-
PDF
- Split View
-
Views
-
Cite
Cite
Gary Wong, Zirui Zhang, Shihua He, Marc-Antoine de La Vega, Kevin Tierney, Geoff Soule, Kaylie Tran, Lisa Fernando, Xiangguo Qiu, Marburg and Ravn Virus Infections Do Not Cause Observable Disease in Ferrets, The Journal of Infectious Diseases, Volume 218, Issue suppl_5, 15 December 2018, Pages S471–S474, https://doi.org/10.1093/infdis/jiy245
- Share Icon Share
Abstract
Ferrets are used for studying infections with wild-type Ebola virus isolates. Here, we investigated whether these animals are also susceptible to wild-type isolates of Marburg virus (MARV). Ferrets were challenged intramuscularly or intranasally with MARV strain Angola and monitored for 3 weeks. Unexpectedly, the animals neither showed observable signs of disease nor died of infection, and viremia was not detected after challenge. All animals were seropositive for MARV-specific immunoglobulin antibodies. Confirmatory studies with MARV strain Musoke and Ravn virus yielded the same outcomes. Therefore, ferrets may be of limited usefulness for studying the pathogenesis of MARV and Ravn virus infections.
The family Filoviridae comprises viruses that are filamentous in morphology and contain a negative-sense, single-stranded RNA genome approximately 19 kb long. Two of the most lethal pathogens known to humans are filoviruses: Ebola virus (EBOV) and Marburg virus (MARV). Along with their lower-profile relatives, including Sudan virus (SUDV), Bundibugyo virus (BDBV), Tai Forest virus, and Ravn virus (RAVV), these pathogens have been known since 1967 to cause outbreaks of severe hemorrhagic disease in humans. After an incubation of 2–21 days, patients report nonspecific signs of illness, such as fever, sore throat, headache, and muscle pains. The infection rapidly progresses, and more-advanced observations include chest pain, abdominal pain, vomiting, diarrhea, and, occasionally, a maculopapular rash. Hemorrhagic manifestations that can be observed in a portion of patients include vomiting or coughing up blood, as well as passing of blood in stool. Petechiae, purpura, ecchymoses, and hematomas may be present because of bleeding underneath the skin. Disseminated intravascular coagulopathy, shock, and multiorgan failure are often hallmarks of terminal disease. The case-fatality rate of EBOV or MARV infections can reach 90% if left untreated, and currently there are no vaccines or therapeutics licensed for use in humans.
Over the past 20 years, small-animal models (mainly mice and guinea pigs) have been developed for studying filovirus infections and screening promising antivirals. Since immunocompetent mice and guinea pigs are not susceptible to infection with wild-type isolates, a popular strategy is to adapt the virus to its host via sequential passages in the livers and spleens of the animals. This method has yielded mouse- and guinea pig–adapted EBOV [1, 2]; mouse-, guinea pig–, and Syrian hamster–adapted MARV [3–5]; mouse- and guinea pig–adapted RAVV [4, 6]; and guinea pig–adapted SUDV [7]. Infections with these viral variants cause uniformly lethal disease in their respective hosts and provide a stringent initial screening test for efficacy, which has helped accelerate the development of potentially promising medical countermeasures against filoviruses. However, small-animal models also have drawbacks in that the host-adapted viruses are not genetically identical to the clinical isolate from humans and that the observed disease in these animals does not always recapitulate all the hallmarks of human filoviral disease.
A breakthrough occurred during 2016, when 2 research groups reported the use of domestic ferrets (Mustela putorius furo) as a small-animal model to study EBOV, SUDV, and BDBV pathogenicity. The results showed that infection off ferrets with wild-type isolates from these 3 species was able to cause uniformly lethal disease with symptoms similar to those in humans. Common clinical signs included diarrhea, dehydration, labored breathing, hunched posture, and altered gait, as well as depression. Multiple organs, including the spleen, kidney, and liver, showed signs of damage from the infection. Additionally, rash and coagulation abnormalities were observed in these animals during advanced disease [8–10], mimicking 2 major characteristics of filoviral disease in humans. On the basis of these results, we decided to also test whether ferrets are also susceptible to MARV and RAVV infections.
METHODS
In the first experiment, 6-month-old male or female ferrets were obtained commercially (Marshall BioResources, NY) for these studies and were housed and cared for as previously described [9]. The animals were challenged intramuscularly (n = 6) or intranasally (n = 5) with 317 median tissue culture doses (TCID50) of MARV strain Angola (MARV/Ang; Marburg virus/H.sapiens-tc/AGO/2005/Angola-368; GenBank accession number KY047763.1). Survival and various clinical, hematological, biochemical, virological, and immunological parameters were monitored for 21 days after infection. Hematological and biochemical parameters were assessed on days 0, 3, 5, and 7 after infection as previously described [9]. Reverse transcription quantitative polymerase chain reaction (PCR) analysis was performed on blood RNA samples at days 0, 3, 5, and 7 after infection to assess for viremia, using previously described conditions and reagents [11]. Enzyme-linked immunosorbent analysis (ELISA) was performed on sera samples (diluted 1:100 and 1:1000) harvested on day 17 after infection, to test for the presence of MARV-specific immunoglobulin G (IgG) antibodies, following a previously established protocol but using γ-irradiated virions instead of His-tagged glycoprotein as capture antigen [12].
In the second experiment, naive ferrets (4 per group) were challenged intramuscularly with 630, 630, and 930 TCID50 MARV/Ang, MARV strain Musoke (MARV/Mus; Marburg virus/H.sapiens-tc/KEN/1980/Mt. Elgon-Musoke; GenBank accession number NC_001608.3), or RAVV (Ravn virus/H. sapiens-tc/KEN/1987/Ravn-CDC811103; GenBank accession number KU179482.1), respectively. The experiment was performed for the different viruses in a fashion similar to that described above.
RESULTS
The results of the first experiment showed that the animals did not die of infection, with no weight loss, fever, or other observable clinical symptoms (ie, decreased appetite or activity) during the study (Supplementary Figure 1A–D). Hematological parameters, including white blood cell, lymphocyte, and platelet counts and lymphocyte percentages, did not decrease substantially after infection. Neutrophil counts and percentages also did not increase (Supplementary Figure 2A–F). Biochemical parameters, including alanine aminotransferase and alkaline phosphatase levels and blood urea nitrogen, creatinine, and glucose concentrations, did not change substantially after infection (Supplementary Figure 2G–K). No animals were viremic (Supplementary Figure 3). ELISAs yielded ODs indicative of MARV seropositivity among animals in both the intramuscularly and intranasally challenged groups (Supplementary Figure 4A and 4B), despite the lack of observable disease.
To ensure that these results were reliable and to investigate whether this phenomenon was also true for other wild-type MARV or RAVV isolates, we performed a second study, in which new naive animals were challenged with MARV/Ang, MARV/Mus, or RAVV. All animals across the 3 experimental groups survived challenge (Figure 1A) without observable clinical signs of disease (Figure 1B–D). Hematological and biochemical parameters did not change substantially after infection (Supplementary Figure 5A–K). Viremia was absent in these animals 3, 5, 7, 10, and 14 days after infection (Supplementary Figure 6). All ferrets were seropositive for MARV- or RAVV-specific IgG 21 days after infection, as determined by ELISAs described above but using their respective γ-irradiated virions as capture antigens (Table 1).
Marburg Virus (MARV) Strain– and Ravn Virus (RAVV)–Specific Immunoglobulin G (IgG) Antibodies for Individual Ferrets 0 and 21 Days After Infection
Day, Ferret . | Reciprocal Dilution of IgG . | ||
---|---|---|---|
MARV/Ang . | MARV/Mus . | RAVV . | |
Day 0 | |||
Ferret 1 | <100 | <100 | <100 |
Ferret 2 | <100 | <100 | <100 |
Ferret 3 | <100 | <100 | <100 |
Ferret 4 | <100 | <100 | <100 |
Day 21 | |||
Ferret 1 | 800 | 6400 | 3200 |
Ferret 2 | 1600 | 6400 | 6400 |
Ferret 3 | 6400 | 6400 | 12800 |
Ferret 4 | 1600 | 6400 | 12800 |
Day, Ferret . | Reciprocal Dilution of IgG . | ||
---|---|---|---|
MARV/Ang . | MARV/Mus . | RAVV . | |
Day 0 | |||
Ferret 1 | <100 | <100 | <100 |
Ferret 2 | <100 | <100 | <100 |
Ferret 3 | <100 | <100 | <100 |
Ferret 4 | <100 | <100 | <100 |
Day 21 | |||
Ferret 1 | 800 | 6400 | 3200 |
Ferret 2 | 1600 | 6400 | 6400 |
Ferret 3 | 6400 | 6400 | 12800 |
Ferret 4 | 1600 | 6400 | 12800 |
Abbreviations: Ang, strain Angola; Mus, strain Musoke.
Marburg Virus (MARV) Strain– and Ravn Virus (RAVV)–Specific Immunoglobulin G (IgG) Antibodies for Individual Ferrets 0 and 21 Days After Infection
Day, Ferret . | Reciprocal Dilution of IgG . | ||
---|---|---|---|
MARV/Ang . | MARV/Mus . | RAVV . | |
Day 0 | |||
Ferret 1 | <100 | <100 | <100 |
Ferret 2 | <100 | <100 | <100 |
Ferret 3 | <100 | <100 | <100 |
Ferret 4 | <100 | <100 | <100 |
Day 21 | |||
Ferret 1 | 800 | 6400 | 3200 |
Ferret 2 | 1600 | 6400 | 6400 |
Ferret 3 | 6400 | 6400 | 12800 |
Ferret 4 | 1600 | 6400 | 12800 |
Day, Ferret . | Reciprocal Dilution of IgG . | ||
---|---|---|---|
MARV/Ang . | MARV/Mus . | RAVV . | |
Day 0 | |||
Ferret 1 | <100 | <100 | <100 |
Ferret 2 | <100 | <100 | <100 |
Ferret 3 | <100 | <100 | <100 |
Ferret 4 | <100 | <100 | <100 |
Day 21 | |||
Ferret 1 | 800 | 6400 | 3200 |
Ferret 2 | 1600 | 6400 | 6400 |
Ferret 3 | 6400 | 6400 | 12800 |
Ferret 4 | 1600 | 6400 | 12800 |
Abbreviations: Ang, strain Angola; Mus, strain Musoke.
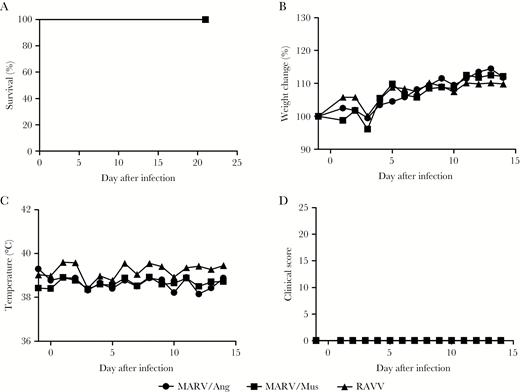
Clinical findings of ferrets infected with Marburg virus strain Angola (ARV/Ang), MARV strain Musoke (MARV/Mus), and Ravn virus (RAVV). Survival (A), weight loss (B), body temperature (C), and clinical scores (D) were monitored after infection. Where applicable, average values for animals in each challenge group are shown for clarity.
DISCUSSION
In this study, domestic ferrets were not susceptible to challenge with MARV/Ang, MARV/Mus, or RAVV under the experimental conditions listed above, unlike their susceptibility to challenge with EBOV, SUDV, or BDBV. It should be noted that experimental conditions for this study, including the challenge dose (at least 200 TCID50), challenge route (intramuscular and intranasal), sex (male and female), and age (6 months), were established to imitate conditions previously successful for evaluating EBOV and BDBV in ferrets [9].
Regrettably, PCR and immunohistochemical analyses were not performed on tissue specimens collected at the study end point to ensure that there was no virus present in tissues, and these study results must be interpreted with caution. First, the animals all received sufficiently high, presumably uniformly lethal doses of the virus, intramuscularly or intranasally. In another study, doses from 2 to 705 plaque-forming units of MARV/Ang administered via aerosol were high enough to kill nonhuman primates by as late as 9 days after infection [13]. In the current study, even though the animals could have experimentally received even higher challenge doses of MARV/Ang (up to 106 TCID50), the absence of clinical symptoms and other indications of disease in this study suggests that the challenge did not play a role in these results. Second, the animals all received live virus, since the challenge virus could be successfully back-titrated. Third, because the ferrets seroconverted, it can be presumed that the virus was able to enter host cells and stimulate an immune response, yet the lack of viremia suggests a localized infection (likely in the liver and spleen, the first major target organs for filoviruses) and no systemic spread. Fourth, all virus stocks were sequenced using Sanger and next-generation sequencing, and no mutations were detected.
The ability of EBOV to evade the type I interferon system and replicate to high viral titers is one of the reasons why this pathogen is so deadly [14]. In studies with mouse-adapted EBOV, it was found that mutations to the VP24 gene, whose function is to antagonize the type I interferon response, was responsible for the acquisition of high virulence in mice [15]. It is plausible that the wild-type, clinical isolates of MARV and RAVV were not able to avoid the ferret interferon response and, thus, were unable to cause disease. Unfortunately, commercial reagents to assess the innate and cell-mediated immune responses in ferrets are currently lacking to investigate this explanation. Alternatively, if a ferret-adapted MARV or RAVV was generated in the future, studies investigating the molecular determinants of virulence may yield further insight as to which genes (and thus which presumed functions) constituted to the “block” in pathogenesis. Until then, ferrets will constitute a great model to study the pathogenicity and, potentially, transmission of EBOV, SUDV, and BDBV but will be of limited use in similar studies involving MARV and RAVV.
Supplementary Data
Supplementary materials are available at The Journal of Infectious Diseases online. Consisting of data provided by the authors to benefit the reader, the posted materials are not copyedited and are the sole responsibility of the authors, so questions or comments should be addressed to the corresponding author.
Notes
Financial support. This work was supported by the National Key Research and Development Program of China (2016YFE0205800), the National Key Program for Infectious Disease of China (2016ZX10004222), the Public Health Agency of Canada, the National Institutes of Health (U19AI109762-1), the Canadian Institutes of Health Research (IER-143487), the Sanming Project of Medicine in Shenzhen (ZDSYS201504301534057), the Shenzhen Science and Technology Research and Development Project (JCYJ20160427151920801), and the National Natural Science Foundation of China International Cooperation and Exchange Program (816110193).
Potential conflicts of interest. All authors: No reported conflicts of interest. All authors have submitted the ICMJE Form for Disclosure of Potential Conflicts of Interest. Conflicts that the editors consider relevant to the content of the manuscript have been disclosed.