-
PDF
- Split View
-
Views
-
Cite
Cite
Myriam Siegwart, Bertrand Gauffre, Elodie Lecerf, Jean-Luc Poëssel, Efficacy of 2 botanical aphicides, chicoric and 3,5-dicaffeoylquinic acids, on aphids susceptible and resistant to synthetic insecticides, Journal of Economic Entomology, Volume 117, Issue 4, August 2024, Pages 1347–1355, https://doi.org/10.1093/jee/toae069
- Share Icon Share
Abstract
Dicaffeoyltartaric acid (diCT) and 3,5-dicaffeoylquinic acid (3,5-diCQ) are described for their aphicidal properties on several aphid species. Intending to valorize diCT and 3,5-diCQ as biocontrol products and because of the high adaptive capacities of aphids to xenobiotics, we sought to determine the existence of adaptation first in Myzus persicae (Sulzer) (Hemiptera: Aphididae) and then other aphids. Resistance of aphids to these biopesticides could be promoted by (i) the existence of resistance to synthetic insecticides that may confer cross-resistance and (ii) the presence of these compounds in wild plants likely which may have led to pre-existing adaptation in aphids. We assessed the resistance levels to diCT and 3,5-diCQ in 7 lab strains (including some resistant to synthetic aphicides) and 7 wild populations of M. persicae using biotests. The activities of detoxification enzymes contributing to insecticide resistance were also measured. Additionally, we followed the same method to characterize susceptibility to these caffeic derivatives in wild populations of Nasonovia ribisnigri (Mosley) (Hemiptera: Aphididae), Brevicoryne brassicae(Linnaeus) (Hemiptera: Aphididae) and, Aphis craccivora(Koch) (Hemiptera: Aphididae). Our results show variability in susceptibility to diCT between populations of M. persicae, but resistance ratios (RR) were low (RR = 3.59). We found no cross-resistance between synthetic insecticides and diCT. Carboxylesterase and glutathione-S-transferase did not seem to be involved in its detoxification. A clone of A. craccivora collected from peanut, a species rich in diCT, was not susceptible to either diCT or 3,5-diCQ, suggesting a common molecular target for these 2 molecules and the existence of a high-effect resistance mechanism. These active botanical substances remain good candidates for M. persicae biocontrol in agriculture.
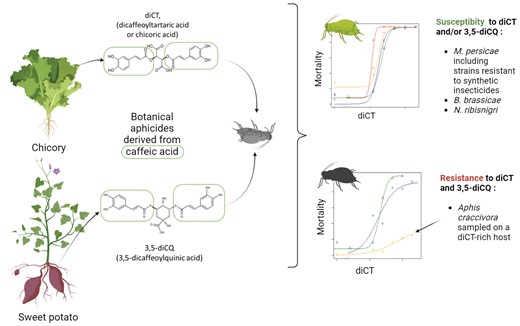
Introduction
Aphids are major insect pests that wreak havoc on various crops. They are the target of insecticide treatments, among which neonicotinoid pesticides, in a wide range of crops, such as sugar beet (Hauer et al. 2017), potato and fruits (Elbert et al. 2008). The adverse effects of neonicotinoids on bee species have led to a progressive ban of these compounds in some European countries (Decourtye and Devillers 2010), where alternative crop protection techniques are urgently needed.
Previous studies have shown that 3,5-dicaffeoylquinic (3,5-diCQ) and dicaffeoyltartaric (chicoric acid, diCT) acids, 2 phenolic compounds derived from caffeic acid, have aphicidal properties following ingestion by several aphid species, including Myzus persicae (Poëssel et al. 2009, 2014, Li et al. 2016, 2021). These compounds, identified in food plants such as sweet potatoes, chicory, and endive, do not have any reported harmful effects on human health or the environment (Alcázar Magaña et al. 2021, Yang et al. 2022). While 3,5-diCQ and diCT are promising candidates as agriculture biocontrol products, their durability depends heavily on preexisting adaptation in aphid species, a significant determinant of resistance evolution speed (Georghiou and Taylor 1977). This question is particularly relevant here because (i) many resistances to xenobiotics, particularly insecticides, have been observed in aphid species, which may result in cross-resistance, that is, resistance to several active ingredients (Foster et al. 2007), and (ii) these molecules are present in diverse plant species (Silva et al. 2014) that are hosts to aphids. Hence, aphid populations might have already evolved tolerance or resistance to these molecules in the wild.
The green peach aphid (M. persicae) is a global and highly polyphagous pest. In addition to causing direct damage to its host plants, it is a vector of over 100 plant viruses that cause diseases and yield losses in many vital crops such as beans, sugar cane, sugar beet, brassicas, tobacco, potatoes, and citrus (Kennedy et al. 1962). In temperate regions such as France, it can be dioecious holocyclic species, that is, it has a primary host, peach, on which it lays eggs, resulting from sexual reproduction that can resist winter cold. Thus, the genetic diversity of M. persicae populations is the greatest on peach trees. Some individuals can reproduce by parthenogenesis all year long on secondary hosts in the absence of frost episodes (Guillemaud et al. 2003). Myzus persicae clones growing on tobacco differ in morphology, color, and year-round asexuality. They were renamed M. persicae nicotianae by Blackman and Eastop (2007).
Myzus persicae is highly adaptable to insecticides. It has developed resistance to more active ingredients than other known insect species (Silva et al. 2012, Bass et al. 2014). Mechanisms for insecticide resistance in the genus Myzus can be classified into 3 categories: (i) reduction of insecticide uptake through decreased cuticle permeability, (ii) detoxification of the active ingredient via variations in the activities of enzymes that cause its excretion, metabolization, or chelation, and (iii) loss of affinity of insecticide molecular targets via genetic mutations (Bass et al. 2014). Four molecular target mutations have been described in this species M. persicae (kdr, super-kdr, MACE, and Rdl). A more recent study demonstrates the association between a mutation in ACCase (A2666V) and the resistance of this species to active substances belonging to the ACCase inhibitor family (Umina et al., 2022). Detoxification, like the overproduction of carboxylesterases E4 or FE4, is another notable resistance mechanism that induces cross-resistance to organophosphates and pyrethroids and, to a lesser extent, carbamates. Additionally, CYP6CY3 overproduction confers resistance to neonicotinoids and nicotine (Bass et al. 2014). Since diCT and 3,5-diCQ are toxic by ingestion and/or phagorepulsive and absent from the pesticide market, detoxification appears as the most likely mechanism of cross-resistance, if it exists, between diCT and 3,5-diCQ and synthetic insecticides (Singh et al. 2020). A similar cross-resistance was reported in M. persicae nicotianae, in which clones that can feed on tobacco, a crop rich in nicotine, developed detoxification mechanisms that confer resistance to synthetic insecticides (Singh et al. 2020). Aphid species that feed on plants naturally rich in diCT and 3,5-diCQ may also be less susceptible to these molecules, like the cabbage aphid, Brevicoryne brassicae, which does not activate some secondary metabolites (glucosinolates) of its host plants but also use these compounds against its predators (Kazana et al. 2007).
In this study, we investigated whether there is adaptation to (i) diCT in 7 wild M. persicae populations collected from peach trees and in 3 M. persicae lab strains resistant to synthetic insecticides and to (ii) diCT and 3,5-diCQ in 3 M. persicae nicotianae clones since this subspecies developed detoxification mechanisms to feed on a host plant rich in nicotine, and 3 populations of other aphid species growing on plants rich in diCT, 3,5-diCQ, or other plant secondary metabolites. We hypothesized that detoxification would be the most likely resistance mechanism; hence, we assessed the involvement of detoxification enzymes, carboxylesterases, and glutathione-S-transferases in diCT/3,5-diCQ adaptation.
Materials and Methods
Aphids
Strains and populations of M. persicae from peach orchards.
Tests for susceptibility to diCT were conducted on 4 lab strains and 7 wild populations of M. persicae sampled in peach orchards in the Rhône Valley.
Three lab strains resistant to synthetic insecticides originated from Anses in Lyon (Casper Research Unit). They were resistant to 2 or 3 families of synthetic insecticides: organophosphates, pyrethroids, and neonicotinoids (Table 1). Strains Res_1, Res_2, and Res_3 were collected from peach trees in the field in 2011, 2012, and 2013, respectively, and reared at Anses on Chinese cabbage (Brassica rapa subspecies chinensis). Res_3 has been found to possess a high level of phenotypic resistance to neonicotinoids (unpublished data). Upon receipt, in 2017, in Avignon, these strains were reared on sweet pepper (Capsicum annuum) to prevent glucosinolates in the aphids’ alimentation. All 3 strains were resistant to neonicotinoids. It should be pointed out that Res_1 does not carry the R81T mutation; thus, resistance may be due to detoxification. In contrast, Res_2 and Res_3 possess the target mutation in the heterozygous and homozygous states, respectively. The former is less resistant to neonicotinoids, an observation consistent with the literature (Mottet et al. 2016).
Genotypes of the 3 lab strains of Myzus persicae resistant to synthetic pesticides from Anses Lyon
Strain . | Pyrethroids . | Carbamate . | Neonicotinoids . | |
---|---|---|---|---|
kdr mutation L1014F . | super-kdr mutation M918T/L . | MACE mutation S431F . | nAChR mutation R81T . | |
Res_1 | RS | RS | SS | SS |
Res_2 | RR | RR | SS | RS |
Res_3 | SS | RS | RS | RR |
Strain . | Pyrethroids . | Carbamate . | Neonicotinoids . | |
---|---|---|---|---|
kdr mutation L1014F . | super-kdr mutation M918T/L . | MACE mutation S431F . | nAChR mutation R81T . | |
Res_1 | RS | RS | SS | SS |
Res_2 | RR | RR | SS | RS |
Res_3 | SS | RS | RS | RR |
Genotypes of the 3 lab strains of Myzus persicae resistant to synthetic pesticides from Anses Lyon
Strain . | Pyrethroids . | Carbamate . | Neonicotinoids . | |
---|---|---|---|---|
kdr mutation L1014F . | super-kdr mutation M918T/L . | MACE mutation S431F . | nAChR mutation R81T . | |
Res_1 | RS | RS | SS | SS |
Res_2 | RR | RR | SS | RS |
Res_3 | SS | RS | RS | RR |
Strain . | Pyrethroids . | Carbamate . | Neonicotinoids . | |
---|---|---|---|---|
kdr mutation L1014F . | super-kdr mutation M918T/L . | MACE mutation S431F . | nAChR mutation R81T . | |
Res_1 | RS | RS | SS | SS |
Res_2 | RR | RR | SS | RS |
Res_3 | SS | RS | RS | RR |
The reference lab strain Susc_Ref (Table 2), considered susceptible to synthetic insecticides, has been reared in the INRAE laboratory since 2008 without insecticide selection pressure. This clone was derived from a single apterous female sampled in an untreated experimental peach orchard in Avignon (France). It was maintained on peach trees from 2008 to 2015 and then on sweet bell pepper plants, as described above, for resistant strains until the experiments.
List of Myzus persicae populations/strains tested for diCT susceptibility. Information is provided on known insecticide resistances for laboratory strains and insecticide treatments in peach orchard for wild populations in the year of field collection
Name . | Population/strain information . | City of origin (France) . |
---|---|---|
Laboratory strains | ||
Susc_Ref | Susceptible to synthetic insecticides | Avignon |
Res_1 | Resistant to neonicotinoids and pyrethroids—collected in 2011 | Saint-Martin-de-Crau |
Res_2 | Resistant to neonicotinoids and pyrethroids—collected in 2012 | Beaumont-Monteux |
Res_3 | Highly resistant to neonicotinoids, organophosphates and pyrethroids—collected in 2013 | Bésayes |
Populations | ||
Wild_1 | One treatment with azadirachtin (insect growth regulator) | Barbentane |
Wild_2 | One treatment with acetamiprid (neonicotinoid) | Jonquières |
Wild_3 | One treatment with paraffin oil (mineral oil) and one treatment with flonicamid (carboxamide) in the year before sampling | Chanas |
Wild_4 | Two treatments with paraffin oil, one treatment with acetamiprid, and one treatment with flonicamid | St Gilles |
Wild_5 | One treatment with paraffin oil | Châteauneuf sur Isère |
Wild_6 | One treatment with paraffin oil | Montfavet |
Wild_7 | One treatment with paraffin oil | Montfavet |
Name . | Population/strain information . | City of origin (France) . |
---|---|---|
Laboratory strains | ||
Susc_Ref | Susceptible to synthetic insecticides | Avignon |
Res_1 | Resistant to neonicotinoids and pyrethroids—collected in 2011 | Saint-Martin-de-Crau |
Res_2 | Resistant to neonicotinoids and pyrethroids—collected in 2012 | Beaumont-Monteux |
Res_3 | Highly resistant to neonicotinoids, organophosphates and pyrethroids—collected in 2013 | Bésayes |
Populations | ||
Wild_1 | One treatment with azadirachtin (insect growth regulator) | Barbentane |
Wild_2 | One treatment with acetamiprid (neonicotinoid) | Jonquières |
Wild_3 | One treatment with paraffin oil (mineral oil) and one treatment with flonicamid (carboxamide) in the year before sampling | Chanas |
Wild_4 | Two treatments with paraffin oil, one treatment with acetamiprid, and one treatment with flonicamid | St Gilles |
Wild_5 | One treatment with paraffin oil | Châteauneuf sur Isère |
Wild_6 | One treatment with paraffin oil | Montfavet |
Wild_7 | One treatment with paraffin oil | Montfavet |
List of Myzus persicae populations/strains tested for diCT susceptibility. Information is provided on known insecticide resistances for laboratory strains and insecticide treatments in peach orchard for wild populations in the year of field collection
Name . | Population/strain information . | City of origin (France) . |
---|---|---|
Laboratory strains | ||
Susc_Ref | Susceptible to synthetic insecticides | Avignon |
Res_1 | Resistant to neonicotinoids and pyrethroids—collected in 2011 | Saint-Martin-de-Crau |
Res_2 | Resistant to neonicotinoids and pyrethroids—collected in 2012 | Beaumont-Monteux |
Res_3 | Highly resistant to neonicotinoids, organophosphates and pyrethroids—collected in 2013 | Bésayes |
Populations | ||
Wild_1 | One treatment with azadirachtin (insect growth regulator) | Barbentane |
Wild_2 | One treatment with acetamiprid (neonicotinoid) | Jonquières |
Wild_3 | One treatment with paraffin oil (mineral oil) and one treatment with flonicamid (carboxamide) in the year before sampling | Chanas |
Wild_4 | Two treatments with paraffin oil, one treatment with acetamiprid, and one treatment with flonicamid | St Gilles |
Wild_5 | One treatment with paraffin oil | Châteauneuf sur Isère |
Wild_6 | One treatment with paraffin oil | Montfavet |
Wild_7 | One treatment with paraffin oil | Montfavet |
Name . | Population/strain information . | City of origin (France) . |
---|---|---|
Laboratory strains | ||
Susc_Ref | Susceptible to synthetic insecticides | Avignon |
Res_1 | Resistant to neonicotinoids and pyrethroids—collected in 2011 | Saint-Martin-de-Crau |
Res_2 | Resistant to neonicotinoids and pyrethroids—collected in 2012 | Beaumont-Monteux |
Res_3 | Highly resistant to neonicotinoids, organophosphates and pyrethroids—collected in 2013 | Bésayes |
Populations | ||
Wild_1 | One treatment with azadirachtin (insect growth regulator) | Barbentane |
Wild_2 | One treatment with acetamiprid (neonicotinoid) | Jonquières |
Wild_3 | One treatment with paraffin oil (mineral oil) and one treatment with flonicamid (carboxamide) in the year before sampling | Chanas |
Wild_4 | Two treatments with paraffin oil, one treatment with acetamiprid, and one treatment with flonicamid | St Gilles |
Wild_5 | One treatment with paraffin oil | Châteauneuf sur Isère |
Wild_6 | One treatment with paraffin oil | Montfavet |
Wild_7 | One treatment with paraffin oil | Montfavet |
Each of the 4 strains was mass-reared on 2 sweet bell pepper plants (C. annuum), transplanted every 20 days with 10 apterous females/plant under long photoperiod conditions (16L:8D) at 21 °C in climatic chambers. The 24-h cohort experiments required placing 25 females per plant on a new plant for 24 h to obtain an average of 50 L1 larvae of the same age within approximately 24 h. The 7 wild populations were from peach shoots collected in early spring 2017 from commercial orchards in the Rhône Valley (France). Identification of morphological criteria was carried out in the laboratory by observation under a binocular magnifier. Apterous M. persicae females from one infested shoot per orchard were placed on one sweet pepper plant for lab acclimation. While these populations might be constituted of different clones, their genetic diversity is likely to be low. In the year of collection, 3 orchards were treated with synthetic insecticides, one with azadirachtin and the others were treated once with paraffin oil (Table 2).
Myzus persicae nicotianae strains from tobacco.
Since M. persicae nicotianae developed detoxification mechanisms against a secondary plant metabolite (nicotine) to feed on tobacco, we aimed to test whether it would affect their susceptibility to diCT and 3-5diCQ. To that aim, 3 M. persicae nicotianae subspecies strains isolated from tobacco crops were also tested for their susceptibility to diCT and 3,5-diCQ. These strains, named Tob_1, Tob_2, and Tob_3, originate from Anses in Lyon (Casper Research Unit). Tob_1 and Tob_3 were sampled in June 2017 in the Drôme department (Rhône Valley), in Eymeux and Lapeyrouse Mornay, respectively. Tob_2 was sampled in early July 2017 in Saint Sorlin en Valloire, also in the Drôme. The resistance phenotypes of these strains are not known. At the genotypic level, strains Tob_1 and Tob_3 are heterozygous for the MACE mutation and homozygous for the R81T mutation in nAchR. Tob_2 is homozygous for both the MACE and R81T mutations, which is evidence of the insecticidal selection pressure these strains have been subjected to. Each of the 3 strains was a clone of a single parthenogenetic female.
Other aphid species.
Populations from commercial fields. Wild populations Nr_1 and Bb_1 of 2 other aphid species, Nasonovia ribisnigri and B. brassicae, were, respectively, obtained in commercial plots of endive (rich in diCT) and rapeseed (rich in sulfur compounds, glucosinolates). The Nr_1 colony of N. ribisnigri found on endive near Arras (France) was sent to us by an association of endive producers in 2018. Apterous females from this colony were placed on chicory plants, a plant of the same species (Cichorium intybus) as endive, on which this population acclimated quickly. Then, they were mass-reared as previously described for the M. persicae populations/strains. The Bb_1 colony of B. brassicae was collected in a rapeseed field at the INRAE Research Centre in Avignon (France) in 2018. We maintained this colony on rapeseed under the same conditions as the other populations.
Populations from an experimental aphid-trap plot.
An experimental plot was set up at INRAE Avignon, including 5 species of plants rich in 3,5-diCQ or diCT: sweet potato (Ipomoea batatas, 3,5-diCQ), wild chicory and endive (C. intybus, diCT), peanut (Arachis hypogaea, diCT), and dandelion (Taraxacum sp., diCT) to trap aphids that settled on these plants. This experiment was carried out over 20 years (2018 and 2019), during which a survey for the presence of aphid colonies on these plants was performed twice a week. Aphid colonies were collected individually and reared in the laboratory on the same plant species as they were trapped. Only one aphid colony collected in September 2018 from peanut plants acclimated well to the rearing conditions and to the Ap3 medium for biotests (see below). The species was morphologically identified as Aphis craccivora Koch, 1854 by Armelle Coeur d’Acier, aphid systematicist at INRAE CBGP (Montpellier, France), and the population was named Ac_1.
Plants
All the plants used for aphid rearing and cohort production and experimental aphid-trap plots were previously grown in potting soil in a greenhouse without using biopesticides. Sweet peppers belonged to the ‘Yellow wonder’ cultivar; peanut seeds were bought at a food store and came from Togo; the dandelion seeds were of the ‘Pissenlit à cœur plein amélioré’ cultivar (Vilmorin); chicory seeds belonged to 2 cultivars: ‘Chicorée sauvage barbe de capucin’ (Caillard) and ‘Chicorée Witloof de Bruxelles’ (endive, Vilmorin); sweet potato was a red-flesh accession from INRAE germplasm (accession D) propagated by cuttings. The diCT and 3,5-diCQ contents of these species and cultivars were measured in different parts (developing and mature leaves and stems) of the plants by HPLC. Plants were grown in a greenhouse under the same conditions as those used for experimental trap plots.
Methods
Biotests. The toxicity of diCT (Merck, cas n° 6537-80-0) and/or 3,5-diCQ (Merck, cas n°2450-53-5) on aphid populations/strains was evaluated by biotests on the Ap3 synthetic diet (Febvay et al. 1988), except for N. ribisnigri that did not tolerate Ap3, but was more tolerant of the “Mittler and Dadd” medium (Mittler and Dadd 1964). The active ingredient was mixed with the appropriate medium at increasing concentrations (0.125, 0.250, 0.5, 1, and 2 mM) on which L1 larvae from the same 24-h cohort were installed. This range of concentrations was chosen because it induced a gradual response of mortalities ranging from 0 to 100% on the reference strain Susc_Ref. The control group was fed an artificial medium lacking any active substances. A biotest is performed on at least 240 individuals in 2 experiments. The individuals were placed in groups of 5 on disks containing artificial diet, between 2 layers of parafilm (Supplementary Fig. S1).
The mortality of aphids was estimated 48 h after the beginning of the test. The number of aphids tested per concentration depended on the fecundity of each population or strain. Since biotest results are variable over time, and all aphids were unavailable at the same time, tests on the reference strain Susc_Ref were performed simultaneously and under the same conditions as the tested strains/populations. The mortality rate of the control group reached 8.6%.
Enzymatic Activities and Protein Dosage
The activities of 2 families of enzymes known to be involved in detoxification in aphids were assayed: carboxylesterases (ESTs) and glutathione-S-transferases (GSTs) in populations/strains of M. persicae collected from peach and the reference strain Susc_Ref.
Specific activities of EST and GST enzyme families were measured on individuals from the same populations as those tested by biotests. The assays of these 2 enzyme families were conducted on the same protein extracts obtained by grinding a pool of 10 apterous female adults aged 10 days in 100 µl of Hepes buffer (50 mM, pH 7.0). These crude extracts were centrifuged at 15,000 × g for 15 min at 4 °C to remove the cell residues. Ninety microliters of supernatant were collected, constituting the protein extract used for the assays. All these steps were performed on ice. The total protein concentrations were determined in triplicate by the Bradford method (Bradford 1976). For each sample, the specific activity was calculated as the ratio of the quantity of product per minute over its total quantity of protein (mg of protein). Extracts that were not analyzed immediately were stored at −80 °C. They were defrosted only once before measuring enzymatic activity. All activities were measured on 2 days to limit bias. α-Naphthyl acetate and 2,4-dinitrochlorobenzene (DNCB) were chosen as the substrates for the ESTs and GSTs. Detailed protocols were previously described in the study of Siegwart et al. (2011).
Statistical Analysis
Statistical analyses were performed with R 4.1.1 software and the RStudio 1.3.1093 interface (R Core 2013).
The resistance level of populations/strains was characterized using probit analyses (drm function), LC calculations (ED function), and LC comparisons (Edcomp function) performed with the drc package (Ritz et al. 2015). The dispersion of model residuals was inspected with a quantile-quantile (QQ) plot (qqnorm function) of the standardized residuals, and their coherence was observed with the resid function of drc. The correct fit of the model was checked with the modelFit function of the same package.
A comparison of enzymatic activities among populations/strains was performed using a linear model that included population/strain and total protein amount as fixed explanatory variables (R package lme4 [Bates et al. 2015]). Esterase activity was log-transformed to improve model fit. The significance of the effects was tested with Wald χ2 tests (ANOVA in the car package [Fox and Weisberg 2018]). The dispersion of the model residuals was inspected with a quantile–quantile (QQ) plot of the standardized residuals, and their uniformity and outliers were inspected with a plot of the residuals against the predicted values. Associated statistical tests were also performed (R package DHARMa [Hartig 2019]).
Assessment of possible enzymatic activity in resistance was analyzed using a linear mixed model that included enzymatic activity as the dependent variable, LC50 and total protein content as fixed explanatory variables and population as a random factor (R package lme4 [Bates et al. 2015]). Analysis of results and residuals was performed as above.
Results
Variation in Susceptibility to diCT and Enzymatic Activity for M. persicae Aphids From Peach Orchards
The concentration of diCT in the diet needed to kill 50% of the individuals in a M. persicae population was low. The LC50 values (median lethal concentrations) ranged from 0.17 mM for Wild_6 to 0.61 mM for Wild_2 (Table 3 and Supplementary Table S1). There were significant differences in susceptibility to diCT among populations and strains; however, the LC50 ratio (RR50) between the least and most susceptible populations, that is, Wild_6 and Wild_2, only reached 3.59. We observe a slight continuous variation in sensitivity to diCT between genotypes without the breakdown that characterizes resistance. The 3 strains with characterized resistances to synthetic insecticides were sensitive to diCT.
Susceptibility to diCT of Myzus persicae L1 larvae from 10 strains/populations and the reference strain Susc_Ref. Resistance ratios (RR50) are based on the most susceptible population (Wild_6)
Pop/strain . | Number of aphids . | LC50 (mM) ± CI95 . | RR50 . | GST activity . | EST activity . | Protein concentration . |
---|---|---|---|---|---|---|
Wild_6 | 540 | 0.17a ± 0.01 | 1.00 | 377.83 ± 80.68bd | 304.15 ± 49.47cd | 0.74 ± 0.09ab |
Wild_4 | 360 | 0.18a ± 0.01 | 1.06 | 410.59 ± 59.20bcd | 449.70 ± 70.05bd | 0.64 ± 0.14ab |
Wild_5 | 396 | 0.21ab ± 0.02 | 1.24 | 518.23 ± 51.75ad | 518.62 ± 90.24bd | 0.57 ± 0.11a |
Res_3 | 306 | 0.23bc ± 0.02 | 1.35 | 467.86 ± 40.01ac | 489.70 ± 111.04b | 0.90 ± 0.10ac |
Res_1 | 300 | 0.24b ± 0.03 | 1.41 | 528.72 ± 49.03a | 387.76 ± 57.67bd | 0.65 ± 0.21ac |
Wild_3 | 240 | 0.25c ± 0.02 | 1.47 | 364.54 ± 36.99bcd | 233.02 ± 81.92ac | 0.85 ± 0.14ac |
Res_2 | 300 | 0.27bd ± 0.02 | 1.59 | 470.77 ± 28.14ab | 339.45 ± 51.31cd | 0.60 ± 0.10ab |
Susc_Ref | 800 | 0.30e ± 0.02 | 1.76 | 185.98 ± 26.64ab | 125.88 ± 15.76bc | 1.66 ± 0.19e |
Wild_7 | 420 | 0.34ef ± 0.02 | 2.00 | 271.08 ± 16.41ab | 138.39 ± 29.35cd | 1.42 ± 0.13de |
Wild_1 | 294 | 0.38ef ± 0.03 | 2.23 | 311.68 ± 41.92b | 197.86 ± 31.81c | 0.95 ± 0.11bc |
Wild_2 | 306 | 0.61f ± 0.04 | 3.59 | 323.44 ± 36.51bcd | 117.12 ± 28.74a | 1.07 ± 011cd |
Pop/strain . | Number of aphids . | LC50 (mM) ± CI95 . | RR50 . | GST activity . | EST activity . | Protein concentration . |
---|---|---|---|---|---|---|
Wild_6 | 540 | 0.17a ± 0.01 | 1.00 | 377.83 ± 80.68bd | 304.15 ± 49.47cd | 0.74 ± 0.09ab |
Wild_4 | 360 | 0.18a ± 0.01 | 1.06 | 410.59 ± 59.20bcd | 449.70 ± 70.05bd | 0.64 ± 0.14ab |
Wild_5 | 396 | 0.21ab ± 0.02 | 1.24 | 518.23 ± 51.75ad | 518.62 ± 90.24bd | 0.57 ± 0.11a |
Res_3 | 306 | 0.23bc ± 0.02 | 1.35 | 467.86 ± 40.01ac | 489.70 ± 111.04b | 0.90 ± 0.10ac |
Res_1 | 300 | 0.24b ± 0.03 | 1.41 | 528.72 ± 49.03a | 387.76 ± 57.67bd | 0.65 ± 0.21ac |
Wild_3 | 240 | 0.25c ± 0.02 | 1.47 | 364.54 ± 36.99bcd | 233.02 ± 81.92ac | 0.85 ± 0.14ac |
Res_2 | 300 | 0.27bd ± 0.02 | 1.59 | 470.77 ± 28.14ab | 339.45 ± 51.31cd | 0.60 ± 0.10ab |
Susc_Ref | 800 | 0.30e ± 0.02 | 1.76 | 185.98 ± 26.64ab | 125.88 ± 15.76bc | 1.66 ± 0.19e |
Wild_7 | 420 | 0.34ef ± 0.02 | 2.00 | 271.08 ± 16.41ab | 138.39 ± 29.35cd | 1.42 ± 0.13de |
Wild_1 | 294 | 0.38ef ± 0.03 | 2.23 | 311.68 ± 41.92b | 197.86 ± 31.81c | 0.95 ± 0.11bc |
Wild_2 | 306 | 0.61f ± 0.04 | 3.59 | 323.44 ± 36.51bcd | 117.12 ± 28.74a | 1.07 ± 011cd |
Glutathion-S-transferase (GST) activities are given in mu/min/mg protein, carboxylesterase (EST) activities are given in nmol of α-Naphtol/min/mg protein and protein concentration in µg/µl. Letters near enzymatic activities indicate whether populations had significantly different specific enzyme activities in a model, including protein dosage as a covariable.
Susceptibility to diCT of Myzus persicae L1 larvae from 10 strains/populations and the reference strain Susc_Ref. Resistance ratios (RR50) are based on the most susceptible population (Wild_6)
Pop/strain . | Number of aphids . | LC50 (mM) ± CI95 . | RR50 . | GST activity . | EST activity . | Protein concentration . |
---|---|---|---|---|---|---|
Wild_6 | 540 | 0.17a ± 0.01 | 1.00 | 377.83 ± 80.68bd | 304.15 ± 49.47cd | 0.74 ± 0.09ab |
Wild_4 | 360 | 0.18a ± 0.01 | 1.06 | 410.59 ± 59.20bcd | 449.70 ± 70.05bd | 0.64 ± 0.14ab |
Wild_5 | 396 | 0.21ab ± 0.02 | 1.24 | 518.23 ± 51.75ad | 518.62 ± 90.24bd | 0.57 ± 0.11a |
Res_3 | 306 | 0.23bc ± 0.02 | 1.35 | 467.86 ± 40.01ac | 489.70 ± 111.04b | 0.90 ± 0.10ac |
Res_1 | 300 | 0.24b ± 0.03 | 1.41 | 528.72 ± 49.03a | 387.76 ± 57.67bd | 0.65 ± 0.21ac |
Wild_3 | 240 | 0.25c ± 0.02 | 1.47 | 364.54 ± 36.99bcd | 233.02 ± 81.92ac | 0.85 ± 0.14ac |
Res_2 | 300 | 0.27bd ± 0.02 | 1.59 | 470.77 ± 28.14ab | 339.45 ± 51.31cd | 0.60 ± 0.10ab |
Susc_Ref | 800 | 0.30e ± 0.02 | 1.76 | 185.98 ± 26.64ab | 125.88 ± 15.76bc | 1.66 ± 0.19e |
Wild_7 | 420 | 0.34ef ± 0.02 | 2.00 | 271.08 ± 16.41ab | 138.39 ± 29.35cd | 1.42 ± 0.13de |
Wild_1 | 294 | 0.38ef ± 0.03 | 2.23 | 311.68 ± 41.92b | 197.86 ± 31.81c | 0.95 ± 0.11bc |
Wild_2 | 306 | 0.61f ± 0.04 | 3.59 | 323.44 ± 36.51bcd | 117.12 ± 28.74a | 1.07 ± 011cd |
Pop/strain . | Number of aphids . | LC50 (mM) ± CI95 . | RR50 . | GST activity . | EST activity . | Protein concentration . |
---|---|---|---|---|---|---|
Wild_6 | 540 | 0.17a ± 0.01 | 1.00 | 377.83 ± 80.68bd | 304.15 ± 49.47cd | 0.74 ± 0.09ab |
Wild_4 | 360 | 0.18a ± 0.01 | 1.06 | 410.59 ± 59.20bcd | 449.70 ± 70.05bd | 0.64 ± 0.14ab |
Wild_5 | 396 | 0.21ab ± 0.02 | 1.24 | 518.23 ± 51.75ad | 518.62 ± 90.24bd | 0.57 ± 0.11a |
Res_3 | 306 | 0.23bc ± 0.02 | 1.35 | 467.86 ± 40.01ac | 489.70 ± 111.04b | 0.90 ± 0.10ac |
Res_1 | 300 | 0.24b ± 0.03 | 1.41 | 528.72 ± 49.03a | 387.76 ± 57.67bd | 0.65 ± 0.21ac |
Wild_3 | 240 | 0.25c ± 0.02 | 1.47 | 364.54 ± 36.99bcd | 233.02 ± 81.92ac | 0.85 ± 0.14ac |
Res_2 | 300 | 0.27bd ± 0.02 | 1.59 | 470.77 ± 28.14ab | 339.45 ± 51.31cd | 0.60 ± 0.10ab |
Susc_Ref | 800 | 0.30e ± 0.02 | 1.76 | 185.98 ± 26.64ab | 125.88 ± 15.76bc | 1.66 ± 0.19e |
Wild_7 | 420 | 0.34ef ± 0.02 | 2.00 | 271.08 ± 16.41ab | 138.39 ± 29.35cd | 1.42 ± 0.13de |
Wild_1 | 294 | 0.38ef ± 0.03 | 2.23 | 311.68 ± 41.92b | 197.86 ± 31.81c | 0.95 ± 0.11bc |
Wild_2 | 306 | 0.61f ± 0.04 | 3.59 | 323.44 ± 36.51bcd | 117.12 ± 28.74a | 1.07 ± 011cd |
Glutathion-S-transferase (GST) activities are given in mu/min/mg protein, carboxylesterase (EST) activities are given in nmol of α-Naphtol/min/mg protein and protein concentration in µg/µl. Letters near enzymatic activities indicate whether populations had significantly different specific enzyme activities in a model, including protein dosage as a covariable.
Variations in GST or EST activities were continuous within the panel of populations or strains tested. The levels of specific activity differed statistically depending on the populations or strains (for GST: F = 4.11 and P < 0.0001 and EST: F = 9.90 and P < 0.0001) (Table 3).
There was more interpopulation variation for EST activities than for GST, with a ratio of 4.4 vs. 2.8, respectively, between the lowest and highest median activities. The 3 strains Res_1, Res_2, and Res_3 had higher EST activities than those of the Wild_2 population, which was the least diCT susceptible. Strains Res_1 and Res_3 had significantly higher GST activities than the Wild_1, Wild_2, Wild_3, Wild_4, and Wild_6 populations. The reference strain Susc_Ref and Wild_5, Wild_7, and Res_2 had intermediate GST activities (Table 3).
The total protein extracted from 10 individuals differed greatly and significantly among the aphid populations or strains tested (F = 5.33; P = 1.2 × 10−6) (Table 3). We extracted 2.9 times more protein from Susc_Ref than from Wild_5.
Analysis of the relationship between diCT sensitivity (measured by LC50) and the level of GST activity showed no relationship (χ2 = 0.50; df = 1, P = 0.48). On the other hand, the same analysis shows a weak negative relationship between GST activity and diCT sensitivity (χ2 = 5.034; df = 1, P = 0.025). Finally, there was a highly significant positive correlation (F = 14.10; df = 1; P = 0.00027) between protein quantity and diCT sensitivity.
Variations in diCT and 3,5-diCQ Susceptibility and Enzymatic Activities of M. persicae nicotianae Strains
Although M. persicae nicotianae clones are capable of detoxifying nicotine found in tobacco plants, we found no indication of reduced susceptibility of the 3 M. persicae clones from tobacco to diCT and 3,5-diCQ. The LC50 values were similar to the reference strain Susc_Ref, and none of the pairwise comparisons were significant (Fig. 1 and Supplementary Table S2).
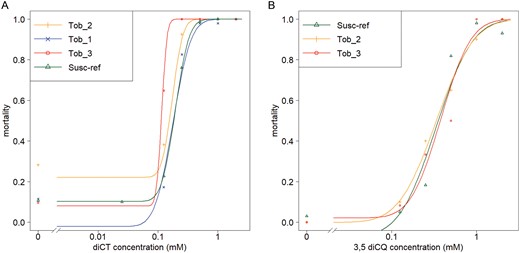
Dose–response curves A) to diCT and B) to 3,5-diCQ of Myzus persicae nicotianae strains (collected from tobacco crops) compared to the reference strain Susc_Ref.
EST activity did not differ among these strains, or Susc_Ref, the susceptible reference strain (df = 2; F = 2.91; P = 0.067). In contrast, small but significant differences were detected among these strains for GST activity (df = 2; F = 29.2; P = 2.4 × 10-8). Tob_1 and Tob_2 had similar activities that were 1.7 (for Tob_1) and 1.4 (for Tob_2) times lower than Susc_Ref (pairwise comparisons: Tob_1—Susc_Ref: t = −4.519 and P < 0.001; Tob_2—Susc_Ref: t = −6.554 and P < 0.001; Tob_2—Tob_1: t = −1.748 and P = 0.194) (Table 4).
Enzymatic activities and protein concentration in 5 populations or strain of aphids
Species . | Pop/strain . | GST activity . | EST activity . | Protein concentration . |
---|---|---|---|---|
Myzus persicae | Susc_Ref | 185.98 ± 26.64 | 125.88 ± 15.76 | 1.66 ± 0.19 |
Aphis craccivora | Ac_1 | 105.21 ± 9.73ns | 49.45 ± 4.46*** | 0.60 ± 0.03* |
Nasonovia ribisnigri | Nr_1 | 111.28 ± 7.70ns | 42.96 ± 1.58*** | 1.36 ± 0.17ns |
M. persicae nicotiniae | Tob_1 | 107.94 ± 9.30*** | 127.57 ± 8.07ns | 1.01 ± 0.02ns |
M. persicae nicotiniae | Tob_2 | 133.47 ± 5.27*** | 135.40 ± 5.32ns | 0.65 ± 0.02ns |
Species . | Pop/strain . | GST activity . | EST activity . | Protein concentration . |
---|---|---|---|---|
Myzus persicae | Susc_Ref | 185.98 ± 26.64 | 125.88 ± 15.76 | 1.66 ± 0.19 |
Aphis craccivora | Ac_1 | 105.21 ± 9.73ns | 49.45 ± 4.46*** | 0.60 ± 0.03* |
Nasonovia ribisnigri | Nr_1 | 111.28 ± 7.70ns | 42.96 ± 1.58*** | 1.36 ± 0.17ns |
M. persicae nicotiniae | Tob_1 | 107.94 ± 9.30*** | 127.57 ± 8.07ns | 1.01 ± 0.02ns |
M. persicae nicotiniae | Tob_2 | 133.47 ± 5.27*** | 135.40 ± 5.32ns | 0.65 ± 0.02ns |
Glutathion-S-transferase (GST) activities are given in mu/min/mg protein; carboxylesterase (EST) activities are given in nmol of α-Naphtol/min/mg protein and protein concentration in µg/µl.
Statistical analysis shows comparisons to the susceptible strain (Susc_Ref) by taking into account the protein concentration effect: *P < 0.05; **P < 0.01; ***P < 0.001.
Enzymatic activities and protein concentration in 5 populations or strain of aphids
Species . | Pop/strain . | GST activity . | EST activity . | Protein concentration . |
---|---|---|---|---|
Myzus persicae | Susc_Ref | 185.98 ± 26.64 | 125.88 ± 15.76 | 1.66 ± 0.19 |
Aphis craccivora | Ac_1 | 105.21 ± 9.73ns | 49.45 ± 4.46*** | 0.60 ± 0.03* |
Nasonovia ribisnigri | Nr_1 | 111.28 ± 7.70ns | 42.96 ± 1.58*** | 1.36 ± 0.17ns |
M. persicae nicotiniae | Tob_1 | 107.94 ± 9.30*** | 127.57 ± 8.07ns | 1.01 ± 0.02ns |
M. persicae nicotiniae | Tob_2 | 133.47 ± 5.27*** | 135.40 ± 5.32ns | 0.65 ± 0.02ns |
Species . | Pop/strain . | GST activity . | EST activity . | Protein concentration . |
---|---|---|---|---|
Myzus persicae | Susc_Ref | 185.98 ± 26.64 | 125.88 ± 15.76 | 1.66 ± 0.19 |
Aphis craccivora | Ac_1 | 105.21 ± 9.73ns | 49.45 ± 4.46*** | 0.60 ± 0.03* |
Nasonovia ribisnigri | Nr_1 | 111.28 ± 7.70ns | 42.96 ± 1.58*** | 1.36 ± 0.17ns |
M. persicae nicotiniae | Tob_1 | 107.94 ± 9.30*** | 127.57 ± 8.07ns | 1.01 ± 0.02ns |
M. persicae nicotiniae | Tob_2 | 133.47 ± 5.27*** | 135.40 ± 5.32ns | 0.65 ± 0.02ns |
Glutathion-S-transferase (GST) activities are given in mu/min/mg protein; carboxylesterase (EST) activities are given in nmol of α-Naphtol/min/mg protein and protein concentration in µg/µl.
Statistical analysis shows comparisons to the susceptible strain (Susc_Ref) by taking into account the protein concentration effect: *P < 0.05; **P < 0.01; ***P < 0.001.
Variation in Susceptibility to diCT and 3,5-diCQ and Enzymatic Activity of Other Aphid Species
The results of our aphid trapping give a total of 11 aphid colonies observed on the field experimental aphid-trap plot including 5 on peanut plants belonging to the species A. craccivora (2), Acyrthosiphon pisum (1), and Aphis fabae (2), 2 on chicory (A. fabae), 1 on sweet potato (Macrosiphum euphorbiae), and 2 on dandelion (Aphis taraxacicola).
Only one population obtained from the trap plots could be reared in the laboratory, the Ac_1 (A. craccivora) population, which was collected on peanuts and tested for susceptibility to diCT and 3,5-diCQ. It was compared to 2 populations of other species, Nr_1 (N. ribisnigri sampled from endive, rich in diCT) and Bb_1 (B. brassicae sampled from rapeseed, rich in glucosinolates) and to the Susc_Ref M. persicae reference clone. The results of the biotests were much more contrasted than those obtained with the M. persicae strains and populations (Fig. 2). Individuals from the Ac_1 population showed an apparent ability to resist both active ingredients to the extent that LC50 values were not calculable with the range of active ingredients used in the biotests. In contrasts, individuals from Bb_1 were very susceptible to 3,5-diCQ (LC50 Bb_1 = 0.09 mM; LC50 Susc_Ref = 0.31; EDcomp Susc_Ref vs Bb_1 P < 10−5) and individuals from the Nr_1 population showed the same susceptibility to diCT as the reference M. persicae strain (LC50 Nr_1 = 0.19; LC50 Susc_Ref = 0.21; EDcomp Susc_Ref vs Nr_1 P = 0.96).
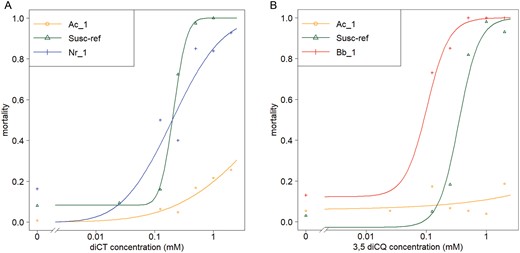
Dose–response curves for A) diCT, B) 3,5-diCQ of populations of 3 aphid species (Aphis craccivora, Nasonovia ribisnigri collected from plants rich in either compound, and Brevicoryne brassicae collected on plants rich in glucosinolates), compared to the M. persicae reference strain Susc_Ref.
GST activities of both Ac_1 and Nr_1 populations were similar to that of the reference strain Susc_Ref (df = 2; F = 2.23; P = 0.123). In contrast, significant differences were detected among populations for EST activities (df = 2; F = 57.15; P = 2.7 × 10−11). The 2 populations belonging to species other than M. persicae had similar EST activities, on average 2.5 (Ac_1) and 2.9 (Nr_1) times lower than Susc_Ref activity (pairwise comparisons: Ac_1—Susc_Ref: t = −5.82 and P < 0.001; Nr_1—Susc_Ref: t = −8.72 and P < 0.001; Nr_1—Ac_1: t = −1.33 and P = 0.371) (Table 4).
The individuals of Ac_1 had lower protein quantity than those of Susc-ref and Nr_1 (Nr_1—Susc_Ref: t = −1.54 and P = 0.274; Nr_1—Ac_1: t = 2.53 and P = 0.040; Susc_Ref—Ac_1: t = 2.91 and P = 0.016) (Table 4).
Discussion
The objective of this study was to investigate (i) whether cross-resistance to botanical aphicides diCT/3,5-diCQ could be detected in M persicae strains resistant to synthetic insecticides or in M. persicae nicotianae subspecies that has developed detoxification mechanisms to feed on tobacco plants and (ii) whether there was diversity in susceptibility to diCT/3,5-diCQ in wild M. persicae populations and in other aphid species trapped on plants rich in diCT/3,5-diCQ or glucosinolates. We also hypothesized that detoxification would be the most likely mechanism of adaptation and measured the activities of the GST and EST enzyme families involved.
Our results did not show any cross-resistance between diCT/3,5-diCQ and synthetic insecticides in 3 strains of M. persicae highly resistant to pyrethroids, Ops, and neonicotinoids. We observed a slight and continuous variation in diCT susceptibility among the tested M. persicae from peach and tobacco crops. This slight variation correlated well with the amount of protein extracted from aphids of the different populations. A population of A. craccivora trapped on a peanut plant showed complete insensitivity to diCT and 3,5-diCQ in the range of concentrations tested in contrast to the B. brassicae and N. ribisnigri populations tested. Lastly, our results do not support the initial hypothesis of a detoxification mechanism by ESTs or GSTs because their activities did not correlate with variations in diCT/3,5-diCQ susceptibility.
The 3 strains of M. persicae resistant to synthetic insecticides were more susceptible to diCT than the reference strain, which showed no resistance to these insecticides, indicating the absence of cross-resistance to diCT and the prominent families of synthetic insecticides already on the market. Cross-resistance involving detoxification primarily not only due to CYPs but also GSTs and ESTs is also well documented and has been reviewed by Li et al. (2007). Our results tend to show that the molecular target of diCT is different from those of already marketed synthetic molecules and that the detoxification tools acquired by strains resistant to current pesticides are not adapted to modify diCT.
Insect adaptation to a toxic host appears to promote the development of resistance (Alyokhin and Chen 2017). We found some indication that aphids feeding on a diCT-rich species may be insensitive to diCT or 3,5-diCQ. The experimental plot, composed of species rich in diCT and 3,5-diCQ, trapped a population of A. craccivora, which proved resistant to these compounds at the tested doses. This was the first time we observed aphids surviving a 2 mM dose of these molecules. This discovery suggests that the mode of action of diCT and 3,5-diCQ might be very close, which was expected given their molecular similarity. Aphis craccivora, known as the black legume aphid, cowpea aphid, or groundnut aphid, is a very polyphagous species with a preference for Fabaceae including peanut (Blackman et al. 2007). There is genetic and morphological evidence for the existence of host races in this species (Takahashi 1966, Coeur d’Acier et al. 2007). Aphis craccivora is also known to be infected by facultative endosymbionts (Russell et al. 2003), and the nature of these endosymbionts depends on the host plant on which it feeds (Brady and White 2013). Overall, genetic diversity is low within this species due to its almost exclusively parthenogenetic mode of reproduction (Wongsa et al. 2017), suggesting that plant host adaptation is unlikely genetic. It is, therefore, possible to hypothesize that the ability of some A. craccivora to feed on diCT-rich plants comes from the detoxification ability of their endosymbionts, as is the case in nicotine-resistant M. persicae clones (Singh et al. 2020). However, since we only tested one population of A. craccivora, it is unclear whether this insensitivity is generalized to this species or whether there are biotypes susceptible to diCT/3,5-diCQ.
We found no indication that the GST and EST enzyme families were responsible for the slight variation in susceptibility to diCT. As for susceptibility to diCT in biotests, we observed a continuum of GST and EST activity levels among the tested populations. Sensitivity to diCT was not correlated with the level of GST activity and was weakly negatively correlated with EST activity. We observed similar results on the A. craccivora population with no difference in GST activity and significantly lower EST activities than the susceptible M. persicae reference clone Susc_Ref. It would have been interesting to determine P450 enzyme activity since this enzyme family plays an important role in xenobiotic detoxification in M. persicae (Bass et al. 2014). However, unlike other enzyme families, the activity of P450 is very challenging to measure. Despite several attempts, we have not been able to develop a robust protocol to measure P450 activity on the M. persicae samples. Nevertheless, strain Res_1 is resistant to neonicotinoids without target mutation, so it possibly carries a variety of other resistance mechanisms, including detoxification that may involve CYPs (Puinean et al. 2010). This strain is highly sensitive to diCT. The CYPs expressed by this strain would therefore not be sufficient to resist diCT.
We observed a continuous variation in susceptibility to diCT. The populations or strains of M. persicae with the highest total protein content per individual were also the least susceptible to diCT, although not with a large resistance ratio. This difference in protein quantities per aphid may be explained by variations in size and/or the number of embryos per female (Raikhel and Dhadialla 1992) between strains or populations. Protein percentage content may be an indicator of individual fitness and their propensity to be more fecund, as observed in another aphid species (Ahsaei et al. 2013). If the total amount of protein extracted is a proxy of the size of the aphids, their lower sensitivity to diCT could, therefore, be explained by a dilution effect of the toxic in the pest’s body. The minor fluctuations in susceptibility observed in the initial phase of this study may result from the diminished capacity of individuals to respond to stress, due to minor genes, in the absence of robust resistance mechanisms. However, outputs from population genetic models simulate that major genes dominate responses to selection for resistance across a wide range of selection pressure intensities, and a literature review supports similar conclusions (Groeters and Tabashnik 2000). Therefore, it is unlikely that the low effect, correlated with the total protein content of individuals, observed here could evolve into actual resistance if this product were to be used in the field. Indeed, upon comparing the total protein levels in individuals from the A. craccivora population showing complete insensitivity to diCT and 3,5-diCQ with those of the reference strain, no discernible difference was noted. This suggests the presence of alternative potent mechanisms, such as molecular target modification, which effectively overshadow the minor sensitivity reduction mechanism described earlier in this paragraph.
We have undertaken this study in the context of the development of diCT and 3,5-diCQ as bioinsecticides against aphids. The perspective of their use in agriculture raises the question of their sustainability. Our objective was to determine whether, in M. persicae, existing resistance to synthetic insecticides due to detoxification mechanisms could lead to cross-resistance to diCT and 3,5-diCQ. We also aimed to determine whether the presence of diCT and 3,5-diCQ at high levels in a number of plant species had led to adaptation to these compounds in certain aphid species.
We did not detect high enough insensitivity to diCT to be considered as resistance in practice in any of the M. persicae populations or strains tested. However, we observed a continuum of susceptibility with significant differences between extremes of up to 3.59-fold at the LC50 (RR50) between populations or strains of M. persicae from peach. These differences observed in a small sample of 14 populations or strains raise questions about the possible existence or evolution of less sensitive populations in nature. Thus, a selection may occur over generations to give rise to a fully resistant population, especially since hormetic phenomena, a favorable stimulation response following exposure to low doses of toxins, may occur in the survivors, as already observed in this species for azadirachtin, another botanical molecule, and synthetic molecules (Cutler et al. 2009). This information must be considered in the recommendations for using the commercial product to propose a dose of use high enough to avoid these pitfalls.
Supplementary Material
Supplementary material is available at Journal of Economic Entomology online.
Acknowledgments
We are very grateful to the French Ministries of Agriculture and Food and Ecological and Solidarity Transition for financial support under its Ecophyto program (Dicabio project). We warmly thank Claire Dufour (INRAE—SQPOV unit) for her valuable advice, Marie-Noëlle Corre for HPLC analyses of 3,5-diCQ and diCT in host plants and AHM unit for the cultivation of experimental plots. Special thanks to Benoit Barres and Christophe Plantamp (Anses—CASPER Unit) for providing insecticide-resistant Myzus persicae clones.
Author Contributions
Myriam Siegwart (Conceptualization [Equal], Data curation [Supporting], Formal analysis [Supporting], Investigation [Supporting], Methodology [Equal], Project administration [Equal], Supervision [Equal], Writing—original draft [Supporting], Writing—review & editing [Equal]), Bertrand Gauffre (Writing—review & editing [Equal]), Elodie Lecerf (Methodology [Supporting]), and Jean-Luc Poëssel (Conceptualization [Equal], Funding acquisition [Supporting], Supervision [Equal], Writing—review & editing [Equal])