-
PDF
- Split View
-
Views
-
Cite
Cite
Ilona Baraniak, Florian Kern, Pavlo Holenya, Paul Griffiths, Matthew Reeves, Original Antigenic Sin Shapes the Immunological Repertoire Evoked by Human Cytomegalovirus Glycoprotein B/MF59 Vaccine in Seropositive Recipients, The Journal of Infectious Diseases, Volume 220, Issue 2, 15 July 2019, Pages 228–232, https://doi.org/10.1093/infdis/jiz089
- Share Icon Share
Abstract
A human cytomegalovirus (HCMV) vaccine is urgently needed to protect against primary infection and enhance existing immunity in HCMV-infected individuals (HCMV+). Using sera from HCMV+ glycoprotein B/MF59 vaccine recipients prior to transplant, we investigated the composition of the immune response. Vaccination boosted preexisting humoral responses in our HCMV+ cohort but did not promote de novo responses against novel linear epitopes. This suggests that prior natural infection has a profound effect on shaping the antibody repertoire and subsequent response to vaccination (“original antigenic sin”). Thus, vaccination of HCMV+ may require strategies of epitope presentation distinct from those intended to prevent primary infection.
Human cytomegalovirus (HCMV) infection is common, with seroprevalence ranging from 60% to 100% [1]. HCMV can promote substantial mortality and morbidity in immunocompromised individuals, including solid organ transplant (SOT) recipients [2]. In these patients, HCMV end-organ disease results from primary infection, reinfection, or reactivation [3]. The most successful vaccine studied to date is recombinant glycoprotein B (gB) with MF59 adjuvant, which demonstrated partial efficacy in reducing viremia after SOT and similar efficacy in preventing primary infection in women and adolescents [4, 5]. Although the mechanism of protection is not fully understood, we have previously reported that higher levels of total anti-gB immunoglobulin G (IgG) antibody correlated with a shorter duration of posttransplantation viremia [6].
In HCMV-infected (HCMV+) individuals, the vaccine clearly boosted preexisting antibody responses [7]. Furthermore, detailed analyses of humoral responses against well-defined antigenic domains (AD1, AD2, AD4, and AD5) in seropositive individuals revealed that only anti-AD2 antibody responses correlated with protection from posttransplantation viremia. Importantly, vaccination only boosted AD2 responses in the 50% of HCMV+ individuals with a preexisting response and did not induce a new AD2 response in those who lacked AD2 antibodies following natural infection. Although there was no evidence that the potent responses toward AD1, AD4, and AD5 impaired protection from AD2, it is possible that a large proportion of the antibodies elicited by natural infection (and thus boosted by vaccination) are nonprotective [7, 8]. We hypothesized that highly immunogenic domains that induce nonprotective responses might facilitate HCMV replication by diverting immune system resources away from domains that might induce more protective responses [7, 9, 10]. To begin addressing this interesting question, we used peptide array technology for scanning antibody responses to linear gB epitopes across all protein domains in 6 HCMV+ SOT recipients.
METHODS
Patient Population
The subpopulation from which samples have been evaluated and described in this work is the cohort of SOT patients who were enrolled in the phase 2 randomized and double-blinded, placebo-controlled HCMV gB vaccine with MF59 adjuvant trial. This trial was registered with ClinicalTrials.gov (identifier NCT00299260) [6]. The vaccine or placebo was given in 3 doses: at day 0 (baseline), 1 month, and 6 months later. Following vaccination, the blood samples from patients were obtained consecutively. The first 5 blood samples were collected before transplantation to measure antibodies (qualitatively and quantitatively) at baseline and after 1, 2, 6, and 7 months. The patients who subsequently underwent transplantation were followed up for 90 days during which serial blood samples were obtained around days 0, 7, 35, 63, and 90 posttransplantation. The level of viral DNA was also tested by measuring HCMV DNA by real-time quantitative polymerase chain reaction [6]. Exclusion criteria included pregnancy (a negative pregnancy test was required before each vaccine dose), receipt of blood products (except albumin) in the previous 3 months, and simultaneous multiorgan transplantation [6]. The study was approved by the UCL & NHS research ethics committee, and all patients gave written informed consent [6].
gB Peptide Microarray
To identify linear gB epitope binding, 15-mer peptides covering the entire gB open reading frame (Towne strain) and overlapping with neighboring peptides by 10 residues (total of 188 peptides) were synthesized and printed to a PepStar multiwell array (JPT Peptide) in triplicate. Microarray binding was performed manually using individual slides immobilized in the ArraySlide 24-4 chamber (JPT Peptide). First, arrays were incubated for 1 hour with sera diluted 1:200 in blocking buffer (Superblock T20 [TBS], ThermoFisher Scientific) followed by a 1-hour incubation with antihuman IgG conjugated to AF647 (Jackson ImmunoResearch) diluted in blocking buffer (0.1 μg/mL). Following each incubation step, arrays were washed 5 times in wash buffer (1× TBS buffer + 0.1% Tween) using an automated plate washer (Wellwash Versa). Array was then dried by centrifugation and scanned at a wavelength of 635 nm using an Axon Genepix 4300 SL50 scanner (Molecular Devices) at a photomultiplier tube setting of 650 and 100% laser power. Images were analyzed using Genepix Pro 7 software (Molecular Devices). Images were reviewed manually for accurate automated peptide identification. For each spot, mean signal intensity was extracted. For each peptide, the MMC2 values were calculated (the mean values of all 3 instances on the microarray, except when the coefficient of variation was >0.5; in this case, the mean of the 2 closest values was assigned to MMC2). Data analysis and graphical presentations were made using R software.
RESULTS
To characterize the antibody profile against linear epitopes of gB, serum samples from 6 HCMV+ gB/MF59 vaccine recipients were analyzed pre- and postvaccination (Figure 1; Supplementary Figures 1 and 3).
![Responses against cytosolic terminal domain (CTD/AD3) in seropositive individuals are dominant and nonprotective. A, Linear structure of defined glycoprotein B (gB) antigenic domains (ADs). The entire open reading frame of human cytomegalovirus gB are shown. The 4 distinct regions of the gB structure are indicated by black bars at the base of the figure, including the ectodomain, membrane proximal domain (MPD), transmembrane domain (TM), and the cytoplasmic domain. Major antigenic regions indicated include AD1, AD2 site 1, AD2 site 2, AD3, AD4 (domain II), and AD5 (domain I). Numbers indicate approximate amino acid residues dividing each region of interest. Diagram was adapted from Nelson et al [13]. B and C,. The highest values of antibody responses against these 5 major ADs prior to vaccination (B) and following vaccination (C) are shown for each naturally seropositive solid organ transplant patient from the R+ group. D, The highest value of immunoglobulin G (IgG) antibody responses against the immunodominant AD3 region are shown for each patient prior to vaccination and postvaccination. Median values of antibody responses are depicted by horizontal lines. Patients were further stratified for viremia posttransplantation (>200 viral genomes/mL of whole blood).](https://oup.silverchair-cdn.com/oup/backfile/Content_public/Journal/jid/220/2/10.1093_infdis_jiz089/1/m_jiz089f0001.jpeg?Expires=1749915695&Signature=tIWrqcmkw1ZH4sWuLYYC5uamd5cb5GR4Co6emdjPQb3o-HihV1bbJ8YG2BOXI2SPfBfQ525lh5F~bLs-MHXOu0EV4VJqfjg67T0Nrr6kQrajdwoAKoAvEE9vZBm5shskdfKBv5H2ypFt0bJkEVCaviMq5m0yeuGhWfc5eZKrQnes5dqgL9rDc5t1~DPqcXuYPqVSyiKAvaLg5l9ikVr31wrYYT9vW6-abK2kROTxRLomzJHMJ2tCfZSwJBqTHbuZJvhKHOhkrO3AmB92eCDfI2PweZ4frDyUa~7ybk-S~uq8qVQ6gPEBsQrwkE47qkEA4xELYy-tpBX-vhYuSwx4kA__&Key-Pair-Id=APKAIE5G5CRDK6RD3PGA)
Responses against cytosolic terminal domain (CTD/AD3) in seropositive individuals are dominant and nonprotective. A, Linear structure of defined glycoprotein B (gB) antigenic domains (ADs). The entire open reading frame of human cytomegalovirus gB are shown. The 4 distinct regions of the gB structure are indicated by black bars at the base of the figure, including the ectodomain, membrane proximal domain (MPD), transmembrane domain (TM), and the cytoplasmic domain. Major antigenic regions indicated include AD1, AD2 site 1, AD2 site 2, AD3, AD4 (domain II), and AD5 (domain I). Numbers indicate approximate amino acid residues dividing each region of interest. Diagram was adapted from Nelson et al [13]. B and C,. The highest values of antibody responses against these 5 major ADs prior to vaccination (B) and following vaccination (C) are shown for each naturally seropositive solid organ transplant patient from the R+ group. D, The highest value of immunoglobulin G (IgG) antibody responses against the immunodominant AD3 region are shown for each patient prior to vaccination and postvaccination. Median values of antibody responses are depicted by horizontal lines. Patients were further stratified for viremia posttransplantation (>200 viral genomes/mL of whole blood).
This allowed the identification of epitopes recognized during natural infection as well those induced or boosted by vaccine. Responses to several previously reported epitopes were observed including some located in the cytosolic terminal domain (CTD). Studies of the serological responses to this region are limited, with 2 studies from the early 1990s showing high serum reactivity to this region, subsequently called AD3 [11, 12]. It was speculated that, due to its location on the intraluminal, cytosolic part of gB, antibodies against this region will be most likely nonneutralizing and nonprotective. Perhaps this assumption explains why AD3 has not been given sufficient attention as a potential antibody target in the past. However, Nelson et al [13] recently analyzed sera from a cohort of HCMV− postpartum women vaccinated with gB/MF59 and subsequently found that 76% of the vaccine-induced linear IgG response recognized CTD/AD3.
Our work with HCMV+ sera shows that this also happens after natural infection, demonstrating that an overwhelming majority of all anti-gB antibodies against linear epitopes were specific for this region (Figure 1B). Interestingly, vaccination boosted preexisting anti-CTD responses to an extremely high level in 3 patients, dwarfing the responses observed to other ADs (Figure 1C and Supplementary Figure 1). The same 3 patients experienced posttransplantation HCMV viremia. In direct contrast, the remaining 3 patients had not developed these antibody responses subsequently following vaccination and had no evidence of posttransplantation viremia (Figure 1D).
Next, we sought to investigate how such potent response toward CTD in these 3 individuals correlated with production of antibodies toward other regions (Figure 2). Interestingly, we could see that high level of antibodies to AD2 and CTD are mutually exclusive. This could potentially suggest that high level of anti-CTD antibodies could hinder generation of anti-AD2 responses, a response that we and others have previously demonstrated to be correlated with protection (Figure 2B) [8]. Although having such a small number of individuals precludes definite conclusions, our results argue that future studies should further investigate this highly immunogenic, cytosolic region of gB and its relationship with other antigenic domains of gB.
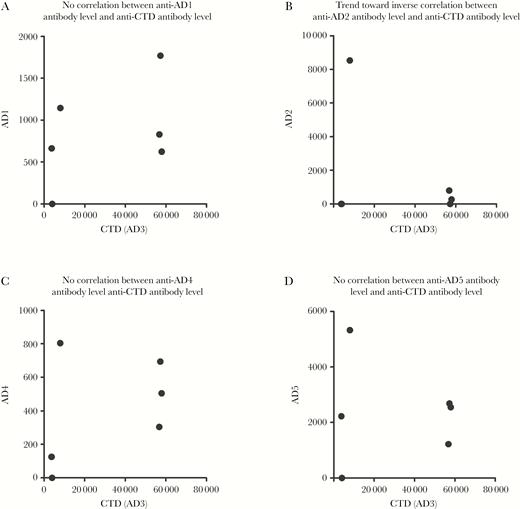
High level of antibodies to antigenic domain (AD) 2 and cytosolic terminal domain (CTD/AD3) are mutually exclusive. A–D, The highest immunoglobulin G response against AD1 (A), AD2 (B), AD4 (C), and AD5 (D) was plotted alongside the respective responses against CTD/AD3 (n = 6).
DISCUSSION
Based on this study of linear epitopes, our data suggest that vaccinating HCMV+ individuals with the gB/MF59 vaccine predominantly boosts preexisting antibody responses rather than inducing de novo responses. It is intriguing that, while CTD is highly immunogenic, responses to this region appear to inversely correlate with protection from viremia. One hypothesis is that inducing a humoral response against CTD HCMV diverts the immune response away from targets more likely to induce protective antibody responses (ie, AD2). A competition model is not unique in HCMV, whereby it is argued AD1 responses may interfere with protective AD2 responses—although in our patient cohort we did not observe a correlation between AD1 responses and the presence/absence of posttransplantation viremia [8]. Additionally, we cannot rule out that the reasons for differences in protection are related to differences in the responses to other important targets for neutralization (eg, complexes involving glycoproteins H and L).
An important implication of this study is that vaccination of HCMV+ individuals with gB/MF59 might simply boost the preexisting antibody responses and, furthermore, in some individuals these might be nonprotective. This concept is consistent with the paradigm of “original antigenic sin,” which describes the tendency of the immune system to preferentially utilize immunological memory originating from a previous antigen encounter. Thus, the original antigenic sin might be responsible for shaping the repertoire of immunological responses evoked by either vaccination or secondary exposure to different versions of the same pathogen (eg, a different strain, or a recombinant protein subunit). As a result, preexisting responses are boosted instead of vaccination promoting the development of novel protective responses that may occur in response to a newly encountered antigen. This phenomenon is well established with studies of influenza virus, dengue virus, and human immunodeficiency virus, and is considered to be a substantial obstacle to successful vaccine development [14]. In this report we show, for the first time, that this immunological phenomenon could also hamper the success of the HCMV gB/MF59 vaccine in certain individuals. This becomes prescient if we consider that a successful vaccine against this highly prevalent pathogen should not only protect against primary infection but also reinfection with a different strain of the virus as well as reactivation of latent infection [1, 15].
We believe that this observation—albeit based on small numbers—illustrates the complexity of developing a universal vaccine strategy against a persistent viral infection highly prevalent in the population. It also supports the hypothesis that deletion of specific regions of gB, or alternative strategies to present gB, may be important, particularly in individuals with prior exposure to HCMV.
Supplementary Data
Supplementary materials are available at The Journal of Infectious Diseases online. Consisting of data provided by the authors to benefit the reader, the posted materials are not copyedited and are the sole responsibility of the authors, so questions or comments should be addressed to the corresponding author.
Notes
Disclaimer. The funding sources had no role in the study design, data collection, data analysis, data interpretation, writing of the manuscript, or the decision to submit for publication.
Financial support. This study was supported by the Rosetrees and Stoneygate Trusts (grant number A1601) and the Royal Free Charity; M. B. R. was also supported by a Medical Research Council (MRC) Fellowship (award number G:0900466). The original clinical trial of gB/MF59 was supported by the US National Institute of Allergy and Infectious Diseases (grant number R01AI051355) and Sanofi Pasteur.
Potential conflicts of interest. F. K. and P. H. are employees of JPT Peptide Technologies GmbH. All other authors report no potential conflicts. All authors have submitted the ICMJE form for Disclosure of Potential Conflicts of Interest. Conflicts that the editors consider relevant to the content of the manuscript have been disclosed.