-
PDF
- Split View
-
Views
-
Cite
Cite
Sanae Sasaki, Tyson H. Holmes, Randy A. Albrecht, Adolfo García-Sastre, Cornelia L. Dekker, Xiao-Song He, Harry B. Greenberg, Distinct Cross-reactive B-Cell Responses to Live Attenuated and Inactivated Influenza Vaccines, The Journal of Infectious Diseases, Volume 210, Issue 6, 15 September 2014, Pages 865–874, https://doi.org/10.1093/infdis/jiu190
- Share Icon Share
Abstract
Background. The immunological bases for the efficacies of the 2 currently licensed influenza vaccines, live attenuated influenza vaccine (LAIV) and inactivated influenza vaccine (IIV), are not fully understood. The goal of this study was to identify specific B-cell responses correlated with the known efficacies of these 2 vaccines.
Methods. We compared the B-cell and antibody responses after immunization with 2010/2011 IIV or LAIV in young adults, focusing on peripheral plasmablasts 6–8 days after vaccination.
Results. The quantities of vaccine-specific plasmablasts and plasmablast-derived polyclonal antibodies (PPAbs) in IIV recipients were significantly higher than those in LAIV recipients. No significant difference was detected in the avidity of vaccine-specific PPAbs between the 2 vaccine groups. Proportionally, LAIV induced a greater vaccine-specific immunoglobulin A plasmablast response, as well as a greater plasmablast response to the conserved influenza nuclear protein, than IIV. The cross-reactive plasmablast response to heterovariant strains, as indicated by the relative levels of cross-reactive plasmablasts and the cross-reactive PPAb binding reactivity, was also greater in the LAIV group.
Conclusions. Distinct quantitative and qualitative patterns of plasmablast responses were induced by LAIV and IIV in young adults; a proportionally greater cross-reactive response was induced by LAIV.
Influenza virus causes seasonal epidemics and occasional pandemics. The best way to prevent influenza outbreaks is to vaccinate the population with a vaccine antigenically matched to the circulating virus. In the United States, 2 types of influenza vaccine are currently available: a trivalent or quadrivalent inactivated influenza vaccine (IIV), which is administered intramuscularly or intradermally, and a quadrivalent live attenuated influenza vaccine (LAIV), which is administered intranasally. The 2 envelope proteins of influenza virus, hemagglutinin (HA) and neuraminidase (NA), are considered the primary antigenic targets of protective antibody (Ab). These antigens can be highly variable from one year to the next, and the vaccine efficacy is reduced when circulating virus strains are antigenically mismatched with the vaccine strains [1, 2].
Several efficacy studies for IIV versus LAIV showed that LAIV had better efficacy than IIV in children, whereas IIV had an efficacy comparable to or better than LAIV in adults (defined as individuals aged 17–49 years old) [3]. LAIV is not currently licensed for use in children <2 years old or in adults aged ≥50 years. However, limited data suggest that the 2 vaccines are similarly effective in adults older than 60 years [4].
Influenza virus–specific serum Ab levels have been shown to correlate with protection against subsequent influenza [5–7]. Therefore, strain-specific circulating Abs are considered a major mediator of protective immunity. The serum Ab response after IIV receipt, measured by neutralization or hemagglutination inhibition assays, is a well-established correlate of vaccine efficacy [8, 9]. In contrast, despite the comparable or superior efficacy of LAIV in different age groups, serum Ab responses to LAIV are always much weaker than after IIV and are generally poor correlates of efficacy [10, 11].
Plasmablasts derived from activated B cells are released from germinal centers and transiently appear in the circulation approximately 7 days after influenza vaccination, before they migrate to different organs. These plasmablasts are highly enriched with vaccine-specific Ab-secreting cells (ASCs) [12]. By studying this plasmablast response, important quantitative and qualitative features of the B-cell response to influenza vaccination have been characterized [12–16]. The goal of the current study was to evaluate vaccine-induced plasmablast responses in healthy young adults vaccinated with either IIV or LAIV, to identify characteristics of the Ab responses that correlate with their known efficacies. Our findings provide new insights into the Ab responses induced by these 2 types of influenza vaccine.
MATERIALS AND METHODS
Human Participants, Vaccines, and Blood Samples
Volunteers 18–30 years old were enrolled and randomly assigned to vaccine groups during the 2010–2011 (n = 31) and the 2011–2012 (n = 41) influenza seasons. Each year, random assignment was to a group that received either 1 dose of seasonal IIV (Fluzone, Sanofi Pasteur) or 1 dose of seasonal LAIV (FluMist, MedImmune). None of the 2010–2011 participants received the monovalent pandemic A/California/7/2009(H1N1) vaccine in 2009. None of the 2011–2012 participants received the 2010 seasonal influenza vaccines. The study was approved by the Stanford Institutional Review Board. Written informed consent was obtained from all participants. The 2010 and 2011 vaccines contained the same 3 strains: an A/California/7/2009(H1N1)-like virus, an A/Perth/16/2009(H3N2)-like virus, and a B/Brisbane/60/2008-like virus. B-cells were isolated from blood samples collected between days 6 and 8 after vaccination, using the RosetteSep Human B-cell Enrichment Cocktail (Stemcell Technologies). Serum was isolated from blood samples collected on day 0 and within 4 days (before or after) day 28.
Enumeration of ASCs
Total and influenza virus–specific immunoglobulin A (IgA) and immunoglobulin G (IgG) ASCs were enumerated using enzyme-linked immunosorbent spot (ELISPOT) analysis, as described elsewhere [15]. For total ASCs, 96-well MultiScreen HTS plates (Millipore) were coated with goat anti-human IgA, IgG, and immunoglobulin M (heavy and light chains; KPL). For influenza virus–specific ASCs, the plates were coated with IIV (Fluzone) or recombinant influenza A virus nuclear protein (NP; Imgenex, at 4.5 µg/mL). Plates were blocked, incubated with freshly isolated B-cells mixed with phosphatase-conjugated goat antibodies against human IgA(α) or IgG(γ) (KPL), and developed for counting spots [15].
Generation of Plasmablast-Derived Polyclonal Antibodies (PPAbs)
PPAbs were collected from ex vivo B-cell cultures in complete medium as previously described [14]. The concentrations of IgA and IgG in PPAbs were determined with the Immuno-Tek Quantitative Human IgA or IgG enzyme-linked immunosorbent assay (ELISA) kits (Zeptometrix), respectively.
Analysis of Influenza-Specific Binding Activity of PPAbs
ELISAs were performed as described elsewhere [13]. Ninety-six–well ELISA plates (Greiner) were coated with IIV (2.7 µg/mL), recombinant HAs (Immune Technology, at 5 µg/mL), or recombinant NP (5 µg/mL). Plates were blocked and then incubated with serially diluted PPAbs. Wells incubated with complete medium served as negative controls. The plates were washed and incubated with peroxidase-conjugated goat anti-IgG(γ) or goat anti-IgA(α) (KPL) and developed with TMB substrate (KPL). The OD450 nm was measured. The cutoff was set as the mean value plus 2 standard deviations of the average OD450 nm values of all negative control wells. The titer was defined as the reciprocal of the highest dilution at which the mean OD450 nm of duplicate wells was greater than the cutoff.
Serum Influenza Virus Neutralization Assay
Influenza virus neutralization assay was performed as described elsewhere [17, 18]. In brief, sera were treated with receptor-destroying enzyme (Vibrio cholera filtrate; Sigma-Aldrich) to eliminate nonspecific inhibitors. The treated serum samples were serially diluted and mixed with virus, incubated to neutralize the virus, then transferred to Madin-Darby canine kidney cells and cultured for 4 days. Virus production was determined by an HA assay. Titer was defined as the reciprocal of the highest dilution of serum that neutralized 200 plaque-forming units of influenza virus. See the Supplementary Data for more details.
Statistical Analysis
Geometric means were compared between groups using 2-sample t tests, adjusted for unequal variances, and paired t tests. Means of ratios were compared between groups by fitting regression models, using the generalized maximum entropy (GME) approach [19] for comparisons at day 0 and robust MM regression [20] for other comparisons. Additionally, regression fitting via the GME approach was used to compare groups. Results were declared statistically significant if P values were ≤.05. Analyses were conducted in SAS 9.4 (Cary) and GraphPad Prism 5 (GraphPad; La Jolla). See the Supplementary Data for more details.
RESULTS
Plasmablast Responses to LAIV and IIV
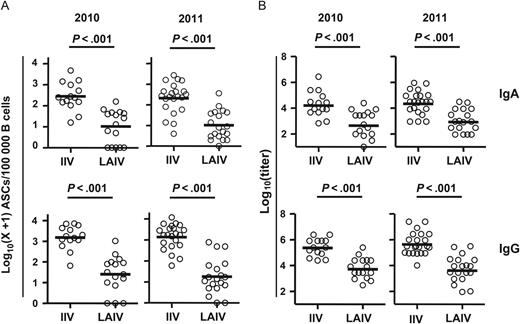
Vaccine-specific plasmablast responses to inactivated influenza vaccine (IIV) and live attenuated influenza vaccine (LAIV). A, Frequency of influenza vaccine–specific immunoglobulin A (IgA) and immunoglobulin G (IgG) antibody-secreting cells (ASCs) on days 6–8 after vaccination for the 2010 and 2011 influenza seasons. B, Titers of vaccine-specific IgA and IgG plasmablast-derived polyclonal antibodies (PPAbs) collected from ex vivo–cultured B cells obtained 6–8 days after the indicated vaccination in 2010 and 2011. Bars indicate geometric means. Hypothesis testing used unpaired t tests to compare the IIV and LAIV groups.
IgA Response in LAIV Recipients Is More Prominent Than That in IIV Recipients
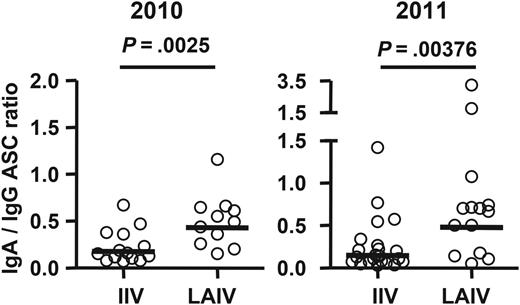
Ratios of vaccine-specific immunoglobulin A (IgA) antibody-secreting cell (ASC) frequency to vaccine-specific immunoglobulin G (IgG) ASC frequency following receipt of live attenuated influenza vaccine (LAIV) or inactivated influenza vaccine (IIV). Subjects with no vaccine-specific IgA or IgG ASCs detected were excluded from this analysis. Bars indicate geometric means. Hypothesis testing used unpaired t tests to compare the IIV and LAIV groups.
Quantity and Avidity of LAIV- and IIV-Induced PPAbs
![Quantity and avidity of vaccine-specific plasmablast-derived polyclonal antibodies (PPAbs) following receipt of live attenuated influenza vaccine (LAIV) or inactivated influenza vaccine (IIV). A, Yield of immunoglobulin A (IgA) or immunoglobulin G (IgG) per antibody-secreting cell, calculated by dividing the total IgA or IgG concentration in the PPAb sample by the total number of IgA or IgG ASCs determined by enzyme-linked immunosorbent spot analysis in each milliliter of B-cell culture, which contained 3 × 106 B cells/mL. B, Concentration of vaccine-specific IgA or IgG in PPAb samples, calculated by multiplying the yield of IgA or IgG per ASC (A) by the number of vaccine-specific IgA or IgG ASCs in each milliliter of B-cell culture, which is calculated using the frequency of vaccine-specific ASCs (Figure 1A) and the total number of 3 × 106 B cells/mL. C, Avidity of vaccine-specific IgA or IgG PPAbs, defined as the concentration of vaccine-specific PPAbs (B) divided by the vaccine-specific enzyme-linked immunosorbent assay titer of PPAbs (Figure 1B) [15]. Samples from 2010 and 2011 study participants given each vaccine were combined for these analyses. Bars indicate geometric means. Hypothesis testing used unpaired t tests to compare the IIV and LAIV groups.](https://oup.silverchair-cdn.com/oup/backfile/Content_public/Journal/jid/210/6/10.1093_infdis_jiu190/3/m_jiu19003.jpeg?Expires=1750133368&Signature=XB6IdSQspwdOKGtqcHxxnWcnkijMA0TF136M2BlIQyiSC3lSiNM5D-pHXmChWlW-NIYpF5BREEnurA6h3NqfVeUNQpPel1jHZwo5WONXn0JyhXjEchaV2i84ur~ApdmTekSKfDXrOJUiwU3Jkaa46qMEdc330bpI6eNSj07mY6l4Ymlm~NnUJWwwERqtKa2qtKG1YgdOll6P5XnFE9n7e9KaiO-hB6ExNcb5NupUVXSLJ20dyVvtfUwUmtIQsf~aVZjfWmR8FODDPWAIBe4XoxEV3K68u0iSXJ8lGSVOF42HzVibn32s9YprlDeVRwwvYuPXu3nN513Tjz6dswkmlg__&Key-Pair-Id=APKAIE5G5CRDK6RD3PGA)
Quantity and avidity of vaccine-specific plasmablast-derived polyclonal antibodies (PPAbs) following receipt of live attenuated influenza vaccine (LAIV) or inactivated influenza vaccine (IIV). A, Yield of immunoglobulin A (IgA) or immunoglobulin G (IgG) per antibody-secreting cell, calculated by dividing the total IgA or IgG concentration in the PPAb sample by the total number of IgA or IgG ASCs determined by enzyme-linked immunosorbent spot analysis in each milliliter of B-cell culture, which contained 3 × 106 B cells/mL. B, Concentration of vaccine-specific IgA or IgG in PPAb samples, calculated by multiplying the yield of IgA or IgG per ASC (A) by the number of vaccine-specific IgA or IgG ASCs in each milliliter of B-cell culture, which is calculated using the frequency of vaccine-specific ASCs (Figure 1A) and the total number of 3 × 106 B cells/mL. C, Avidity of vaccine-specific IgA or IgG PPAbs, defined as the concentration of vaccine-specific PPAbs (B) divided by the vaccine-specific enzyme-linked immunosorbent assay titer of PPAbs (Figure 1B) [15]. Samples from 2010 and 2011 study participants given each vaccine were combined for these analyses. Bars indicate geometric means. Hypothesis testing used unpaired t tests to compare the IIV and LAIV groups.
LAIV Induces a Greater Cross-reactive Plasmablast Response Than IIV
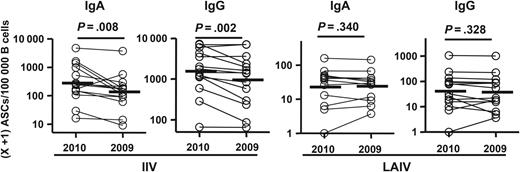
Frequencies of immunizing vaccine-specific versus heterovariant-specific antibody-secreting cells (ASCs) following receipt of 2010 inactivated influenza vaccine (IIV) and live attenuated influenza vaccine (LAIV). Frequencies of ASCs specific for the homologous vaccine antigen (2010 vaccine) and the heterovariant influenza virus antigen (2009 vaccine) from each individual are shown as circles connected by a line. These ASCs were detected with enzyme-linked immunosorbent spot plates coated with the 2010 or 2009 IIV. Bars indicate geometric means. Hypothesis testing used a paired t test.
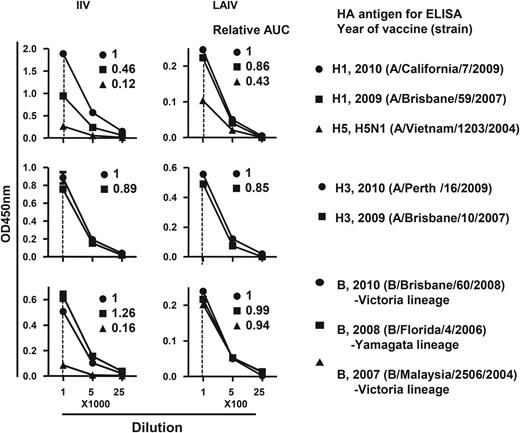
Cross-reactive hemagglutinin (HA) binding activity of plasmablast-derived polyclonal antibodies (PPAbs) after immunization with 2010 inactivated influenza vaccine (IIV) and live attenuated influenza vaccine (LAIV). A pool of PPAbs was assembled by combining equal amounts of immunoglobulin G (IgG) from each PPAb sample in the IIV or LAIV groups. Each PPAb pool was serially diluted, starting at a concentration of 9 ng/mL for LAIV and 4.1 ng/mL for IIV of IgG, and analyzed with an enzyme-linked immunosorbent assay (ELISA) for binding to recombinant HAs from indicated influenza virus strains. Relative binding activity to each HA (numbers in each panel) was defined as the ratio of its area under curve (AUC) to that of the relevant 2010 vaccine strain (circle in each panel, assigned a value of 1).
Vaccine- and Heterovariant-Specific Serum Neutralizing Ab Response Induced by LAIV and IIV
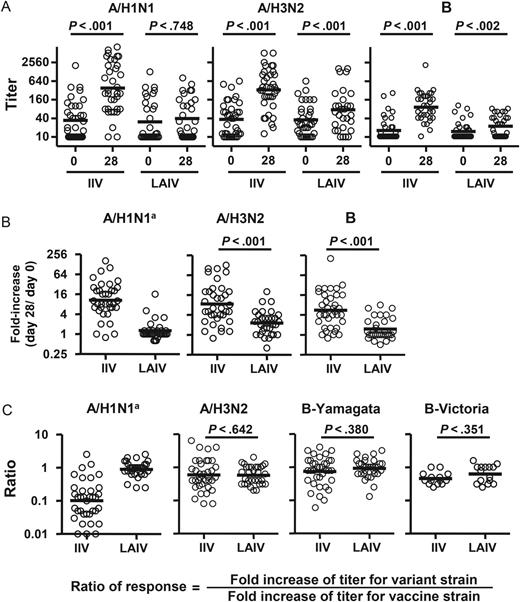
Vaccine- and heterovariant-specific serum neutralizing antibody responses to live attenuated influenza vaccine (LAIV) or inactivated influenza vaccine (IIV) immunization. A, Serum neutralization titers against the 3 vaccine strains before (day 0) and after (day 28 ± 4) vaccination. B, Fold-increase in titer after vaccination. C, Cross-reactive neutralizing antibody responses, calculated as the ratio of titer fold-increase for the heterovariant strain to the titer fold-increase against the respective homologous vaccine strain. The vaccine strains tested were A/California/7/2009(H1N1), A/Perth/16/2009(H3N2), and B/Brisbane/60/2008. The heterovariant strains tested were A/Brisbane/59/2007(H1N1), A/Brisbane/10/2007(H3N2), B/Florida/4/2006 (Yamagata lineage), and B/Malaysia/2506/2004 (Victoria lineage). The 2011 serum samples were not tested with B/Malaysia/2506/2004 (Victoria). Samples from all 2010 and 2011 study participants were included. Bars indicate geometric means, which were calculated across triplicate assays per sample via fitting a Weibull distribution model. Hypothesis testing employed robust MM regression. aComparison between the TIV and LAIV groups was not performed because of the lack of response in the LAIV recipients.
Heterovariant serum Ab responses were then evaluated using the ratio of titer fold-increase for the heterovariant strain over the titer fold-increase for the homologous vaccine component (Figure 6C). Significant differences were not detected in H3N2 and B cross-reactivity between the 2 vaccine groups. In the IIV recipients, the mean neutralization response for the H1N1 variant was 10-fold lower than that for the H1N1 vaccine strain (ratio of response, 0.1; Figure 6C).
Plasmablast Response to NP in LAIV Recipients Is More Prominent Than That in IIV Recipients
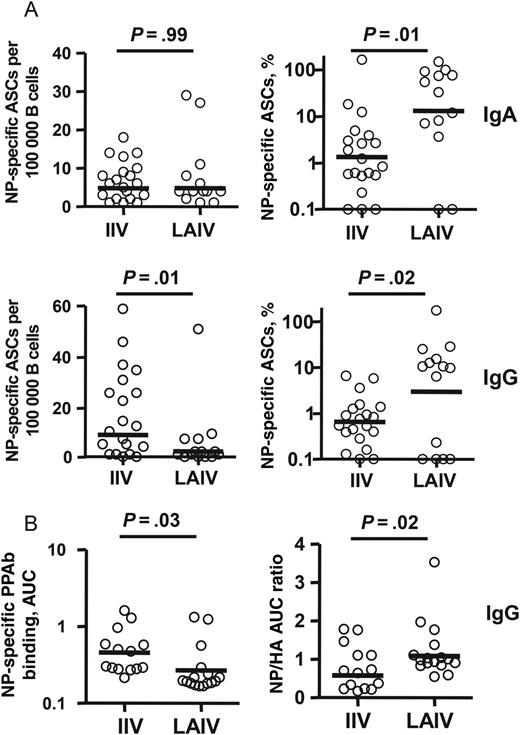
Nuclear protein (NP)–specific plasmablast responses to live attenuated influenza vaccine (LAIV) and inactivated influenza vaccine (IIV). A, Frequency of NP-specific immunoglobulin A (IgA) and immunoglobulin G (IgG) antibody-secreting cells (ASCs) (left panels) and percentage of NP-specific ASCs among total vaccine-specific ASCs (right panels) after immunization with 2011 LAIV or IIV. The NP-specific ASCs were measured with enzyme-linked immunosorbent spot plates coated with recombinant NP. B, Binding activity of NP-specific IgG plasmablast-derived polyclonal antibodies (PPAbs) from recipients of 2010 IIV or recipient of 2010 LAIV (left panel) and relative NP binding activity normalized to HA (H1N1) binding activity (right panel). The binding activity was calculated as the area under curve (AUC) of serially diluted PPAb samples, using an enzyme-linked immunosorbent assay (ELISA) with recombinant NP- or HA-coated ELISA plates, starting at a dilution of 1:100 for NP or 1:1000 for HA. Bars indicate geometric means. Means were compared between IIV and LAIV groups by an unpaired t test or by fitting a regression model, using the generalized maximum entropy approach.
DISCUSSION
The goal of this study was to compare the vaccine induced B-cell responses to LAIV and IIV in young adults. We demonstrated that IIV induced significantly more vaccine-specific ASCs than did LAIV in the peripheral blood 6–8 days after immunization (Figure 1), which is the peak time for plasmablast responses to IIV [12, 25] and LAIV [13, 26] (unpublished data). Although IIV induced a significantly greater quantity of vaccine-specific PPAbs than LAIV, no differences in vaccine-specific PPAb avidity were detected between the 2 vaccine groups (Figure 3). We also found that LAIV induced a greater relative IgA plasmablast response (normalized to the IgG response; Figure 2), as well as a greater relative Ab response to NP (normalized to the response to HA), than did IIV (Figure 7). Perhaps most interestingly, LAIV also elicited greater cross-reactive plasmablast responses than IIV against variant influenza virus strains, when normalized to the responses against homologous vaccine strains (Figures 4 and 5). These findings extend and expand our understanding of the different qualities and quantities of B-cell responses to 2 current influenza vaccines and shed light on the mechanisms underlying their respective contributions to protective immunity.
Secretory IgA is a critical component of the acquired mucosal immune response and the first line of defense against respiratory viral infections. A few studies have shown that mucosal HA-specific IgA responses after LAIV are greater than those induced by IIV in seronegative adults [27, 28], but the lack of sensitive and standardized methods for collection and analysis of mucosal secretory antibodies has discouraged direct measurement of such antibodies in most clinical studies of influenza vaccination. We previously compared PPAbs and serum samples from influenza vaccine recipients and reported that plasmablast responses are better reflections of the ongoing IgA response than serum Ab levels [14]. In the current study, we extended these findings and demonstrated that LAIV induces a relatively greater IgA plasmablast response than IIV (Figure 2), which is in agreement with the notions that a mucosal Ab response is preferentially induced following immunization at the mucosal site and that the peripheral plasmablast response provides an alternate window for studying mucosal Ab responses.
Currently used influenza vaccines, particularly IIV, provide limited efficacy against circulating strains that are HA mismatched with the vaccinating strain, and universal influenza vaccines that protect against a broader range of seasonal and pandemic influenza viruses are highly desirable. One of the approaches to develop such vaccines is by inducing antibodies to the conserved components of the virus, such as the HA stalk domain [29, 30], matrix protein 2 [31], or NP [24]. It was reported that systemic immunization with NP in mice accelerated clearance of influenza virus in an Ab-dependent manner, suggesting a possible protective role of NP-specific antibodies [24]. Interestingly, despite large amounts of NP in commercial IIV preparations [32, 33], the circulating anti-NP response after IIV vaccination was much weaker than the anti-HA Ab response [24]. In the current study, we demonstrated that LAIV induced a greater relative plasmablast response to NP than did IIV. These results suggest that the native NP carried by LAIV or, more likely, the NP synthesized de novo in LAIV-infected cells is more immunogenic than the NP carried by IIV. It will be important to compare the characteristics of specific antibodies induced by the native NP in LAIV with those induced by the NP component of IIV and to determine their contributions to the efficacy of each vaccine in cross-protection.
We observed that LAIV induced relatively greater cross-reactive PPAb binding than IIV to HAs of heterovariant H1N1 and B strains, whereas both LAIV and IIV induced cross-reactive PPAb responses to the HA of the heterovariant A/Brisbane/10/2007(H3N2) (Figure 5). The HA amino acid sequence of A/Brisbane/10/2007 is highly homologous to that of the 2010–2011 H3N2 vaccine strain A/Perth/16/2009, with only a few different amino acid residues in HA1 [34]. This might explain the high levels of cross-reactive PPAb binding and serum neutralizing reactivity against the 2 different H3N2 strains. We previously reported that the inactivated 2009 pandemic monovalent H1N1 vaccine (A/California/7/2009 or A[H1N1]pdm09) induced a better cross-reactive H1N1 response in young adults than did the H1N1 component of the 2009 seasonal IIV (A/Brisbane/59/2007) [16]. Similarly, the 2010 IIV used in the current study also induced a substantial cross-reactive PPAb response because the 2010 influenza vaccines contain the same A(H1N1)pdm09 strain as its H1N1 component as the 2009 monovalent vaccine (Figure 5; unpublished data). Interestingly, compared with the 2010 IIV, the 2010 LAIV induced even better cross-reactive PPAb binding to HAs of the heterovariant H1N1 and heterosubtypic H5N1 strains (Figure 5).
The HAs derived from the different H1N1 or H5N1 strains have distinct head domains but share conserved stalk domains [23]. The 2009 seasonal IIV induced an Ab response that predominantly recognized the head domain rather than the stalk domain of the HA [16, 35]. On the other hand, the better cross-reactive HA-specific PPAb response following LAIV immunization was at least in part due to the relatively greater induction of a stalk-specific response (Figure 5). The greater cross-reactive Ab response seen after LAIV immunization is in agreement with a previous study that showed a reduced frequency of influenza due to infection with a mismatched influenza virus strain in adults who received LAIV versus those who received IIV [36]. This difference could also contribute to the better cross-protection of LAIV against mismatched strains in children [37, 38]. It will be of interest to determine whether an Ab response against the HA stalk that blocks membrane fusion of the viral particle [29, 30] contributes to the cross-protection efficacy of LAIV.
In agreement with previous reports [10, 11, 13, 26], we showed that the serum neutralizing Ab response to LAIV was significantly weaker than the response to IIV. Interestingly, despite the substantial cross-reactive PPAb binding to the HA of the heterovariant H1N1 strain after IIV receipt, the mean cross-reactive neutralization response for the H1N1 variant was roughly 10-fold lower than that for the H1N1 vaccine strain (Figure 6C), indicating that conventional serological analysis does not provide a complete picture of the B-cell response to influenza vaccines. Since the low Ab concentration in PPAb samples prevented direct analysis of their individual neutralizing activity, our analysis of PPAb function was limited to an examination of binding activity. Although viral neutralization is considered a primary mechanism of the protective Ab response, influenza virus–specific nonneutralizing antibodies may also contribute to viral clearance through different mechanisms, including NA-specific antibodies [39, 40], Fc receptor–mediated phagocytosis [41, 42], complement-dependent cytotoxicity [43], and Ab-dependent cell-mediated cytotoxicity [44, 45]. In the current study, we observed substantial differences in the quality and specificity of the plasmablast responses induced by LAIV and IIV. Further analyses of these differences in the context of both neutralizing and nonneutralizing reactivities should provide more insights to the basis of protective immunity following immunization with different types of influenza vaccines.
Notes
Acknowledgments. We thank our study subjects, for their participation; C. Zhang, for technical assistance; S. Mackey, for coordinating the clinical study; S. Swope, S. Cathey, C. Walsh, S. French, and M. Ugur, for enrolling subjects, administering vaccine, and collecting samples and clinical data; and T. Quan, K. Spann, S. Batra, and B. Tse, for screening and scheduling subjects and providing regulatory and clinical data management support.
Financial support. This work was supported by the National Institutes of Health (NIH; grants AI090019, AI057229, and AI089987) and the National Center for Research Resources, NIH (Clinical and Translational Science Award UL1RR025744).
Potential conflicts of interest. H. B. G. is on the scientific advisory board of Novartis Vaccines, a major producer of influenza vaccines, and a consultant for Vaxart, a developer of novel influenza vaccines. All other authors report no potential conflicts.
All authors have submitted the ICMJE Form for Disclosure of Potential Conflicts of Interest. Conflicts that the editors consider relevant to the content of the manuscript have been disclosed.
References
Author notes
Presented in part: 31st Annual Meeting of the American Society for Virology, Madison, Wisconsin, July 2012. Abstract W14-7.