-
PDF
- Split View
-
Views
-
Cite
Cite
Estefanía Rodríguez, Wing Hang Ip, Viktoria Kolbe, Kristin Hartmann, Gundula Pilnitz-Stolze, Nilgün Tekin, Sergio Gómez-Medina, César Muñoz-Fontela, Susanne Krasemann, Thomas Dobner, Humanized Mice Reproduce Acute and Persistent Human Adenovirus Infection, The Journal of Infectious Diseases, Volume 215, Issue 1, 1 January 2017, Pages 70–79, https://doi.org/10.1093/infdis/jiw499
- Share Icon Share
Severe human adenovirus (HAdV) infections are an increasing threat for immunosuppressed individuals, particularly those who have received stem cell transplants. It has been previously hypothesized that severe infections might be due to reactivation of a persistent infection, but this hypothesis has been difficult to test owing to the lack of a permissive in vivo model of HAdV infection. Here we established a humanized mouse model that reproduces features of acute and persistent HAdV infection. In this model, acute infection correlated with high mortality, weight loss, liver pathology, and expression of viral proteins in several organs. In contrast, persistent infection was asymptomatic and led to establishment of HAdV-specific adaptive immunity and expression of early viral genes exclusively in the bone marrow. These findings validate the use of humanized mice to study acute and persistent HAdV infection and strongly suggest the presence of cellular reservoirs in the bone marrow.
Human adenoviruses (HAdVs) cause asymptomatic-to-mild respiratory infections in children and young adults [1]. Some species also cause infections of the gastrointestinal tract and the conjunctiva. However, in immunocompromised patients, HAdV may cause severe symptoms, such as hepatitis, nephritis, meningoencephalitis, and pneumonia. This is especially problematic in patients undergoing transplantation [2, 3]. In these patients, it is thought that HAdV infections arise from the reactivation of a persistent/latent infection in the donor bone marrow and/or in the recipient [4]. However, this hypothesis has not been experimentally addressed. Presently, no specific treatments exist against severe HAdV infections, which may have mortality rates of up to 70%, especially in pediatric patients undergoing stem cell transplantation [5].
Several studies have found HAdV DNA, mainly of species C origin, in tonsils, adenoids, and mucosal lymphocytes [6–9] long after recovery from infection. HAdV DNA has also been detected in tumors [10–12], mainly within tumor-infiltrated lymphoid cells [11]. In addition, persistent HAdV infections have been established in blood-derived cell lines [13–16]. These data point to hematopoietic cells as a putative reservoir for HAdV. To date, the mechanisms that control persistent infection in vivo and the specific cell reservoir remain unknown. This is in part due to the lack of small-animal models to study HAdV persistence in vivo. Animal models have been difficult to establish because of the species-specificity of adenoviruses. HAdV can only infect human cells, and classical mouse models are not permissive for HAdV infection.
In recent years, the establishment of humanized mice harboring human hematopoietic cells has rapidly developed, and such models have proven to be useful for the study of several human diseases. However, only a few studies have utilized humanized mice to investigate human viral infections [17–21], including Epstein-Barr virus, dengue virus, human immunodeficiency virus (HIV), and, very recently, Ebola virus [22–26]. Owing to the species-specificity of HAdVs and the long-lasting human engraftment in these mice [27], we reasoned that they may constitute a potential model for studying the pathogenesis of acute and persistent HAdV infection [22].
Here we present a humanized mouse model for HAdV that can reproduce both acute/severe and persistent/latent HAdV infections. We have observed that 34% of HAdV-infected humanized NSG (huNSG) mice developed liver pathology leading to viral hepatitis and HAdV disease dissemination, consistent with acute HAdV infection. Conversely, 66% of the animals remained asymptomatic and developed antigen-specific T-cell responses. These mice harbored HAdV persistent infection in bone marrow and peripheral blood cells. Our model constitutes a novel in vivo system to study the pathophysiology of HAdV infections in a human context and may be a useful tool to investigate mechanisms of persistence and virus reactivation.
METHODS
Generation of Humanized Mice and Detection of Human Engraftment
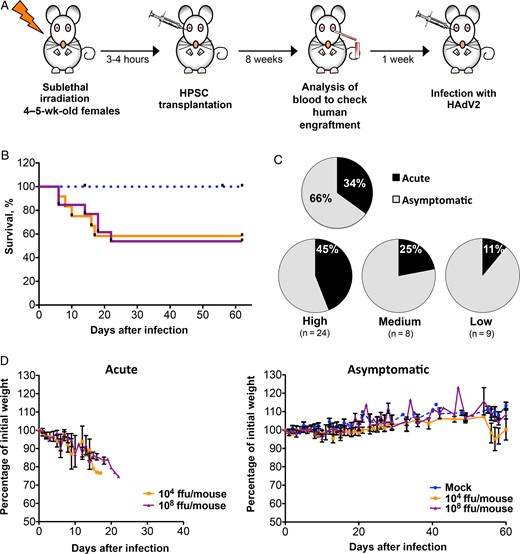
Morbidity and mortality due to human adenovirus (HAdV) in humanized NSG-A2 mice. A, Diagram showing the protocol followed to generate the NSG-A2 humanized mice. B, Kaplan–Meier survival curve of mice with high human chimerism (defined as mice in which >30% of peripheral blood cells were hCD45+ cells). Mice were infected with either 104 or 108 fluorescence-forming units (ffu)/mouse or were mock infected (with phosphate-buffered saline). C, The percentage of mice with acute (black) or asymptomatic (gray) infection. The upper chart shows the total disease distribution. The lower charts show the disease distribution by human engraftment in peripheral blood, with high engraftments defined as a peripheral blood cell population in which >30% of cells were hCD45+, medium engraftment defined as one in which 30%–15% were hCD45+, and low engraftment defined as one in which <15% were hCD45+). Disease distribution was calculated as the percentage of mice that developed an acute or asymptomatic infection per group. The total number of animals per group is shown in parentheses. D, Graph showing weight loss of acute and asymptomatic mice with high human engraftment, depicted as the percentage of initial weight. Mean values and standard errors of the mean are represented. A total of 30 mice were used for this analysis (6 were mock infected, 11 were infected with 104 ffu/mouse, and 13 were infected with 108 ffu/mouse).
Eight weeks after HSPC transplantation, blood samples were collected from animals, and human chimerism was quantified by flow cytometry after red blood cell depletion (RBC Lysis Buffer; Biolegend) and staining with anti-human CD45 antibody (clone HI30; Biolegend). Samples were analyzed in a FACS Canto II flow cytometer (BD Bioscience). For data analysis, animals were classified into 3 groups on the basis of the level of human engraftment, with the high-engraftment group comprising animals in which >30% of peripheral blood cells were human hematopoietic cells (hCD45+), the medium-engraftment group comprising those in which the hCD45+ cell proportion was 15%–30%, and the low-engraftment group comprising those in which the hCD45+ cell proportion was <15%.
Virus Production, Infections, and Disease Monitoring
Wild-type HAdV serotype 2 (HAdV2) from species C (ATCC) was produced in either A549 or HEK293 cells and was purified with CsCl. Amicon Ultra columns (Ultracel-100K, Millipore) were used for buffer exchange (with phosphate-buffered saline [PBS]) and to obtain a high concentrated stock. Virus stocks were titered in the same cell lines by immunofluorescence against the E2A protein as previously described [28].
On week 9 after transplantation, mice were intravenously infected with 104 or 108 fluorescence-forming units per mouse of HAdV2 or were mock infected (with 1× PBS intravenously). Weight loss and signs of disease (lethargy and ruffled fur) were monitored daily. Animals that lost >25% of their initial body weight were euthanized. Asymptomatic mice were euthanized 2 months after infection. At the time of death, different organs/tissues were harvested and either snap frozen in liquid nitrogen and stored at −80°C or fixed for histologic preparation.
RNA Extraction and Reverse-Transcription Polymerase Chain Reaction (RT-PCR)
TRIzol (ThermoFisher Scientific)/chloroform extraction was used to obtained RNA from frozen tissue, followed by DNase treatment (Qiagen) and purification using the RNeasy mini kit (Qiagen). RNA extraction of peripheral blood mononuclear cells and bone marrow cells was done by cell lysis in RLTplus buffer (Qiagen) and using the RNeasy Mini kit (Qiagen). One microgram of total RNA was reversed transcribed by using the Reverse Transcription System and random primers (Promega). PCR was performed in the RotorGene Q cycler (Qiagen), using the SensiMix SYBR-Hi-ROX (Bioline). All values were normalized to GAPDH levels. Samples were run in duplicates. Primers for genes encoding E1A, hexon, and GAPDH have been previously described [11].
Histopathologic and Immunohistochemical (IHC) Analyses
Organs were fixed in formalin (ProTaqs 4% formaldehyde; Quartett) and then embedded in paraffin. Bones were first immersed in Calfix solution (Protaqs, Quartett) and decalcified (Protaqs Calless, Quartett). Sections were cut (thickness, 4 µm) and stained with hematoxylin-eosin. For analysis of fibrosis, samples were stained with Picro Sirius Red solution (Sirius red dye in picric acid) according to standard procedures. For the detection of specific proteins, antigen retrieval was performed by boiling deparaffinized sections for 30–60 minutes in 10 mM citrate buffer (pH 6.0). For monocyte/macrophage detection, samples were incubated with Iba1 antibody (1:1500; Wako Chemicals). To stain proliferating cells, samples were incubated with Ki67 antibody (dilution, 1:1000; Sigma Aldrich). The following antibodies were used to stain adenoviral proteins: E1A monoclonal antibody (M73; dilution, 1:3) [29], E2A-72K monoclonal antibody (B6-8; dilution, 1:3) [30], and HAdV5 RbAb (L133; dilution, 1:400) [31]. For detection of specific binding, the Ultra View Universal DAB Detection Kit (Ventana, Roche) was used.
Analysis of HAdV-Specific T-Cell Responses
Splenocytes were resuspended in Roswell Park Memorial Institute medium containing 25 µg of HAdV2 (inactivated at 60°C for 30 minutes), 1 µg of purified mouse anti-human CD28 (BD Bioscience), brefeldin A (dilution, 1:1000; BD GolgiPlug; BD Bioscience), and Fc receptor–blocking solution (dilution, 1:100; Human TruStain FcX; Biolegend). A positive control for nonspecific T-cell activation was included by resuspending an aliquot of splenocytes in Roswell Park Memorial Institute medium, 1:1000 BD GolgiPlug, 1:100 Human TruStain FcX, 20 ng/mL PMA, and 1 µg/mL ionomycin. All samples were incubated overnight at 37°C. Cells were stained on the following day with different antibodies to detect interferon γ (IFN-γ) production in CD8+ T cells by flow cytometry (FACS Canto II, BD Bioscience). Data were analyzed with FlowJo software (TreeStar).
Enzyme-Linked Immunosorbent Assay (ELISA) to Detect HAdV-Specific Antibodies
ELISA plates were coated with heat-inactivated HAdV2 particles overnight, as described elsewhere [32]. On the next day, plates were blocked with 20% fetal bovine serum (FBS) and then incubated with each serum (1:20 dilution in PBS plus 10% FBS) for 2 hours at room temperature. Plates were washed with PBS-Tween 0.05% and incubated for 1 hour at room temperature with 1:1000 dilution of mouse anti-human immunoglobulin M (IgM)–horseradish peroxidase (Southern Biotech). HAdV-specific antibodies were detected by incubation with tetramethylbenzidine substrate (Biolegend) and absorbance reading at 450 nm. For background measurement, absorbance at 570 nm was subtracted from the value at 450 nm. Measurements were done in duplicate. The assay was validated by a standard curve of serial dilutions of HAdV-positive serum. A titration curve for the coating antigen was performed; the detection limit was estimated in 1.12 ng/µL of inactivated antigen. Plates were coated with 700 ng/µL of inactivated antigen. The threshold to consider samples positive was calculated as the average of mock values plus 1 SD.
Statistical Analysis
For statistical analysis, the Kruskal–Wallis Test was used, followed by the Dunn multiple comparison post hoc test. Analysis was done in Prism 5 (GraphPad).
RESULTS
HAdV Causes Disease in huNSG Mice
To generate humanized mice, we used NSG transgenic mice expressing human HLA-A2.1 (NSG-A2), in which the immune system was reconstituted with cord blood–isolated HSPCs (Figure 1A and Supplementary Figures 1 and 2). Nine weeks after transplantation, animals were infected with 104 or 108 fluorescence-forming units of HAdV2 per mouse. We first analyzed the overall morbidity and mortality by measuring survival, changes in body weight, and disease development. Thirty-four percent of infected mice (14 of 41) developed signs of disease, such as lethargy, ruffled fur, and progressive weight loss, and died of infection between day 6 and day 26 after infection, while 66% (27 of 41) remained asymptomatic (Figure 1C). We reasoned that these differences could be due to variations in the degree of human chimerism. In agreement with this hypothesis, we observed that highly engrafted mice had significant weight loss and decreased survival when infected with either dose of HAdV2 (Figure 1B and 1D). Highly engrafted mock-infected mice (Figure 1B and 1D), as well as nonhumanized mice (Supplementary Figure 3), remained asymptomatic, indicating that morbidity was associated with HAdV infection and not with hematopoietic chimerism. HAdV-infected mice with medium and those with low engraftment did not show significant weight loss or decreased survival as compared to mock animals (Supplementary Figure 4). These results strongly suggest that the ability of HAdV to cause disease in our model depends on the availability of target human cells, strengthening the idea that our system mimics in vivo HAdV infection in a human context.
Acutely Infected Mice Developed Viral Hepatitis due to HAdV2 Replication
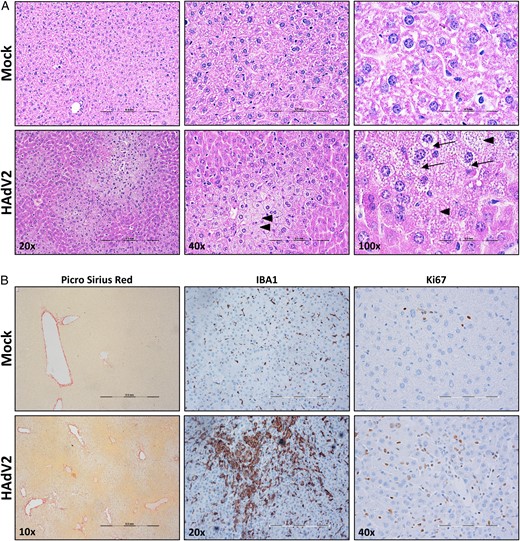
Liver pathology analysis in mice with acute human adenovirus (HAdV) infection. Representative micrographs of liver sections from HAdV2-infected and mock-infected humanized NSG-A2 mice, stained with hematoxylin-eosin (A) and Picro Sirius Red (B), which was used to stain collagen in damaged livers, highlighting fibrotic tissue; IBA1 antibody, which was use to stain monocytes/macrophages; and Ki67 antibody, which was used to mark proliferating cells. Arrowheads indicate cells lacking a nucleus. Arrows highlight vacuolization in hepatocytes. Scale bar represents 0.5 µm in all magnifications.
Adenovirus Gene and Protein Expression Differentiates Acute From Persistent Infection
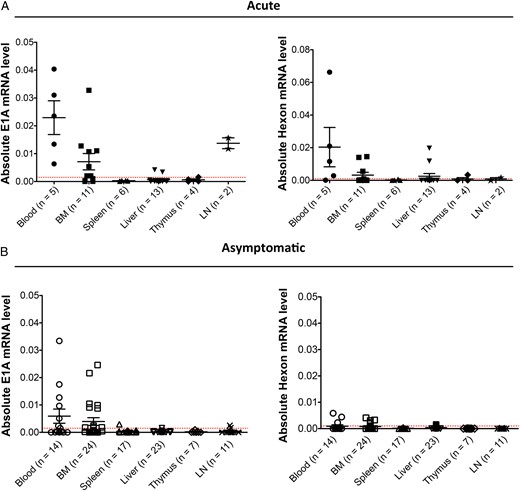
Detection of human adenovirus (HAdV) messenger RNA (mRNA) in different tissues. Absolute mRNA levels for E1A and hexon expression are shown. A, Graph showing E1A (left panel) and hexon (right panel) expression in mice with acute humanized NSG-A2 infection. B, Graph displays values for the same genes in asymptomatic infected animals. Each dot represents one animal, mean values and standard errors of the mean are shown for each tissue. The number of samples per group is shown in parentheses. The gene encoding GAPDH was used as housekeeping gene to normalize the data. The red dotted line is the threshold for a sample to be positive, and it is calculated on the basis of the mRNA levels for each gene in the mock-infected animals. Abbreviations: BM, bone marrow; LN, lymph node.
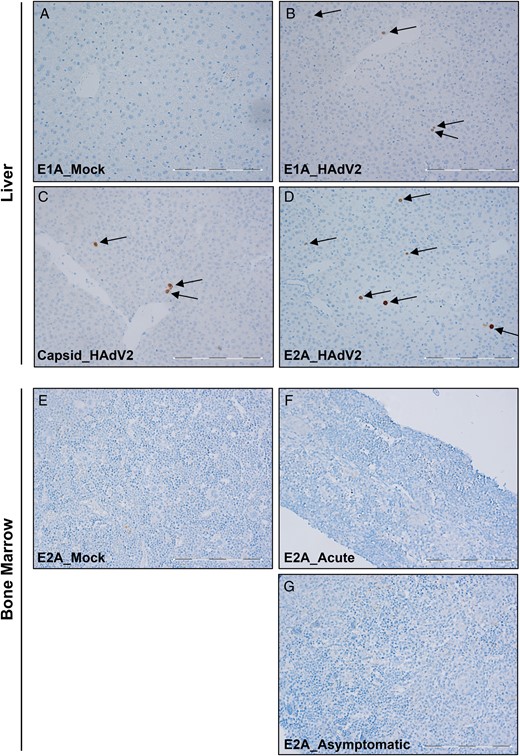
Detection of human adenovirus (HAdV) proteins in liver and bone marrow. E1A, E2A, and capsid proteins in formalin-fixed, paraffin-embedded tissue samples underwent immunohistochemical analysis. A–D, Representative micrographs of liver sections from mice with acute infection, showing results of E1A staining (A and B), capsid staining (C), and E2A staining (D). E–G, Representative micrographs of bone marrow sections stained with E2A antibody, showing results for mock-infected mice (E), mice with acute infection (F), and mice with asymptomatic infection (G). Arrows point to cells that stained positive for each antibody. The scale bar is 0.5 µm. All pictures were taken at 20 times the original magnification.
To investigate whether HAdV gene expression could still be found in asymptomatic-infected mice 2 months after infection, we performed RT-PCR to detect E1A and hexon expression in the same tissues. In these animals, only E1A expression but low or no hexon expression could be detected in the bone marrow and peripheral blood (Figure 3B). Similar to what we have observed in the bone marrow of acutely infected mice, no viral proteins were detected by IHC in the bone marrow of HAdV-infected asymptomatic animals (Figure 4G). These results indicate that HAdV might be causing a persistent infection in the bone marrow of HAdV humanized mice with acute or asymptomatic infection.
Adaptive Immune Responses Mark Acute Versus Persistent Infection
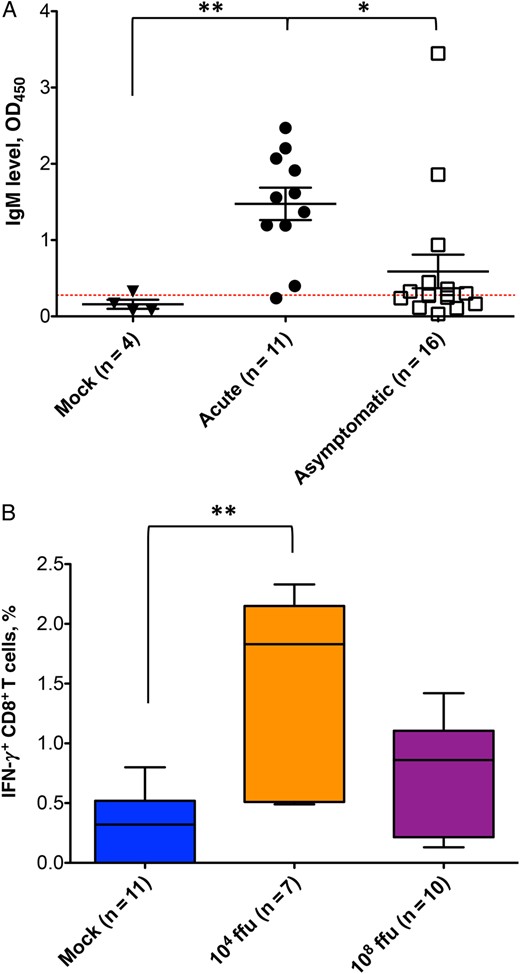
Adaptive immune response to human adenovirus (HAdV) infection in humanized NSG-A2 mice. A, Immunoglobulin M (IgM) levels measured by enzyme-linked immunosorbent assay (ELISA) in the serum of mock-infected mice and those with acute or asymptomatic infection are shown. The red dotted line indicates the threshold for a positive sample and is calculated as the average of the mock values plus 1 standard deviation. The Kruskal–Wallis test was performed for statistical analysis (P = .0021), followed by a Dunn multiple comparison test. *P < .05 for comparisons of the acute infection group to the asymptomatic infection group; **P < .005 for comparison of the mock infection group to the acute infection group. B, The graph shows the percentage of interferon γ (IFN-γ)–expressing CD8+ T cells after specific restimulation of T-cell splenocytes. The Kruskal–Wallis test was performed for statistical analysis (P = .0024), followed by a Dunn multiple comparison test. **P < .005 for comparison of the mock infection group to the group infected with 104 fluorescence-forming units of HAdV. The number of samples per group is shown in parentheses.
DISCUSSION
Adenoviruses cause severe disease in immunocompromised patients, especially in pediatric patients undergoing allogeneic stem cell transplantation [35–38]. These patients develop disseminated HAdV disease between 2 and 3 months after transplantation, with the highest incidence ranging from 5% to 47% within the first 100 days after transplantation and with higher mortality rates than infections in adults [5, 34]. Severe HAdV infections in stem cell transplant recipients cause severe manifestations, such as hemorrhagic cystitis, pneumonia, nephritis, encephalitis, myocarditis, and fulminant hepatitis [34, 39]. It is thought that these infections arise from reactivation of latent/persistent HAdV infection, rather than reinfection [38]. Once established, severe HAdV infections are very difficult to cure, and broad-spectrum antiviral drugs are not efficient in most cases, leading to multiorgan failure and death. Owing to the species specificity of adenoviruses, no previous studies have established a permissive mouse model system to study severe HAdV infection, pathology, and treatment. We are the first to present a humanized mouse model, huNSG-A2, that is susceptible to HAdV and shows signs of lytic and persistent infections.
In our study, we have observed that 34% of HAdV-infected humanized mice developed acute/permissive infection. This was correlated with significant weight loss and decreased survival, as well as the onset of liver pathology, including increased hepatocyte vacuolization. These observations resemble the liver phenotype reported in immunosuppressed patients who developed HAdV-induced hepatitis [40]. Similar phenotypes have been observed in studies that tested the biodistribution of replication-deficient adenoviral vectors or the oncolytic effects of replication-competent, cell-targeted vectors, but these studies used viral loads that were 50–1000 times the viral loads we used in the present study [33, 41, 42]. In our model, we used relatively low amounts of a wild-type virus (HAdV type 2), and, in addition to previous observations, we found that humanized mice with acute infection developed liver fibrosis, increased monocyte/macrophage tissue infiltration and activation, and increased cellular proliferation. Moreover, we have observed systemic virus dissemination in 2 mice from which HAdV type 2 could be isolated.
It has been shown by Engler et al that the acute hepatotoxicity observed during HAdV infection is associated with E1A expression, which induces cytokine production (ie, tumor necrosis factor α), causing severe liver inflammation and tissue damage [33]. In line with this, we have observed that huNSG mice with acute infection expressed high levels of E1A in different tissues (liver, bone marrow, lymph nodes, and peripheral blood). Some of these body sites were also positive for transcripts of the late gene hexon, which encodes a major capsid protein. Moreover, we found cells positive for E1A, E2A, and capsid proteins in liver sections from the same animals. These results suggest that, at least in part, E1A expression could be responsible for the development of HAdV-induced hepatitis. Even though previous studies have suggested that HAdV may produce abortive infections in mouse cells [43–45], our results show an active/lytic replication of HAdV in huNSG mice. This is supported by the detection of viral proteins in liver tissue and the rescue of viral particles ex vivo, together with increased hCD45+ cell infiltration in the liver of mice with acute infection (unpublished observation). Whether virus replication occurred in hepatocytes or in tissue-infiltrated human blood cells in our specific experimental setup remains unknown and is the aim of current investigations. Interestingly, the lymph nodes of mice with acute infection that were positive for E1A expression did not show any hexon expression, which would suggest that an abortive infection occurred in this lymphoid tissue.
HAdV-positive samples have been found in tonsil and adenoids [6, 8], lymphocytes of the human mucosa, and the gastrointestinal tract long after an acute or asymptomatic infection [7, 9, 46]. In most cases, the presence of HAdV-positive samples was analyzed by detection of E1A and/or hexon DNA sequences. Moreover, some groups could isolate infectious virus from human specimens [6], while others could not [7], suggesting different states of latent/persistent/chronic infection in human subjects. In the last years, several authors have established in vitro systems with blood-derived cell lines to study HAdV persistent infections [13, 16]; however, it remains unclear whether viral gene expression and virus progeny production are characteristics of persistent HAdV infections in vivo.
In our study, the majority (66%) of HAdV-infected humanized mice were asymptomatic, as they did not show any signs of disease or weight loss. During both macroscopic and microscopic analyses, the organs of these animals showed a phenotype similar to that of noninfected (ie, mock-infected) animals. Interestingly, we found few HAdV genome copies in the bone marrow of asymptomatic infected animals, accompanied by high levels of E1A mRNA, 2 months after infection; however, very low or no hexon expression was detected. Our results are in agreement with those of previous studies using cell lines persistently infected with HAdV [13, 16]. Besides, some peripheral blood samples were also positive for E1A mRNA. Although this is a rare event in healthy humans, some studies have found HAdV-positive samples in human peripheral blood donors [47]. Similarly, hepatitis B virus has also been observed to cause persistent infections in peripheral blood lymphocytes and in bone marrow cells, showing also a limited expression of its viral genes [4]. Interestingly, viral proteins could not be detected in the bone marrow of HAdV-infected huNSG mice with acute or asymptomatic infection. These data suggest that within all hematopoietic tissues with human engraftment in our model, a persistent HAdV infection has been established in the bone marrow of these animals. Furthermore, our results suggest that, during persistent infections in vivo, only early viral mRNAs are transcribed, but no viral proteins are produced. This might serve as a mechanism for the virus to evade the immune system and persist. Further experiments to identify the specific cell subpopulation harboring persistent HAdV infection are warranted.
In summary, the differences in viral gene and protein expression in different mouse organs may serve as a tool to differentiate between active/lytic infection, when both early and late genes and proteins are expressed, or persistent/latent infection, when only early but not late genes are expressed or proteins are produced. Accordingly, the immune response to HAdV infection in our model could distinguish between mice with acute and those with persistent infection. Humanized mice that died from the disease showed a significant increase in IgM production as compared to asymptomatic mice. Although this could be due to differences in the timing of sample acquisition, our data suggest that an acute infection was taking place in these animals. On the other hand, and as previously shown for a recombinant HAdV type 5 [48], asymptomatic mice developed HAdV antigen–specific T-cell responses, which surprisingly were significantly higher in mice that were infected using a lower virus load. These could be due to the inhibition of T-cell function caused by excess of antigen (T-cell exhaustion) when using a high viral dose, which is likely to happen because of the HLA-A2–restricted oligoclonal T-cell responses in this model [23, 49, 50]. Further experiments are needed to understand HAdV immune regulation in these animals.
Our humanized mouse model of HAdV infection opens up new opportunities to study HAdV pathology and immune response in vivo. It will permit the investigation of new mechanisms controlling virus persistence and reactivation, and it will contribute to our understanding of the origin of HAdV infections in immunosuppressed patients. Ultimately, our mouse model could be effectively used for the development and validation of new specific antiviral strategies against HAdV infection.
Supplementary Data
Supplementary materials are available at http://jid.oxfordjournals.org. Consisting of data provided by the author to benefit the reader, the posted materials are not copyedited and are the sole responsibility of the author, so questions or comments should be addressed to the author.
Notes
Acknowledgments. We thank the animal caretakers from the HPI animal facility, especially Ursula Müller and Ines Jambor, for their daily support of the mouse work; Dr Carol Stoking, for careful revision of the manuscript; and the cord blood donors, nurses, and doctors from the Asklepios Klinik Nord (Hamburg, Germany) who were involved in this project.
Financial support. This work was supported by the Deutsche Forschungsgemeinschaft (grant DO 343/7-1 to T. D.), the Wilhelm Sander-Stiftung (to T. D.), the Freie und Hansestadt Hamburg (to the Heinrich Pette Institute, Leibniz Institute for Experimental Virology), and the Bundesministerium für Gesundheit (to the Heinrich Pette Institute, Leibniz Institute for Experimental Virology).
Potential conflicts of interest. All authors: No reported conflicts. All authors have submitted the ICMJE Form for Disclosure of Potential Conflicts of Interest. Conflicts that the editors consider relevant to the content of the manuscript have been disclosed.
References
Author notes
Presented in part: 3rd Meeting on Emerging Oncogenic Viruses, San Pietro in Bevagna, Manduria, Italy, 4–8 June 2014; 11th International Adenovirus Meeting, La Jolla, California, 16–20 July 2014; ICGEB DNA Tumor Virus Meeting, Trieste, Italy, 21–26 July 2015; International Workshop on Humanized Mice 5, Zurich, Switzerland, 28–30 January 2016; 12th International Adenovirus Meeting, Barsinghausen, Germany, 16–20 August 2016.