-
PDF
- Split View
-
Views
-
Cite
Cite
Prasanna Jagannathan, Katherine Bowen, Felistas Nankya, Tara I. McIntyre, Ann Auma, Samuel Wamala, Esther Sikyomu, Kate Naluwu, Mayimuna Nalubega, Michelle J. Boyle, Lila A. Farrington, Victor Bigira, James Kapisi, Fran Aweeka, Bryan Greenhouse, Moses Kamya, Grant Dorsey, Margaret E. Feeney, Effective Antimalarial Chemoprevention in Childhood Enhances the Quality of CD4+ T Cells and Limits Their Production of Immunoregulatory Interleukin 10, The Journal of Infectious Diseases, Volume 214, Issue 2, 15 July 2016, Pages 329–338, https://doi.org/10.1093/infdis/jiw147
- Share Icon Share
Abstract
Background. Experimental inoculation of viable Plasmodium falciparum sporozoites administered with chemoprevention targeting blood-stage parasites results in protective immunity. It is unclear whether chemoprevention similarly enhances immunity following natural exposure to malaria.
Methods. We assessed P. falciparum–specific T-cell responses among Ugandan children who were randomly assigned to receive monthly dihydroartemisinin-piperaquine (DP; n = 87) or no chemoprevention (n = 90) from 6 to 24 months of age, with pharmacologic assessments for adherence, and then clinically followed for an additional year.
Results. During the intervention, monthly DP reduced malaria episodes by 55% overall (P < .001) and by 97% among children who were highly adherent to DP (P < .001). In the year after the cessation of chemoprevention, children who were highly adherent to DP had a 55% reduction in malaria incidence as compared to children given no chemoprevention (P = .004). Children randomly assigned to receive DP had higher frequencies of blood-stage specific CD4+ T cells coproducing interleukin-2 and tumor necrosis factor α (P = .003), which were associated with protection from subsequent clinical malaria and parasitemia, and fewer blood-stage specific CD4+ T cells coproducing interleukin-10 and interferon γ (P = .001), which were associated with increased risk of malaria.
Conclusions. In this setting, effective antimalarial chemoprevention fostered the development of CD4+ T cells that coproduced interleukin 2 and tumor necrosis factor α and were associated with prospective protection, while limiting CD4+ T-cell production of the immunoregulatory cytokine IL-10.
Protective immunity to malaria has been achieved using nonconventional vaccination regimens that permit Plasmodium falciparum sporozoites to infect humans and establish liver infection but block progression to the clinically symptomatic blood stage of infection. These successful approaches include vaccination with irradiated [1, 2] or genetically attenuated sporozoites [3], as well as infection with unaltered sporozoites combined with administration of antimalarial drugs that eradicate blood-stage parasites but allow the pre-erythrocytic parasite stages to develop [4–9]. This latter approach, termed “chemoprophylaxis and sporozoites (CPS)”, has resulted in sterilizing immunity to malaria in previously naive adults that is dose dependent [10] and persists for at least 2 years [4, 5].
The mechanisms by which CPS enhances the development of antimalarial immunity are not clear. One possibility is that selective blockade of blood-stage parasite infection enhances exposure to pre–erythrocytic stage parasite antigens, leading to a more robust immune response against those stages [5, 6, 11]. Alternatively, CPS may enhance protection against blood-stage parasites, as recently shown in a Plasmodium chaubadi model of CPS [9]. In humans, CPS has been shown to induce P. falciparum blood-stage specific, multifunctional CD4+ T cells producing interleukin 2 (IL-2), tumor necrosis factor α (TNF-α), and interferon γ (IFN-γ), which may be associated with protection [4]. Moreover, selective blockade of blood-stage parasite infection may prevent the development of parasite-induced immunoregulatory mechanisms. For instance, the regulatory cytokine interleukin 10 (IL-10) is upregulated in P. falciparum infection and protects against immunopathology from a variety of parasitic infections, but it may also interfere with the effectiveness and durability of immune responses [12–15]. Suppression of such immunoregulatory pathways may enhance the development of antimalarial immunity through the generation of more-effective T-cell responses.
There is conflicting evidence on the impact of chemoprevention on the development of antimalarial immunity in childhood and the risk of malaria after chemoprevention has stopped. Studies from Tanzania and Gambia reported that children receiving highly effective chemoprophylaxis had a higher incidence of malaria (known as “rebound malaria”) than those receiving placebo in the year following the intervention [16, 17]. In contrast, more-recent studies have reported no association between intermittent preventive therapy with sulfadoxine-pyrimethamine and the risk of malaria following the intervention [18, 19], and one study reported a sustained decrease in the risk of malaria following the intervention [20]. Differences in these findings could be due to differences in transmission intensity, ages of study subjects, drug efficacy and mechanisms, and dosing strategies (continuous prophylaxis versus intermittent therapy).
In this study, we evaluated the impact of continuous artemisinin-based chemoprevention on antimalarial immunity when given to children naturally exposed to malaria in a high-endemicity setting. We hypothesized that selective suppression of blood-stage P. falciparum infection by chemoprevention may enhance naturally acquired immunity by limiting parasite-induced immunoregulatory mechanisms and minimizing the deleterious effects of chronic antigenemia.
METHODS
Study Participants and Design
Samples were obtained from infants enrolled in a randomized, controlled, open-label trial comparing the efficacy and safety of 3 regimens versus no therapy for the prevention of malaria in Tororo, a district in eastern Uganda with year-round malaria transmission and an entomological inoculation rate estimated at 310 infectious bites per year [21]. Details of this trial have been described elsewhere [22] (Supplementary Data), and written informed consent was obtained from the parent or guardian of all study participants. The substudy described in this report includes only infants randomly assigned to receive dihydroartemisinin-piperaquine (DP) monthly (n = 87) or no chemoprevention (n = 90) who reached at least 24 months of age and had a blood sample collected at that age. Study drugs were administered at home without supervision. Chemoprevention was given from 6 months through 24 months of age, and participants were followed for 1 additional year until they reached 36 months of age. Monthly assessments were done to ensure compliance with study protocols and perform routine blood smears. Children who presented with a fever (tympanic temperature, ≥38.0°C) or a history of fever in the previous 24 hours had a blood sample obtained by finger prick for a thick smear. If positive for malaria parasites, the patient received a diagnosis of malaria and was given artemether-lumefantrine. Approximately 6–10 mL of blood was collected at age 24 months. Peripheral blood mononuclear cells (PBMCs) were isolated by density gradient centrifugation (Ficoll-Histopaque; GE Life Sciences) and cryopreserved.
Piperaquine Levels and Adherence Scores
Piperaquine drug levels in plasma were measured at 4–6 random time points during the intervention in subjects randomly assigned to receive DP. Piperaquine was measure by liquid chromatography/tandem mass spectroscopy and demonstrated a lower limit of quantitation of 10 ng/mL with a calibration range of 10–100 ng/mL [23]. Adherence scores were calculated on the basis of pharmacokinetic models incorporating age, weight, and days since the last piperaquine dosing [24]. Subjects were characterized into 2 groups of adherence, based on adherence scores, as follows: low, 0 to <2; and high, ≥2.
Malaria Parasite Antigens and Intracellular Cytokine Staining
P. falciparum blood-stage 3D7 parasites were grown by standard methods (mycoplasma free). Red blood cells (RBCs) infected with mature asexual-stage parasites (iRBCs) were purified magnetically and cryopreserved in glycerolyte before use [15]. Uninfected RBCs (uRBCs) were used as controls. Density-purified [25], cryopreserved P. falciparum NF54 sporozoites were obtained from the Seattle Biomedical Research Institute/Center for Infectious Diseases Research (Steffan Kappe [principal investigator], Seattle, Washington) and maintained at 1.2 × 106 sporozoites/vial in liquid nitrogen before use. On the day of assay, sporozoites were thawed at room temperature and counted by hemocytometer. A medium condition was used as a control.
Thawed PBMCs were rested overnight in 10% fetal bovine serum (Gibco) and stimulated with uRBCs, iRBCs, sporozoites, medium, or phytohemagglutinin at 1 × 106 cells/condition with anti-CD28 and anti-CD49d (0.5 µg/mL; BD Pharmingen). An effector to target cell ratio of 2:1 was used with uRBCs and iRBCs, and a ratio of 20:1 for sporozoites (Supplementary Data). Brefeldin A and monensin (BD Pharmingen) were added at 6 hours (10 µg/mL). At 24 hours of incubation, cells were washed, fixed, and permeabilized, and surface and intracellular staining was performed with standard protocols (Supplementary Data).
Statistical Methods
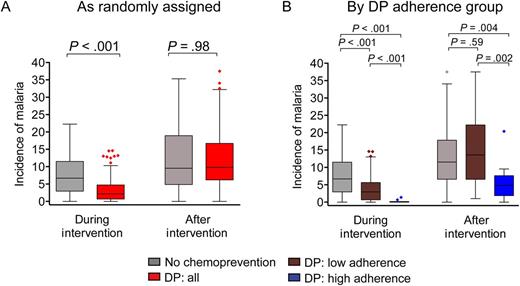
Antimalarial chemoprevention with dihydroartemisinin-piperaquine (DP) does not result in rebound incidence of malaria following cessation and may lead to sustained protection among those who are highly adherent. Incidence of malaria, in cases per person-year at risk, during the intervention (6–24 months of age) and after the intervention (24–36 months of age), stratified by randomization assignment (A) and DP adherence strata (B). Groups include no chemoprevention (n = 90) and all children randomly assigned to receive DP (n = 87; A) and no chemoprevention (n = 90), low adherence to DP (n = 77), and high adherence to DP (n = 10; B). Time at risk excludes 14 days after each treatment for malaria. P values represent between-group comparisons of malaria incidence, using a negative binomial regression model. Incidence rate ratios, 95% confidence intervals, and multivariate adjustment are shown in Table 2.
Frequencies of P. falciparum–specific cytokine–producing T cells (alone or in combination) were reported after background subtraction of the frequency of the identically gated population of cells from the same sample stimulated with control. Background-subtracted responses were consider positive if values were >0.01% of that for the parent population. For multivariate regression models, nonnormal variables were log transformed. Comparisons of cellular frequencies and proportions between groups were performed using the Wilcoxon rank sum test. Statistical analyses of global cytokine profiles were performed by partial permutation tests, using SPICE software, and findings were presented using pie graphs [26]. Negative binomial regression was used to estimate associations between chemoprevention group/adherence strata and immune parameters and the IRR and PRR of malaria in the year following cessation of chemoprevention, with multivariate adjustment as above. Two-sided P values were calculated for all test statistics, and a P value of <.05 was considered significant.
RESULTS
Study Cohort and Clinical Outcomes
The study cohort consisted of 177 infants who were randomly assigned to receive no chemoprevention (n = 90) or DP (n = 87) from 6 through 24 months of age and then followed for an additional year after the chemoprevention intervention. Study drugs were administered at home, without direct observation by study staff. Although DP was associated with a protective efficacy of 58% (95% confidence interval [CI], 45%–67%) in the primary analysis, posttrial analysis of piperaquine levels revealed that adherence to DP therapy was poor [22]. Therefore, we calculated adherence scores on the basis of pharmacokinetic modeling of plasma piperaquine levels [24] and stratified participants randomly assigned to the DP group into low (n = 77) and high (n = 10) adherence groups. Baseline characteristics independently associated with malaria risk in this setting [27], including location of residence, maternal age, housing construction type, bed net use, and primary caregiver education, were similar between the adherence strata (Table 1). Among highly adherent children, the preventive efficacy of DP during the intervention period was extremely high, with 97% fewer cases of incident malaria than among children randomly assigned to receive no chemoprevention (P < .001; Figure 1 and Table 2), and 94% fewer cases of incident malaria as compared to children with low adherence to DP (IRR, 0.06; 95% CI, .02–.21; P < .001.)
Characteristic . | No Chemoprevention (n = 90) . | Monthly DP Adherence Strata . | |
---|---|---|---|
Low (n = 77) . | High (n = 10) . | ||
Female sex | 41 (45.6) | 41 (53.3) | 6 (60) |
Location of residence | |||
Rural | 85 (94.4) | 71 (92.2) | 8 (80) |
Town | 2 (2.2) | 5 (6.5) | 1 (10) |
Unknown | 3 (3.3) | 1 (1.3) | 1 (10) |
Maternal age in y | |||
16 to <22 | 31 (34.4) | 23 (29.9) | 2 (20) |
22 to <36 | 53 (58.9) | 46 (59.7) | 7 (70) |
36 to <45 | 6 (6.7) | 8 (10.4) | 1 (10) |
House construction | |||
Non-ideal home | 81 (91.0) | 68 (88.3) | 7 (70) |
Ideal homea | 6 (6.7) | 8 (10.4) | 2 (20) |
Unknown | 3 (3.3) | 1 (1.3) | 1 (10) |
Primary caregiver education | |||
Primary school or less | 69 (76.7) | 67 (87.0) | 7 (70) |
More than primary school | 18 (20.0) | 9 (11.7) | 2 (20) |
Unknown | 3 (3.3) | 1 (1.3) | 1 (10) |
Nights sleeping under an ITN,b proportion (%) | 2779/2847 (97.6) | 2385/2454 (97.2) | 305/317 (96.2) |
Parasitemia at the time of blood specimen collection | 11 (12.5) | 14 (18.7) | 2 (20) |
Characteristic . | No Chemoprevention (n = 90) . | Monthly DP Adherence Strata . | |
---|---|---|---|
Low (n = 77) . | High (n = 10) . | ||
Female sex | 41 (45.6) | 41 (53.3) | 6 (60) |
Location of residence | |||
Rural | 85 (94.4) | 71 (92.2) | 8 (80) |
Town | 2 (2.2) | 5 (6.5) | 1 (10) |
Unknown | 3 (3.3) | 1 (1.3) | 1 (10) |
Maternal age in y | |||
16 to <22 | 31 (34.4) | 23 (29.9) | 2 (20) |
22 to <36 | 53 (58.9) | 46 (59.7) | 7 (70) |
36 to <45 | 6 (6.7) | 8 (10.4) | 1 (10) |
House construction | |||
Non-ideal home | 81 (91.0) | 68 (88.3) | 7 (70) |
Ideal homea | 6 (6.7) | 8 (10.4) | 2 (20) |
Unknown | 3 (3.3) | 1 (1.3) | 1 (10) |
Primary caregiver education | |||
Primary school or less | 69 (76.7) | 67 (87.0) | 7 (70) |
More than primary school | 18 (20.0) | 9 (11.7) | 2 (20) |
Unknown | 3 (3.3) | 1 (1.3) | 1 (10) |
Nights sleeping under an ITN,b proportion (%) | 2779/2847 (97.6) | 2385/2454 (97.2) | 305/317 (96.2) |
Parasitemia at the time of blood specimen collection | 11 (12.5) | 14 (18.7) | 2 (20) |
Data are no. (%) of children, unless otherwise indicated.
Abbreviations: DP, dihydroartemisinin-piperaquine; ITN, insecticide-treated bed net.
a Defined as the use of nonearth floors, nonthatched roof, and nonmud walls [27].
b Assessed at the time of monthly routine visits to the clinic.
Characteristic . | No Chemoprevention (n = 90) . | Monthly DP Adherence Strata . | |
---|---|---|---|
Low (n = 77) . | High (n = 10) . | ||
Female sex | 41 (45.6) | 41 (53.3) | 6 (60) |
Location of residence | |||
Rural | 85 (94.4) | 71 (92.2) | 8 (80) |
Town | 2 (2.2) | 5 (6.5) | 1 (10) |
Unknown | 3 (3.3) | 1 (1.3) | 1 (10) |
Maternal age in y | |||
16 to <22 | 31 (34.4) | 23 (29.9) | 2 (20) |
22 to <36 | 53 (58.9) | 46 (59.7) | 7 (70) |
36 to <45 | 6 (6.7) | 8 (10.4) | 1 (10) |
House construction | |||
Non-ideal home | 81 (91.0) | 68 (88.3) | 7 (70) |
Ideal homea | 6 (6.7) | 8 (10.4) | 2 (20) |
Unknown | 3 (3.3) | 1 (1.3) | 1 (10) |
Primary caregiver education | |||
Primary school or less | 69 (76.7) | 67 (87.0) | 7 (70) |
More than primary school | 18 (20.0) | 9 (11.7) | 2 (20) |
Unknown | 3 (3.3) | 1 (1.3) | 1 (10) |
Nights sleeping under an ITN,b proportion (%) | 2779/2847 (97.6) | 2385/2454 (97.2) | 305/317 (96.2) |
Parasitemia at the time of blood specimen collection | 11 (12.5) | 14 (18.7) | 2 (20) |
Characteristic . | No Chemoprevention (n = 90) . | Monthly DP Adherence Strata . | |
---|---|---|---|
Low (n = 77) . | High (n = 10) . | ||
Female sex | 41 (45.6) | 41 (53.3) | 6 (60) |
Location of residence | |||
Rural | 85 (94.4) | 71 (92.2) | 8 (80) |
Town | 2 (2.2) | 5 (6.5) | 1 (10) |
Unknown | 3 (3.3) | 1 (1.3) | 1 (10) |
Maternal age in y | |||
16 to <22 | 31 (34.4) | 23 (29.9) | 2 (20) |
22 to <36 | 53 (58.9) | 46 (59.7) | 7 (70) |
36 to <45 | 6 (6.7) | 8 (10.4) | 1 (10) |
House construction | |||
Non-ideal home | 81 (91.0) | 68 (88.3) | 7 (70) |
Ideal homea | 6 (6.7) | 8 (10.4) | 2 (20) |
Unknown | 3 (3.3) | 1 (1.3) | 1 (10) |
Primary caregiver education | |||
Primary school or less | 69 (76.7) | 67 (87.0) | 7 (70) |
More than primary school | 18 (20.0) | 9 (11.7) | 2 (20) |
Unknown | 3 (3.3) | 1 (1.3) | 1 (10) |
Nights sleeping under an ITN,b proportion (%) | 2779/2847 (97.6) | 2385/2454 (97.2) | 305/317 (96.2) |
Parasitemia at the time of blood specimen collection | 11 (12.5) | 14 (18.7) | 2 (20) |
Data are no. (%) of children, unless otherwise indicated.
Abbreviations: DP, dihydroartemisinin-piperaquine; ITN, insecticide-treated bed net.
a Defined as the use of nonearth floors, nonthatched roof, and nonmud walls [27].
b Assessed at the time of monthly routine visits to the clinic.
Incidence of Malaria During and After Chemoprevention Intervention, Stratified by Treatment Arm and Adherence
Treatment Arm . | Children, No. . | Malaria Incidencea . | During Intervention . | Malaria Incidencea . | After Intervention . | ||||||
---|---|---|---|---|---|---|---|---|---|---|---|
Univariate . | Multivariateb . | Univariate . | Multivariateb . | ||||||||
IRR (95% CI) . | P Value . | IRR (95% CI) . | P Value . | IRR (95% CI) . | P Value . | IRR (95% CI) . | P Value . | ||||
No chemoprevention | 90 | 6.93 | Reference | Reference | 10.85 | Reference | Reference | ||||
DPc | |||||||||||
All children | 87 | 3.09 | 0.45 (.34–.60) | <.001 | 0.45 (.34–.59) | <.001 | 10.77 | 0.99 (.79–1.25) | .96 | 1.07 (.86–1.32) | .55 |
Low adherence | 77 | 3.51 | 0.51 (.38–.67) | <.001 | 0.51 (.39–.67) | <.001 | 11.66 | 1.07 (.84–1.35) | .59 | 1.14 (.92–1.41) | .25 |
High adherence | 10 | 0.20 | 0.03 (.01–.09) | <.001 | 0.03 (.01–.10) | <.001 | 4.94 | 0.45 (.26–.78) | .004 | 0.52 (.31–.88) | .014 |
Treatment Arm . | Children, No. . | Malaria Incidencea . | During Intervention . | Malaria Incidencea . | After Intervention . | ||||||
---|---|---|---|---|---|---|---|---|---|---|---|
Univariate . | Multivariateb . | Univariate . | Multivariateb . | ||||||||
IRR (95% CI) . | P Value . | IRR (95% CI) . | P Value . | IRR (95% CI) . | P Value . | IRR (95% CI) . | P Value . | ||||
No chemoprevention | 90 | 6.93 | Reference | Reference | 10.85 | Reference | Reference | ||||
DPc | |||||||||||
All children | 87 | 3.09 | 0.45 (.34–.60) | <.001 | 0.45 (.34–.59) | <.001 | 10.77 | 0.99 (.79–1.25) | .96 | 1.07 (.86–1.32) | .55 |
Low adherence | 77 | 3.51 | 0.51 (.38–.67) | <.001 | 0.51 (.39–.67) | <.001 | 11.66 | 1.07 (.84–1.35) | .59 | 1.14 (.92–1.41) | .25 |
High adherence | 10 | 0.20 | 0.03 (.01–.09) | <.001 | 0.03 (.01–.10) | <.001 | 4.94 | 0.45 (.26–.78) | .004 | 0.52 (.31–.88) | .014 |
Abbreviations: CI, confidence interval; DP, dihydroartemisinin-piperaquine; IRR, incidence rate ratio.
a Data are cases/person-years at risk.
b Multivariate model includes housing location, type, maternal age, and education level of primary caregiver.
c DP adherence strata based on average adherence score (0 to <2, low adherence; >2, high adherence).
Incidence of Malaria During and After Chemoprevention Intervention, Stratified by Treatment Arm and Adherence
Treatment Arm . | Children, No. . | Malaria Incidencea . | During Intervention . | Malaria Incidencea . | After Intervention . | ||||||
---|---|---|---|---|---|---|---|---|---|---|---|
Univariate . | Multivariateb . | Univariate . | Multivariateb . | ||||||||
IRR (95% CI) . | P Value . | IRR (95% CI) . | P Value . | IRR (95% CI) . | P Value . | IRR (95% CI) . | P Value . | ||||
No chemoprevention | 90 | 6.93 | Reference | Reference | 10.85 | Reference | Reference | ||||
DPc | |||||||||||
All children | 87 | 3.09 | 0.45 (.34–.60) | <.001 | 0.45 (.34–.59) | <.001 | 10.77 | 0.99 (.79–1.25) | .96 | 1.07 (.86–1.32) | .55 |
Low adherence | 77 | 3.51 | 0.51 (.38–.67) | <.001 | 0.51 (.39–.67) | <.001 | 11.66 | 1.07 (.84–1.35) | .59 | 1.14 (.92–1.41) | .25 |
High adherence | 10 | 0.20 | 0.03 (.01–.09) | <.001 | 0.03 (.01–.10) | <.001 | 4.94 | 0.45 (.26–.78) | .004 | 0.52 (.31–.88) | .014 |
Treatment Arm . | Children, No. . | Malaria Incidencea . | During Intervention . | Malaria Incidencea . | After Intervention . | ||||||
---|---|---|---|---|---|---|---|---|---|---|---|
Univariate . | Multivariateb . | Univariate . | Multivariateb . | ||||||||
IRR (95% CI) . | P Value . | IRR (95% CI) . | P Value . | IRR (95% CI) . | P Value . | IRR (95% CI) . | P Value . | ||||
No chemoprevention | 90 | 6.93 | Reference | Reference | 10.85 | Reference | Reference | ||||
DPc | |||||||||||
All children | 87 | 3.09 | 0.45 (.34–.60) | <.001 | 0.45 (.34–.59) | <.001 | 10.77 | 0.99 (.79–1.25) | .96 | 1.07 (.86–1.32) | .55 |
Low adherence | 77 | 3.51 | 0.51 (.38–.67) | <.001 | 0.51 (.39–.67) | <.001 | 11.66 | 1.07 (.84–1.35) | .59 | 1.14 (.92–1.41) | .25 |
High adherence | 10 | 0.20 | 0.03 (.01–.09) | <.001 | 0.03 (.01–.10) | <.001 | 4.94 | 0.45 (.26–.78) | .004 | 0.52 (.31–.88) | .014 |
Abbreviations: CI, confidence interval; DP, dihydroartemisinin-piperaquine; IRR, incidence rate ratio.
a Data are cases/person-years at risk.
b Multivariate model includes housing location, type, maternal age, and education level of primary caregiver.
c DP adherence strata based on average adherence score (0 to <2, low adherence; >2, high adherence).
We next evaluated whether chemoprevention alters the development of immunity to malaria, by measuring its impact on malaria incidence and the prevalence of parasitemia during the year of observation after the intervention ended. From 24 to 36 months of age, the incidence of malaria was similar among children previously randomly assigned to receive no chemoprevention or DP (Figure 1 and Table 2). However, children highly adherent to DP had 55% fewer cases of incident malaria in the year after chemoprevention was stopped than children randomly assigned to receive no chemoprevention (P = .004) and 58% fewer cases of incident malaria than children with low adherence to DP (P = .002; Figure 1). Importantly, these associations remained significant after adjustment for factors associated with the risk of malaria in this setting [27], including location of residence, housing type, and maternal age and education (Table 2). Furthermore, in the year after the intervention ceased, children highly adherent to DP had lower rates of blood smear positivity, as assessed by monthly surveillance smears (median, 0.30 blood smear–positive months/months observed) than children randomly assigned to receive no chemoprevention (median, 0.67 blood smear–positive months/months observed; P = .04) and children with low adherence to DP (median, 0.73 blood smear–positive months/months observed; P = .03). Together, these results suggest that monthly DP chemoprevention may not only reduce the risk of malaria during administration, but, if taken regularly, it may also enhance the acquisition of immunity that protects against parasitemia, resulting in sustained clinical protection after chemoprevention is stopped.
Impact of Antimalarial Chemoprevention on the Magnitude and Functional Quality of P. falciparum–Specific CD4+ T-Cell Responses
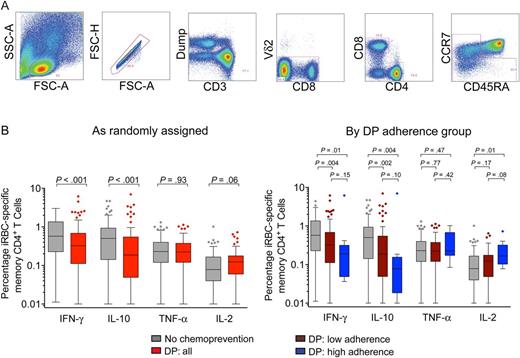
Impact of antimalarial chemoprevention on magnitude of CD4+ T cells responding to red blood cells infected with mature asexual stage parasites (iRBC). A, Gating strategy to identify memory T-cell subsets. Gating strategy to identify cytokine-producing populations shown in Supplementary Data. B, Magnitude of background-subtracted iRBC-specific CD4+ T cells producing interferon γ (IFN-γ), interleukin 10 (IL-10), tumor necrosis factor α (TNF-α), and interleukin 2 (IL-2) stratified by chemoprevention randomization assignment (left) and adherence strata (right). Gray bars represent responses from children given no chemoprevention (NC; n = 90); red bars represent responses from children given dihydroartemisinin-piperaquine (DP; n = 87); maroon bars represent responses from children receiving DP with low adherence, as measured by plasma piperaquine levels (n = 77); and blue bars represent children receiving DP who were highly adherent (n = 10). Abbreviations: FSC, forward scatter; SSC, side scatter.
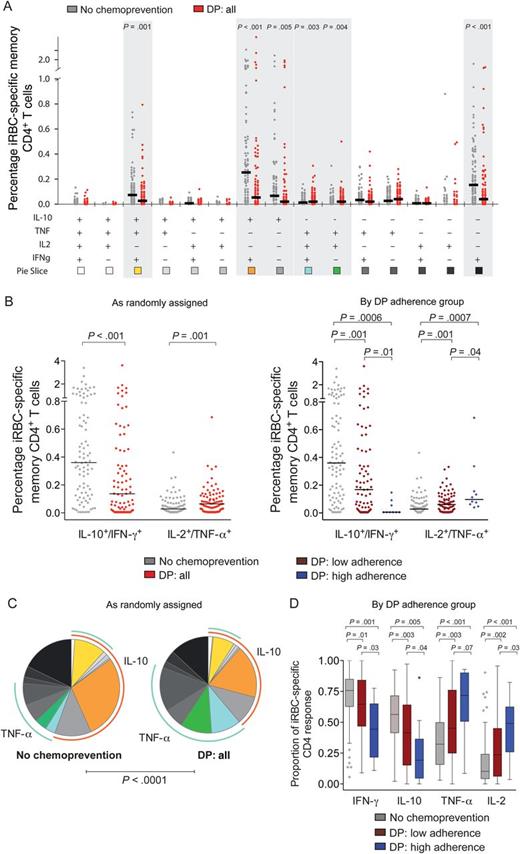
Antimalarial chemoprevention alters the composition of CD4+ T-cell responses to blood-stage parasite antigens. A, Absolute percentage of each individual combination of CD4+ T cells producing interferon γ (IFN-γ), interleukin 10 (IL-10), tumor necrosis factor α (TNF-α), and interleukin 2 (IL-2) in response to red blood cells infected with mature asexual stage parasites (iRBC), stratified by chemoprevention randomization assignment. Gray dots represent responses from children given no chemoprevention (NC; n = 90), and red dots represent responses from children given dihydroartemisinin-piperaquine (DP; n = 87). Horizontal black lines indicate the median response for each group. Shaded bars reveal significantly different responses (P < .05, by the Wilcoxon rank sum test). Responses stratified by adherence strata are shown in Supplementary Data, and sporozoite-specific responses are shown in Supplementary Data. B, Absolute percentage of iRBC-specific IFN-γ/IL-10– and TNF-α/IL-2–coproducing CD4+ T cells stratified by chemoprevention randomization assignment (left) and adherence strata (right). Gray dots represent responses from children given no chemoprevention (NC; n = 90); red dots represent responses from children given DP (DP; n = 87); maroon dots represent responses from children receiving DP with low adherence, as measured by plasma piperaquine levels (n = 77); and blue dots represent children receiving DP who were highly adherent (n = 10). Horizontal black lines indicate the median response for each group. C and D, Relative proportion of each individual combination (C) or any IFN-γ–, IL-10–, TNF-α–, and IL-2–producing CD4+ T cells (D) among total iRBC-specific CD4 response, stratified by chemoprevention randomization assignment (C) and adherence strata (D). For pie charts (C), blue arcs represent the total proportion of CD4+ T cells producing TNF-α; orange arcs represent the proportion of CD4+ T cells producing IL-10.
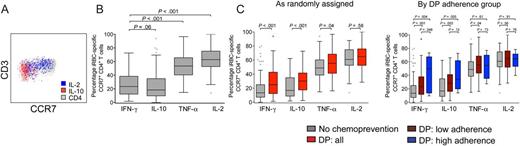
Red blood cells infected with mature asexual-stage parasite (iRBC)–specific CD4+ T-cell responses exhibit distinct memory phenotypes. A, Representative plot showing CCR7 expression by interleukin 10 (IL-10)+ and interleukin 2 (IL-2)+ iRBC-specific memory CD4+ T cells. B, CCR7 expression on iRBC-specific memory CD4+ T cells producing IL-10, interferon γ (IFN-γ), tumor necrosis factor (TNF-α), and IL-2 (right). C, CCR7 expression on iRBC-specific cytokine-producing CD4+ T cells, stratified by chemoprevention assignment (left) and dihydroartemisinin-piperaquine (DP) adherence strata (right). Gray bars represent responses from children given no chemoprevention (NC; n = 90); red bars represent responses from children given DP (DP; n = 87); maroon bars represent responses from children receiving DP with low adherence, as measured by plasma piperaquine levels (n = 77); and blue bars represent children on DP who were highly adherent (n = 10).
Associations Between P. falciparum–Specific T-Cell Responses and Protection From Subsequent Malaria
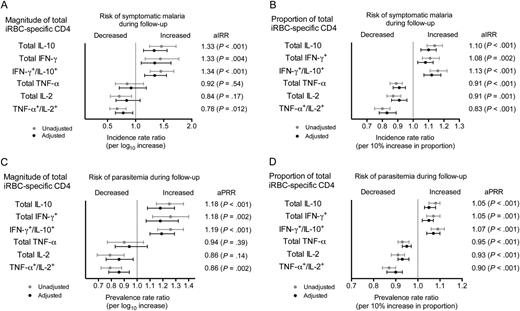
Plasmodium falciparum-specific T-cell responses and protection from subsequent malaria. A and B, Magnitude (A) and proportion (B) of CD4+ T cells responding to red blood cells infected with mature asexual stage parasites (iRBC) and associations with incidence of symptomatic malaria in the year following the cessation of chemoprevention. C and D, Magnitude (C) and proportion (D) of iRBC-specific CD4+ T cells and associations with the prevalence of parasitemia in the year following the cessation of chemoprevention. Gray lines represent unadjusted point estimates and 95% confidence intervals, and black lines represent estimates adjusted for treatment arm, considering adherence, housing structure, location of residence, maternal age, and educational level of primary caregiver. The magnitude of sporozoite-specific CD4+ T cells and associations with the incidence of malaria and the prevalence of parasitemia are shown in Supplementary Data. Abbreviations: aIRR, adjusted incidence rate ratio; aPRR, adjusted prevalence rate ratio; IFN-γ, interferon γ; IL-2, interleukin 2; IL-10, interleukin 10; TNF-α, tumor necrosis factor α.
DISCUSSION
In this study, children randomly assigned to receive antimalarial chemoprevention during 6–24 months of age had significantly greater frequencies of P. falciparum–specific CD4+ T-cell responses coproducing IL-2 and TNF-α, which were associated with protection, and significantly lower frequencies of CD4+ T cells coproducing IL-10 and IFN-γ, which were associated with an increased risk of subsequent malaria. Our data suggest that regular adherence to potent malaria chemoprevention may alter antimalarial immunity through selective blockage of blood-stage parasite infection, in agreement with findings from CPS studies in mice [6–9] and malaria-naive adults [4, 5, 10]. These CPS studies and the success of vaccination with irradiated sporozoites established proof of principle that sterilizing immunity to homologous challenge could be achieved by strategies that allow for pre–erythrocytic stage parasite infection but limit blood-stage parasite infection. Our data raise the tantalizing possibility that children who are highly adherent to DP chemoprevention might demonstrate sustained protection against naturally occurring malaria parasite strains, despite their antigenic diversity.
Routine chemoprevention in children has never been widely implemented in African populations, in part because of logistical constraints and concerns that decreased exposure to malaria parasite antigens among children receiving chemoprevention might result in incident rebound malaria when treatment with the drugs is stopped. While some studies have shown evidence for such a rebound effect [16, 17, 36–39], others have shown a sustained decrease in incident malaria among children previously receiving chemoprevention [20]. It is possible that, for chemoprevention to lead to sustained protection, prevention of blood-stage parasite infection needs to be complete or nearly so. Mounting evidence indicates that frequent or recurrent parasitemia triggers multiple immunoregulatory mechanisms that interfere with the development of durable, efficacious T-cell responses [12]. In our setting, children with low-to-moderate adherence to DP still experienced a very high incidence of malaria during the intervention, which may explain the lack of sustained protection following DP cessation in these groups. Given the poor adherence observed, the use of alternate dosing strategies, including directly observed therapy and/or more-simplified dosing, and other strategies to improve adherence will need to be considered.
The precise mechanisms of protection in experimental models of CPS have been unclear. We have previously shown that chemoprevention was associated with diminished IFN-γ enzyme-linked immunospot responses to several blood-stage antigens but did not enhance IFN-γ responses to pre–erythrocytic stage parasite antigens that were associated with protection [40]. Our present results are consistent with this finding. While we did not observe higher percentages of circulating T cells targeting pre–erythrocytic stage parasite antigens in children receiving DP, this does not exclude the possibility that pre–erythrocytic stage parasite responses may be enhanced in the liver and other tissues [41]. Pathogen-specific T cells exhibit notable functional heterogeneity, however, and measurement of a single parameter of T-cell function (ie, IFN-γ production) may overlook others that are more critical for protection. In our study, chemoprevention enhanced iRBC-specific IL-2– and TNF-α–producing CD4+ T-cell responses associated with protection and suppressed IL-10 production by T-helper type 1 cells, which can be exploited by pathogens to facilitate chronic infection [13, 14, 42, 43]. Pathogen-specific IL-2, TNF-α, and/or IFN-γ–producing CD4+ T cells have been increasingly recognized as a critical determinant of protection from several viral, bacterial, and parasitic infections [31, 33, 44–46] and are found with increased frequency in adults living in high-endemicity settings, who are generally protected against symptomatic P. falciparum infection [47]. Furthermore, individuals protected in experimental CPS studies were found to have increased percentages of iRBC-specific CD4+ T cells producing IFN-γ, IL-2, and/or TNF-α [4, 5], and CD4+ T-cell production of TNF-α and IL-2 induced by vaccination with RTS,S has also been associated with protection in naturally exposed children and experimentally challenged adults [48–50].
The use of childhood chemoprevention to enhance immunity merits further investigation, as the primary limitation of our study was the small number of highly adherent children. It is possible that unmeasured socioeconomic or behavioral confounders may explain the lower incidence of malaria in the highly adherent group. However, several such factors, including maternal age and education level, house construction type, and location of residence, did not significantly differ between the adherence groups. In addition, because the large number of statistical comparisons performed increases the risk of type-1 errors, caution is warranted in interpreting these results, and confirmatory studies are needed. We are unable to state definitively whether the immunologic correlates of protection observed in this study play a causal role in protection or, rather, result from lower blood-stage malaria parasite exposure. Our findings may not be readily generalizable to all epidemiologic settings, as this study was conducted in a region of exceptionally high malaria exposure intensity, where residents sustain on average 1 infectious mosquito bite per day [21]. The protection conferred by experimental CPS has been shown to be dose dependent, requiring monthly inoculations of 10–15 bites from malaria parasite–infected mosquitoes [10], which is similar in magnitude to the exposure experienced by children in Tororo.
In conclusion, in this high-transmission setting, children randomly assigned to receive DP had greater percentages of iRBC-specific CD4+ T cells coproducing IL-2 and TNF-α, which were associated with protection from subsequent malaria and parasitemia, and fewer CD4+ T cells coproducing IL-10/IFN-γ, which were associated with an increased risk of malaria. Among children highly adherent to the intervention, antimalarial chemoprevention appeared to be associated with sustained clinical protection. Monthly DP chemoprevention may represent a potent tool for malaria control for children living in areas of endemicity, both by directly preventing episodes of malaria and, potentially, by enhancing the development of a more effective, adult-like immune response against this deadly disease.
Notes
Acknowledgments. We thank all parents and guardians of the study participants, for kindly giving their consent; the study participants, for their cooperation; all members of the study team, for their tireless effort and excellent work; and S. Kappe and the Seattle Biomedical Research Institute, for provision of P. falciparum sporozoites.
Financial support. This work was supported by the National Institute of Child Health and Human Development (PO1 HD059454), the National Center for Advancing Translational Sciences (UL1 TR000004), the National Institute of Allergy and Infectious Diseases (R01AI093615 and K24AI113002 to M. E. F. and K23 AI100949 to P. J.), the Burroughs Wellcome Fund/American Society of Tropical Medicine and Hygiene (to P. J.), and the Australian government National Health and Medical Research Council (early career fellowship to M. J. B.). The Burnet Institute is supported by the National Health and Medical Research Council Infrastructure for Research Institutes Support Scheme and Victorian State Government Operational Infrastructure Support.
Potential conflicts of interest. All authors: No reported conflicts. All authors have submitted the ICMJE Form for Disclosure of Potential Conflicts of Interest. Conflicts that the editors consider relevant to the content of the manuscript have been disclosed.
References
Author notes
Presented in part: Gordon Research Conference on Malaria, Girona, Spain, 27 July 2015. Abstract 45.