-
PDF
- Split View
-
Views
-
Cite
Cite
Jedeliza B Ferrater, Francesc Gómez-Marco, Andrew K Yoshimoto, Thomas D Greene, Gregory S Simmons, Matthew P Daugherty, Paul F Rugman-Jones, Development of a sterile insect technique as a control strategy for the Asian citrus psyllid: establishing the effect of sterilizing X-rays on fecundity, fertility, and survival, Journal of Economic Entomology, Volume 117, Issue 4, August 2024, Pages 1356–1366, https://doi.org/10.1093/jee/toae098
- Share Icon Share
Abstract
The Asian citrus psyllid (ACP), Diaphorina citri Kuwayama (Hemiptera: Psyllidae), is a major pest of citrus due to its role as the vector of the bacterium that causes huanglongbing. In commercial citrus, ACP control currently relies on the application of insecticides, which may not be sustainable long-term, nor practical in urban areas. The sterile insect technique (SIT) is an alternative strategy in which large numbers of pests are reared, sterilized using radiation, and then released into the field to compete with wild individuals for matings, suppressing population growth. As a fundamental step toward the development of SIT for ACP, this study sought to identify the optimum radiation dose required to sterilize ACP without affecting their survival and mating capacity. Virgin adult ACP of both sexes were subjected to doses of X-ray irradiation ranging from 40 to 480 Gy, then paired with a nonirradiated mate and allowed to produce offspring. Fecundity was estimated as the number of eggs laid, and fertility as the proportion of those eggs that hatched. Females were more radio-sensitive than males, exhibiting a major drop in fecundity at even the lowest dose and 100% sterility at 80 Gy. In contrast, a fivefold higher dose (400 Gy) did not achieve complete sterility in males, with around 5% offspring survival. However, F1 progeny of males exposed to 320 Gy or higher were subsequently found to be 100% sterile. This confirmation of inherited sterility suggests that balancing the sterilizing effects of radiation against its mortality-inducing effects may warrant further evaluation.
Introduction
The Asian citrus psyllid (ACP), Diaphorina citri Kuwayama (Hemiptera: Psyllidae), is considered to be the most serious threat to global citrus production because it transmits the bacterium, Candidatus Liberibacter asiaticus (CLas), associated with the highly destructive citrus greening disease, huanglongbing (HLB; Halbert and Manjunath 2004, Bové 2006, Li et al. 2021). Following infection, trees eventually produce unmarketable fruits and subsequently die within 5–10 years (Grafton-Cardwell et al. 2013). This has resulted in severe economic impacts to the citrus industry worldwide (FAO 2015, Rwomushana et al. 2017, Dala-Paula et al. 2019, Britt et al. 2020, Pazolini et al. 2021, Garcia-Figuera et al. 2022).
To date, there are no known treatments for HLB as part of existing integrated pest management programs (da Graça et al. 2016, Li et al. 2021). As a result, much effort has been directed towards control of the vector to limit disease incidence. In Florida, where ACP is already well established in commercial citrus, chemical control, noninsecticidal control (exclusion mesh and individual protective covers), and biological control are commonly applied strategies to suppress ACP numbers (Diepenbrock et al. 2023). In California, where ACP is not yet widely established in commercial orchards, a strategy of preclusion and eradication of ACP is currently pursued via the area-wide application of conventional and organic insecticides during periods of the year when citrus flush (the new soft shoots that ACP prefer to feed and lay eggs on) is most abundant (Grafton-Cardwell et al. 2013, Boina and Bloomquist 2015, Li et al. 2021, Garcia-Figuera et al. 2022, Milosavljević et al. 2022, UC ANR Asian Citrus Psyllid Distribution and Management 2022). However, for reasons of cost, mitigation of the development of insecticide resistance, and maintenance of integrated pest management (IPM) programs for other key citrus pests, it is foreseen that long-term sustainable area-wide management of ACP will require the incorporation of additional tools and control strategies (Hall and Gottwald 2011). Furthermore, in ACP-infested areas outside of commercial citrus production, the application of conventional insecticides is impractical, because of the size of the area requiring treatment, challenges relating to the use of pesticides in urban areas (e.g., homeowners preventing access to residential trees; Hoddle and Pandey, 2014), and public and government pressure to reduce (and even remove) pesticide use. In urban areas of California, an alternative involving classical biological control releases of an imported parasitoid wasp, Tamarixia radiata (Hymenoptera: Eulophidae), is already employed to control “reservoir” ACP populations on residential citrus that might otherwise act as propagules for the invasion of commercial citrus (Milosavljević et al. 2017, 2022). However, additional pesticide-free control methods have received little attention.
One tool that remains unexplored in the control of ACP is the sterile insect technique (SIT); a method in which large numbers of an insect pest species are reared, sterilized by exposure to a radiation source, and then released into the field where they compete with wild individuals of the same species for mating (Knipling 1955, Dyck et al. 2021). The sterilized insects behave and function more or less normally, but the irradiation process damages their chromosomes, leading to the formation of dominant lethal mutations in the DNA of the germ cells (LaChance 1967, Curtis and Robinson 1971) and mitotically active somatic cells, which in turn results in the death of irradiated cells or developing embryos, following fertilization (Helinski et al. 2006, Helinski and Knols 2009, Robinson 2021). Given their “evolutionary directive” to mate with multiple females, males have typically been considered the functional element of SIT, and following their release, an excess of sterile males in the environment increases the likelihood that a wild female will mate with one of those sterile males; a cross which subsequently produces very few (often zero) offspring, and this ultimately leads to a reduction of the wild pest population (Knipling 1955). Indeed, early studies, many of which focused on dipterans, suggested that females had little impact, and were effectively “surplus to requirement” in the sterilizing program. However, recent work has shown that releasing both sexes following sterilization is more effective than male-only releases for controlling European grapevine moth (Saour et al. 2023).
Over the years, SIT has played a significant role in the management of many pests in containment and eradication programs (Klassen et al. 2021). For example, it has been successfully employed to eradicate the screwworm, Cochliomyia hominivorax (Diptera: Calliphoridae), from the Americas (Snow 1988) and North Africa (Lindquist et al. 1992), and the tsetse fly, Glossina austensi (Diptera: Glossinidae), from parts of East Africa (Vreysen et al. 2000, Klassen and Curtis 2005). SIT has also been used to prevent the establishment of the Mediterranean fruit fly, Ceratitis capitata (Diptera: Tephritidae), in Central and Latin America (Dyck et al. 2021), the Spanish Mediterranean coast (Plá et al. 2021), and California (Enkerlin 2021, USDA-APHIS 2022). Other examples of effective SIT programs include preventing the establishment of and later eradicating, pink bollworm, Pectinophora gossypiella (Lepidoptera: Gelechiidae), in south-western USA and northern Mexico (Simmons et al. 2021), and the area-wide suppression of codling moth, Cydia pomonella (Lepidoptera: Tortricidae), in Canada, and false codling moth, Thaumatotibia leucotreta (Lepidoptera: Tortricidae), in South Africa (Simmons et al. 2021).
Increasing public concern about pesticide residues in food and the environment, the development of resistance to pesticides by ACP, and the vulnerability of important natural enemies and pollinators to the same pesticides have created demand for sustainable strategies such as SIT as part of area-wide integrated pest management (AW-IPM; Bourtzis and Vreysen 2021, Li et al. 2021). In comparison to other biological pest control methods, the autocidal nature of SIT removes any concerns over nontarget effects, since it does not introduce new species or genetic material into the environment (Hendrichs et al. 2002, Helinski and Knols 2009, Bourtzis and Vreysen 2021, Kapranas et al. 2022). The success of any SIT program depends on in-depth knowledge of a pest’s biology and ecology, the competitiveness of the irradiated individuals, and the continuous area-wide release of sterile insects over time (Vreysen et al. 2007). It is also dependent upon the development of efficient mass-rearing, handling, and release methods.
The use of SIT for ACP may further benefit from the knowledge that like some other Hemiptera and most (if not all) Lepidoptera, it possesses holokinetic or “diffuse” chromosomes (Drinnenberg et al. 2014, Marec et al. 2021). Achieving total sterility following irradiation in the males of holokinetic species has often proved to be difficult (they are radioresistant), but their cytogenetics are such that sterility can be inherited and amplified in any progeny that do result from partially sterilized males (LaChance and Degrugillier 1969, LaChance et al. 1970, Marec et al. 2021). This inherited sterility (also referred to as F1 sterility) is a common feature of many moths, and it has been argued, could be a very useful component of some of the operational SIT programs targeting moths (Marec et al. 2021, Simmons et al. 2021, Saour et al 2023). Since the potential for inherited sterility may also be high in ACP, this obviously demands investigation and the determination of any impacts.
The primary goal of this study was to investigate the radiation biology of ACP, with the aim of determining the radiation dose needed to induce full or partial sterility in the parental generation and F1 progeny without impairing their performance (e.g., mating activity, dispersal, and longevity). The specific objectives of this study were to: (i) determine the radiation doses that are sufficient to induce sterility in male and female ACP, (ii) investigate the impact of irradiation on the longevity and mating competitiveness of ACP, and (iii) determine whether “incomplete sterility” can be inherited by the F1 generation (i.e., F1 sterility). To our knowledge, this is the first investigation of the radiation biology of ACP and is the first step to evaluating the feasibility of developing SIT for its control.
Materials and Methods
Plant Material Preparation
Bergera koenigii (syn. Murraya koenigii) (Rutaceae: Sapindales) is a widely used rearing host for ACP because it is able to withstand continuous psyllid feeding, and it is not a host for CLas (Skelley and Hoy 2004, Parra et al. 2016, Beloti et al. 2018). Seeds from mature fruits of B. koenigii were collected in the field (USDA National Clonal Germplasm Repository for Citrus, Riverside, CA, USA) and processed according to the following protocol. The seeds were extracted from the pulp with warm water, surface-sterilized with 5% (v/v) sodium hypochlorite for 10 min, rinsed thoroughly with warm water, and finally rinsed with ambient temperature water before air-drying for 24–48 h.
The processed seeds were used to produce plants of 2 specific sizes (growth stages). For radiation bioassays, seeds were sown in individual 150-mm tall borosilicate glass test-tubes containing a moistened substrate of a 1:1 mix of perlite (Airlite Processing of Florida, Vero Beach, FL, USA) and vermiculite (Verkite, Tampa, FL, USA), and fertilized with Osmocote 14–14–14 (N–P–K) (The Scotts Company, OH, USA). Tubes were plugged with foam or cotton to prevent desiccation. The seeds were allowed to grow until they were approximately 5–6 wk-old (Supplementary Fig. 1A) in a growth room at 25 °C and 55% relative humidity (RH), with 16:8 (L:D) photoperiod. For maintaining ACP colonies and housing adult ACP immediately prior to irradiation (see below), 6-month old B. koenigii plants with flush were grown from seed in a greenhouse in black square form plastic pots (3.25 L × 3.25 W × 3.5 H in; Greenhouse Megastore, West Sacramento, CA, USA) containing UC Soil mix III (UC Agricultural Operations 2023; Supplementary Fig. 1B). The plants were moved to the growth room as needed.
Insect Preparation
ACP was obtained from a colony established in 2011 at the University of California Riverside, Insectary & Quarantine Facility. This colony was originally collected from citrus trees in Southern California and has been maintained in insect-rearing cages (W 47.5 × D 47.5 × H 47.5 cm) (Bugdorm, MegaView Science Co., Ltd., Taiwan) containing B. koenigii plants with young flush. Prior to each radiation bioassay, ACP adults were taken from the colony and introduced onto flushing B. koenigii plants suitable for ACP oviposition and feeding. The plants were placed in a controlled growth room at 25 °C and 55% RH with 16:8 (L:D) (Skelley and Hoy 2004, Parra et al. 2016), and after 7 days of oviposition, the parental ACP were removed. The plants continued to be maintained in the growth room, allowing ACP eggs to hatch and the nymphs to feed and develop. Approximately 10–12 days after the parent ACP were removed, fifth instar nymphs were collected from plants and transferred to individual test-tubes containing a single 5–6 wk-old B. koenigii seedling. The test-tubes were checked daily, and newly emerged virgin adult ACP was collected, sexed, and housed in single-sex cages containing 6-month old B. koenigii plants for 3 days. The ACP were then recollected and either prepared for irradiation (described below) or immediately incorporated into our bioassays as nonirradiated partners (wild types) or controls.
Insect Irradiation
One hundred adult ACP were transferred into each of several, single-sex, heavy-weight 40-dram, plastic “prescription” vials fitted with child-proof caps (United States Plastic Corporation, Lima, OH, USA). The inside of the cap was lined with moist filter paper to prevent desiccation of the insects, and the caps were further sealed with a strip of parafilm. Each vial was labeled with the radiation dose it was to receive. For shipment, the vials were placed into an insulated foam shipping box that uses a system of gel refrigerant designed to hold stable temperatures at 3–4 °C for several days (GTS-89 Cold Chain Technologies, Franklin, MA, USA). Temperatures during shipping were recorded with an iButton temperature logger (iButtonLink LLC, Whitewater, WI, USA) to confirm temperature stability. The insects were shipped under California Department of Food and Agriculture Plant Pest Permit #3283 by overnight courier service to the USDA-APHIS-PPQ in Salinas, CA, USA, for irradiation the following day. Irradiation treatments were applied to the ACP vials using an RS 2400V X-ray irradiator (Radsource Technologies, Buford, GA, USA; Supplementary Fig. 1C), operating at 160 kV and 25 mA to deliver a dose rate of 14.5 Gy/min. The irradiator has a single chamber that hoses a carousel, which in turn, holds 6 carbon fiber canisters (12.8 cm internal height × 10 cm internal diameter) fitted with steel lids that lock in place. In each canister, a single 40-dram vial containing the ACP was held in the center by top and bottom disks of hard polyurethane foam, shaped to serve as jigs to securely hold the vials in the same position across irradiation runs. To keep the insects immobile during irradiation, temperature in the irradiation chamber was maintained at 6–8 °C by operation of an external chilling system. The delivered dose rate at the center of the 40-dram vials was confirmed using a calibrated NIST traceable Accu-Dose Ion Chamber (RadCal©).
Since male hemipterans are often more radiation-resistant than females (e.g., Calvitti et al. 1997, 1998, Welsh et al. 2017), several pilot bioassays were conducted to determine an appropriate range of radiation doses to test for each sex (data not shown). As a result, the range of doses selected for males was fivefold higher than those for females. Radiation doses selected for females were 40, 60, 80, and 100 Gy. Those for males were 80, 160, 240, 320, 400, and 480 Gy. Nonirradiated ACP served as controls (0 Gy) but were included in the shipments, thereby removing the shipping process as a variable in our experimental design. After irradiation, the vials of psyllids were immediately returned to the temperature-stable shipping container and shipped back to UCR the same day. On their return to UCR, 32–34 h after their initial collection from the colony, the irradiated ACP and nonirradiated control individuals were allowed to reacclimate to ambient room temperature for 1–2 h before incorporation into the bioassays. All bioassays were performed at the UCR Insectary & Quarantine Facility.
The Effect of Irradiation Dose on Parental Fecundity and Sterility
Individual virgin females from each of the irradiation dose treatments (40, 60, 80, and 100 Gy), were paired with a nonirradiated virgin male (I♀ × N♂) (n = 10 pairs per treatment). Similarly, virgin males from each irradiation dose treatment (80, 160, 240, 320, 400, and 480 Gy), were paired with a nonirradiated virgin female (N♀ × I♂) (n = 10 pairs per treatment). Control crosses were included for both bioassays and involved the pairing of a female or male, which was shipped to the irradiation facility but not irradiated, with a nonirradiated (and nontransported) member of the opposite sex. Each experimental pair was confined in an individual 150-mm tall borosilicate glass test-tube, containing a single B. koenigii seedling (Supplementary Fig. 1A), for 3 days. Each pair was subsequently transferred to a new test-tube, with a new seedling, every 3 days, and allowed to mate and lay eggs for a total of 15 days. The experiment was carried out inside a controlled growth room at 25 °C, 55% RH, and a 16:8 (L:D) photoperiod. The number of eggs laid per treatment was counted under a stereomicroscope shortly after every transfer of the parents, and the plants were subsequently maintained under the experimental conditions awaiting egg hatch. Each vial was checked daily for hatched nymphs for 15 days, allowing the number of eggs that hatched to be recorded. Nymphs were collected, and reared to adulthood, and then housed in single-sex plexiglas cages (W 15.24 × L 15.24 × H 30.48 cm) containing a 6-month old flushing B. koenigii plant (Supplementary Fig. 1B). These offspring were subsequently used in F1 sterility bioassays (see below).
F1 Sterility Bioassays
Regardless of the amount of irradiation received, no F1 offspring from the crosses involving irradiated females survived to adulthood (see Results section). In contrast, in all irradiated male treatments, except for the highest dose (480 Gy), a number of both male and female offspring survived to adulthood. From among the surviving offspring of fathers exposed to 0, 80, 160, 240, 320, and 400 Gy, respectively, 10 F1 males and 10 F1 females were randomly selected and paired with a nonirradiated, virgin member of the opposite sex, resulting in 12 different crosses and 120 total F1 pairings. Each pair was again housed in a vial containing a single B. koenigii seedling and checked daily following the same protocol outlined above. Any emerging F2 nymphs were removed upon hatching and placed in single-sex plexiglass cages containing a flushing 6-month old B. koenigii plant, to evaluate survival.
Adult Mortality Postirradiation
On receipt following the irradiation treatment, actively moving psyllids from each irradiation dose and the control were allowed to acclimatize to the lab for at least 1 h. Three groups of 16 females and another 3 groups of 5 males were confined in single-sex plexiglass cages (W 15.24 × L 15.24 × H 30.48 cm) with a 6-month old flushing B. koenigii plant. The cages were placed in a growth room at 25 °C, 55% RH, and a 16:8 (L:D) photoperiod and surveyed at 7, 14, and 21 days, and the number of dead adults for each radiation treatment was recorded.
Data Analyses
We explored the effects of irradiation on D. citri biology via a series of analyses on fecundity, egg hatch, and survival of irradiated adults. All analyses were performed using R version 4.3.0 (R Core Team 2023).
Separate generalized linear models were used to analyze the effect of radiation dose on fecundity stemming from matings involving irradiated female or male D. citri (Crawley 2009). Both models assumed negative binomial error to appropriately capture the count nature of the data (i.e., number of eggs laid per female). For the analysis of irradiated females, dose was treated as a continuous variate but was included as a fixed effect for the analysis of irradiated males due to an apparent nonlinear effect. For males, a significant effect of dose was followed up with pairwise contrasts of the number of eggs laid between the 0 Gy treatment and all other individual doses (Crawley 2009). A similar generalized linear model with negative binomial error was used to analyze F1 fecundity, which included dose and F1 sex as covariates, and employed likelihood ratio tests to compare the full model to a series of reduced models to identify the minimum adequate model needed to appropriately describe the data (Crawley 2009).
Three pairs of models were used to analyze, separately, fertility (egg hatch) from matings involving irradiated females, irradiated males, and F1 offspring. First, a generalized linear model with binomial error (i.e., logistic regression) was used to compare the proportion of females for which at least some eggs laid ultimately hatched (Crawley 2009). In each model, radiation dose was treated as a covariate. For F1 fertility, the model also included an effect of F1 sex, and model simplification was used to identify the most parsimonious and adequate model. Next, a generalized linear mixed-effects model (GLMM) was used to analyze the proportion of all eggs that hatched (Pinhero and Bates 2000). Each model included dose as a covariate, binomial error, and a random effect of replicate identity to capture autocorrelation in hatch rate among eggs laid by individual females. For F1 fertility, the model included an effect of F1 sex and employed model simplification via likelihood ratio tests to identify the minimum adequate model.
Finally, the survival of irradiated female and male D. citri was analyzed using separate generalized linear mixed-effects models. Each model included radiation dose as a covariate, census week (i.e., time postirradiation) as a fixed effect, binomial error to capture the survival of individuals within groups of 5 males or 16 females, and a random effect of individual identity nested within the group to capture temporal autocorrelation stemming from repeated measures (Pinhero and Bates 2000, Crawley 2009). Again, model simplification via likelihood ratio tests was used to identify the most parsimonious and adequate model.
Results
Sterilization of ACP Females and Males
Sterilizing effect of irradiation on females. Virgin female ACP subjected to different radiation doses (0, 40, 60, 80, and 100 Gy) were paired with a nonirradiated virgin male (I♀ × N♂), and allowed to mate and produce offspring for 15 days. Results of the GLM analysis showed a significant and negative effect of dose on fecundity (χ2 = 17.17, df = 1, P < 0.0001; coefficient ± SE = −0.018 ± 0.005). Control crosses laid as many as 683 eggs with a mean (± SE) of 443.7 (± 71.57) eggs vs. 92.3 (± 12.95) eggs for all irradiated females, approximately equating to an 80% reduction (Fig. 1A).
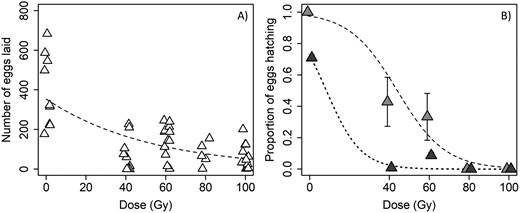
The effect of different irradiation doses on A) fecundity and B) egg hatch of mating pairs in which female D. citri were irradiated and paired with nonirradiated males (I♀ × N♂). In A), the dashed line denotes the fit of the negative binomial GLM. In B), lighter gray symbols denote the proportion (±SE) of females for which at least some eggs hatched, and the dashed line denotes the fit of the logistic regression model; darker symbols and the dotted line show the effect of dose on the proportion (±SE) of all eggs that hatched. In both panels, points are jittered or offset slightly for clarity.
A negative effect of the dose on egg hatch was also apparent. The logistic regression showed a significant and negative effect of dose on the proportion of females that had at least a single egg hatch (χ2 = 32.898, df = 1, P < 0.0001; coefficient ± SE = −0.083 ± 0.026). One hundred percent of control females produced at least 1 viable egg, while no females irradiated at 80 or 100 Gy laid any eggs that subsequently hatched (Fig. 1B). Across all eggs that were laid, the hatch rate was significantly and negatively related to radiation dose (χ2 = 248.5, df = 1, P < 0.0001; coefficient ± SE = −0.114 ± 0.007). Nearly 80% of the eggs laid by control females hatched but declined to approximately 1% and 10% at 40 and 60 Gy, respectively (Fig. 1B).
Sterilizing effect of irradiation on males. Nonirradiated virgin females produced as many as 523 eggs when mated with a nonirradiated male, with a mean ± SE of 266.30 ± 31.78 (Fig. 2A). Across all male radiation doses, there was a marginally significant effect on fecundity (χ2 = 12.434, df = 6, P = 0.0529), but without any clear pattern. Fecundity was lowest at 80 and 480 Gy, and highest at 320 Gy, but pairwise contrasts subsequently found no significant difference between 0 Gy and all other doses (Fig. 2A).
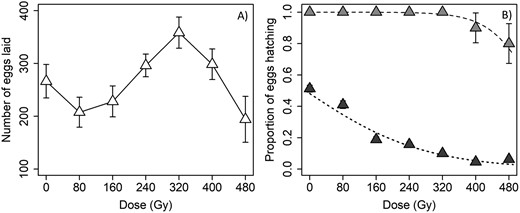
The effect of radiation dose on mean (±SE) A) fecundity (eggs laid per female) and B) egg hatch (fertility) when male D. citri were irradiated and crossed with nonirradiated virgin females (I♂ × N♀). In B) lighter gray symbols denote the mean proportion (±SE) of females for which at least some eggs hatched, and the dashed line denotes the fit of the logistic regression model; darker symbols and the dotted line show the effect of radiation dose on the proportion (±SE) of all eggs that hatched.
With respect to egg hatch, the proportion of females that had at least some eggs hatch was significantly and negatively affected by dose (χ2 = 7.626, df = 1, P = 0.0058; coefficient ± SE = −0.019 ± 0.011). However, complete sterility (zero egg hatch) was not achieved. One hundred percent of females had some eggs hatch at doses up to 320 Gy, compared to 90% and 80% at 400 and 480 Gy, respectively (Fig. 2B). The overall egg hatch rate was also significantly and negatively affected by male radiation dose (χ2 = 155.1, df = 1, P < 0.0001; coefficient ± SE = −0.007 ± 0.001). Overall, the hatch rate declined from more than 50% for females mated to nonirradiated males to approximately 5% at the highest doses (Fig. 2B).
Fecundity and Sterility of the F1 Offspring of Irradiated Fathers
The final model for effects on the fecundity of F1 daughters (paired with nonirradiated males) and F1 sons (paired with nonirradiated females) retained only a significant effect of F1 sex (χ2 = 7.184, df = 1, P = 0.0074); the effect of dose and the interaction between dose and sex were dropped during model simplification. Mated F1 sons produced more than twice as many eggs (mean ± SE: 86.65 ± 11.95) as mated F1 daughters (33.72 ± 7.82).
Although eggs were laid in all F1 treatments, there was substantial variability in the viability of those eggs across different treatment combinations. With respect to the proportion of females that produced any viable eggs, the final model included only a significant and negative effect of dose (χ2 = 19.108, df = 1, P < 0.0001; coefficient ± SE = −0.009 ± 0.002). The proportion of females that had some eggs hatch declined from 100% for the offspring of nonirradiated adults to less than 10% at 400 Gy (Fig. 3). The final model for the overall proportion of eggs that hatched also included only a significant and negative effect of radiation dose (χ2 = 35.891, df = 1, P < 0.0001; coefficient ± SE = −0.017 ± 0.003). The overall proportion of eggs hatching declined from more than 50% for the offspring of nonirradiated adults to complete sterility (0% hatch rate) at 400 Gy (Fig. 3).
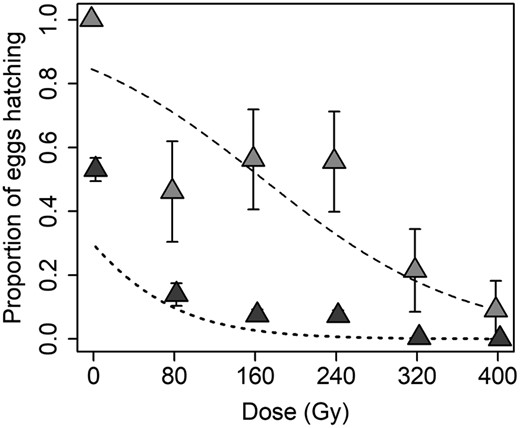
The effect of paternal radiation dose on the viability of eggs laid following the pairing of F1 sons and daughters of irradiated fathers with a nonirradiated mate. Lighter gray symbols denote the proportion (±SE) of females for which at least some eggs hatched, and the dashed line denotes the fit of the logistic regression model; darker symbols and the dotted line show the effect of radiation dose on the proportion (±SE) of all eggs that hatched.
Survival After Irradiation
For the proportion of irradiated females surviving, the final model included only a significant effect of census week (χ2 = 925.21, df = 2, P < 0.0001); dose and the dose-by-census week interaction were dropped during model simplification. Female mean survival at week 1 ranged between approximately 75% and 80%, falling to 40%–55% at week 2 and 15%–40% at week 3 (Fig. 4A).
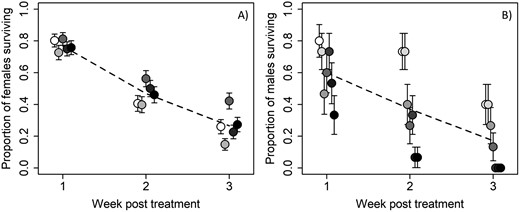
The effect of irradiation on survival of A) D. citri adult females, showing no strong influence of radiation dose (points represent mean (±SE) survival of groups of 16 females for each radiation dose—census week combinations), and B) D. citri adult males which showed a decline of survival by dose over time posttreatment (points represent mean (±SE) survival of groups of 5 males for each radiation dose—census week combinations). White point indicates control (0 Gy). Darker colors indicate increasing dose (females, 20 Gy increments from 40 to 100 Gy; males 80 Gy increments from 80 to 480 Gy).
Male D. citri were subjected to higher doses of radiation in order to impart sterility to the F1 individuals (see above). The final model for male survival included a significant effect of census week (χ2 = 127.37, df = 2, P < 0.0001) and a significant and negative effect of radiation dose (χ2 = 22.698, df = 1, P < 0.0001; coefficient ± SE = −0.101 ± 0.02). The mean proportion of males surviving ranged from approximately 80% for nonirradiated males to 30% at the highest doses after 1 wk, between approximately 75% for control males to 6% at the highest doses after 2 wks, and between 40% at the lowest doses and no survival at the 3 highest doses after 3 wk (Fig. 4B).
Discussion
This study investigated the radiation biology of ACP as a first step in determining the feasibility of implementing SIT for this pest. SIT relies on inducing sterility in the females of a wild population after mating with released sterile males that carry dominant lethal mutations in their sperm as a result of exposure to ionizing radiation (Robinson 2021). We subjected groups of 3- to 7-day-old ACP to X-ray irradiation with doses up to 100 Gy for females and up to 480 Gy for males. Females were found to be very sensitive to radiation, and 100% sterility was achieved at doses ≥ 80 Gy. Males were found to be more radiation-resistant and, even at a dose of 480 Gy, achieved only 95% sterility. However, the F1 offspring of male parents irradiated at 320 Gy and higher (both males and females) were found to be 100% sterile. Thus, this inherited sterility indicates that 320 Gy of X-ray irradiation may be an effective dose for the further development of an SIT program for ACP. That dose was found to have some impact on the lifespan of irradiated males, but over 30% were still active 14 days postirradiation (compared with around 70% in the control treatment).
Biological Effects of Radiation on Parental and F1 Generations
Radiosensitivity in female ACP and radioresistance in male ACP were clearly demonstrated in this study. The fecundity of irradiated females showed a decline even at the lowest radiation dose of 40 Gy, resulting in an ~80% reduction in the number of eggs laid by females in all radiation treatments compared to the control. In contrast, irradiating males did not affect their ability to induce oviposition in nonirradiated female partners. A similar pattern was evident in the fertility data, measured as egg hatch. Compared to the control, where 100% of females produced some viable eggs, the proportion of irradiated females that laid at least 1 viable egg decreased to around 40% at 40 Gy, and declined further at higher doses. Furthermore, among those eggs that were laid by irradiated females, only a very low proportion hatched at 40 and 60 Gy, and none hatched at 80 Gy and above. In contrast, among the normal females that mated with an irradiated male, the majority (>80%) still laid at least 1 viable egg, even at the highest dose (480 Gy). However, if one considers just the overall viability of the entire clutch of eggs laid by these females, the hatch rate was again significantly impacted at all doses, falling to around 10% and 5% of the eggs hatched at 320 and 400 Gy, respectively.
The finding that females are more sensitive to radiation than males corroborates with findings from other Hemiptera. Females have been shown to require lower doses than males in the red cotton stainer, Dysdercus koenigii (Hemiptera: Pyrrhocoridae) (Srivastava and Deshpande 1983, Srivastava et al. 1985), the greenhouse whitefly, Trialeurodes vaporariorum (Hemiptera: Aleyrodidae) (Calvitti et al. 1997), and the brown marmorated stink bug, Halomorpha halys (Hemiptera: Pentatomidae) (Mazzoni et al. 2017). Indeed, this may be a common pattern across Hemiptera (Marec et al. 2021). Similar patterns of male radioresistance have also been observed in Lepidoptera. Male codling moth, C. pomonella (Bloem et al. 1999), and false codling moth, T. leucotreta (Bloem et al. 2003), respectively, require a twofold and fourfold higher dose of radiation than females to achieve sterility. It has been suggested that these differences between the sexes could be attributed to the presence of nurse cells among the mitotically active oocytes during endomitosis (LaChance and Leverich 1962, LaChance and Bruns 1963). Nurse cells effectively function to supply the developing oocyte with organelles and other cytoplasmic components that are required for embryonic development. If radiation has a detrimental effect on such cytoplasmic components, this would be expected to reveal itself in mature oocytes but not in sperm, which are essentially cytoplasm-free (Marec et al. 2021). It has also been postulated, at least in Lepidoptera, that this difference in radiosensitivity between males and females could be a simple reflection of differences in the stage of development of the reproductive cells of each sex at the time of irradiation. If, as in our study, irradiation is performed on newly emerged adults, eupyrene spermiogenesis in the males is expected to have been completed, and the nuclei in eupyrene sperm are in interphase. In contrast, female meiosis is arrested at metaphase I in the nuclei of mature oocytes, and the process does not resume until the eggs have been fertilized (Traut 1977). As a consequence, irradiation of newly emerged females may disrupt the normal course of meiosis, including chromosome segregation, following fertilization of the oocytes.
Although we found that complete sterility could not be directly induced by irradiating ACP males, all male and female F1 offspring that resulted from matings between fathers exposed to 320 Gy (and higher doses) and nonirradiated females were subsequently shown to be completely sterile. This is strong evidence that sterility was inherited by the F1 generation. Inherited sterility was documented in Lepidoptera as early as the 1930s (Astaurov and Frolova 1935, Ostriakova-Varshaver 1937) but received little further attention until the 1960s when it was discovered in a North American study of the codling moth (Proverbs 1962). It was later documented in Oncopeltus fasciatus (Hemiptera: Lygaeidae) (LaChance and Degrugillier 1969, LaChance et al. 1970). The mechanism of inherited sterility is believed to involve the initial fragmentation of chromosomes in the irradiated parents and the subsequent inheritance of these chromosomal fragments by the F1 offspring (Carpenter et al. 2005). Hemipterans and Lepidopterans have characteristic holokinetic chromosomes in which a localized centromere is absent (Drinnenberg et al. 2014, Marec et al. 2021). This fosters an environment in which radiation-induced deleterious effects (i.e., fragmented chromosomes) are stable and can be transmitted to offspring. Indeed, in O. fasciatus, such fragments can be transmitted through as many as 3 generations of outcrosses to normal females (LaChance and Degrugillier 1969). When radiation-induced deleterious effects are passed to the F1 generation, this is expected to result in a reduction in egg hatch and any surviving offspring being both highly sterile and predominately male (Vreysen et al. 2016, Marec et al. 2021). These expectations were largely met by our data for ACP.
The range of radiation doses (40–100 Gy) that we subjected female ACP to was not detrimental to their survival compared to the control group, although at all doses, fecundity was greatly reduced, and fertility was ~0%. In contrast, male survival was negatively correlated with radiation dose, although, of course, the males experienced much higher doses than the females (in order to achieve sterility). When exposed to 320 Gy or higher, no males survived after 3 wk, compared with around 40% in the control group. However, at that dose, the survival rate at the 2-wk mark was around half that of the control group (Fig. 4). This contrasts with observations of Nezara viridula (Hemiptera: Pentatomidae), in which Horrocks et al. (2020) compared the longevity of control males with those subjected to a dose of radiation sufficient to impart 100% sterility and found no discernable difference. However, while their comparison was also based on a 2-wk period, the dose of radiation applied to render the male N. viridula sterile was only 28 Gy. Similar findings have been reported in Drosophila suzukii (Diptera: Drosophilidae), where the longevity of both males and females was not affected when they were subjected to 120 Gy, a dose that induced 96% sterility (Lanouette et al. 2017). With reference to these 2 studies, given the relative amount of radiation required to sterilize ACP, it is perhaps not too surprising that it impacted longevity. Adult longevity in our study compares with reports postulating that ACP can live for longer than a month in the field (Mead 1977, Halbert and Manjunath 2004) and a more empirical laboratory study that shows longevity is affected by temperature, varying from a mean of 50.6 days at 20 °C to 33.5 days at 30 °C (Liu and Tsai 2000). Although adult male longevity was not measured in that study, it has since been shown that males likely have similar (perhaps even slightly shorter) lifespans (Meng et al. 2022). Thus, our findings highlight the need to balance the sterilizing effects of radiation against its mortality-inducing effects.
It is possible that the females in our experiments were not able to realize their full fecundity since our experimental design meant that females were isolated with just a single male. This precluded the opportunity for females to mate with more than 1 male, which may not be the case in the wild, where mating with multiple males may be beneficial to both fecundity and fertility (Ridley 1988). That said, there is evidence that ACP females actually lay more eggs and live longer when paired with a single male for 2 wk, compared to females held with more than 1 male for 2 wk (Wenninger and Hall 2008), so perhaps female ACP do actually seek to avoid multiple mating. Overflooding ratio experiments will help to understand the real effect of females mating with multiple males (sterile and nonsterile).
Implications for Developing an SIT Program
Worldwide, it has been observed that the progression of HLB can be reduced by controlling the vector (Bové 2006). The findings presented herein suggest the potential for using SIT to control ACP. In identifying effective radiation doses that impart significant levels of sterility, we considered males and females separately. However, it would be impractical to separate males and females in a mass-rearing program, so mixed-sex irradiation will be required. This may even be advantageous if irradiated females distract wild males, deplete wild sperm from wild males, and/or reduce the amount of mating between wild males and females. For instance, in the cactus moth, Cactoblastis cactorum (Lepidoptera: Pyralidae), releasing sterile males and females has proven more effective in the control of pest populations than releasing males alone (Hight et al. 2005). For ACP, we have identified that a dose of 320 Gy will render females 100% sterile and also achieve a high level of male sterility albeit with a reduction in male longevity (i.e., a potential fitness cost). Importantly, at this dose, any surviving F1 progeny that were fathered by the irradiated males, were determined to be completely sterile. Further evaluation may identify lower doses (e.g., between 240 and 320 Gy) that offer a better balance of parental sterility, parental survival, and F1 sterility.
Simply identifying that the irradiation process impacts survival does not preclude SIT as a potential control method for ACP. In our study, males exposed to 320 Gy still remained active for 2 wk, affording them a good window of opportunity to compete for mates. Furthermore, any radiation-induced effects on longevity may be countered in an actual area-wide SIT program by optimizing the frequency of releases. Operational programs for the moths, C. cactorum and T. leucotreta, both implement twice-weekly releases during target times (Hight et al. 2005, Hofmeyr et al. 2015). Likewise, treatment plans recently implemented in California to eradicate the Mexican fruit fly, Anastrepha ludens (Diptera: Tephritidae), proposes releasing sterilized flies every 3–4 days for up to 6 months (2 life cycles of the pest) (CDFA 2022).
In the present study, we have only considered the effects of radiation on individual fitness through a series of matings in which a single irradiated ACP was confined with a single normal ACP. The population-level fitness of any SIT release would almost certainly be impacted by the size of the sterilized population released, specifically relative to the resident wild population. The next stage of this work will focus on the evaluation of the performance of different-sized groups of ACP, subjected to either 240 Gy or 320 Gy, in order to estimate the ratio of sterile males to wild males (overflooding ratio) that might be required to effectively suppress a wild population of ACP. Timing is also likely to be critical to the success of any SIT release. The presence of ACP is correlated with the availability of citrus flush (Hall and Albrigo 2007), so the release of irradiated psyllids at early and mid-flush season would likely impart the greatest reduction in wild populations. While F1 sterility may contribute to a continuation of the suppressive effect on wild populations, the impact of serial releases of freshly irradiated ACP, at regular intervals throughout the flush season should also be investigated.
In California, the use of SIT for area-wide control of ACP would be compatible with and complementary to existing tactics that include a successful classical biological control program that has involved the rearing, release, and establishment of T. radiata, a parasitoid of ACP imported from Pakistan (Hoddle and Hoddle 2013, Milosavljević et al. 2022), and efforts to conserve and/or augment indigenous natural enemies (Gomez-Marco et al. 2019, 2022). However, releasing a vector organism such as ACP may be a concern for some growers because of the prospect of increasing (if only temporarily) the actual vector population. In the case of ACP, we believe that such concerns are not justified. It has been repeatedly reported that CLas is only efficiently transmitted by ACP that acquired the pathogen during the nymphal stage of its development (Xu et al. 1988, Hung et al. 2004, Inoue et al. 2009, Pelz-Stelinski et al. 2010, Ammar et al. 2016, 2020, Canale et al. 2017, Wu et al. 2018). In contrast, ACP that acquire the bacteria as adults are very poor vectors of the pathogen. It is thought that the multiplication of the pathogen within the insect vector is essential for effective transmission and that CLas simply multiplies too slowly in adult ACP to reach a sufficient titer to be subsequently transmitted (Inoue et al. 2009, Pelz-Stelinski et al. 2010). After feeding on an infected host plant as an adult, a latent period of at least 18–21 days is necessary for significant bacterial multiplication and/or translocation of CLas into the salivary glands of the vector (Ammar et al. 2011, 2020, Wu et al. 2018). Thus, as was the case in our study, program ACP could be reared on CLas-free curry leaf plants that are not a host reservoir of the bacterial pathogen (Skelley and Hoy 2004, Parra et al. 2016, Beloti et al. 2018). Since the ultimate goal of an SIT program would be to prevent the spread of CLas, program ACP would then be released into an area where, at worst HLB is currently so rare that the area is at that time considered HLB-free. So, the chances of a released psyllid encountering an infected host plant are very small. Furthermore, ACP is not strong fliers (Sakamaki 2005, Arakawa and Miyamoto 2007, Martini et al. 2014), more or less confining released ACP at target sites. Finally, even in the highly unlikely event that a released psyllid did acquire CLas, it is unlikely that the ACP would then survive long enough for the pathogen to multiply to an extent that the individual would become a vector. In our present study, adult ACP irradiated at 320 Gy survived for less than 3 wk after irradiation.
For routine and operational area-wide releases of irradiated ACP, availability of mass-rearing systems will need to be put in place. Protocols already exist for the rearing of the biological control agent T. radiata (see above) that allow the production of 5,000–7,000 ACP adults per square meter (sqm) of greenhouse space, in 20 days, on B. koenigii (CDFA 2017). The use of B. koenigii as a rearing host for ACP is very important as it is not a reservoir of the CLas pathogen (Skelley and Hoy 2004, Parra et al. 2016, Beloti et al. 2018), ensuring a constant supply of CLas-free ACP for the SIT. Shipping conditions (i.e., temperature, packing, and storage) will also require optimization to find the best strategy for bulk transport and sterilization of ACP, minimizing mortality in ACP during the irradiation process.
Supplementary Material
Supplementary material is available at Journal of Economic Entomology online.
Acknowledgments
We wish to thank Dr. Richard Stouthamer for his helpful suggestions and feedback on the design and presentation of this research. We also thank Sen Miao and David Borjon for providing assistance in the growing of B. koenigii (curry leaf plants) and rearing of the psyllids for the experiments. Finally, we thank the staff of United States Department of Agriculture—Animal and Plant Health Inspection Service during the repacking and shipment of irradiated ACP for bioassays at UC Riverside.
Funding
This work was supported by USDA-APHIS; Agreements 16-8130-0729-CA, AP18PPQS&T00C231, and AP21QS&T00C148.
Author Contributions
Jedeliza Ferrater (Conceptualization [equal], Data curation [lead], Formal analysis [supporting], Investigation [lead], Methodology [equal], Project administration [equal], Validation [equal], Visualization [lead], Writing—original draft [lead], Writing—review & editing [lead]), Francesc Gomez Marco (Data curation [supporting], Investigation [supporting], Methodology [equal], Writing—review & editing [supporting]), Andrew Yoshimoto (Investigation [supporting]), Thomas Greene (Investigation [supporting]), Greg Simmons (Conceptualization [equal], Funding acquisition [equal], Investigation [supporting], Methodology [equal], Resources [equal], Writing—review & editing [supporting]), Matthew Daugherty (Data curation [supporting], Formal analysis [lead], Methodology [supporting], Writing—review & editing [supporting]), and Paul Rugman-Jones (Conceptualization [equal], Funding acquisition [equal], Investigation [supporting], Methodology [supporting], Project administration [equal], Resources [equal], Supervision [lead], Validation [equal], Writing—original draft [supporting], Writing—review & editing [lead])
References
Author notes
Francesc Gómez-Marco Present address: Institute of Agrifood Research and Technology, Ctra. de Cabrils, Km 2, 08348 Cabrils, Barcelona, Spain
Andrew K. Yoshimoto Present address: Department of Entomology, Michigan State University, 288 Farm Lane, East Lansing, MI 48824, USA