-
PDF
- Split View
-
Views
-
Cite
Cite
Hanushree Arumugam, Ka Heng Wong, Zheng Yao Low, Sunil Lal, Wee Sim Choo, Plant extracts as a source of antiviral agents against influenza A virus, Journal of Applied Microbiology, Volume 136, Issue 3, March 2025, lxaf056, https://doi.org/10.1093/jambio/lxaf056
- Share Icon Share
Abstract
The influenza virus, especially influenza A (IAV), has remained a constant global health threat due to its high morbidity rate and ability to undergo antigenic shifts and drifts, causing pandemics and epidemics. Due to the rapid evolution of IAV, novel therapeutics are urgently required to combat these viruses effectively, as they develop resistance against current therapeutics. Natural products have been the subject of debate for alternative IAV therapy, where the abundance of bioactive compounds offers numerous potentials for novel anti-IAV drug discovery. Therefore, this review discusses the antiviral effects of natural plant extracts against IAV. Examples are Silybum marianum, Scutellaria baicalensis, Angelica dahurica, Peganum harmala, Sambucus nigra, Echinacea purpurea, Panax ginseng, and Camellia sinensis. Most studies found that Si. marianum inhibits viral ribonucleic acid (RNA) synthesis. In contrast, Sc. baicalensis, A. dahurica, Sa. nigra, C. sinensis, and E. purpurea were effective in preventing the entry or binding of IAV into host cells. On the other hand, Sc. baicalensis and Pa. ginseng exert their anti-IAV effect via immunomodulation. Peganum harmala, on the contrary, exhibits a direct virucidal effect against IAV. These studies have shown promising results from using natural products against IAV, which may aid in formulating combinatorial compounds as anti-IAV therapy.
Introduction
The influenza virus, commonly known as the ‘common cold’, is the etiological agent of multiple respiratory pandemics and seasonal endemics. Affecting approximately 5 million individuals annually, accompanied by 650 000 deaths, especially among elderly individuals (aged ≥65 years), there is a need to address the demand for effective antiviral and vaccines (World Health Organization [WHO] 2024). Influenza viruses (Orthomyxoviridae family) are RNA viruses that can be classified into four different types, namely A, B, C, and D, with the former two, influenza A virus (IAV) and influenza B virus (IBV), possessing a greater relevancy to human respiratory infection. Of these, IAV has contributed to major human pandemics over the past few decades (van Doorn and Yu 2020, Low et al. 2023) and, thus, is the focus of this review.
Accounting for four pandemics—the 1918 Spanish Flu pandemic, the 1957 Asian flu pandemic, the 1968 Hong Kong flu pandemic, and the 2009 Swine flu pandemic, the IAV is the most documented and monitored influenza virus. These were all brought by novel IAV subtypes that have capacities for rapid circulation among the global population (Saunders-Hastings and Krewski 2016, van Doorn and Yu 2020). IAV predominantly infects the upper respiratory tract, causing mild to moderate symptoms of sore throat, fever, cough, fatigue, and potentially lethal secondary bacterial infection of the lower respiratory tract and pneumonia in high-risk individuals (Kalil and Thomas 2019, Wong and Lal 2023).
The first known pandemic, the 1918 Spanish flu, has racked up a notoriously high number of deaths at an estimated 50–100 million count. This was stupefying over the rest of the recorded influenza pandemic, with an average of 0.5–2 million recorded deaths (Saunders-Hastings and Krewski 2016). Animal reservoirs, such as swine and aquatic birds, are believed to drive the pandemic potential of IAV as it facilitates the phenomenon referred to as the ‘antigenic shift’ and ‘antigenic drift’ (Saunders-Hastings and Krewski 2016). Co-infection of avian and swine IAV in the reservoir facilitates the reassortment of the segmented influenza ribonucleic acid (RNA) genomes, causing a ‘shift’ in the HA and NA antigenic proteins of IAV, which partook in virus infection and release, potentially causing the emergence of new variants (Marshall et al. 2013, Ren et al. 2016). In addition, the lack of proofreading and high error rates of the viral RNA-dependent RNA polymerase of the virus causes an accumulation of mutations in the RNA genome of IAV, leading to a “drift” that produces antigenic variants of IAV (Wong and Lal 2023). Hence, these gave rise to the highly destructive variant of IAVs with great pandemic potential. Apart from that, the constantly evolving virus facilitates the development of drug resistance, further restricting the already limited treatment options (Shao et al. 2017). Currently, the United States Food and Drug Administration-approved antiviral drugs for IAV include the matrix protein 2 (M2) ion channel inhibitors (rimantadine, amantadine), NA inhibitors (oseltamivir, zanamivir), and polymeric acid protein endonuclease inhibitors (baloxavir marboxil). Of these, amantadine is no longer favoured due to the high prevalence of resistance among IAV strains (O’Hanlon and Shaw 2019, Lampejo 2020).
With the mounting issue of IAV drug resistance and pandemic scares, there is an urgent demand for novel and alternative therapeutic approaches against the IAV. Natural products, predominantly plants, have long been a rich source of compounds with therapeutic applications (El Sayed 2000, Musarra-Pizzo et al. 2021). Sourcing natural products for new antiviral drugs is not a novel concept. It has yielded successful outcomes such as oseltamivir, synthesized using quinic and shikimic acids as starting products (Zhang et al. 2020). Multiple in vitro studies have also shown potent antiviral activities of phytochemicals such as terpenoids, flavonoids, coumarins, polyphenols, and peptides against various human viruses, further proving the viability of investigating natural plant sources for anti-IAV therapeutics (Kurokawa et al. 2010, Musarra-Pizzo et al. 2021). Hence, this review aims to present and discuss the antiviral effects of natural plant extracts against IAV.
Potential natural antivirals against IAV
The medicinal usage of plant extracts is no stranger, with well-known plant derivative drugs such as aspirin, digitoxin, morphine, and atropine circulating in the market (Kurokawa et al. 2010, Musarra-Pizzo et al. 2021). Given the prosperous past accompanied by the excellent efficacy and minimal to no side effects of plant extracts against various viruses, this review covers the anti-IAV activities of various plant extracts. Using either in vitro or in vivo studies or a combination of both studies, certain plant extracts have been shown to be promising sources of anti-IAV therapeutics (Serkedjieva and Velcheva 2003, Droebner et al. 2007, Ehrhardt et al. 2007, 2013, Pu et al. 2009, Saha et al. 2010, Cecil et al. 2011, Liu et al. 2011, Wu et al. 2011, 2015, Zhang et al. 2013, Jin et al. 2020, Alahmad et al. 2022, Juárez-Méndez et al. 2022, Lim et al. 2024) (Table 1).
Plants . | Phytochemicals or extracts . | Antiviral activities . | Reference . |
---|---|---|---|
Milk thistle (Silybum marianum (L.) Gaernt.) | Silymarin | • Reduced cytopathic effect (CPE) in A/PR/8/34-infected MDCK cells treated with 100 μg ml−1 of silymarin • Inhibition of late viral RNA synthesis | Song and Choi (2011) |
Silybin | • Inhibit IAV replication and reduce mortality in infected mice. • Inhibits the activation of ERK/p38-MAPK and IKK pathways, and the expression of Atg7 and Atg3 induced by IAV infection. | (Dai et al. 2013) | |
Chameleon plant (Houttuynia cordata) | Quercetin | • Reduced CPE in A/WS/33-infected MDCK cells treated with 10 μg ml−1 of quercetin-3-rhamnoside • Broad-spectrum anti-IAV activity with IC50 values ranging from 2.74 to 7.76 µg ml−1 in infected MDCK and A549 cells • Inhibition of both early infection stage and late stages of viral RNA synthesis | Song and Choi (2011), Wu et al. (2015) |
Baikal skullcap (Scutellaria baicalensis Georgi) | Baicalin | • Inhibition of viral NS1 bindings to host p85β protein, blocking NS1-mediated PI3K/Akt signalling, which typically support viral replication | Nayak et al. (2014) |
Baicalein | • Enhancement of antiviral signalling through IFN induction | Zhi et al. (2019) | |
Goldenseal (Hydrastis canadensis) | Berberine | • Inhibited growth of PR/8/34 and WS/33 strains in A549 cells, RAW 264.7 macrophage-like cells and murine bone marrow-derived macrophages • Interference with post-translational processes of viral protein trafficking and maturation • Disrupted production of inflammatory mediators TNF-α and PGE2, implicated in IAV pathogenesis and pathophysiology, respectively • Improved lung histopathology and mortality rates in mice infected with A/FM/1/47 (H1N1) | Cecil et al. (2011), Wu et al. (2011) |
Asiatic dayflower (Commelina communis L.) | Homonojirimycin | • Oral administration in PR/8/34-infected mice models showed improved survival rates and virus titers • Elevated serum and lung levels of IFN-γ and IL-10, with decreased TNF-α and IL-6 levels in mice, demonstrated immunomodulatory potential | Zhang et al. (2013) |
Mongolian plant (Thalictrum simplex) | Thalimonine | • Dose-dependent reduction in CPE and expression of viral proteins (HA, NA, and NP) in avian strains (A/Weybridge and A/Rostock) • EC90 values of 1.2 μmol l−¹ for A/Weybridge and 4.5 μmol l−¹ for A/Rostock | Serkedjieva and Velcheva (2003) |
Syrian rue (Peganum harmala L.) | β-carboline indole | • Harmalol, harmaline and harmane showed potent anti-IAV activity against avian H5N1 (IC50: 0.02, 3.42, and 0.023 µg ml−1) and human influenza A/H1N1 (IC50: 0.035, 0.033, and 0.056 µg ml−1) • Harmalol and harmane inhibited viral replication; while harmaline is virucidal | Hegazy et al. (2023) |
Dahurian angelica (Angelica dahurica) | Isoimperatorin and Oxypeucedanin | • Broad-spectrum anti-IAV efficacy • Inhibition of neuraminidase during late stages of the virus life cycle, causing virus yield reduction of 81.4%–84.6% in vitro | Lai et al. (2021) |
Oxypeucedanin | • Significantly inhibited the synthesis of IAV, NA, and nucleoprotein (NP) • Inhibits caspase-3 and Bax activity exploited by IAV, an excellent anti-apoptotic agent | Lee et al. (2020) | |
Siberian ginseng (Eleutherococcus senticosus) | Isofraxidin | • Intranasal administration in PR/8/34-infected mice improved lung histopathology and survival rates • Immunomodulatory potential due to the reduced serum levels of pro-inflammatory cytokines (TNF-α, IL-1β, IL-6, and MIP-2) • Suppressed platelet aggregation and reduced platelet activation markers’ expression | Jin et al. (2020) |
Cistus incanus extract (CYSTUS052) | Various polyphenols, primarily flavan-3-ols, and proanthocyanidins | • Broad-spectrum antiviral activity against PR/8/34 (prototype) and Thailand/1(KAN-1)/2004 (clinical isolate) by inhibiting viral uptake into host cells • Mice administered with aerosolized CYSTUS052 before infection conferred protective effects against infection | Droebner et al. (2007), Ehrhardt et al. (2007) |
Hypericum perforatum L. extract | Various polyphenols | • Reduced CPE in A/Gansu/1771/2006-infected MDCK cells with IC50 of 40 µg ml−1 • Oral administration in infected mice models showed improved arterial oxygen saturation and disease mortality, in addition to lowered virus titers • Upregulation of IL-10 and IFN-γ, and downregulation of IL-6 and TNF-α in lung tissue and serum of treated mice | Pu et al. (2009), Alahmad et al. (2022) |
Ribes nigrum folium | Various polyphenols | • Reduced replication of PR/8/34 and Nordrhein-Wesfalen/173/2009 (H1N1pan) in A549 cells when pre- and co-treated with LADANIA067, likely by disrupting virus internalisation | Ehrhardt et al. (2013) |
Diospyros anisandra | Various compounds | • Compounds in n-hexane extracts exhibited synergistic activity in inhibiting haemagglutinin of the AH1N1pdm09 virus | Juárez-Méndez et al. (2022) |
Amla (Phyllanthus emblica Linn) | Pentagalloyl-glucose | • A/WSN/33-infected MDCK and A549 cells showed reduced virus titers with IC50 of 2.36 ± 0.29 µg ml−1 • Inhibition of virus adsorption via haemagglutination and disruption of virus particle release | Liu et al. (2011) |
Pu'er tea (Camellia sinensis var. assamica) | Strictinin (ellagitannin) | • Inhibition of IAV replication in MDCK cells during co-treatment, with an IC50 range of 0.09–0.24 μmol l−¹, likely by targeting viral entry pathways | Saha et al. (2010) |
Red pitahaya (Hylocereus polyrhizus) | Betacyanin | • Downregulated IAV nucleoprotein expression by 1.519-fold at 24 h post-treatment • Reduced virus titers by 24% at 48 h and 43% at 72 h | Lim et al. (2024) |
Black elder (Sambucus nigra L.) | Cyanidin 3-sambubioside | • Prevented viral entry and transmission in the post-infectious stage • Inhibition of sialidase activity | Roschek et al. (2009), Kinoshita et al. (2012) |
Flavonoids | • Bound to IAV mannose-rich HA binding domain, blocked the host cell binding and recognition | Roschek et al. (2009), Swaminathan et al. (2013) | |
Acidic polysaccharide | • Induced dendritic cell (DC) maturation and T-cell stimulation • Enhanced inflammatory cytokine. IL-6, TNF-α and IFN-γ production supports T cell-mediated immune response • Promoted antibody production and suppressed viral replication | Stich et al. (2022), Tiralongo et al. (2016) | |
Rubini standardized elderberry extract | • Considerable titre reduction of IAV • Reduction in symptoms | Krawitz et al. (2011), Młynarczyk et al. (2018), Hudson (2012) | |
Purple coneflower (Echinacea purpurea (L.) Moench) | Echinaforce (EF)/Echinaforce Hotdrink (EFH) | • Inhibited viral entry into the host cells • Stimulated the release of pro-inflammatory cytokines • Prevented resistance in IAV in continuous usage • Increased recovery rate | Sharma et al. (2009), Ross (2016), Vimalanathan et al. (2013), Yoo et al. (2012) |
Alkylamides | • Stimulated anti-inflammatory IL-10 and suppress pro-inflammatory TNF-α production • Together with polysaccharides and circhoric acid, alkylamine enhanced the production of natural killer cells and white blood cells and stimulates the phagocytic activity of macrophages | Aucoin et al. (2021), Barnes et al. (2010), Goel et al. (2002), Rauš et al. (2015) | |
Red ginseng (Panax ginseng C.A.Mey.) | Red ginseng (RG) extract | • Suppressed pro-inflammatory cytokines such as IL-6 and IL-8. • Diminished reactive oxygen species in viral infected cells via inhibition of mitogen-activated protein kinase (MAPK) pathway. • Anti-oxidative effects gave rise to an increased survival rate | Chan et al. (2011), Lee et al. (2014), Yin et al. (2013) |
Saponins (Ginsenosides) | • Co-administration of saponins, RG extract, and inactivated flu vaccine stimulates higher antibody production • Prevented secondary IAV infection | Wang et al. (2018), Xu et al. (2012) | |
Polysaccharides | • Prevented excessive immune stimulation, reducing disease symptoms | Yin et al. (2013), Xu et al. (2012) | |
Green tea (Camellia sinensis (L.) Kuntze) | Catechin (EGCG and ECG) | • Prophylactic effect in preventing IAV infection • Bound to neuraminidase (NA) residue, obstructing sialic acid binding and preventing the release of new virions • Inhibits the internalization of NA into host cells, preventing viral penetration and entry. • Upregulated IFN-λ2 and induced human β-defensin 3 (HBD3) via the p38 MAPK signalling pathway, stimulating cellular response and cytokine production against IAV • Enhanced CD4 + and natural killer cell activity, producing a potent cytotoxic effect on infected cells. | Nanri et al. (2021), Kim et al. (2016), Sharma et al. (2017), Mou et al. (2020), Low et al. (2020) |
Plants . | Phytochemicals or extracts . | Antiviral activities . | Reference . |
---|---|---|---|
Milk thistle (Silybum marianum (L.) Gaernt.) | Silymarin | • Reduced cytopathic effect (CPE) in A/PR/8/34-infected MDCK cells treated with 100 μg ml−1 of silymarin • Inhibition of late viral RNA synthesis | Song and Choi (2011) |
Silybin | • Inhibit IAV replication and reduce mortality in infected mice. • Inhibits the activation of ERK/p38-MAPK and IKK pathways, and the expression of Atg7 and Atg3 induced by IAV infection. | (Dai et al. 2013) | |
Chameleon plant (Houttuynia cordata) | Quercetin | • Reduced CPE in A/WS/33-infected MDCK cells treated with 10 μg ml−1 of quercetin-3-rhamnoside • Broad-spectrum anti-IAV activity with IC50 values ranging from 2.74 to 7.76 µg ml−1 in infected MDCK and A549 cells • Inhibition of both early infection stage and late stages of viral RNA synthesis | Song and Choi (2011), Wu et al. (2015) |
Baikal skullcap (Scutellaria baicalensis Georgi) | Baicalin | • Inhibition of viral NS1 bindings to host p85β protein, blocking NS1-mediated PI3K/Akt signalling, which typically support viral replication | Nayak et al. (2014) |
Baicalein | • Enhancement of antiviral signalling through IFN induction | Zhi et al. (2019) | |
Goldenseal (Hydrastis canadensis) | Berberine | • Inhibited growth of PR/8/34 and WS/33 strains in A549 cells, RAW 264.7 macrophage-like cells and murine bone marrow-derived macrophages • Interference with post-translational processes of viral protein trafficking and maturation • Disrupted production of inflammatory mediators TNF-α and PGE2, implicated in IAV pathogenesis and pathophysiology, respectively • Improved lung histopathology and mortality rates in mice infected with A/FM/1/47 (H1N1) | Cecil et al. (2011), Wu et al. (2011) |
Asiatic dayflower (Commelina communis L.) | Homonojirimycin | • Oral administration in PR/8/34-infected mice models showed improved survival rates and virus titers • Elevated serum and lung levels of IFN-γ and IL-10, with decreased TNF-α and IL-6 levels in mice, demonstrated immunomodulatory potential | Zhang et al. (2013) |
Mongolian plant (Thalictrum simplex) | Thalimonine | • Dose-dependent reduction in CPE and expression of viral proteins (HA, NA, and NP) in avian strains (A/Weybridge and A/Rostock) • EC90 values of 1.2 μmol l−¹ for A/Weybridge and 4.5 μmol l−¹ for A/Rostock | Serkedjieva and Velcheva (2003) |
Syrian rue (Peganum harmala L.) | β-carboline indole | • Harmalol, harmaline and harmane showed potent anti-IAV activity against avian H5N1 (IC50: 0.02, 3.42, and 0.023 µg ml−1) and human influenza A/H1N1 (IC50: 0.035, 0.033, and 0.056 µg ml−1) • Harmalol and harmane inhibited viral replication; while harmaline is virucidal | Hegazy et al. (2023) |
Dahurian angelica (Angelica dahurica) | Isoimperatorin and Oxypeucedanin | • Broad-spectrum anti-IAV efficacy • Inhibition of neuraminidase during late stages of the virus life cycle, causing virus yield reduction of 81.4%–84.6% in vitro | Lai et al. (2021) |
Oxypeucedanin | • Significantly inhibited the synthesis of IAV, NA, and nucleoprotein (NP) • Inhibits caspase-3 and Bax activity exploited by IAV, an excellent anti-apoptotic agent | Lee et al. (2020) | |
Siberian ginseng (Eleutherococcus senticosus) | Isofraxidin | • Intranasal administration in PR/8/34-infected mice improved lung histopathology and survival rates • Immunomodulatory potential due to the reduced serum levels of pro-inflammatory cytokines (TNF-α, IL-1β, IL-6, and MIP-2) • Suppressed platelet aggregation and reduced platelet activation markers’ expression | Jin et al. (2020) |
Cistus incanus extract (CYSTUS052) | Various polyphenols, primarily flavan-3-ols, and proanthocyanidins | • Broad-spectrum antiviral activity against PR/8/34 (prototype) and Thailand/1(KAN-1)/2004 (clinical isolate) by inhibiting viral uptake into host cells • Mice administered with aerosolized CYSTUS052 before infection conferred protective effects against infection | Droebner et al. (2007), Ehrhardt et al. (2007) |
Hypericum perforatum L. extract | Various polyphenols | • Reduced CPE in A/Gansu/1771/2006-infected MDCK cells with IC50 of 40 µg ml−1 • Oral administration in infected mice models showed improved arterial oxygen saturation and disease mortality, in addition to lowered virus titers • Upregulation of IL-10 and IFN-γ, and downregulation of IL-6 and TNF-α in lung tissue and serum of treated mice | Pu et al. (2009), Alahmad et al. (2022) |
Ribes nigrum folium | Various polyphenols | • Reduced replication of PR/8/34 and Nordrhein-Wesfalen/173/2009 (H1N1pan) in A549 cells when pre- and co-treated with LADANIA067, likely by disrupting virus internalisation | Ehrhardt et al. (2013) |
Diospyros anisandra | Various compounds | • Compounds in n-hexane extracts exhibited synergistic activity in inhibiting haemagglutinin of the AH1N1pdm09 virus | Juárez-Méndez et al. (2022) |
Amla (Phyllanthus emblica Linn) | Pentagalloyl-glucose | • A/WSN/33-infected MDCK and A549 cells showed reduced virus titers with IC50 of 2.36 ± 0.29 µg ml−1 • Inhibition of virus adsorption via haemagglutination and disruption of virus particle release | Liu et al. (2011) |
Pu'er tea (Camellia sinensis var. assamica) | Strictinin (ellagitannin) | • Inhibition of IAV replication in MDCK cells during co-treatment, with an IC50 range of 0.09–0.24 μmol l−¹, likely by targeting viral entry pathways | Saha et al. (2010) |
Red pitahaya (Hylocereus polyrhizus) | Betacyanin | • Downregulated IAV nucleoprotein expression by 1.519-fold at 24 h post-treatment • Reduced virus titers by 24% at 48 h and 43% at 72 h | Lim et al. (2024) |
Black elder (Sambucus nigra L.) | Cyanidin 3-sambubioside | • Prevented viral entry and transmission in the post-infectious stage • Inhibition of sialidase activity | Roschek et al. (2009), Kinoshita et al. (2012) |
Flavonoids | • Bound to IAV mannose-rich HA binding domain, blocked the host cell binding and recognition | Roschek et al. (2009), Swaminathan et al. (2013) | |
Acidic polysaccharide | • Induced dendritic cell (DC) maturation and T-cell stimulation • Enhanced inflammatory cytokine. IL-6, TNF-α and IFN-γ production supports T cell-mediated immune response • Promoted antibody production and suppressed viral replication | Stich et al. (2022), Tiralongo et al. (2016) | |
Rubini standardized elderberry extract | • Considerable titre reduction of IAV • Reduction in symptoms | Krawitz et al. (2011), Młynarczyk et al. (2018), Hudson (2012) | |
Purple coneflower (Echinacea purpurea (L.) Moench) | Echinaforce (EF)/Echinaforce Hotdrink (EFH) | • Inhibited viral entry into the host cells • Stimulated the release of pro-inflammatory cytokines • Prevented resistance in IAV in continuous usage • Increased recovery rate | Sharma et al. (2009), Ross (2016), Vimalanathan et al. (2013), Yoo et al. (2012) |
Alkylamides | • Stimulated anti-inflammatory IL-10 and suppress pro-inflammatory TNF-α production • Together with polysaccharides and circhoric acid, alkylamine enhanced the production of natural killer cells and white blood cells and stimulates the phagocytic activity of macrophages | Aucoin et al. (2021), Barnes et al. (2010), Goel et al. (2002), Rauš et al. (2015) | |
Red ginseng (Panax ginseng C.A.Mey.) | Red ginseng (RG) extract | • Suppressed pro-inflammatory cytokines such as IL-6 and IL-8. • Diminished reactive oxygen species in viral infected cells via inhibition of mitogen-activated protein kinase (MAPK) pathway. • Anti-oxidative effects gave rise to an increased survival rate | Chan et al. (2011), Lee et al. (2014), Yin et al. (2013) |
Saponins (Ginsenosides) | • Co-administration of saponins, RG extract, and inactivated flu vaccine stimulates higher antibody production • Prevented secondary IAV infection | Wang et al. (2018), Xu et al. (2012) | |
Polysaccharides | • Prevented excessive immune stimulation, reducing disease symptoms | Yin et al. (2013), Xu et al. (2012) | |
Green tea (Camellia sinensis (L.) Kuntze) | Catechin (EGCG and ECG) | • Prophylactic effect in preventing IAV infection • Bound to neuraminidase (NA) residue, obstructing sialic acid binding and preventing the release of new virions • Inhibits the internalization of NA into host cells, preventing viral penetration and entry. • Upregulated IFN-λ2 and induced human β-defensin 3 (HBD3) via the p38 MAPK signalling pathway, stimulating cellular response and cytokine production against IAV • Enhanced CD4 + and natural killer cell activity, producing a potent cytotoxic effect on infected cells. | Nanri et al. (2021), Kim et al. (2016), Sharma et al. (2017), Mou et al. (2020), Low et al. (2020) |
Plants . | Phytochemicals or extracts . | Antiviral activities . | Reference . |
---|---|---|---|
Milk thistle (Silybum marianum (L.) Gaernt.) | Silymarin | • Reduced cytopathic effect (CPE) in A/PR/8/34-infected MDCK cells treated with 100 μg ml−1 of silymarin • Inhibition of late viral RNA synthesis | Song and Choi (2011) |
Silybin | • Inhibit IAV replication and reduce mortality in infected mice. • Inhibits the activation of ERK/p38-MAPK and IKK pathways, and the expression of Atg7 and Atg3 induced by IAV infection. | (Dai et al. 2013) | |
Chameleon plant (Houttuynia cordata) | Quercetin | • Reduced CPE in A/WS/33-infected MDCK cells treated with 10 μg ml−1 of quercetin-3-rhamnoside • Broad-spectrum anti-IAV activity with IC50 values ranging from 2.74 to 7.76 µg ml−1 in infected MDCK and A549 cells • Inhibition of both early infection stage and late stages of viral RNA synthesis | Song and Choi (2011), Wu et al. (2015) |
Baikal skullcap (Scutellaria baicalensis Georgi) | Baicalin | • Inhibition of viral NS1 bindings to host p85β protein, blocking NS1-mediated PI3K/Akt signalling, which typically support viral replication | Nayak et al. (2014) |
Baicalein | • Enhancement of antiviral signalling through IFN induction | Zhi et al. (2019) | |
Goldenseal (Hydrastis canadensis) | Berberine | • Inhibited growth of PR/8/34 and WS/33 strains in A549 cells, RAW 264.7 macrophage-like cells and murine bone marrow-derived macrophages • Interference with post-translational processes of viral protein trafficking and maturation • Disrupted production of inflammatory mediators TNF-α and PGE2, implicated in IAV pathogenesis and pathophysiology, respectively • Improved lung histopathology and mortality rates in mice infected with A/FM/1/47 (H1N1) | Cecil et al. (2011), Wu et al. (2011) |
Asiatic dayflower (Commelina communis L.) | Homonojirimycin | • Oral administration in PR/8/34-infected mice models showed improved survival rates and virus titers • Elevated serum and lung levels of IFN-γ and IL-10, with decreased TNF-α and IL-6 levels in mice, demonstrated immunomodulatory potential | Zhang et al. (2013) |
Mongolian plant (Thalictrum simplex) | Thalimonine | • Dose-dependent reduction in CPE and expression of viral proteins (HA, NA, and NP) in avian strains (A/Weybridge and A/Rostock) • EC90 values of 1.2 μmol l−¹ for A/Weybridge and 4.5 μmol l−¹ for A/Rostock | Serkedjieva and Velcheva (2003) |
Syrian rue (Peganum harmala L.) | β-carboline indole | • Harmalol, harmaline and harmane showed potent anti-IAV activity against avian H5N1 (IC50: 0.02, 3.42, and 0.023 µg ml−1) and human influenza A/H1N1 (IC50: 0.035, 0.033, and 0.056 µg ml−1) • Harmalol and harmane inhibited viral replication; while harmaline is virucidal | Hegazy et al. (2023) |
Dahurian angelica (Angelica dahurica) | Isoimperatorin and Oxypeucedanin | • Broad-spectrum anti-IAV efficacy • Inhibition of neuraminidase during late stages of the virus life cycle, causing virus yield reduction of 81.4%–84.6% in vitro | Lai et al. (2021) |
Oxypeucedanin | • Significantly inhibited the synthesis of IAV, NA, and nucleoprotein (NP) • Inhibits caspase-3 and Bax activity exploited by IAV, an excellent anti-apoptotic agent | Lee et al. (2020) | |
Siberian ginseng (Eleutherococcus senticosus) | Isofraxidin | • Intranasal administration in PR/8/34-infected mice improved lung histopathology and survival rates • Immunomodulatory potential due to the reduced serum levels of pro-inflammatory cytokines (TNF-α, IL-1β, IL-6, and MIP-2) • Suppressed platelet aggregation and reduced platelet activation markers’ expression | Jin et al. (2020) |
Cistus incanus extract (CYSTUS052) | Various polyphenols, primarily flavan-3-ols, and proanthocyanidins | • Broad-spectrum antiviral activity against PR/8/34 (prototype) and Thailand/1(KAN-1)/2004 (clinical isolate) by inhibiting viral uptake into host cells • Mice administered with aerosolized CYSTUS052 before infection conferred protective effects against infection | Droebner et al. (2007), Ehrhardt et al. (2007) |
Hypericum perforatum L. extract | Various polyphenols | • Reduced CPE in A/Gansu/1771/2006-infected MDCK cells with IC50 of 40 µg ml−1 • Oral administration in infected mice models showed improved arterial oxygen saturation and disease mortality, in addition to lowered virus titers • Upregulation of IL-10 and IFN-γ, and downregulation of IL-6 and TNF-α in lung tissue and serum of treated mice | Pu et al. (2009), Alahmad et al. (2022) |
Ribes nigrum folium | Various polyphenols | • Reduced replication of PR/8/34 and Nordrhein-Wesfalen/173/2009 (H1N1pan) in A549 cells when pre- and co-treated with LADANIA067, likely by disrupting virus internalisation | Ehrhardt et al. (2013) |
Diospyros anisandra | Various compounds | • Compounds in n-hexane extracts exhibited synergistic activity in inhibiting haemagglutinin of the AH1N1pdm09 virus | Juárez-Méndez et al. (2022) |
Amla (Phyllanthus emblica Linn) | Pentagalloyl-glucose | • A/WSN/33-infected MDCK and A549 cells showed reduced virus titers with IC50 of 2.36 ± 0.29 µg ml−1 • Inhibition of virus adsorption via haemagglutination and disruption of virus particle release | Liu et al. (2011) |
Pu'er tea (Camellia sinensis var. assamica) | Strictinin (ellagitannin) | • Inhibition of IAV replication in MDCK cells during co-treatment, with an IC50 range of 0.09–0.24 μmol l−¹, likely by targeting viral entry pathways | Saha et al. (2010) |
Red pitahaya (Hylocereus polyrhizus) | Betacyanin | • Downregulated IAV nucleoprotein expression by 1.519-fold at 24 h post-treatment • Reduced virus titers by 24% at 48 h and 43% at 72 h | Lim et al. (2024) |
Black elder (Sambucus nigra L.) | Cyanidin 3-sambubioside | • Prevented viral entry and transmission in the post-infectious stage • Inhibition of sialidase activity | Roschek et al. (2009), Kinoshita et al. (2012) |
Flavonoids | • Bound to IAV mannose-rich HA binding domain, blocked the host cell binding and recognition | Roschek et al. (2009), Swaminathan et al. (2013) | |
Acidic polysaccharide | • Induced dendritic cell (DC) maturation and T-cell stimulation • Enhanced inflammatory cytokine. IL-6, TNF-α and IFN-γ production supports T cell-mediated immune response • Promoted antibody production and suppressed viral replication | Stich et al. (2022), Tiralongo et al. (2016) | |
Rubini standardized elderberry extract | • Considerable titre reduction of IAV • Reduction in symptoms | Krawitz et al. (2011), Młynarczyk et al. (2018), Hudson (2012) | |
Purple coneflower (Echinacea purpurea (L.) Moench) | Echinaforce (EF)/Echinaforce Hotdrink (EFH) | • Inhibited viral entry into the host cells • Stimulated the release of pro-inflammatory cytokines • Prevented resistance in IAV in continuous usage • Increased recovery rate | Sharma et al. (2009), Ross (2016), Vimalanathan et al. (2013), Yoo et al. (2012) |
Alkylamides | • Stimulated anti-inflammatory IL-10 and suppress pro-inflammatory TNF-α production • Together with polysaccharides and circhoric acid, alkylamine enhanced the production of natural killer cells and white blood cells and stimulates the phagocytic activity of macrophages | Aucoin et al. (2021), Barnes et al. (2010), Goel et al. (2002), Rauš et al. (2015) | |
Red ginseng (Panax ginseng C.A.Mey.) | Red ginseng (RG) extract | • Suppressed pro-inflammatory cytokines such as IL-6 and IL-8. • Diminished reactive oxygen species in viral infected cells via inhibition of mitogen-activated protein kinase (MAPK) pathway. • Anti-oxidative effects gave rise to an increased survival rate | Chan et al. (2011), Lee et al. (2014), Yin et al. (2013) |
Saponins (Ginsenosides) | • Co-administration of saponins, RG extract, and inactivated flu vaccine stimulates higher antibody production • Prevented secondary IAV infection | Wang et al. (2018), Xu et al. (2012) | |
Polysaccharides | • Prevented excessive immune stimulation, reducing disease symptoms | Yin et al. (2013), Xu et al. (2012) | |
Green tea (Camellia sinensis (L.) Kuntze) | Catechin (EGCG and ECG) | • Prophylactic effect in preventing IAV infection • Bound to neuraminidase (NA) residue, obstructing sialic acid binding and preventing the release of new virions • Inhibits the internalization of NA into host cells, preventing viral penetration and entry. • Upregulated IFN-λ2 and induced human β-defensin 3 (HBD3) via the p38 MAPK signalling pathway, stimulating cellular response and cytokine production against IAV • Enhanced CD4 + and natural killer cell activity, producing a potent cytotoxic effect on infected cells. | Nanri et al. (2021), Kim et al. (2016), Sharma et al. (2017), Mou et al. (2020), Low et al. (2020) |
Plants . | Phytochemicals or extracts . | Antiviral activities . | Reference . |
---|---|---|---|
Milk thistle (Silybum marianum (L.) Gaernt.) | Silymarin | • Reduced cytopathic effect (CPE) in A/PR/8/34-infected MDCK cells treated with 100 μg ml−1 of silymarin • Inhibition of late viral RNA synthesis | Song and Choi (2011) |
Silybin | • Inhibit IAV replication and reduce mortality in infected mice. • Inhibits the activation of ERK/p38-MAPK and IKK pathways, and the expression of Atg7 and Atg3 induced by IAV infection. | (Dai et al. 2013) | |
Chameleon plant (Houttuynia cordata) | Quercetin | • Reduced CPE in A/WS/33-infected MDCK cells treated with 10 μg ml−1 of quercetin-3-rhamnoside • Broad-spectrum anti-IAV activity with IC50 values ranging from 2.74 to 7.76 µg ml−1 in infected MDCK and A549 cells • Inhibition of both early infection stage and late stages of viral RNA synthesis | Song and Choi (2011), Wu et al. (2015) |
Baikal skullcap (Scutellaria baicalensis Georgi) | Baicalin | • Inhibition of viral NS1 bindings to host p85β protein, blocking NS1-mediated PI3K/Akt signalling, which typically support viral replication | Nayak et al. (2014) |
Baicalein | • Enhancement of antiviral signalling through IFN induction | Zhi et al. (2019) | |
Goldenseal (Hydrastis canadensis) | Berberine | • Inhibited growth of PR/8/34 and WS/33 strains in A549 cells, RAW 264.7 macrophage-like cells and murine bone marrow-derived macrophages • Interference with post-translational processes of viral protein trafficking and maturation • Disrupted production of inflammatory mediators TNF-α and PGE2, implicated in IAV pathogenesis and pathophysiology, respectively • Improved lung histopathology and mortality rates in mice infected with A/FM/1/47 (H1N1) | Cecil et al. (2011), Wu et al. (2011) |
Asiatic dayflower (Commelina communis L.) | Homonojirimycin | • Oral administration in PR/8/34-infected mice models showed improved survival rates and virus titers • Elevated serum and lung levels of IFN-γ and IL-10, with decreased TNF-α and IL-6 levels in mice, demonstrated immunomodulatory potential | Zhang et al. (2013) |
Mongolian plant (Thalictrum simplex) | Thalimonine | • Dose-dependent reduction in CPE and expression of viral proteins (HA, NA, and NP) in avian strains (A/Weybridge and A/Rostock) • EC90 values of 1.2 μmol l−¹ for A/Weybridge and 4.5 μmol l−¹ for A/Rostock | Serkedjieva and Velcheva (2003) |
Syrian rue (Peganum harmala L.) | β-carboline indole | • Harmalol, harmaline and harmane showed potent anti-IAV activity against avian H5N1 (IC50: 0.02, 3.42, and 0.023 µg ml−1) and human influenza A/H1N1 (IC50: 0.035, 0.033, and 0.056 µg ml−1) • Harmalol and harmane inhibited viral replication; while harmaline is virucidal | Hegazy et al. (2023) |
Dahurian angelica (Angelica dahurica) | Isoimperatorin and Oxypeucedanin | • Broad-spectrum anti-IAV efficacy • Inhibition of neuraminidase during late stages of the virus life cycle, causing virus yield reduction of 81.4%–84.6% in vitro | Lai et al. (2021) |
Oxypeucedanin | • Significantly inhibited the synthesis of IAV, NA, and nucleoprotein (NP) • Inhibits caspase-3 and Bax activity exploited by IAV, an excellent anti-apoptotic agent | Lee et al. (2020) | |
Siberian ginseng (Eleutherococcus senticosus) | Isofraxidin | • Intranasal administration in PR/8/34-infected mice improved lung histopathology and survival rates • Immunomodulatory potential due to the reduced serum levels of pro-inflammatory cytokines (TNF-α, IL-1β, IL-6, and MIP-2) • Suppressed platelet aggregation and reduced platelet activation markers’ expression | Jin et al. (2020) |
Cistus incanus extract (CYSTUS052) | Various polyphenols, primarily flavan-3-ols, and proanthocyanidins | • Broad-spectrum antiviral activity against PR/8/34 (prototype) and Thailand/1(KAN-1)/2004 (clinical isolate) by inhibiting viral uptake into host cells • Mice administered with aerosolized CYSTUS052 before infection conferred protective effects against infection | Droebner et al. (2007), Ehrhardt et al. (2007) |
Hypericum perforatum L. extract | Various polyphenols | • Reduced CPE in A/Gansu/1771/2006-infected MDCK cells with IC50 of 40 µg ml−1 • Oral administration in infected mice models showed improved arterial oxygen saturation and disease mortality, in addition to lowered virus titers • Upregulation of IL-10 and IFN-γ, and downregulation of IL-6 and TNF-α in lung tissue and serum of treated mice | Pu et al. (2009), Alahmad et al. (2022) |
Ribes nigrum folium | Various polyphenols | • Reduced replication of PR/8/34 and Nordrhein-Wesfalen/173/2009 (H1N1pan) in A549 cells when pre- and co-treated with LADANIA067, likely by disrupting virus internalisation | Ehrhardt et al. (2013) |
Diospyros anisandra | Various compounds | • Compounds in n-hexane extracts exhibited synergistic activity in inhibiting haemagglutinin of the AH1N1pdm09 virus | Juárez-Méndez et al. (2022) |
Amla (Phyllanthus emblica Linn) | Pentagalloyl-glucose | • A/WSN/33-infected MDCK and A549 cells showed reduced virus titers with IC50 of 2.36 ± 0.29 µg ml−1 • Inhibition of virus adsorption via haemagglutination and disruption of virus particle release | Liu et al. (2011) |
Pu'er tea (Camellia sinensis var. assamica) | Strictinin (ellagitannin) | • Inhibition of IAV replication in MDCK cells during co-treatment, with an IC50 range of 0.09–0.24 μmol l−¹, likely by targeting viral entry pathways | Saha et al. (2010) |
Red pitahaya (Hylocereus polyrhizus) | Betacyanin | • Downregulated IAV nucleoprotein expression by 1.519-fold at 24 h post-treatment • Reduced virus titers by 24% at 48 h and 43% at 72 h | Lim et al. (2024) |
Black elder (Sambucus nigra L.) | Cyanidin 3-sambubioside | • Prevented viral entry and transmission in the post-infectious stage • Inhibition of sialidase activity | Roschek et al. (2009), Kinoshita et al. (2012) |
Flavonoids | • Bound to IAV mannose-rich HA binding domain, blocked the host cell binding and recognition | Roschek et al. (2009), Swaminathan et al. (2013) | |
Acidic polysaccharide | • Induced dendritic cell (DC) maturation and T-cell stimulation • Enhanced inflammatory cytokine. IL-6, TNF-α and IFN-γ production supports T cell-mediated immune response • Promoted antibody production and suppressed viral replication | Stich et al. (2022), Tiralongo et al. (2016) | |
Rubini standardized elderberry extract | • Considerable titre reduction of IAV • Reduction in symptoms | Krawitz et al. (2011), Młynarczyk et al. (2018), Hudson (2012) | |
Purple coneflower (Echinacea purpurea (L.) Moench) | Echinaforce (EF)/Echinaforce Hotdrink (EFH) | • Inhibited viral entry into the host cells • Stimulated the release of pro-inflammatory cytokines • Prevented resistance in IAV in continuous usage • Increased recovery rate | Sharma et al. (2009), Ross (2016), Vimalanathan et al. (2013), Yoo et al. (2012) |
Alkylamides | • Stimulated anti-inflammatory IL-10 and suppress pro-inflammatory TNF-α production • Together with polysaccharides and circhoric acid, alkylamine enhanced the production of natural killer cells and white blood cells and stimulates the phagocytic activity of macrophages | Aucoin et al. (2021), Barnes et al. (2010), Goel et al. (2002), Rauš et al. (2015) | |
Red ginseng (Panax ginseng C.A.Mey.) | Red ginseng (RG) extract | • Suppressed pro-inflammatory cytokines such as IL-6 and IL-8. • Diminished reactive oxygen species in viral infected cells via inhibition of mitogen-activated protein kinase (MAPK) pathway. • Anti-oxidative effects gave rise to an increased survival rate | Chan et al. (2011), Lee et al. (2014), Yin et al. (2013) |
Saponins (Ginsenosides) | • Co-administration of saponins, RG extract, and inactivated flu vaccine stimulates higher antibody production • Prevented secondary IAV infection | Wang et al. (2018), Xu et al. (2012) | |
Polysaccharides | • Prevented excessive immune stimulation, reducing disease symptoms | Yin et al. (2013), Xu et al. (2012) | |
Green tea (Camellia sinensis (L.) Kuntze) | Catechin (EGCG and ECG) | • Prophylactic effect in preventing IAV infection • Bound to neuraminidase (NA) residue, obstructing sialic acid binding and preventing the release of new virions • Inhibits the internalization of NA into host cells, preventing viral penetration and entry. • Upregulated IFN-λ2 and induced human β-defensin 3 (HBD3) via the p38 MAPK signalling pathway, stimulating cellular response and cytokine production against IAV • Enhanced CD4 + and natural killer cell activity, producing a potent cytotoxic effect on infected cells. | Nanri et al. (2021), Kim et al. (2016), Sharma et al. (2017), Mou et al. (2020), Low et al. (2020) |
Milk thistle (Si. marianum (L.) Gaernt)
Silybum marianum (L.) Gaertn., more commonly known as the milk thistle (MT), is a distinctive purple-flowered Mediterranean plant under the family of Asteraceae (Abenavoli et al. 2018). Therapeutic uses of MT have been well-established for centuries, primarily for treating liver and biliary tract diseases due to its hepatoprotectant and antioxidant activities (Sayin et al. 2016). The primary bioactive components of MT come from the seeds, which contain many flavanolignans compounds such as silybin, silychristin, silydianin A and B, isosilybin A and B, dehydrosilybin, and minor amounts of quercetin and taxifolin, with mixtures of silybin A and silybin B that make up for silibinin, a major active constituent of silymarin (Bijak 2017, Liu et al. 2019). Recently, many studies have also reported the great potential of MT in treating metabolic syndromes such as fatty liver, hypercholesterolaemia, and diabetes mellitus with the capacity to increase β-cells regeneration, improve insulin sensitivity, inhibit gluconeogenesis, exhibit potent antioxidant, and anti-inflammatory activity, to name a few (Tajmohammadi et al. 2018).
Going in-depth, the major bioactive compounds mentioned in MT extract mainly revolve around treating liver disease. For instance, silybin/silibinin was found to be an effective radical scavenger, potent lipid peroxidation inhibitor, and a great antifibrotic agent in non-alcoholic fatty liver disease (Salvoza et al. 2022, Nasiri-Ansari and Kassi 2023). Apart from that, silybin was reported to mitigate lipopolysaccharide (LPS)-induced inflammation by regulating the NF-κB pathway in porcine mammary epithelial cells, reducing the expression of proinflammatory cytokines and factors (Surai et al. 2024). Silydianin, on the other hand, is reported to be more effective than silymarin in enhancing endogenous antioxidant levels like glutathione peroxidase, making it a potent hepatoprotective agent (Anthony and Saleh 2013). Similarly, silychristin and isosilybin were cited to be more active than silybin in free radical scavenger potential, making them potent antioxidant and hepatoprotective agents (Taleb et al. 2018). Interestingly, isosilybin was also reported to be a potential candidate for anticancer therapeutics where isosilybin A and B successfully inhibited cell growth and promoted cell death with strong cell-cycle G1 arrest in human prostate carcinoma LNCaP and 22Rv1 cells (Deep et al. 2007). Silymarin complex is no different than the others; it possesses strong antioxidant, hepatoprotective, antifibrotic, and anticancer properties (Ranjan and Gautam 2023). In addition, there have been reports pointing towards the prospects of silymarin for treating neurological disorders, mainly brain disorders, including psychiatric, neurodegenerative and cognitive disorders (Ranjan and Gautam 2023).
Owing to its great pharmacological value, this light brown compound is further studied beyond typical treatment for the liver, such as antiviral treatment for hepatitis C virus, dengue virus, chikungunya virus, and IAV, to name a few (Liu et al. 2019). However, there has been a limited report, and the mechanism of how it works has yet to be unravelled. For instance, silymarin (Fig. 1) exhibited 98% inhibitory activity against the influenza A/PR/8/34 virus at 100 μg ml−1, a staggering contrast compared to the conventional oseltamivir, which showed only 52% inhibition at the same concentration (Song and Choi 2011). In addition, silybin was also found to inhibit IAV replication and reduce mortality in infected mice (Dai et al. 2013). The same study indicates silybin (Fig. 2) reduces oxidative stress, inhibits the activation of extracellular signal-regulated kinase (ERK)/p38 MAPK and IκB kinase (IKK) pathways, and the expression of autophagy-related proteins (Atg) such as Atg7 and Atg3 induced by IAV infection (Dai et al. 2013).
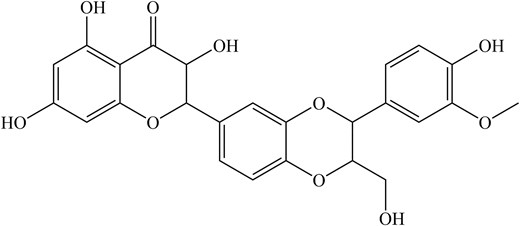
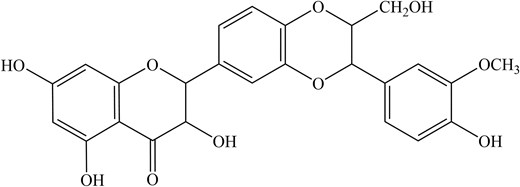
In summary, the silymarin and silybin from MT inhibit IAV replication and potentially inhibit the oxidative stress-related gene, subsequently decreasing the expression of autophagic-related genes induced by IAV infection. A summary of phytochemical compounds in MT and their antiviral properties is shown in Table 1.
Chinese skullcap (Sc. baicalensis Georgi)
Scutellaria baicalensis Georgi, also known as the Baikal skullcap or Chinese skullcap (CS), is a blue, purplish-red, or purple-flowered plant under the family of Lamiaceae and primarily found in Korea, Mongolia, Russia, China, and other south Asian countries (Zhao et al. 2016). The therapeutic use of CS has been well-traced for more than two centuries, primarily by the Chinese to treat colds, diarrhoea, dysentery, hypertension, haemorrhaging, insomnia, inflammation, respiratory infections, and lung and liver complications mainly due to its excellent hepatoprotective, antibacterial, and antiviral activities (Zhao et al. 2016, Chanchal et al. 2023). The principal bioactive compounds of MT are baicalin and aglycone baicalein, both coming from the roots (Chanchal et al. 2023). Other notable compounds from CS include the wogonin, wogonoside, chrysin, and oroxylin A, to name a few (Li-Weber 2009). To date, more than 50 flavonoids and their glycosides have been identified in CS, mainly in the roots (Wang et al. 2019, Chanchal et al. 2023). In addition to the traditional therapeutic applications, recent studies have revealed the great potential of CS in anticancer, anti-inflammation, antioxidant, and strong neuroprotective effects, to name a few (Dzięcioł et al. 2024).
As mentioned above, the pharmacological effects of CS were mainly antibacterial, hepatoprotective, and antiviral, with recent indications of immunization, anticancer, neuroprotective, cardiovascular improvement, and anti-inflammatory effects (Song et al. 2020). Notably, there is emerging evidence of CS on anti-ageing, anti-osteoporosis, anti-prostatic hyperplasia, anti-Alzheimer, anti-melanin, and anti-pruritic (Song et al. 2020). Diving into the phytochemical compounds, both wogonin and wogonoside have been shown to suppress inflammation and cancer growth such as human lung epithelial, breast and colorectal cancer, and the latter inhibits viral replication such as hepatitis B virus (HBV), human papillomavirus, and Varicella-zoster virus (Yang et al. 2013, Li et al. 2015, Huynh et al. 2020). Wogonin also scavenges free radicles, a potent neuroprotective and hepatoprotective agent that protects cells from damage (Zahra et al. 2019). Similarly, oroxylin A (OA) has been reported as a synergistic anticancer agent to 5-fluorouracil, enhances the inhibition of HT-29 tumour growth murine hepatoma 22 (H22) in vitro and in vivo, respectively (Tuli et al. 2023). The potent antioxidant properties of OA are also well-documented, with excellent cytoprotective effects against induced oxidative damage in human umbilical vein endothelial cells (Sajeev et al. 2022). Chrysin, a precursor to synthesis wogonin, baicalein, baicalin, and wogonoside, similarly exhibits anticancer properties with well-established studies showing effective suppression of prostate, breast, lung, liver, colon, and pancreatic cancers, to name a few (Gharari et al. 2020, Stompor-Gorący et al. 2021). Recently, chrysin has also been reported to exhibit anti-inflammatory activity in vitro and in vivo, with the latter showing attenuated synovial inflammation and pain-related factors in mice (Liu et al. 2014, Liao et al. 2020). Apart from that, there is emerging evidence of chrysin’s neuroprotective potential, including providing great prospects in treating a plethora variety of neurological disorders, such as Alzheimer’s and Parkinson’s disease, epilepsy, and multiple sclerosis, to name a few (Mishra et al. 2021).
Looking into the antiviral aspect, these are mainly attributed to the main phytochemical compounds, baicalin and baicalein. Despite that, there have been increasing reports of antiviral properties in chrysin and oroxylin A (Zhi et al. 2019). For instance, baicalin (Fig. 3), a flavonoid, has been shown to disrupt IAV-host interaction where NS1-p85β protein binding was inhibited, counteracting the NS1-mediated PI3K/Akt signalling, which was used to facilitate better viral replication (Nayak et al. 2014). Notably, baicalin can suppress IAV NS1-induced cell cycle arrest in the G0/G1 phase, which was exploited for favourable viral protein accumulation and replication via downregulation of P53 and P21 and upregulating Cyclin D1/CDK4 and Cyclin E1/CDK2 (Jiang et al. 2013, Liu et al. 2022). Besides that, both baicalin and baicalein (Fig. 4) also induce IFN signalling, enhancing antiviral signalling against IAV-infected cells (Nayak et al. 2014, Zhi et al. 2019).
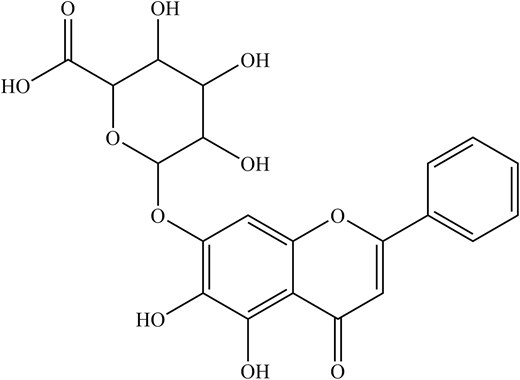
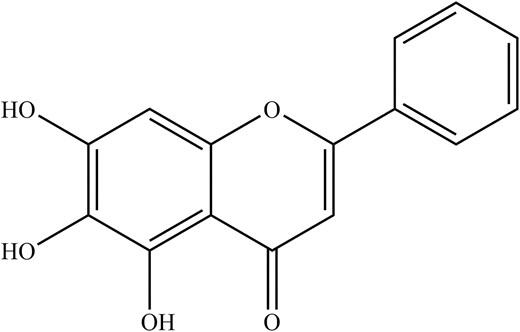
In summary, the baicalin from CS inhibits IAV replication by disrupting IAV-host interaction where NS1-p85β protein binds and potentially suppresses IAV NS1-induced cell cycle arrest in the G0/G1 phase. Apart from that, both baicalin and baicalein are capable of enhancing antiviral signalling against IAV-infected cells. A summary of phytochemical compounds in CS and their antiviral properties is shown in Table 1.
Angelica dahurica
Angelica dahurica, commonly known as Dahurian angelica (DA), is a greenish-white flowered plant with a purplish-green ribbed stem natively to eastern, northern, and southeastern Asia (Zhao et al. 2022). The medicinal use of DA has been well-established in traditional Chinese medicine, as it is used to treat cold fever, headache, toothache, and skin diseases, to name a few (Lee et al. 2020). However, misuse of the DA root could also lead to spasms (Batiha et al. 2022). DA contains a broad spectrum of bioactive compounds with volatile oils and coumarins such as isoimperatorin, imperatorin, oxypeucedanin, xanthotoxol, and byakangelicol, to name a few, being the main constituents (Yang and Li 2023). To date, more than 150 coumarins have been identified from DA, including coumarin glycosides and coumarin derivatives, mainly from the stems and roots (Zhao et al. 2022). Of these, furanocoumarins are the most abundant and notable compounds from DA with established medicinal indications such as anti-inflammatory, anticancer, antioxidant, and antibacterial activities, to name a few (Kang et al. 2019).
Coumarins, being the primary bioactive compound in DA extract, has been noted for its exceptional therapeutic indications. For instance, isoimperatorin and imperatorin have been reported to increase the activities of antioxidant enzymes, potentiate gamma-aminobutyric acid (GABA) receptors, and inhibit the degrative GABA transaminase (GABA-T) enzyme, making them a potential treatment for central nervous system related disease (Budzynska et al. 2013, Kozioł and Skalicka-Woźniak 2016). Besides that, oxypeucedanin and imperatorin are potent acetylcholinesterase (AChE) inhibitors, a positive therapeutic advancement for learning and memory processes (Kozioł and Skalicka-Woźniak 2016). Notably, byakangelicol and imperatorin work synergistically to inhibit β-secretase (BACE-1), an essential enzyme for the AChE mechanism (Vassar et al. 2009). Apart from that, both imperatorin and isoimperatorin showed significant antihypertensive properties, with the former having up to 4-fold vasodilation, possibly due to its profound antioxidant effect, which lowers the NADPH oxidase and subsequently the lipid peroxidase levels (Cao et al. 2013). The impact of imperatorin and isoimperatorin extends to antimicrobial properties. When combined with ampicillin and ceftazidime, isoimperatorin and imperatorin significantly increase the capacity to inhibit the formation of biofilm with reduced risk of bacterial resistance by Pseudomonas aeruginosa, an essential mechanism to colonize human tissue (Zou et al. 2021). Moving forward, xanthotoxol, together with byakangelicin, byakangelicol, oxypeucedanin, imperatorin, and isoimperatorin, were found to inhibit the production of NO, IL-1β, IL-6, and TNF-α in LPS-induced RAW 264.7 macrophage cells, an excellent anti-inflammatory agent (Shi et al. 2024).
Concerning the antiviral activity of DA, oxypeucedanin (Fig. 5) and isoimperatorin (Fig. 6) were among the extracts that have shown significant anti-IAV activities against the A/FM/1/47 (H1N1), A/WSN/33 (H1N1, S31N, amantadine resistant strain), A/Puerto Rico/8/34 (H1N1), and A/Chicken/Guangdong/1996 (H9N2) strains at the late stage of the viral lifecycle by targeting neuraminidase (NA) (Lee et al. 2020, Lai et al. 2021). Oxypeucedanin also significantly inhibited the synthesis of IAV, NA, and NP down to 20% protein expression after 2 h of viral infection (Lee et al. 2020). In addition, oxypeucedanin significantly inhibited caspase-3 and Bax activity exploited by IAV, exerting an excellent anti-apoptotic effect (Lee et al. 2020). Other studies have also revealed a staggering 81.4 and 84.6% virus yield reduction in A/Puerto Rico/8/34 (H1N1) IAV strains by isoimperatorin in a time of addition assay at 6–8 h and 8–10 h, respectively (Lai et al. 2021).
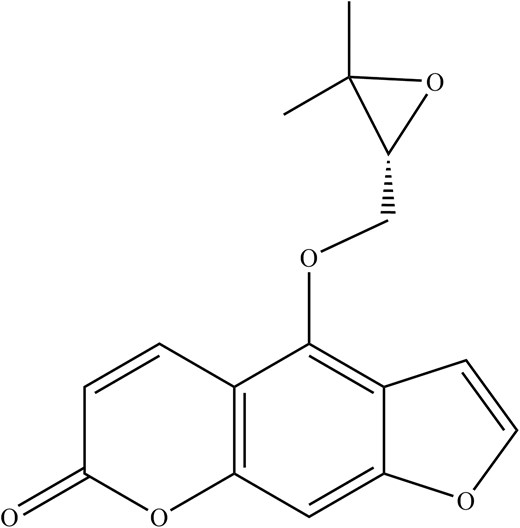
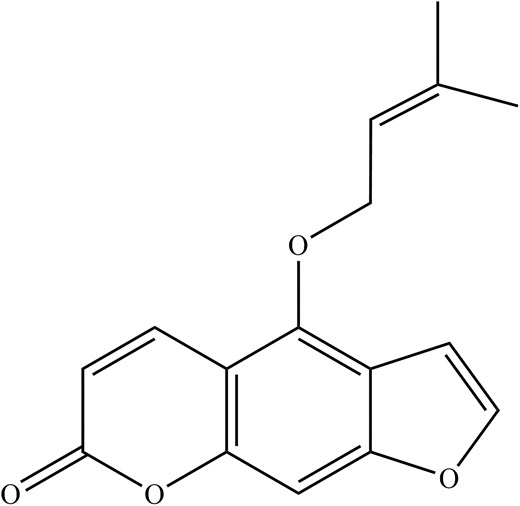
In summary, the oxypeucedanin from DA inhibits IAV replication by disrupting neuraminidase during the late stages of the IAV life cycle and significantly inhibits the synthesis of IAV, NA, and NP, in addition to inhibiting caspase-3 and Bax cell apoptosis activity exploited by the IAV. Apart from that, isoimperatorin from DA was also noted for its broad-spectrum anti-IAV and excellent neuraminidase inhibition in IAV-infected cells. A summary of major phytochemical compounds against IAV in DA and their antiviral properties is shown in Table 1.
Syrian rue (Pe. harmala L.)
Peganum harmala, commonly known as Syrian rue (SR), is a white-flowered carrying green veins, short creeping root plant with alternate spaced ovate leaves, natively to the Mediterranean region of Europe, Central Asia, and southern South America (Zhu et al. 2022). The traditional use of SR has been well-perceived in treating cancer, apoplexy, asthma, and jaundice, to name a few (Wang et al. 2016). In modern days, SR was found to be an effective agent in candidiasis, anti-inflammatory, anti-cholinesterase, antibacterial, antimicrobial, antitumour, angiogenesis, antiparasitic, antioxidant, and many more (Shahrajabian et al. 2021). The principle compounds present in SR are β-carboline indole alkaloids, including harmaline, harmine, harmalol, harmane, and harmol, primarily found in the seeds (Moloudizargari et al. 2013, Zhu et al. 2022). Another notable compound that was reported to carry significant health-promoting effects is quinazoline (e.g. vasicine and vasicinone), which was reported to be antitussive, antiplasmodial and vasorelaxant (Moloudizargari et al. 2013, Liu et al. 2015, Sharifi-Rad et al. 2021). Of these, harmine, harmaline and harmalol are the most abundant and notable compounds from SR with established medicinal indications such as anti-inflammatory, anticancer, antibacterial, and recently reported antiviral activities, to name a few (Zhu et al. 2022).
Going in-depth, harmine blocks LPS-induced inflammation in RAW264 cells via inhibition of NF-κB and NLR Family Pyrin domain-containing 3 (NLRP3) inflammasome pathway, significantly reduces oxidative stress and proinflammatory cytokines such as IL-6, IL-1β, and TNF-α, while increasing activities of superoxide dismutase and glutathione, making it a potent anti-inflammatory and antioxidative agent to protect neurons and reduce lung and kidney injury (Liu et al. 2018, Zhang et al. 2020). Apart from that, harmine was reported to ameliorate memory impairment by inhibiting AChE, akin to imperatorin found in A. dahurica. The activity of harmine in neurons extends to antidepressants where it strongly inhibits monoamine oxidase A that transforms dopamine to dihydroxyphenylacetic acid, in addition to increasing the brain-derived-neurotrophic factor protein, a serum protein found reduced in many depressed patients (Esteban et al. 2017, Liu et al. 2017). Besides that, harmine has exhibited a vast range of antitumour effects in gastric, lung, breast, liver, and colon cancer, to name a few, by binding and interfering with the synthesis and replication of DNA replication and its regulators such as Cdk1/cyclin B, Cdk2/cyclin A, and Cdk5/p25, in addition to inhibiting DNA topoisomerases (Song et al. 2004, Shu et al. 2019). Falling under the same β-carboline indole alkaloids, the therapeutic indications of harmaline and harmalol are largely the same with recent emerging evidence on various antiviral activities.
For instance, the whole seed extract of SR showed a maximum inhibition rate of 69.1% on HIV-1 reverse transcriptase activity (Ma et al. 2013). Besides that, harmine was also reported to be the most effective antiviral agent against the enterovirus 71 (EV71) virus, a major contributor to hand food mouth disease, in vitro with CC50 values between 400.0 and 500.0 μmol l−1 (Chen et al. 2018). Concerning the antiviral activity against the IAV, the β-carboline indole (Fig. 7) from the SR seed has also inhibited viral protein synthesis, exhibiting good anti-IAV activity in MDCK cells with CC50 values of 122.9 and 133.9 µg ml−1 for crude and total alkaloid extracts, respectively (Moradi et al. 2017). Hegazy et al. (2023) also demonstrated potent anti-IAV activities of β-carboline indole against avian H5N1 and human influenza A/H1N1 with IC50 values of 0.02, 3.42, and 0.023 µg ml−1, respectively, for the former and 0.035, 0.033, and 0.056 µg ml−1, respectively, for the latter, with a marginal improvement over the conventional amantadine at IC50 of 17.59 µg ml−1 (Hegazy et al. 2023). The study has suggested that harmalol and harmane interfere with viral replication; harmaline, on the other hand, exerts a direct virucidal effect against the virus (a cell-free mechanism) (Hegazy et al. 2023).
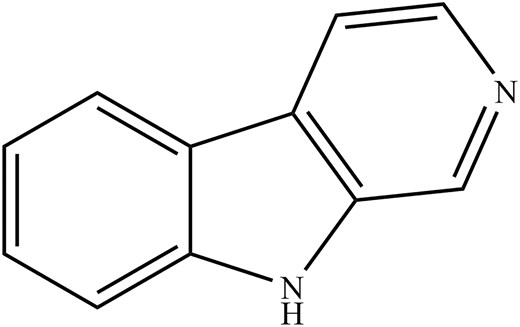
In summary, the β-carboline indole that entails harmalol, harmine, and harmaline from Pe. Harmala L. (SR) inhibits IAV replication by disrupting viral protein synthesis of IAV and displays virucidal effects against the IAV. However, research on the use of SR in the antiviral aspects is still at its infant stage, and more research is needed to unravel the antiviral mechanism of SR. A summary of the primary phytochemical compound against IAV in SR and its antiviral properties is shown in Table 1.
Black elder (Sa. nigra L.)
The genus Sambucus, otherwise known as elders, is a flowering plant from the family of Adoxaceae (Krawitz et al. 2011). Of the seven different species of Sambucus, the most common species is Sa. nigra, which is predominantly found in Europe, northern Africa as well as central and western Asia (Krawitz et al. 2011, Młynarczyk et al. 2018). This review focuses on Sa. nigra as this species was known to have the highest amount of total organic acids, flavanol concentration, and phenolic compounds than other Sambucus species, thus being the most promising candidate for anti-IAV therapy (Mikulic‐Petkovsek et al. 2015, 2016). Therapeutic uses of the black elder have been noted throughout the years, primarily due to the bioactive compounds found in the plant’s fruits and flowers (Mikulic-Petkovsek et al. 2016). The bark, leaves, seeds, and unripe fruits of the black elders, however, are not safe for consumption due to the presence of cyanogenic glycosides, which may be hydrolyzed in the body, causing the release of toxic cyanide (Młynarczyk et al. 2018). The main composition of elder flowers is hydroxycinnamic acids and flavanols (quercetin, kaempferol), while the elderberries are primarily rich in polyphenols such as anthocyanins in addition to flavanols (Mikulic‐Petkovsek et al. 2015, Sidor and Gramza-Michałowska 2015).
Anthocyanins are coloured, water-soluble pigments that give elderberries their reddish-blue colour and have been noted to have potent antioxidant effects (Młynarczyk et al. 2018, Torabian et al. 2019). The most abundant form of anthocyanin in elderberries is the cyanidin 3-sambubioside (Fig. 8), thus making it a compound of interest against IAV (H1N1) (Sidor and Gramza-Michałowska 2015). Cyanidin 3-sambucioside and other elderberry extracts were able to exert inhibitory effects by preventing entry and transmission of IAV to other cells in the post-infectious stage, as shown in haemagglutinin inhibition, plaque reduction and flow cytometry assay (Torabian et al. 2019). Interestingly, elderberry showed greater potency in the pre-treated, post-, and late stages of infection. However, cyanidin 3-sambucioside produces no immunomodulatory effects.
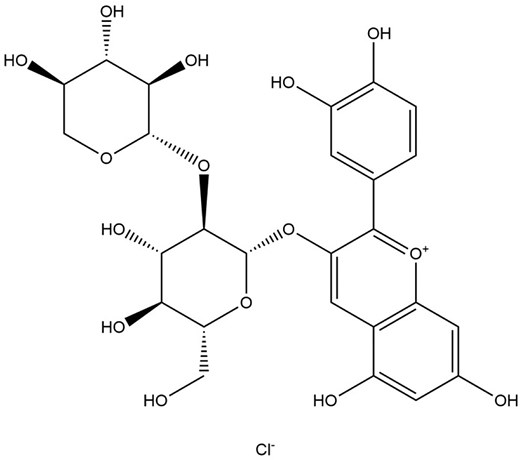
Apart from that, potent anti-IAV activities from other flavonoids in the fruit of Sa. nigra (elderberries) were demonstrated using direct analysis in real-time mass spectroscopy (Roschek et al. 2009). As such, the 5,7,3′,4′-tetra-O-methylquercetin and 5,7-dihydroxy-4-oxo-2-(3,4,5-trihydroxyphenyl)chroman-3-yl-3,4,5-trihydroxycyclohexanecarboxylate were found to bind IAV’s mannose-rich HA binding domain, and this blocks the host cell binding and recognition, consequently halting the virus from infecting the subsequent host cells (Roschek et al. 2009). Cyanidin 3-sambubioside showed a potent inhibitor of sialidase activity via the binding of 356–364 and 395–432 neuraminidase residues using mass spectrometry, suggesting an anti-IAV effect (Swaminathan et al. 2013, Torabian et al. 2019).
While the identification of compound isolates may be helpful in modern drug development, it was, however, identified that the whole concentrated elderberry juice (CJ-E) was able to produce stronger anti-IAV (H1N1) effects than oseltamivir in in vivo studies (Kinoshita et al. 2012). This was achieved by triggering the immune system of mice to produce more potent anti-IAV effects than oseltamivir, which reduces immune stimulation. In the same study, CJ-E was fractionated into low, medium, and high molecular weights and its subfractions to further identify the phytochemical compounds responsible for the immunomodulatory effects against the virus by administering these fractions in the mice and investigating the antibody production. Through this, the high molecular fraction, particularly the acidic-polysaccharide-rich fraction (Fr. II subfraction of the high molecular weight), exerted the most potent suppressive and stimulating effect on virus replication and antibody production, respectively (Kinoshita et al. 2012). Apart from that, water-derived elderberry extracts (EC15) and their polysaccharide fractions (CPS, BOUND, and UNBOUND) were reported to induce DCs maturation, enhanced inflammatory cytokine IL-6, TNF-α, and IFN-γ production which supports T cell-mediated immune response, and strengthen antibody production thereby suppressing virus replication (Stich et al. 2022). Given this, elderberry extract may serve as an effective alternative to oseltamivir for immunosuppressed patients, as it may prevent prolonged therapy.
A significant virus inhibitory effect from Rubini standardized elderberry extract was reported with a virus titre reduction of 30% and 25% on pre-treated MDCK cells upon 48 h of IAV (KAN-1, H5N1) and B (B/Mass) virus infection, respectively (Krawitz et al. 2011). In a double-blinded clinical trial on elderberry supplement (Rubini), 312 participants were assigned treatment and control capsules to take before, during, and after travel with varying doses (Tiralongo et al. 2016). This study showed that the treatment group experienced milder symptoms than the control group. In addition, only 29 of the 312 participants in the study had the illness; however, these results may not be statistically significant to conclude the inhibition potency of elderberry extract. Unfortunately, clinical trials investigating elderberry’s anti-IAV activities are scarce; therefore, further clinical research should be conducted to understand elderberry’s therapeutic potential against IAV.
In summary, black elder extracts contain cyanidin 3-sambubioside and flavonoids that prevent the IAV entry via the inhibition of sialidase activity and block the host cell binding and recognition motif, thereby suppressing viral replication (Roschek et al. 2009, Kinoshita et al. 2012, Swaminathan et al. 2013). The acidic-polysaccharide and Rubini standardized elderberry extract have suppressed viral replication and reduced symptoms in IAV infection (Tiralongo et al. 2016, Młynarczyk et al. 2018). A summary of phytochemical compounds in black elder and their antiviral properties is shown in Table 1.
Purple coneflower [E. purpurea (L.) Moench]
The Echinacea genus comes under the family of Asteraceae, in which the species E. purpurea is the most widely used in its native continent, North America (Hudson 2012). Due to its abundance and effectiveness in curing diseases, E. purpurea was traditionally used by the natives in treating respiratory infections, mainly by enhancing the strength of the immune system to combat the infection (Hudson 2012). The plant contains an abundance of phytochemical compounds such as caffeic acids, polysaccharides, glycoproteins, flavonoids, polyacetylenes, and alkylamides (Sharma et al. 2009). Given the therapeutic potential of E. purpurea, several experiments have been done to examine the anti-IAV properties of the plant. Various parts of E. purpurea may be therapeutically used, such as the roots, aerial parts of the plant, and the flowers. However, most studies focused on the effects of the whole plant extract, with some on the root (Hudson 2012). This is because the root extract of E. purpurea contains constituents different from the aerial parts, such as polyacetylene derivatives, polysaccharides, and glycoproteins (Ross 2016).
Two studies have shown the great potential of EF, a standardized E. purpurea extract comprising 95% aerial and 5% root parts of the plant against different strains of IAV at both the recommended dose as well as at lower doses. EF was found to be the most effective at the early stages of viral replication (Pleschka et al. 2009, Hudson 2012). According to Pleschka and colleagues, EF was deduced to obstruct the binding of the virus HA to the host cell receptor in a haemagglutination assay, inhibiting the viral entry into the host cells (Pleschka et al. 2009). Another study further confirmed this, which found that EF was less effective against intracellular IAV (H3N2) than extracellular viruses (Sharma et al. 2009). It was also shown that through the Raybiotech fluorescent antibody tray assay, EF could stimulate the release of proinflammatory cytokines, which may help control disease symptoms (Sharma et al. 2009). In addition, the EF used was free of polysaccharides and endotoxins, which further asserted the effect of EF on proinflammatory cytokines (Sharma et al. 2009).
Apart from that, a study investigated the difference in bioactive compounds and their anti-IAV effects in varying parts of the plant by preparing tinctures of E. purpurea from fresh and dried parts (Vimalanathan et al. 2013). It was noted that fresh herbs, the aerial parts of E. purpurea, gave the greatest anti-IAV effect at the lowest minimal inhibitory concentration and most significant logarithmic maximal inhibitory dilution compared to roots and dried forms of the plant. However, further analysis showed no correlation between both fresh and dried herbs. It was suggested that the combination of fresh herbs and root extract, containing anti-inflammatory alkylamides (Fig. 9), is needed to provide both antiviral and anti-inflammatory effects, and it is commonly found in both EF and standardized EFH extracts (Vimalanathan et al. 2013, Rauš et al. 2015). Besides that, alkylamides were found to stimulate anti-inflammatory IL-10 and suppress proinflammatory TNF-α production (Aucoin et al. 2021). Polysaccharides and circhoric acid found in the aerial parts of E. purpurea have also been postulated to work with alkylamides to enhance the production of natural killer cells and white blood cells and stimulate macrophages’ phagocytic activity during infection (Goel et al. 2002, Barnes et al. 2010, Aucoin et al. 2021, Ahmadi 2024).
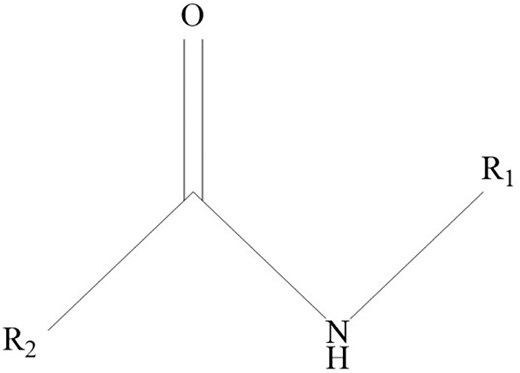
In an attempt to compare the effects of EFH with oseltamivir, a double-blind clinical trial was conducted on 473 patients presenting early-stage flu symptoms (Rauš et al. 2015). The study yielded significant findings as the recovery rates for both EFH and oseltamivir were similar at 1.5% and 4.1% on day 1 and 90.1% and 84.8% on day 10, respectively. It was also noted that patients given EFH experienced fewer adverse reactions to the treatment than oseltamivir. In an earlier study, resistant strains were developed in the oseltamivir-treated H5N1 virus but not in the EF-treated setting in a sequential passage analysis (Pleschka et al. 2009). Interestingly, the resistant strains developed from oseltamivir treatment were susceptible to EF treatment, therefore making EF a potential alternative treatment for the early stage of infection compared to the neuraminidase inhibitor (Pleschka et al. 2009). However, further studies are required to confirm its anti-IAV action (Pleschka et al. 2009).
In summary, purple coneflower contains alkylamides that suppress inflammation and inhibit IAV growth (Rauš et al. 2015). EF and EFH extracts from purple coneflower inhibit viral entry to host cells, stimulate proinflammatory cytokines and increase recovery rate (Sharma et al. 2009, Yoo et al. 2012, Vimalanathan et al. 2013, Ross 2016). A summary of phytochemical compounds in purple coneflower and their antiviral properties is shown in Table 1.
Red ginseng (Pa. ginseng C.A. Mey.)
Ginseng is a traditional herbal medicine under the family of Araliaceae, which is the root of the plant genus Panax, with Pa. ginseng being the most documented species (Yoo et al. 2012). Based on their processing conditions, the species may be further subdivided into white ginseng, produced through sun drying, and RG, produced through steaming, followed by drying to reduce moisture levels (Chan et al. 2011, Lim et al. 2015). Furthermore, additional findings have also suggested the steaming process in RG increases the bioactivity of the root (Lim et al. 2015). Henceforth, RG confers a more significant anti-IAV effect. The root has been predominantly used in Asian countries such as Korea and Japan for over 2000 years, as it harbours many pharmacological benefits, such as immunomodulation and antioxidative effects (Nguyen and Nguyen 2019). This may be due to the composition of bioactive compounds such as acidic polysaccharides, polyphenolic compounds, phytosterol, amino acids, peptides, and ginsenosides (saponins), the primary bioactive components in ginseng (Yoo et al. 2012, Nguyen and Nguyen 2019). Therefore, Pa. ginseng [Red ginseng (RG)] and its bioactive components in inhibiting IAV are discussed next.
Given the long beneficiary history of Pa. ginseng, it has undoubtedly garnered much interest from the public audience and researchers. For instance, two studies sought to determine if RG could confer protective effects through in vivo studies using mice and ferret models, respectively, with varying doses of RG extract (Yoo et al. 2012, Park et al. 2014). One of the studies showed that oral administration of RG extract to mice 2 weeks preceding IAV H1N1 and H3N2 infection showed a significantly higher survival rate of 80% compared to the control group at 20% (Yoo et al. 2012). The protective effects of RG extract do not stop here. Similar results were obtained by another study, where mice and ferrets fed RG extract for 60 days before IAV (H5N1) infection showed a survival rate of 45% and 40%, respectively, with an increase in survival rate as the time of RG extract feeding increased (Park et al. 2014). It was suggested that the effects of RG against IAV may be achieved through immune enhancement instead of a direct-acting treatment agent. Thus, the immunomodulatory effects of RG may be helpful against IAV. This effect was further characterised when one study found that IAV-infected A549 cells (human alveolar type II-like epithelial cells) treated with RG extract decreased the secretion of proinflammatory cytokines interleukin-6 (IL-6) and IL-8, as compared to untreated cells (Lee et al. 2014). Furthermore, the same study has also shown diminished reactive oxidative species (ROS) in infected A549 cells in the presence of RG extract via ROS-sensitive fluorescent probes (Lee et al. 2014). It was suggested that RG and its primary ginsenosides produce antioxidative effects by inhibiting the MAPK pathway, hence downregulating the production of ROS (Park et al. 2014).
Ginsenosides (Fig. 10) are believed to be the primary bioactive component in ginseng and have been reported to have attributed to many immunomodulatory effects of RG (Yin et al. 2013). Aside from that, polysaccharides (Fig. 11) in ginseng have also been noted to play a role in macrophage activation, further contributing to immunomodulation. The two compounds mentioned above have since then been the subject of research concerning the therapeutic effects of ginseng. The polysaccharide fraction produced the lowest nitric oxide level by dendritic cells (Tip-DC) among the five different treatment settings (Korean whole RG extract, saponin extract, polysaccharide extract, phosphate buffer saline-control, and Oseltamivir) upon challenged with IAV (H1N1), suggesting it may effectively prevent excessive immune stimulation, reducing disease symptoms (Yin et al. 2013). The administration of whole RG extract and saponin extract, along with inactivated flu vaccine, stimulated a significantly higher titer of antibody production in mice compared to the administration of the vaccine on its own (Xu et al. 2012). Therefore, this highlights the protection conferred by RG extract and its bioactive components, which can increase the efficacy of flu vaccines, thus increasing the survival rate of patients.
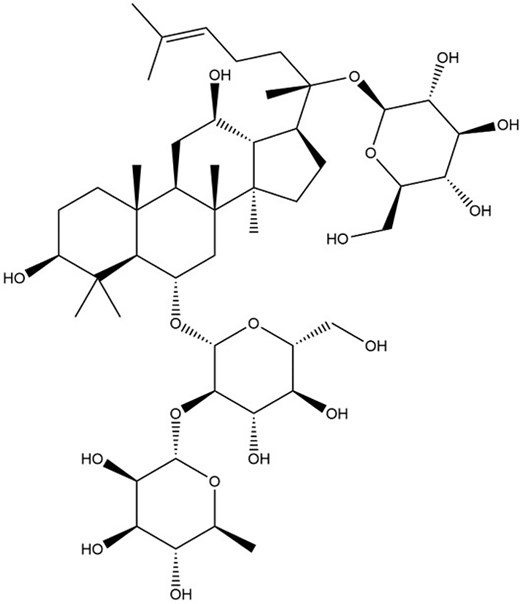
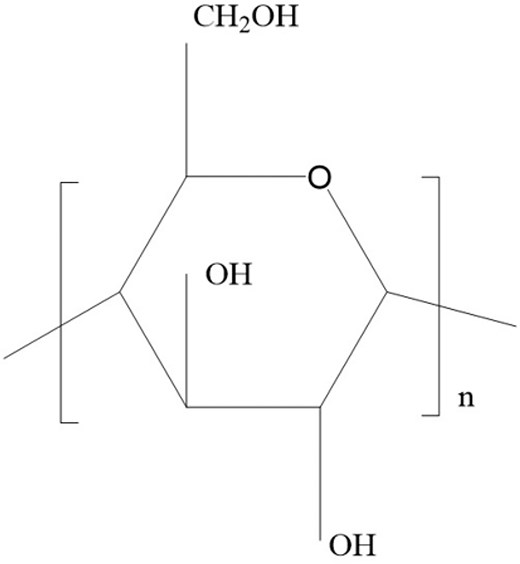
Besides that, fermented RG conferred higher protection against IAV strains (H1N1, H3N2, H5N1, and H7N2) than RG and was also able to protect the mice against secondary IAV infection (Wang et al. 2018). This may be attributed to the higher saponin composition in fermented RG (Wang et al. 2018). Therefore, this calls for more studies concerning this effect to unravel the maximum potential of RG against IAV.
In summary, RG exerts its anti-IAV effects mainly through immunomodulatory effects conferred by the ginsenosides (saponins) and polysaccharides (Xu et al. 2012). RG extract is also immuno-enhancing and provides considerable antioxidative effects (Chan et al. 2011, Yin et al. 2013, Lee et al. 2014). A summary of phytochemical compounds in RG and their antiviral properties is shown in Table 1.
Green tea (Ca. sinensis (L.) Kuntze)
Green tea, which comes from the leaves of the shrub Ca. sinensis, is one of the most widely consumed beverages, predominantly in the Asian community (Lee et al. 2012). The drink is made by brewing the leaves of the plant, and its consumption has been proven to confer beneficial human health properties such as antimicrobial, antiviral, antioxidative, and immunomodulatory effects (Lee et al. 2012). These beneficial properties have been linked to the major class of phytochemical compounds in green tea that belong to the flavonoid family, known as catechins (Müller and Downard 2015). There are four major catechins found in green tea, epigallocatechingallate (EGCG), epicatechingallate (ECG), catechin-5-gallate (C5G) and aspalathin (ASP), with EGCG accrediting for ∼50% of catechins found in green tea (Müller and Downard 2015, Kim et al. 2016). Notably, EGCG (Fig. 12) is the principal bioactive compound in green tea, with evidence pointing towards this compound as health-beneficial and antiviral (Sharma et al. 2017). Therefore, due to its known antiviral effects against a broad range of viruses, the principal compounds of green tea and their effects against IAV are discussed next.
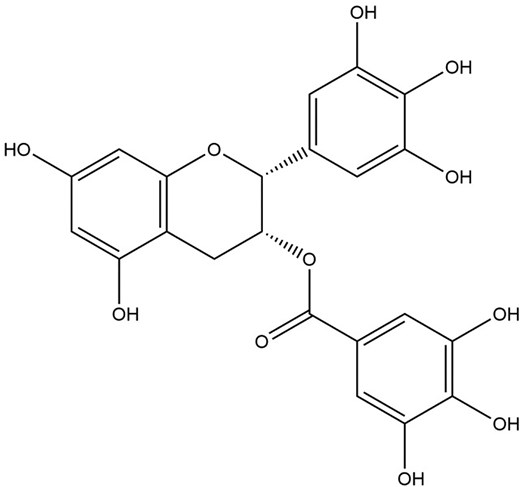
Due to its well-known and broad acceptance role in today’s beverages, it is no stranger that green tea has garnered much attention, be it for casual or health benefits. A study conducted on 3327 employees from four different Japanese companies found a marked reduction in employees developing IAV as the consumption of green tea increased (Nanri et al. 2021). However, as the study was conducted on a case-control basis, there may be a bias risk, where the study participants may provide inaccurate data regarding their lifestyle, diet, and symptoms due to the limitations of memory recollection.
Nevertheless, the antiviral potential of green tea and its polyphenols have grown with numerous studies. For instance, it was found that the green tea catechins EGC and EGCG were among the most effective polyphenols with the lowest IC50 (half inhibitory concentration) dosages against both IAV and IBV in MDCK cells, accessed among various polyphenols (Yang et al. 2014). EGCG, ECG, and C5G bind to neuraminidase residue beside the ‘430 cavity’, a secondary site for sialic acid binding, obstructing the binding of sialic acid to the primary active site of the enzyme, and therefore prevent the release of new virus progeny (Müller and Downard 2015). Furthermore, the binding of catechins to neuraminidase differs from the binding of the conventional zanamivir and oseltamivir drugs, allowing it to overcome drug resistance.
EGCG is capable of inhibiting the viral entry of IAV as well as the neuraminidase-mediated internalization of the virus into host cells, suggesting that EGCG causes physical damage to the lipid bilayer of the virus, causing the loss of the cell membrane penetration ability (Kim et al. 2016). The mechanism of action of EGCG was also further investigated, where it was found that EGCG modulates its action against IAV H1N1 in bronchial epithelial cells (BEAS-2B) by upregulating the (interferon) IFN-λ2 and inducing human β-defensin 3 (HBD3) via the p38 MAPK signalling pathway (Mou et al. 2020, Zhu et al. 2020). The activation of the MAPK pathway allows for the activation of cellular responses such as cytokine production, suggesting immunomodulatory and anti-IAV effects in green tea (Mou et al. 2020). The immunomodulatory effects also correspond to the findings of another study, where green tea catechin metabolites enhance the activity of CD4+ and natural killer cells, observed by higher production of adenosine triphosphate (ATP) and granzymes in the CD4+ and natural killer cells, respectively, indicating a greater cytotoxic effect (Kim et al. 2016). Together, these studies suggest the great potential of EGCG as an alternative mechanism of action against IAV compared to existing conventional drugs. Henceforth, further detailed studies should be carried out to elucidate the antiviral mechanism of action of green tea.
In summary, the catechins EGCG in green tea inhibited viral entry, neuraminidase-mediated internalization of IAV into host cells and played a part in immunomodulation (Kim et al. 2016, Sharma et al. 2017, Mou et al. 2020). A summary of phytochemical compounds in green tea and their antiviral properties is shown in Table 1.
Future prospects of plant extracts as anti-IAV therapy
Plant extracts generally exert low toxicity levels and have expanded their usage in a variety of applications, ranging from health supplements, prophylactic treatment in disease prevention, antimicrobial agents, and alleviating products to cosmetics and facials (Kesheh et al. 2022, Jeyaraj et al. 2023). Due to the rapid development of the virus, presenting an alternative drug and therapeutic approach is essential, especially amid the emerging antimicrobial resistance. Of no doubt, owing to the significant anti-IAV effect of plant extracts in IAV therapy, it could also be repurposed as an interim treatment to the rampant development of the COVID-19 pandemic.
To better access the potential of plant extract and its bioactive compounds as an antiviral, cell-based screening, multiplex assays, data mining, in silico bioinformatics, and cheminformatics databases can be explored (Low et al. 2020). Concerning the advancement of in-silico-based assays, the potential for phytochemicals in natural products can be further elaborated and enhanced with modifications such as nano-delivery, lipid carrier, and extended-release formulation to provide a better antiviral outcome upon administration. On account of this, nanocarriers are proposed as a future approach to drug delivery of bioactive compounds to target sites. The advantages of nanocarriers are well summarized by Stan et al. (2021). In brief, nanocarriers may overcome issues associated with ADMET (adsorption, distribution, metabolism, elimination, toxicity), drug stability, and bioactivity while facilitating controlled release at the key target site.
Despite the widely reported antiviral activity of various plant extracts against IAV, plant extracts are not widely used as antiviral drugs. This is most likely due to the lack of standardization in the composition of plant extracts. The composition of plant extracts varies depending on factors such as the plant’s genotype, growing conditions, extraction procedure, and ecosystem changes (Azmir et al. 2013, Jeyaraj et al. 2023). Various extraction procedures, be they conventional or non-conventional, have been used to ensure the suitability and satisfaction of phytochemical yield, and the extraction method has been proven to impact the extraction quality (Azmir et al. 2013, Dilukshi Vichakshana et al. 2022, Jeyaraj et al. 2023). Therefore, standardization of the composition of plant extracts should be carried out by providing the exact content of the active compound responsible for antiviral activity.
While the advantages of plant extracts are apparent with their beneficial biological activities and abundant nature, the long maturation periods and rigid growing conditions of specific plants add difficulty in harvesting for broad usage of plant extracts. For instance, elderberry plants mature in 3–4 years, and the yield, depending on growing condition, cultivar, and planting distance, may vary from 1.3 kg/bush for wild-harvested genotypes to 23.0 kg/bush for cultivars (Młynarczyk et al. 2018). In addition, elderberry is not suitable for mechanical harvesting as it does not separate easily from the pedicel, further limiting the plant extracts’ desired quantity and quality (Młynarczyk et al. 2018). However, research on the anti-IAV activity of plant extracts will continue to grow, and more compounds responsible for the activity will be uncovered.
Conclusion
MT, Baikal skullcap, SR, DA, Black elder, Purple coneflower, RG, and green tea have all proven to be potential candidates for anti-IAV therapy (Fig. 13). MT contains silymarin and silybin that inhibit viral RNA synthesis and replication of IAV. Baikal skullcap contains baicalin and baicalein, which mainly modulate host proteins by enhancing antiviral signalling and blocking the IAV-NS1-mediated signalling pathway, thereby inhibiting viral replication. SR contains β-carboline indole that exerts an antiviral effect, possibly through direct virucidal activity against IAV. Dahurian angelica contains isoimperatorin and oxypeucedanin that inhibit IAV-NA and NP, halting virus protein synthesis. Black elders extract contains cyanidin 3-sambubioside, flavanoids, and acidic-polysaccharide that play a role in preventing the viral entry of IAV through the action of inhibition of sialidase activity, blocking the host cell binding recognition motif and suppresses viral replication, respectively. Purple coneflower contains alkylamides that suppress inflammation and inhibit IAV growth. EF and EFH from purple coneflower improved recovery by inhibiting viral entry to host cell receptors and stimulating proinflammatory cytokines. RG exerts its anti-IAV effects mainly through immunomodulatory effects by its saponins and polysaccharides. Lastly, green tea inhibited viral entry and neuraminidase-mediated internalization of IAV into host cells, mainly through the actions of EGCG (catechin). Of these, the combination of purple coneflower and black elder was more effective than conventional antivirals such as oseltamivir for the early treatment against IAV through in vivo studies and clinical trials. Research on other plant extracts has also shown their potential as candidates for anti-IAV therapy. Therefore, there is a call for more research in the future to unravel the full anti-IAV potential of these natural products, paving the way for more treatment options, especially with the rapid drug-resistant development of IAV.
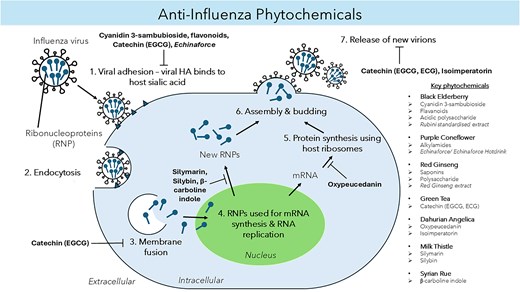
A graphical summary of key phytochemicals and plant extracts with anti-IAV properties of black elderberry, purple coneflower, RG, green tea, DA, MT, and SR. Antiviral activity occurs by targeting different stages of the viral life cycle, causing virus inhibition and/or modulating the host’s antiviral immune response. **Baikal skullcap is omitted here because its phytochemicals play a role in modulating the host’s immune system.
Author contributions
Hanushree Arumugam (Conceptualization, Writing – original draft), Ka Heng Wong (Conceptualization, Writing – original draft), Zheng Yao Low (Conceptualization, Writing – original draft), Sunil Lal (Conceptualization, Writing – original draft), and Wee Sim Choo (Writing – review & editing)
Conflict of interest
None declared.
Funding
This work was funded by the Fundamental Research Grant Scheme (Project No. FRGS/1/2020/SKK0/MUSM/02/1) from the Ministry of Higher Education, Malaysia.
Data availability
Data sharing is not applicable to this article as no datasets were generated or analysed during the current study.