 (72.7%) | 16 (64.0%) | 24 (80.0%) | .19 |
Cyclosporine | 13 (23.6%) | 9 (36.0%) | 4 (13.3%) | .06 |
MMF/MPS | 38 (69.1%) | 18 (72.0%) | 20 (66.7%) | .67 |
Azathioprine | 4 (7.2%) | 3 (12.0%) | 1 (3.3%) | .32 |
Sirolimus | 2 (3.6%) | 1 (4.0%) | 1 (3.3%) | .99 |
Abbreviations: IQR, interquartile range. MMF, Mycophenolate mofetil; MPS, Mycophenolate sodium.
aAll patients that received study vaccine and had peripheral blood mononuclear cells collected at both time points.
bBased on documentation in the transplant chart for the 2015–16 influenza season.
NOTE: χ 2 or Fisher exact tests were used for dichotomous variables, whereas Mann-Whitney U test was used for continuous variables.
. | All (n = 55) . | Standard-Dose Vaccine (n = 25) . | High-Dose Vaccine (n = 30) . | P Value . |
---|---|---|---|---|
Median age, years (IQR) | 57 (46–63) | 59 (50–63) | 57 (43–63) | .41 |
Male sex | 39 (70.9%) | 22 (88.0%) | 17 (56.7%) | .02 |
Median time from transplantation to vaccination, months (IQR) | 58 (14–90) | 42 (12–90) | 64 (16–94) | .85 |
Within 1 year of transplantation | 12 (21.8%) | 6 (24.0%) | 6 (20.0%) | .72 |
Previous year vaccination | 40 (72.7%) | 19 (76.0%) | 21 (70.0%) | .62 |
History of documented influenzab | 1 (1.8%) | 0 (0%) | 1 (3.3%) | .99 |
Antithymocyte globulin within 6 months prior | 1 (1.8%) | 1 (4.0%) | 0 (0%) | .46 |
Type of Transplant | ||||
Kidney | 22 (40.0%) | 6 (24.0%) | 16 (53.3%) | .29 |
Liver | 9 (16.4%) | 5 (20.0%) | 4 (13.3%) | |
Lung | 8 (14.5%) | 5 (20.0%) | 3 (10.0%) | |
Heart | 7 (12.7%) | 4 (16.0%) | 3 (10.0%) | |
Combined | 9 (16.4%) | 5 (20%) | 4 (13.3%) | |
Immunosuppression | ||||
Prednisone | 44 (80.0%) | 19 (76.0%) | 25 (83.3%) | .50 |
Tacrolimus | 40 (72.7%) | 16 (64.0%) | 24 (80.0%) | .19 |
Cyclosporine | 13 (23.6%) | 9 (36.0%) | 4 (13.3%) | .06 |
MMF/MPS | 38 (69.1%) | 18 (72.0%) | 20 (66.7%) | .67 |
Azathioprine | 4 (7.2%) | 3 (12.0%) | 1 (3.3%) | .32 |
Sirolimus | 2 (3.6%) | 1 (4.0%) | 1 (3.3%) | .99 |
. | All (n = 55) . | Standard-Dose Vaccine (n = 25) . | High-Dose Vaccine (n = 30) . | P Value . |
---|---|---|---|---|
Median age, years (IQR) | 57 (46–63) | 59 (50–63) | 57 (43–63) | .41 |
Male sex | 39 (70.9%) | 22 (88.0%) | 17 (56.7%) | .02 |
Median time from transplantation to vaccination, months (IQR) | 58 (14–90) | 42 (12–90) | 64 (16–94) | .85 |
Within 1 year of transplantation | 12 (21.8%) | 6 (24.0%) | 6 (20.0%) | .72 |
Previous year vaccination | 40 (72.7%) | 19 (76.0%) | 21 (70.0%) | .62 |
History of documented influenzab | 1 (1.8%) | 0 (0%) | 1 (3.3%) | .99 |
Antithymocyte globulin within 6 months prior | 1 (1.8%) | 1 (4.0%) | 0 (0%) | .46 |
Type of Transplant | ||||
Kidney | 22 (40.0%) | 6 (24.0%) | 16 (53.3%) | .29 |
Liver | 9 (16.4%) | 5 (20.0%) | 4 (13.3%) | |
Lung | 8 (14.5%) | 5 (20.0%) | 3 (10.0%) | |
Heart | 7 (12.7%) | 4 (16.0%) | 3 (10.0%) | |
Combined | 9 (16.4%) | 5 (20%) | 4 (13.3%) | |
Immunosuppression | ||||
Prednisone | 44 (80.0%) | 19 (76.0%) | 25 (83.3%) | .50 |
Tacrolimus | 40 (72.7%) | 16 (64.0%) | 24 (80.0%) | .19 |
Cyclosporine | 13 (23.6%) | 9 (36.0%) | 4 (13.3%) | .06 |
MMF/MPS | 38 (69.1%) | 18 (72.0%) | 20 (66.7%) | .67 |
Azathioprine | 4 (7.2%) | 3 (12.0%) | 1 (3.3%) | .32 |
Sirolimus | 2 (3.6%) | 1 (4.0%) | 1 (3.3%) | .99 |
Abbreviations: IQR, interquartile range. MMF, Mycophenolate mofetil; MPS, Mycophenolate sodium.
aAll patients that received study vaccine and had peripheral blood mononuclear cells collected at both time points.
bBased on documentation in the transplant chart for the 2015–16 influenza season.
NOTE: χ 2 or Fisher exact tests were used for dichotomous variables, whereas Mann-Whitney U test was used for continuous variables.
Prevaccine Influenza-Specific Immunity
Before immunization, the number of influenza-specific cytokine-producing cells in the whole cohort ranged between 26 and 825 per 106 CD4+ T cells and between 0 to 283 per 106 CD8+ T cells, depending on the cytokine and the antigen (Supplementary Table 1).
Postvaccine Influenza-Specific Immunity
In the whole cohort, responses were overall greater for influenza-specific CD4+ than for CD8+ T cells (Figure 1). In addition, responses were similar across the 3 vaccine antigens (Figure 1). For both CD4+ and CD8+ T cells, TNF-α and IL-2 were the cytokines contributing the most to polyfunctional cells (Figure 2A and B). It is interesting to note that more than one third of polyfunctional CD4+ T cells but only 11% of polyfunctional CD8+ T cells were triple-positive cells (Figure 2A and B).

Comparison of median number of influenza-specific cytokine-producing CD4+ and CD8+ T cells after stimulation with influenza A/H1N1, influenza A/H3N2, and influenza B between recipients of standard-dose (SD) and high-dose (HD) vaccines. Results were expressed as number of vaccine-elicited cytokine-producing CD4+ cells/106 CD4+ T cells and CD8+ cells/106 CD8+ T cells. Prevaccination frequencies were subtracted from postvaccination frequencies to highlight vaccine-elicited responses. IFN-γ, interferon-γ; IL, interleukin; TNF-α, tumor necrosis factor α.
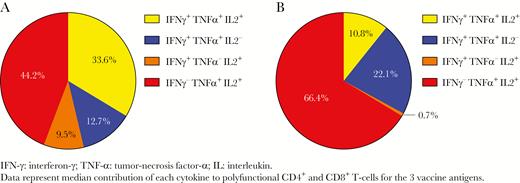
Relative contribution of interferon-γ (IFN-γ), tumor necrosis factor α (TNF-α ), and interleukin (IL)-2 to polyfunctional CD4+ (A) and CD8+ (B) T cells among the study cohort. Data represent the median contribution of each cytokine to polyfunctional CD4+ and CD8+ T cells for the 3 vaccine antigens.
CD4+ T-Cell Responses>
The HD vaccine elicited a 1.4- to 1.6-fold increase in polyfunctional CD4+ T cells, whereas the SD elicited no increase in polyfunctional CD4+ T cells (Figure 3A). After stimulation with influenza A/H1N1, patients who received the HD vaccine had a greater increase in influenza-specific IFNγ +, TNFα +, and polyfunctional CD4+ T cells compared with SD vaccine (P = .003, P = .016, and P = .003, respectively) (Figure 1 and Supplementary Figure 2). There was also a trend towards a greater increase in IL4+ CD4+ T cells among recipients of the HD vaccine (P = .049) (Figure 1 and Supplementary Figure 2). After stimulation with influenza A/H3N2, patients who received the HD vaccine had a greater increase in influenza-specific TNFα + and polyfunctional CD4+ T cells compared with SD vaccine (P = .014 and P = .008, respectively) (Figure 1 and Supplementary Figure 2). There was also a trend towards a greater increase in IFNγ + and IL4+ CD4+ T cells among recipients of the HD vaccine (P = .043 and P = .035, respectively) (Figure 1 and Supplementary Figure 2). Finally, after stimulation with influenza B, patients who received the HD vaccine had a greater increase in influenza-specific IFNγ +, TNFα +, IL2+, polyfunctional, and IL4+ CD4+ T cells compared with SD vaccine (P = .009, P = .003, P = .017, P = .001, and P = .001, respectively) (Figure 1 and Supplementary Figure 2).
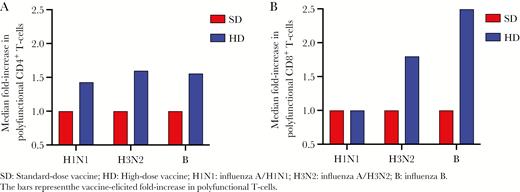
Median fold-increase in influenza-specific polyfunctional CD4+ (A) and CD8+ (B) T cells. The bars represent the vaccine-elicited fold-increase in polyfunctional T cells. B, influenza B; HD, high-dose vaccine; H1N1, influenza A/H1N1; H3N2, influenza A/H3N2; SD, standard-dose vaccine.
CD8+ T-Cell Responses
The HD vaccine elicited a 1.8- to 2.5-fold increase in polyfunctional CD8+ T cells in response to stimulation with influenza A/H3N2 and B, but no increase after stimulation with influenza A/H1N1. As a comparison, the SD elicited no increase in polyfunctional CD8+ T cells (Figure 3B). After stimulation with influenza A/H1N1, patients who received the HD vaccine had trend towards a greater increase in influenza-specific IFNγ + and TNFα + CD8+ T cells (P = .047 for both) (Figure 1 and Supplementary Figure 3). There was no difference in influenza-specific cytokine-producing CD8+ T cells after stimulation with influenza A/H3N2 (Figure 1 and Supplementary Figure 3). Patients who received the HD vaccine had a greater increase in influenza-specific TNFα + and polyfunctional CD8+ T cells after stimulation with influenza B (P = .007 and P = .002, respectively) (Figure 1 and Supplementary Figure 3).
Factors Associated With Development of Cell-Mediated Immunity
For this subanalysis, our definition of an adequate CMI response to vaccine was any increase in antigen-specific polyfunctional T cells for at least 2 influenza antigens. Based on this definition, an overall positive CMI response was observed in 35 patients for CD4+ (24 HD; 11 SD) and 28 patients for CD8+ (18 HD; 10 SD). In an analysis of factors related to a positive response, lung transplant recipients were less frequent among CD4+ responders (9%) than nonresponders (30%; P = .048). Likewise, recipients of the HD vaccine were more likely to be CD4+ responders (69%) than nonresponders (30%; P = .006) (Table 2).
Clinical Factors Associated With CD4+ and CD8+ T-Cell Polyfunctional Response to at Least 2 Influenza Antigensa
. | CD4+ . | CD8+ . | ||||
---|---|---|---|---|---|---|
. | Nonresponders n = 20 . | Responders n = 35 . | P Value . | Nonrespondersn = 27 . | Respondersn = 28 . | P Value . |
Female sex | 5 (25.0) | 11 (31.4) | 0.61 | 5 (18.5) | 11(39.3) | .09 |
Previous year vaccination | 13 (65.0) | 27 (77.1) | 0.33 | 19 (70.4) | 21 (75.0) | .70 |
Prednisone | 14 (70.0) | 30 (85.7) | 0.16 | 20 (74.1) | 24 (85.7) | .33 |
Calcineurin inhibitor | 19 (95.0) | 34 (97.1) | 0.99 | 26 (96.3) | 27 (96.4) | .99 |
Mycophenolate | 13 (65.0) | 25 (71.4) | 0.62 | 16 (59.3) | 22 (78.6) | .12 |
Lung transplantation | 6 (30.0) | 3 (8.6) | 0.048 | 5 (18.5) | 4 (14.3) | .73 |
Within 12 months of transplant | 3 (15.0) | 9 (25.7) | 0.50 | 8 (29.6) | 4 (14.3) | .21 |
≥Than 65 years old | 3 (15.0) | 5 (14.3) | 0.99 | 4 (14.8) | 4 (14.3) | .99 |
High-dose influenza vaccine | 6 (30.0) | 24 (68.6) | 0.006 | 12 (44.4) | 18 (64.3) | .14 |
. | CD4+ . | CD8+ . | ||||
---|---|---|---|---|---|---|
. | Nonresponders n = 20 . | Responders n = 35 . | P Value . | Nonrespondersn = 27 . | Respondersn = 28 . | P Value . |
Female sex | 5 (25.0) | 11 (31.4) | 0.61 | 5 (18.5) | 11(39.3) | .09 |
Previous year vaccination | 13 (65.0) | 27 (77.1) | 0.33 | 19 (70.4) | 21 (75.0) | .70 |
Prednisone | 14 (70.0) | 30 (85.7) | 0.16 | 20 (74.1) | 24 (85.7) | .33 |
Calcineurin inhibitor | 19 (95.0) | 34 (97.1) | 0.99 | 26 (96.3) | 27 (96.4) | .99 |
Mycophenolate | 13 (65.0) | 25 (71.4) | 0.62 | 16 (59.3) | 22 (78.6) | .12 |
Lung transplantation | 6 (30.0) | 3 (8.6) | 0.048 | 5 (18.5) | 4 (14.3) | .73 |
Within 12 months of transplant | 3 (15.0) | 9 (25.7) | 0.50 | 8 (29.6) | 4 (14.3) | .21 |
≥Than 65 years old | 3 (15.0) | 5 (14.3) | 0.99 | 4 (14.8) | 4 (14.3) | .99 |
High-dose influenza vaccine | 6 (30.0) | 24 (68.6) | 0.006 | 12 (44.4) | 18 (64.3) | .14 |
aχ 2 or Fisher exact tests were used for groups comparisons.
Clinical Factors Associated With CD4+ and CD8+ T-Cell Polyfunctional Response to at Least 2 Influenza Antigensa
. | CD4+ . | CD8+ . | ||||
---|---|---|---|---|---|---|
. | Nonresponders n = 20 . | Responders n = 35 . | P Value . | Nonrespondersn = 27 . | Respondersn = 28 . | P Value . |
Female sex | 5 (25.0) | 11 (31.4) | 0.61 | 5 (18.5) | 11(39.3) | .09 |
Previous year vaccination | 13 (65.0) | 27 (77.1) | 0.33 | 19 (70.4) | 21 (75.0) | .70 |
Prednisone | 14 (70.0) | 30 (85.7) | 0.16 | 20 (74.1) | 24 (85.7) | .33 |
Calcineurin inhibitor | 19 (95.0) | 34 (97.1) | 0.99 | 26 (96.3) | 27 (96.4) | .99 |
Mycophenolate | 13 (65.0) | 25 (71.4) | 0.62 | 16 (59.3) | 22 (78.6) | .12 |
Lung transplantation | 6 (30.0) | 3 (8.6) | 0.048 | 5 (18.5) | 4 (14.3) | .73 |
Within 12 months of transplant | 3 (15.0) | 9 (25.7) | 0.50 | 8 (29.6) | 4 (14.3) | .21 |
≥Than 65 years old | 3 (15.0) | 5 (14.3) | 0.99 | 4 (14.8) | 4 (14.3) | .99 |
High-dose influenza vaccine | 6 (30.0) | 24 (68.6) | 0.006 | 12 (44.4) | 18 (64.3) | .14 |
. | CD4+ . | CD8+ . | ||||
---|---|---|---|---|---|---|
. | Nonresponders n = 20 . | Responders n = 35 . | P Value . | Nonrespondersn = 27 . | Respondersn = 28 . | P Value . |
Female sex | 5 (25.0) | 11 (31.4) | 0.61 | 5 (18.5) | 11(39.3) | .09 |
Previous year vaccination | 13 (65.0) | 27 (77.1) | 0.33 | 19 (70.4) | 21 (75.0) | .70 |
Prednisone | 14 (70.0) | 30 (85.7) | 0.16 | 20 (74.1) | 24 (85.7) | .33 |
Calcineurin inhibitor | 19 (95.0) | 34 (97.1) | 0.99 | 26 (96.3) | 27 (96.4) | .99 |
Mycophenolate | 13 (65.0) | 25 (71.4) | 0.62 | 16 (59.3) | 22 (78.6) | .12 |
Lung transplantation | 6 (30.0) | 3 (8.6) | 0.048 | 5 (18.5) | 4 (14.3) | .73 |
Within 12 months of transplant | 3 (15.0) | 9 (25.7) | 0.50 | 8 (29.6) | 4 (14.3) | .21 |
≥Than 65 years old | 3 (15.0) | 5 (14.3) | 0.99 | 4 (14.8) | 4 (14.3) | .99 |
High-dose influenza vaccine | 6 (30.0) | 24 (68.6) | 0.006 | 12 (44.4) | 18 (64.3) | .14 |
aχ 2 or Fisher exact tests were used for groups comparisons.
When each antigen was analyzed individually, recipients of the HD vaccine were still more likely than recipients of the SD vaccine to have polyfunctional CD4+ T cells against influenza A/H1 (77% vs 48%; P = .028), A/H3 (80% vs 52%; P = .028), and B (83% vs 36%; P < .001). It is interesting to note that receipt of a lung transplant was not associated with a lower likelihood of polyfunctional CD4+ T cells when antigens were analyzed individually. Likewise, gender, previous year vaccination, immunosuppressive regimen, age ≥65 years, and receiving a transplant <12 months ago were overall not associated with the presence or absence of polyfunctional cells when antigens were analyzed individually (data not shown).
Relationship Between Humoral and Cell-Mediated Immunity
There was no correlation between antibody titers and influenza-specific cytokine-producing CD4+ or CD8+ T cells in the whole cohort as well as when SD and HD groups were analyzed separately (data not shown). We then compared whether SC was associated with the proportion of influenza-specific T cells. Overall, SC in the cohort was 29.1% (n = 16), 50.9% (n = 28), and 52.7% (n = 29) for influenza A/H1N1, A/H3N2, and B, respectively (Figure 4). After stimulation with influenza A/H1N1, there was no difference in influenza-specific cytokine-producing CD4+ or CD8+ T cells between patients who seroconverted and those who did not (Figure 4). After stimulation with influenza A/H3N2, there was no difference in influenza-specific cytokine-producing cells between patients who seroconverted and those who did not, except that SC was associated with a greater median frequency of IFNγ + CD4+ T cells (P = .048) (Figure 4). After stimulation with influenza B, SC was associated with a greater median frequency of TNFα +, IL4+, IL2+, and polyfunctional CD4+ T cells (P = .003, P = .03, P = .005, and P = .01, respectively) and IL2+CD8+ T cells (P = .03) (Figure 4).

Heatmap of influenza-specific cytokine-producing CD4+ and CD8+ T cells in response to stimulation with influenza A/H1N1, influenza A/H3N2, and influenza B. Each row represents a single patient. Values are expressed as Z-score per column. Blue and red colors represent higher and lower percentage of cytokine-producing cells, respectively. IFN-γ, interferon-γ; IL, interleukin; TNF-α, tumor necrosis factor α.
DISCUSSION
We compared the development of T-cell immune responses in adult organ transplant recipients that received HD and SD influenza vaccine. Our study showed that HD vaccine elicited significantly greater T-cell responses than the SD vaccine in SOT recipients and is consistent with our previous data showing better humoral immunogenicity of the HD vaccine as well [14]. It is interesting to note, however, that the humoral immune response was not directly correlated with the CMI response, and CMI responses were similar across the 3 vaccine antigens. The greater T-cell immunogenicity of the HD vaccine is especially important because some studies have shown that CMI is a better correlate of protection against influenza than humoral immunity in elderly persons, who are known for poor vaccine responses [15, 16], although this has not been confirmed in the SOT setting. In addition, whereas humoral immunity may protect against infection, cellular immune responses have been shown to reduce the severity of disease [20]. The improved cellular immunogenicity of the HD vaccine was especially evident for CD4+ T-cell responses, in keeping with some findings that influenza vaccine elicits a greater CD4+ than CD8+ response [15, 21, 22]. Moreover, our study also showed that the HD vaccine elicited an up to 2.5-fold increase in polyfunctional cells, whereas the SD vaccine elicited no increase in polyfunctional cells. This is very relevant, because polyfunctional cells are considered to be correlated with the quality of the T-cell response in several models (reviewed in [23]). In other pathogens, polyfunctional T cells have been associated with better outcomes after infection [24] and better protection after vaccination [17, 18]. In influenza, it has been shown that polyfunctional CD4+ cells were functionally superior than monofunctional cells, by producing higher amounts of cytokine per cell, more CD40L, and more degranulation [25]. Data have shown that non-immunocompromised patients develop polyfunctional T cells after influenza immunization [26]. In patients infected with H1N1, a higher proportion of polyfunctional CD4+ T cells was associated with reduced disease severity [27]. In mice, polyfunctional cells expressing IFN-γ and perforin have been shown to protect against lethal influenza infection [28].
Although several studies have shown better humoral immunity of the HD vaccine in elderly adults [10–13], there are limited data analyzing cell-mediated immune responses with the HD vaccine. Two studies in non-immunocompromised adults were not able to demonstrate a significant difference in CMI between HD and SD vaccine recipients [29, 30]. This may be because the SD vaccine already elicits a reasonable degree of CMI in immunocompetent persons, and therefore the incremental benefit of HD vaccine may not be demonstrable [15, 16, 30]. In the SOT setting, the SD vaccine elicited poor CMI responses, and therefore we were able to demonstrate a significant difference. Despite the randomization, there was a nonsignificant overrepresentation of kidney transplant recipients in the HD group, whereas other organ types were more frequent in the SD group. We cannot exclude that this partially contributed to the better cellular responses of the HD vaccine recipients.
We also evaluated the clinical factors associated with the development of CMI. Lung transplant recipients were at risk for lower CD4+ T-cell immunity, even though this should be interpreted with caution given the small number of lung transplant patients in our cohort. The lower response in lung transplant patients is most likely related to their net state of immunosuppression and is consistent with the work from Mazzone et al [31] who showed that lung transplant recipients did not develop CMI after the SD vaccine, whereas kidney transplant recipients did develop CMI after the SD vaccine, at either a similar or lower level than immunocompetent subjects [5, 32].
Studies have shown conflicting data for the correlation between humoral and cell-mediated immunity in the general population after the SD vaccine [21, 30, 32, 33], whereas a moderate correlation between CD4 and antibody responses was found for the HD vaccine [30]. In the SOT setting, the majority of studies (using SD vaccine) have not been able to establish a strong correlation between humoral and cellular immunity [5, 32, 34], except Egli et al [35] who found a correlation between SC and IL4+CD4+ T cells after stimulation with influenza A/H1N1. Even though our findings did not highlight a significant correlation between antibody titers and cellular immunity, patients who seroconverted were more likely to have higher vaccine-elicited cellular responses, especially after stimulation with influenza B. The absence of correlation between humoral and cellular immunity observed in our study could also be related to the sample size or the absence of other postvaccination study time-points.
Overall, the proportion of influenza-specific cytokine-producing cells in our study was low, ranging between 0 and 200 cells per 106 CD4+ or CD8+ in the SD vaccine group, compared with 100–2000 and 0–600 cells per 106 CD4+ and CD8+ cells, respectively, in the HD vaccine group. Overall, these responses are similar to those in non-immunocompromised individuals [31, 32, 35]. Using enzyme-linked immunospot, studies have shown that non-immunocompromised persons who received the SD vaccine developed less than 1800 IFNγ + spot-forming cells/106 PBMCs [29, 33, 36, 37], whereas transplant recipients developed 68 IFNγ + spot-forming cells/106 PBMCs [34]. Using flow cytometry, several studies have also shown the proportion of influenza-specific cells to be approximately 100–5000 cells per 106 CD8+ [22, 38–40] and 50–6000 cells per 106 CD4+ T cells after the SD vaccine [22, 26, 37–41]. The variability in CMI between studies is likely due to patient age, varying seasonal SD vaccine formulations, exposure to antigenically variable strains, different antigen stimulation techniques, and different methods used to determine CMI.
Our study has some limitations. We had a small cohort on which to test CMI. Nevertheless, we were able to show significant differences between SD and HD vaccine. However, having a bigger sample size as well as another postvaccination timepoint (such as Day 7) might have helped to explain the lack of correlation between humoral and cellular immunity. In addition, a CMI level that correlates with clinical protection is not defined; therefore, it is unknown whether the CMI elicited by the SD or HD vaccine is protective from natural influenza infection. We purposely did not include IL-4 in our definition of polyfunctional cells, based on the available literature mostly focusing on TNF-α, IFN-γ, and IL-2 [17, 18]. However, given the small percentage of IL4+CD4+ or CD8+ T cells, it is unlikely that including IL-4 would have had any significant influence on the results. A 6-month postvaccination timepoint would help in evaluating sustainability of vaccine response and is a subject for future study.
CONCLUSIONS
In conclusion, we provide novel data that demonstrates enhanced T-cell immunity elicited by the HD vaccine when compared with the SD vaccine in SOT recipients, with an up to 2.5-fold increase in influenza-specific cells in the HD group. These data, alongside prior data showing better humoral immunogenicity in SOT recipients and a similar safety profile when compared with the SD vaccine [14], provide evidence that HD vaccine may be the preferred vaccine in this population from both a humoral and T-cell immunity perspective.
Supplementary Data
Supplementary materials are available at The Journal of Infectious Diseases online. Consisting of data provided by the authors to benefit the reader, the posted materials are not copyedited and are the sole responsibility of the authors, so questions or comments should be addressed to the corresponding author.
Notes
Acknowledgments. We acknowledge Peter Ashton and Srinivas Nellimarla for their logistical support during the study.
Financial support. This work was funded by the Multi-Organ Transplant Program. FluzoneHD was purchased from Sanofi, Canada. Fluviral was obtained from the Ontario public health supply. A. G. L. is funded by a Swiss National Science Foundation Advanced Postdoc Mobility Grant (P300PB_171603). C. H. was supported by an early Postdoc.Mobility grant from the Swiss National Science Foundation (P2BEP3_175265).
Potential conflicts of interest. D. K. has received clinical trials grants from Roche and GSK and honoraria from Sanofi, GSK. A. H. has received a clinical trials grant from Roche. All authors have submitted the ICMJE Form for Disclosure of Potential Conflicts of Interest. Conflicts that the editors consider relevant to the content of the manuscript have been disclosed.
Presented in part: 2019 American Transplant Congress, June 1–5, 2019, Boston, MA.
References
Author notes
A. H. and D. K. are co-senior authors.