-
PDF
- Split View
-
Views
-
Cite
Cite
Winnie Thabisa Ramaloko, Nontuthuko Excellent Maningi, John Osei Sekyere, Global prevalence, resistance rates, and underlying resistance mechanisms of clinical Mycoplasma and Ureaplasma species, Journal of Applied Microbiology, Volume 136, Issue 1, January 2025, lxae308, https://doi.org/10.1093/jambio/lxae308
- Share Icon Share
Abstract
Mycoplasmas are significant pathogens in human health, implicated in a range of clinical conditions from respiratory infections to urogenital disorders. Their resistance to commonly used antibiotics poses a substantial challenge to treatment and control. This study aims to provide a comprehensive overview of the global distribution of clinical mycoplasmas, elucidate their resistance to various antibiotics, and identify the genetic and molecular mechanisms underlying their resistance. A systematic review and meta-analysis were conducted, collating data from peer-reviewed publications between 2012 and 2024. The UK (100%) and Germany (98%) reported high numbers of respiratory mycoplasmas, with 7% and 2% being resistant to macrolides. For urogenital mycoplasmas, Iceland (99%) and Estonia (94%) reported a high prevalence of Mycoplasma species, whereas the UK (85%), France (82%), and the USA (82%) reported a high prevalence of Ureaplasma species. High resistance rates in Mycoplasma and Ureaplasma have been reported in Greenland (100%) and the UK (86%), respectively. The rising resistance rates in these species underscore an urgent need for updated treatment guidelines and the development of novel therapeutic options. Our findings highlight the importance of tailored antibiotic stewardship and the potential of genomic insights in guiding effective treatment strategies.
Antibiotic resistance in urogenital and respiratory mycoplasmas have been reported globally. Various mycoplasmas exhibit varying prevalence and resistance rates across different regions, yet they possess comparable resistance mechanisms.
Introduction
Mycoplasma and Ureaplasma species are collectively referred to as mycoplasmas. These species are unique among bacteria for their lack of a cell wall (Iliopoulou et al. 2017, Sethi et al. 2017, Gautier-Bouchardon 2018). They are usually found on the epithelial surfaces of the respiratory and urogenital tissues in humans (Gautier-Bouchardon 2018). Their existence may be harmless until an individual is in an immunosuppressed state where they can cause various infections (Chu et al. 2020). The most common clinical mycoplasmas include Mycoplasma genitalium (MG), M. hominis (MH), M. pneumoniae (MP), and Ureaplasma spp., namely Ureaplasma parvum (UP) and U. urealyticum (UU). Genital mycoplasmas are transmitted through vaginal, anal, and oral sexual contact. Transmission rates increase with unprotected sex and individuals with multiple partners. Undetected transmission is common since genital mycoplasmas are associated with asymptomatic infections. Urogenital mycoplasmas may also be transmitted vertically from mother to child during natural birth colonizing the respiratory and genital tracts of the newborn or these pathogens may be transmitted from mother to fetus during pregnancy (Chu et al. 2020). MP is transmitted through droplets during coughing, sneezing, and talking. Transmission rates are higher in crowded places such as schools and military barracks (Parrott et al. 2016).
Mycoplasmas use virulence factors such as adhesins to attach to the epithelial cells lining of the urogenital tract targeting the mucosal cells (Ammar et al. 2014). Mycoplasmas also can invade the immune detection system and this allows them to escape the immune system, leading to chronic infections (Ammar et al. 2014). Urogenital mycoplasmas (MG, MH, UU, and UP) cause sexually transmitted diseases such as acute non-gonococcal urethritis in males (Wiesenfeld and Manhart 2017) and pelvic inflammation disease in females (Fernández-Huerta et al. 2020, Hart et al. 2020). If left untreated, infections may result in severe effects such as ectopic pregnancy, cervicitis, and, possibly, infertility in women (Taylor-Robinson 2017, Wiesenfeld and Manhart 2017, Vesty et al. 2020). Infections may also cause adverse pregnancy outcomes such as spontaneous abortion, preterm delivery, stillbirth, and miscarriage (Redelinghuys et al. 2014, Iliopoulou et al. 2017). MG is the most common urogenital mycoplasma and has been reported in many countries, including South Africa (Redelinghuys et al. 2015), the USA (Taylor-Robinson 2017), and the Netherlands (Braam et al. 2017). MP is the most common respiratory mycoplasma, causing respiratory infections, particularly in children (Chaudhry et al. 2016, Waites et al. 2017, Big Mohammadi et al. 2020). It has a complex adherence mechanism that includes the P1 protein, encoded by the p1 gene (Chaudhry et al. 2016, Waites et al. 2017). This protein establishes the host cellular interactions and it is used to differentiate MP strains into two primary types: type 1 and type 2 (Diaz et al. 2015, Parrott et al. 2016).
Mycoplasma and Ureaplasma infections have been successfully treated with antibiotics such as macrolides, fluoroquinolones, and tetracyclines (Sethi et al. 2017, Hart et al. 2020). Macrolides (erythromycin, azithromycin, and clarithromycin) (Akashi et al. 2018, Big Mohammadi et al. 2020, Dumke and Spornraft-Ragaller 2021) are recommended as the first line of treatment, and fluoroquinolones (moxifloxacin, levofloxacin, and ciprofloxacin) (Sharratt et al. 2021) as the second-line antibiotics (Dumke and Spornraft-Ragaller 2021). Tetracyclines are usually recommended in cases where macrolides and fluoroquinolones are ineffective. The most common second-generation tetracyclines are minocycline and doxycycline, which are considered safer as they bind to calcium, thus reducing the chances of tooth discoloration during treatment (Chang et al. 2021).
Prolonged use and over-prescription of antibiotics have hugely contributed to the emergence of resistance against antibiotics that are used for mycoplasma infections (Wiesenfeld and Manhart 2017, Hart et al. 2020). Because of their dearth of a cell wall, mycoplasmas are naturally resistant to all β-lactams and other antibiotics that mainly target the cell wall; therefore, treatment options are limited (Murray et al. 2017, Chernov et al. 2018, Hart et al. 2020). Their resistance to antibiotics leads to persistent and or/chronic infections (Gautier-Bouchardon 2018). Acquired resistance has been increasing in many countries. Countries such as Japan reported an increase in macrolide and fluoroquinolones in MG (Deguchi et al. 2018). Macrolide resistance reached above 70% in 2017, while fluoroquinolone resistance reached roughly 10% after the year 2015 (Deguchi et al. 2018). This is especially true in high-risk populations, particularly in developing countries (Schneider et al. 2015, Meygret et al. 2018).
The most common resistance mechanism in mycoplasmas is the alteration of the drug target sites through mutations and methylation of the DNA sequence (Morozumi et al. 2017, Waites et al. 2017). Point mutation is a more prevalent mechanism in mycoplasmas, while resistance through efflux pumps and biofilm formation are the least common and less explored mechanisms in these pathogens. A point mutation in the V domain of 23S rRNA is the underlying mechanism for macrolide resistance (Caballero et al. 2014, Chang et al. 2021), while fluoroquinolone resistance occurs through the modification of DNA gyrase (gyrA/B) and topoisomerase (parC/E) target genes (Wang et al. 2019, Sharratt et al. 2021). Mutations in the ribosomal proteins such as L4 and L22 in the 50S ribosomal subunit also lead to macrolide resistance in mycoplasmas (Zhang et al. 2021). However, these mutations are extremely rare (Guo et al. 2014). Tetracycline resistance occurs through the acquisition of the tet(M) gene (Wang et al. 2019) or it may occur through mutations in the 16S rRNA target gene, particularly in MG (Le Roy et al. 2021). The tet(M) gene is found on the Tn916 transposon, which encodes the Tet(M) protein and protects the ribosome from tetracycline (Chernova et al. 2016).
This study seeks to provide an in-depth analysis of the global prevalence of Mycoplasma and Ureaplasma species, their resistance rates to key antibiotics, and the underlying genetic and molecular mechanisms contributing to this resistance. By generating and analyzing data from diverse geographical regions, the study aims to provide insights that could inform the development of targeted therapeutic strategies and guide public health policies. Understanding the global landscape of these pathogens is essential not only for effective clinical treatment but also for the development of sensitive, specific, and rapid diagnostic assays and prevention strategies. This study contributes to that effort by mapping the current state of Mycoplasma and Ureaplasma resistance, exploring how genetic changes contribute to their survival strategies, and highlighting areas for future research and intervention.
Materials and methods
Literature search
PubMed and Google Scholar databases were searched using the following terms: Mycoplasma AND resistan*/virulen*/pathogen*, Ureaplasma AND resistan*/virulen*/pathogen*, Mycoplasma AND resistan*/virulen*, Ureaplsama AND resistan*/virulen*, Mycoplasma AND diagnos*, and Ureaplasma AND diagnos*.
Inclusion and exclusion criteria
Articles were included based on the following criteria: (i) published after 2012, (ii) published in English, (iii) cover clinical mycoplasma, and (iv) cover resistance and resistance mechanisms in clinical mycoplasmas. A total of 324 articles were included for analyses. Articles published before 2012, non-English articles, retracted articles, and those with topics that do not cover clinical mycoplasma and their resistance were excluded.
Data extraction
Data extracted from the 324 articles included the following: geographical distribution, sample source, species, gender, age group, type of infection and symptoms, diagnostic methods, antibiotics administered and their resistance rate, resistance mechanisms, and the sample size. These data were tabulated in Microsoft Excel (Table S1) and were used for downstream analyses.
Data analyses
Data such as total infections, antibiotic resistance infections, resistance determinants, and mechanisms in genital and respiratory mycoplasmas were analyzed using Microsoft Excel 365. These analyses were done using data from different geographical locations. The resistance rates were calculated using this formula: “resistance rate = # of resistant isolates/# of positive (tested) isolates × 100”. Infection and resistance rates against macrolides, fluoroquinolones, and tetracyclines were analyzed for all urogenital mycoplasmas. For respiratory mycoplasmas (MPs), the macrolide resistance rate and the P1 genotypes were analyzed. The p1 gene is used to differentiate the MP variants (Parrott et al. 2016). The Shapiro–Walk test for normality was done to assess the distribution of data (Mishra et al. 2019). If the P-value was >.05, the null hypothesis was accepted, and the data were classified as being normally distributed.
Analysis of literature search
A literature search resulted in 3884 papers. Duplicates, retracted papers, and papers not addressing antimicrobial resistance in mycoplasmas were removed, resulting in 388 papers. Papers addressing antimicrobial resistance in non-clinical mycoplasmas and papers not reported in English were removed (n = 64) (Fig. 1). Hence, 324 papers were selected for the qualitative analysis (Table S2). The publication time distribution for this study is shown in sheet 2 of Table S2. This review summarizes the global prevalence, and antimicrobial resistance in Mycoplasma and Ureaplasma spp. and their underlying mechanisms, infections, and resistance (Table S2). Available evidence on prevalence, antibiotic resistance, and resistance mechanisms in mycoplasmas was analyzed from articles published between 2012 and 2024. According to the evidence we gathered, urogenital mycoplasmas are generally not as commonly reported as respiratory mycoplasmas. Our meta-analysis indicates a higher burden of infection in East Asia, with reports of outbreaks frequently documented in countries such as China and Japan. The data emphasize the need for region-specific treatment guidelines and alternative therapeutic options in high-resistance areas.
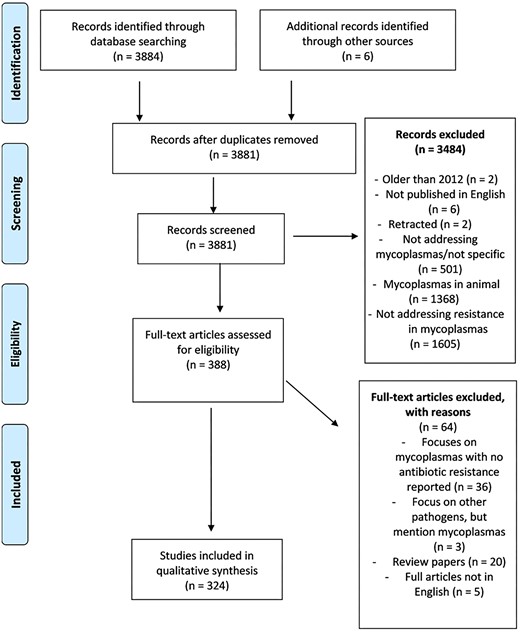
A PRISMA (Preferred Reporting Items for Systematic Reviews and Meta-Analyses, Page et al. 2021) chart that shows the criteria used for sorting data and a summary of articles that are eligible for inclusion in the analyses of infection rate, resistance rate, and resistance mechanisms in Mycoplasma and Ureaplasma spp.
Results
Prevalence and antibiotic resistance in urogenital mycoplasmas
Overall, urogenital mycoplasmas (MH, MG, UP, and UU) infections have been reported in five continents, represented by 34 countries. Mycoplasma spp., specifically MH and MG have been reported in 33 countries. In these 33 countries, MH was reported in 10 countries, while MG was predominant and was reported in 18 countries (Table S3). For Ureaplasma infections, UU was predominant in 10 countries while UP and Ureaplasma spp. (not specified) were both reported in seven countries. Eleven countries reported both Mycoplasma and Ureaplasma infections. Most of these countries were in Europe and Asia, while others were in Africa, and North and South America. Such countries included the USA (M: 17%, U: 82%), Australia (M: 37, U: 45), China (M: 0.08%, U: 4%), France (M: 1%, U: 82%), India (M: 35%, U: 17%), Mexico (M: 9%, U: 72%), Germany (M: 20%, U: 22%), Japan (M: 36%, U: 9%), Tunisia (M: 6%, U: 10%), Italy (M: 9%, U: 62), and the UK (M: 85%, U: 98%), (Fig. 2a). Fifteen countries reported Mycoplasma spp. only, and Ureaplasma spp. were found in one country, Greece (Fig. 2b). Prevalence and infection rates depend on population and sample sizes. Countries with low population sizes tend to report high levels of resistance and the resistance determinants dominate in such populations.
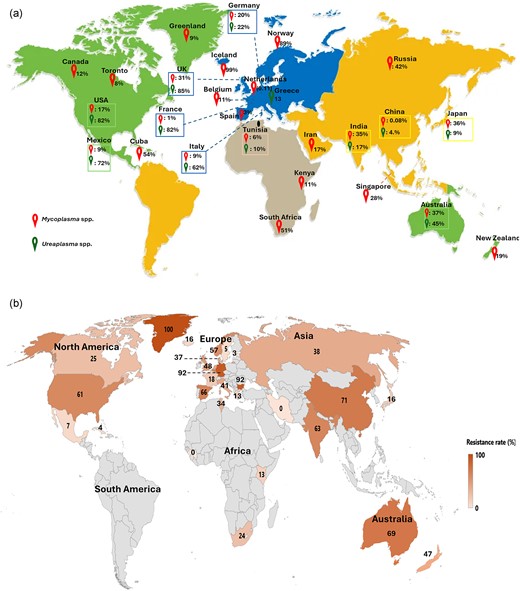
(a) The global infection rate for Mycoplasma and Ureaplasma species. These figures are based on the reported instances from 324 publications, which show the prevalence in various places. (b) Geographic distribution of total antibiotic resistance in Mycoplasma and Ureaplasma spp. from the lowest (0%) to the highest (100%). The rate was estimated using resistant isolates of positive bacteria. .
The resistance rates among Mycoplasma and Ureaplasma spp. in different countries were calculated based on the reported infections from 324 analyzed papers, irrespective of the period (Fig. 2b). The highest resistance was reported in Greenland (100%, n = 26), followed by India (95%, n = 35), Bulgaria (92%, n = 23), and Australia (72%, n = 2274). Greenland and Bulgaria reported antimicrobial resistance in Mycoplasma spp. only, while Australia, India, Japan, Mexico, the UK, and the USA reported resistance in both spp. Greenland's population is small and geographically isolated, limiting the diversity and number of people exposed to mycoplasmas. In such small populations, infections might circulate within tight-knit communities, leading to a limited pool of pathogens and potentially higher resistance rates if a particular resistant strain becomes dominant. This is sometimes seen in isolated or remote areas where certain resistant strains spread more easily within the local population due to fewer outside interactions. Countries such as Cuba (4%), Estonia (3%), Mexico (7%), and Sweden (5%) reported lower resistance rates. Iran and Guinea both reported 0% resistance in their studies. Instructively, countries with low resistance rates reported mycoplasma infections only. The lowest resistance rates were reported in Estonia (16%, n = 18) and Cuba (4%, n = 3). Of the countries that reported high resistance rates, China, the USA, and Mexico reported both Mycoplasma and Ureaplasma infections.
Our analysis reveals a worrying increase in the prevalence of resistant Mycoplasma and Ureaplasma strains worldwide, with significant regional variations. The overall average resistance rate of macrolides in 25 204 Mycoplasma spp. was 59%, while 51 272 Ureaplasma spp. presented 19% resistance (Fig. 3a and b). For mycoplasma infections (Fig. 3a), most spp. were reported to be resistant to macrolides (n = 8659, 59%), followed by fluoroquinolones (n = 5434, 39%), tetracyclines (n = 314, 2%), and multi-drug resistance (MDR) (n = 309, 2%). Out of these 309 MDR strains, MG represented 99.7% (n = 308), while MH (n = 1) represented only 0.3%. For Ureaplasma spp., resistance to fluoroquinolones was the most reported (n = 3448, 66%) compared with macrolides (n = 980, 19%) and tetracycline resistance (n = 406, 8%). Interestingly, Ureaplasma spp. showed a higher MDR prevalence (n = 406, 10%) than Mycoplasma spp., which had only 2% of MDR isolates (Fig. 3b).
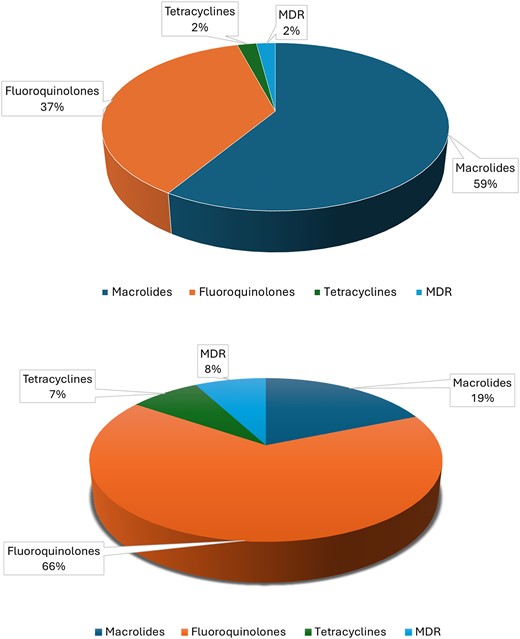
Represents the overall resistance in urogenital mycoplasma against different antibiotics such as macrolides, tetracyclines, fluoroquinolones, and MDR. Image (a) represents the resistance in Mycoplasmas spp. and image (b) represents the resistance in Ureaplasma spp.
Antimicrobial resistance mechanisms in urogenital mycoplasmas
Resistance mechanisms through mutations and gene acquisition are shown in Fig. 4a. Antibiotic resistance through gene mutations is the most common mechanism found in mycoplasmas. The A2058&9/G/C/T point mutations for macrolide resistance were the most common resistance mechanisms. These were found in 21 of 29 countries, while A2029/G/C/T (n = 1) was the least common resistance mechanism reported and was found in one country (USA) (Table S4). Overall, the USA and China reported the most diverse resistance mechanisms for all three classes of antibiotics (macrolides, fluoroquinolones, and tetracyclines). Both these countries reported six of seven resistance determinants. In contrast, countries such as Tunisia and South Africa reported only one kind of resistance mechanism for fluoroquinolone resistance. For fluoroquinolones, the most prevalent mutations were detected in the parC/E genes, and were detected in all species. These mutations were detected in 554 (29.4%) Mycoplasma and 374 (8.5%) Ureaplasma species. Antibiotic resistance in Mycoplasma and Ureaplasma spp. differed for countries that reported both infections. China reported >40% of mutations in the quinolone resistance-determining regions (QRDRs), 28% (n = 90) for gyrA and 16% (n = 577) for parC/E (Table S4). However, the USA reported lesser resistance determinants for macrolides (9%) and fluoroquinolones (11%). It is worth noting that this country reported 15% of tetracycline resistance in Mycoplasma species.
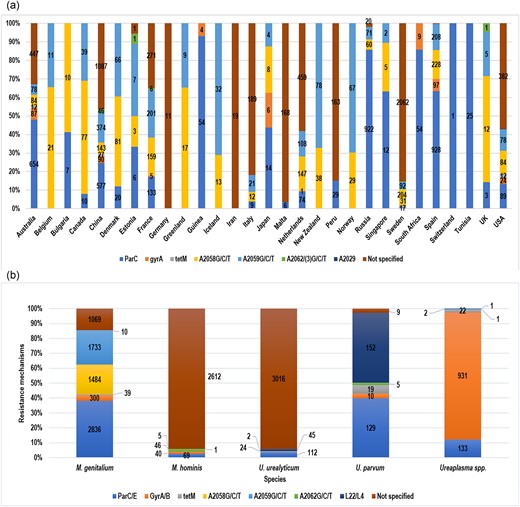
(a) Image showing the resistance rates based on different resistance mechanisms in various locations. (b) Image showing the distribution of resistance determinants that were reported in Mycoplasma spp. (MG and MH) and Ureaplasma spp.
Different resistance determinants in different spp. are shown in Fig. 4b. This analysis revealed that all species presented a diverse resistance profile for macrolide, fluoroquinolone, and tetracycline resistance. In addition, resistance mechanisms were not specified, of which most were reported for macrolide resistance. MG isolates stand out with a rich resistome, presenting high numbers of resistance determinants for all three classes of antibiotics. This species presented a high number of mutations, including parC/E (n = 2836), gyrA/B (n = 300), A2059/G/C/T (n = 1733), A2058/G/C/T (n = 1484), and tet (n = 39). Nonetheless, the L22/L4 mutations were not reported in these isolates. MH species, on the other hand, show a rather poor resistance profile, where the highest number of mutations reported were 69 (A2062G/C/T). MH reported similar mutations to MG, except for A2058&59G/T/C. Furthermore, the L22/L4 mutations were detected in MH. The L22/L4 mutations were commonly reported in Ureplasma spp. (n = 197, 98.2%), where UP and UU reported 45 (22.5%) and 152 (76%) mutations, respectively. Notably, none of the A2058/G/C/T mutations for macrolide resistance were detected in Ureaplasma spp.
Other reported mutations conferring macrolide resistance in Mycoplasma and Ureaplasma spp. included mutations at position 2062 (Escherichia coli numbering) and mutations at ribosomal proteins L22 and L4 (Table S5). This was the last mutation, which was the most predominant, and detected only in Ureaplasma spp., found in the genes that encode the L22/L4 ribosomal proteins (n = 199, 5.80%) (Fig. 4b). These genes are responsible for macrolide resistance mostly in Ureaplasma spp. A total of 87 species harbored the tet(M) gene, which is associated with resistance against tetracycline mycoplasmas. Lastly, the least common mutations, C234T/P625 (silent mutation) and A2029 (unknown function), were reported (Table S5). Nonetheless, since these mutations are silent, they are not associated with antibiotic resistance in mycoplasmas.
Prevalence and antimicrobial resistance in respiratory mycoplasmas
MP infections, as well as macrolide-resistant infections, are shown in Fig. 5. Respiratory mycoplasmas were reported in >20 countries (Table S6). MP was more prevalent in Asian countries. Korea was the leading country with 24 252 MP infections, followed by China, Japan, and Taiwan with 7627, 5850, and 2621, respectively (Fig. 5). Other countries with high MP infections were France (n = 29 806) and the USA (n = 29 891). Countries such as Cuba (n = 27), Italy (n = 23), and Iran (n = 6) reported a lower number of infections.
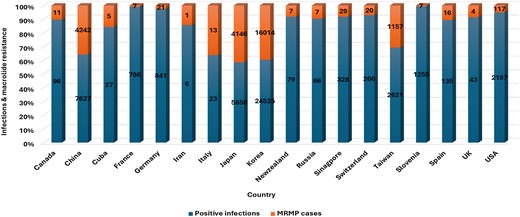
The total number of reported cases, positive MP cases, and macrolide resistance (MRMP) rates in different locations. Reported cases refer to samples collected for each study, while positive cases are those samples that tested positive for MP.
Korea presented the highest number of studied samples (n = 383 896) and reported 6% (n = 24 525) of MP-positive samples, of which 65% (n = 16 014) were resistant to macrolides (P = .16). Japan also reported a high number of studied samples (n = 810 151): 1% (n = 5850) were positive cases of which 71% (n = 4146) were resistant to macrolides (P = .13) (Table S6). In China, 7627 (20%) positive cases were reported from 38 213 studied cases: Of these, 4242 (56%) cases were resistant to macrolides (P = .31). The USA showed the lowest MRMP cases (n = 117, 5%) from 2187 positive cases (P = .23). This resulted from a total number of 29 891 studied samples (Fig. 5). Slovenia reported 1% MP-positive cases from 9431 studied samples, and France also reported 1% MP infections from 29 806 studied samples. Three countries, Cuba (n = 27), Iran (n = 6), and Italy (n = 23), reported <30 MP infections (Table S6).
Antibiotic resistance in MP differs yearly in different countries. A high annual macrolide resistance in MP infections was reported in France (99.8% from 2000 to 2011), followed by 99.7% in Singapore from 2012 to 2014 and 99.2% in Spain from 2013 to 2017 (Table S7). Table S8 shows the different resistance mechanisms associated with macrolide resistance in MP isolates. Mutations reported for macrolide resistance in MP species are shown in Table S7. The most common 23S rRNA point mutations that confer macrolide resistance in MP are A2063G/T/C and A2064G/T/C. These mutations were reported in all countries with MP infections. Korea reported the highest rate of 67.8% and 47.3% of A2063G/T/C and A2064G/T/C, respectively. Instructively, the C2617A/T/G mutations, which were the least reported, were reported only in Japan (n = 4) and Germany (n = 1). The remaining macrolide mutations such as A2589/90T, A2431G/C/T, G22491A, G2601C, and C2617A/T/C were reported only once or twice in different countries. Table S8 shows different resistance mechanisms associated with antibiotic resistance in MP species. Resistance through biofilm formation and efflux pumps were reported only in the USA (n = 1) and China (n = 2) (Table S8). These are the least studied resistance mechanisms in MP isolates.
Association of the P1 lineages with infection and macrolide resistance in MP
MP strains can be differentiated based on the typing and sequencing of the p1 adhesin molecule gene, which encodes the main attachment protein (Parrott et al. 2016). The summary of the p1 gene analysis is shown in Table S9. The P1 type 1 MP strains are the most prevalent type. Figure 6 shows the global variations of the MP isolate based on the p1 adhesin gene. Overall, the P1 type 1 MP strains are more prevalent, accounting for 68% of reported strains; this is followed by P1 type 2, representing 23% of reported strains. The most common variant of P1 type 2 is P1 type 2c, which was reported only in Japan (Fig. 6). Japan and Germany are the only countries that reported all five MP P1 types (Type 1, 2, 2a, 2b, 2c, and 2j/2 g). P1 type 2a, 2b, 2j, and 2g were the lowest reported types in our analyses, each accounting for only 1% of the reported typed MP species (Fig. 6).
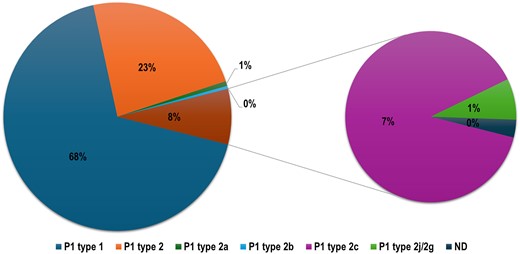
Diagram showing the prevalence of the dominating and least common MP lineages based on the p1 gene such as P1 type, P1 type 2, P1 type 2a, P1 type 2b, P1 type 2c, and non-typable/not detected.
Discussion
This review summarizes the global prevalence of Mycoplasma and Ureaplasma spp., their antimicrobial resistance, and their underlying resistance mechanisms. According to the evidence gathered, urogenital mycoplasmas are generally not as commonly reported as respiratory mycoplasmas. Our meta-analysis indicates a higher burden of infection in East Asia, with more outbreaks frequently documented in countries such as China and Japan. The data emphasize the need for region-specific treatment guidelines and alternative therapeutic options in high-resistance areas.
The effectiveness of macrolides against mycoplasmas has been declining for over a decade. The efficacy of 1 g macrolide (azithromycin) against MG infections was reported to decrease from 85% before 2009 to 67% after 2009, respectively (Lau et al. 2015). The macrolide efficacy was sitting at 60% in 2015 (Lau et al. 2015). Furthermore, the overall efficacy of fluoroquinolones has also decreased. This was supported by our study, which reported an average fluoroquinolone resistance rate of 37% and 66% in Mycoplasma and Ureaplasma spp., respectively (Fig. 3a and b). It was expected that resistance to macrolides would be higher than fluoroquinolones due to macrolides being the first line of treatment for mycoplasma infections. In this study, the opposite was true. This may be because most studies had unspecified macrolide resistance mechanisms. This may also be because susceptibility tests for macrolides are not routinely done in other mycoplasmas such as MH as they are naturally resistant to these antibiotics (Wang et al. 2019, Boujemaa et al. 2020).
An estimated average of 12.5% for macrolide resistance determinants was reported in Ureaplasma spp. (Table S4). These species usually express higher minimum inhibitory concentrations (MICs) than Mycoplasma spp., particularly M. hominis. Specifically, Waites et al. (2020) reported MIC50 values of 0.5 and 0.25 µg/ml for Ureaplasma spp. and MH, respectively (Waites et al. 2020). The MIC of Ureaplasma spp. was 2-fold higher than that of MH. Furthermore, the MIC for fluoroquinolone, namely 0.5 mg/ml (Ureaplasma spp.) and 0.063 (MH) mg/ml, was 8-fold higher; MG showed MIC of <0.5 mg/ml. Therefore, it is not surprising that MH showed higher resistance rates than UP. MH is naturally resistant to 14-membered and 15-membered lactone-ring macrolides such as azithromycin and erythromycin through the A2057A mutation (Wang et al. 2019). Furthermore, Ureaplasma spp. showed high MIC values even in the absence of underlying resistance genes (Waites et al. 2020).
The most common mutations for macrolide resistance in Mycoplasma spp. are A2058G and A2059G (Braam et al. 2017). Countries such as Norway (Gossé et al. 2016), Netherlands (Braam et al. 2020), and Iceland (Hilmarsdóttir et al. 2020) reported >50% of the A2058G and A2059G point mutations. These are the most prevalent determinants for macrolide resistance in Mycoplasma spp., particularly MG. Likewise, our study calculated an average of 23% and 20% of A2059G/C/T and A2058G/C/T point mutations in MG, respectively (Table S4). Moreover, an increase in macrolide resistance in MG from 4.8% between 2005 and 2009 to 42.2% between 2010 and 2017 has been reported (Hamasuna et al. 2018b). Although point mutations are the most common resistance mechanisms in mycoplasmas, not all mutations are associated with antibiotic resistance, even when they occur in the resistance-determining regions (Tagg et al. 2013). There are missense and nonsense/sense point mutations that occur in the resistance-determining regions. Although C234T has been reported in other countries (Table S5), its significance is unknown (Tagg et al. 2013). Mutations at the L4 and L22 ribosomal proteins were only reported in China (n = 170, 85%). Although these mutations are associated with macrolide resistance, they were reported to have less impact on treatment failure (Read et al. 2018).
Nevertheless, mutations in the QRDRs were mostly reported. This was expected because Mycoplasma and Ureaplasma species use mutation in the QRDRs as the primary resistance mechanism for fluoroquinolones. The gyrA and parC amino acid changes increased from 0% to 4.7% between 2005 and 2017, while parC single nucleotide polymorphisms increased from 22.6% to 53% (Hamasuna et al. 2018b). Three parC and gyrA sense mutations, which were G to A/C point mutations, were reported (Li et al. 2020). These mutations might not result in antibiotic resistance as they do not change the protein structure and functions (Li et al. 2020). Ke et al. (2020) reported on the presence of mutations in the gyrA gene that did not lead to increased MIC values and thus fluoroquinolone resistance (Ke et al. 2020). Two strains had silent mutations that did not change any protein structure and/or function (Ke et al. 2020). For fluoroquinolone resistance, only mutations in the QRDRs were reported to be associated with resistance (Hamasuna et al. 2018a). A study by Wang et al. (2019) reported on a UP isolate that was resistant to fluoroquinolone (high MIC, 4 mg/ml for levofloxacin) but retained its wild-type sequence (Wang et al. 2019). The evidence presented above suggests that macrolides and fluoroquinolones are becoming inactive against mycoplasmas’ infections.
Dual mutations conferring resistance to the same antibiotic are common. The existence of parC/E and gyrA/B mutations and various other mutations in the V domain of 23S rRNA for macrolide resistance was reported in Mycoplasma (Table S4) and Ureaplasma spp. (Table S5). It is unclear whether the coexistence of A2058/9G (josamycin resistance) and A2062G (pristinamycin resistance) mutations in MG can significantly reduce its transmission potential or its biological fitness (Guschin et al. 2015). In Australia, most reported cases of macrolide resistance in MG infections co-occurred with fluoroquinolone resistance, implying multi-drug resistance in MG isolates. Multidrug-resistant mycoplasmas have been reported in other countries/regions, including the USA (Chambers et al. 2019, Bachmann et al. 2020), Australia (Bodiyabadu et al. 2021), and Japan (Deguchi et al. 2015).
Although antimicrobial resistance is increasing in mycoplasmas, there is still hope in tetracyclines. Tetracycline resistance was lower than 10% in both Mycoplasma and Ureaplasma spp. (Tables S4 and S5), which is also shown in Fig. 3a and b). This is not surprising because, usually, tetracycline is used when macrolides and fluoroquinolones are ineffective. This suggests that most mycoplasmas are still susceptible to tetracycline. For this review, the presence of the tet(M) gene was the only reported mechanism for tetracycline resistance. Nonetheless, the presence of horizontally acquired genes, tet(M), and the Tn1545-like transposon, which were linked to tetracycline resistance in Ureaplasma spp., was reported (Zhu et al. 2012, Boujemaa et al. 2020).
Another trend worth noting is co-infections amongst mycoplasmas, particularly urogenital mycoplasmas. Due to Mycoplasmas’ and Ureaplasmas’ commensal nature, MH usually coexisted with Ureaplasma spp. while MG coexisted with other sexually transmitted infections (Hart et al. 2020). Moreover, Zhu et al. (2012) also reported that 13.91% of UU–UM co-infections showed higher resistance (resistant to seven out of nine antibiotics) than UU or MH single infections (Zhu et al. 2012). The coexistence of different species increases the chances of ineffective treatment owing to dual or multi-drug-resistant infections. MG showed a rich resistome, harboring resistance determinants for more than one antibiotic class. This could be due to its coexistence with various infections other than mycoplasmas, suggesting that it can easily adapt to the environment and antibiotic selection pressure, resulting in a high chance of resistance development. Furthermore, MG was reported to be resistant to more than one antibiotic compared with other mycoplasmas.
Another issue that is worth looking at is the coexistence of different species (polymicrobial infections) and co-resistance to several classes of antibiotics. Polymicrobial infections make the treatment of infections burdensome and also increase the chances of multi-drug resistance in the responsible bacteria (Zhu et al. 2012). At least 10% of co-resistance was reported in this study, with a lower rate in Mycoplasma spp. (2%) but higher than Ureaplasma spp. (8%) (Fig. 3a and b). MG was isolated from polymicrobial infections at a high rate despite lower rates of co-infections in Mycoplasma spp. (Table S3). The likelihood of having macrolide-resistant MG was higher among participants who had received any sexually transmitted infections (STI) diagnoses in the previous 5 years than among those who had none (e.g. 44.4% CI: 18.9%, 73.3% vs. 10.6% CI: 4.6%, 22.6%) (Pitt et al. 2020).
MO macrolide resistance (MPMR) cases were reported in >15 countries in the world (Table S6). These were reported in infants as young as 2 days to adults older than 65 years. Nonetheless, resistance was more common in children than adults (Table S2). This is expected because, unlike fluoroquinolones and tetracyclines, macrolides are the preferred first choice in children due to their low toxicity and lower minimum (Yang et al. 2017). Fluoroquinolones and tetracyclines may cause musculoskeletal problems and tooth discoloration in children, respectively (Lee et al. 2018). Asian countries had the highest burden of macrolide resistance, with Korea ranking high (n = 16 014), followed by China (n = 4242). Although MPMR in Europe is not as high as in Asia, it has been reported in more than five European countries, including France (n = 7), Germany (n = 21), Italy (n = 13), and Spain (n = 16) (Fig. 5).
Macrolide resistance in respiratory mycoplasmas has spread rapidly and widely since 2000, resulting in increased disease severity, complications, and prolonged hospitalization in some cases (Zhou et al. 2014, Pereyre et al. 2016). Infections with macrolide-resistant MP (MRMP) require more time to treat than macrolide-sensitive infections (Chen et al. 2020). Macrolide resistance in MP infections is a concern due to prolonged symptoms. Furthermore, in many cases, symptoms such as fever persist for >2 days despite macrolide usage. Owing to limited therapeutic options, the global rise in MRMP infections is a cause for concern (Chen et al. 2020). The global prevalence of MRMP infections is rapidly increasing, ranging from 23.3% to 100% in Asia, 3.5% to 13.2% in North America, and <10% in European countries (Pereyre et al. 2016). The rising resistance rates in Asia could be due to antibiotics being easily accessible and their non-regulated usage, which leads to antibiotic over-prescription and misuse in this region (Noor et al. 2023). Akashi et al. (2018) reported yearly variations in the prevalence of MRMP infections in Japan, demonstrating an increase from 55.6% to 81.6% between 2008 and 2012, followed by a gradual decrease to 43.6% by 2015 (Akashi et al. 2018). Our analysis revealed an overall decrease in the infection rate. Even so, a country in Asia (Korea) still leads in MP infections (Fig. 5).
The MRMP rate has been increasing yearly, particularly in East Asian countries such as China, Korea, and Japan (Valentine-King et al. 2019). The resistance rate rises as MP infections spread due to the increased demand and use of antibiotics. Understanding the state of resistance through resistance patterns over time will inform the re-evaluation of antibiotic prescription and usage. The widespread use of macrolides has resulted in the rapid global emergence of MRMP, representing >1% and 2% in Asia and Europe, respectively (Eshaghi et al. 2013). Moreover, an increase from 4.9% to 10.2% in MRPM in the USA was observed between 2015 and 2018 (Waites et al. 2019). MRMP has been linked to point mutations and nucleotide displacement in domain V of the 23S rRNA gene (Eshaghi et al. 2013, Meyer Sauteur et al. 2014). In 2012, infection rates of 30%, 18.6%, and 7.5% have been reported in children (2–11 years), teenagers (12–17 years), and adults (18–64 years), respectively (Chan et al. 2013). In recent years, a study by Big Mohammadi et al. (2020) reported MP infection rates of 41.1% in children and 13.0% in adults. Macrolide resistance MP infections is common in children compared to fluoroquinolone and tetracycline resistance. Some macrolides are still effective against some strains of MP (Zhou et al. 2014, Han et al. 2021); in such cases, the second (fluoroquinolone) and third (tetracycline) lines of treatment are not prescribed. Given that MP infections are common in children, macrolide resistance will also be high in this population with lower levels of fluoroquinolone and tetracycline resistance. This may be due to the low administration of fluoroquinolones and tetracyclines owing to their toxicity in children (Lee et al. 2021). Studies have shown no association between gender and resistance rates in respiratory mycoplasmas. However, the opposite is correct for urogenital mycoplasmas. Higher levels of quinolone resistance in MG have been reported in males (19%) than their female counterparts (1.6%) (Sweeney et al. 2019).
Other resistance mechanisms in mycoplasma resistance were biofilm formation and effective efflux pumps. Based on our analysis, antibiotic resistance through biofilm formation and efflux pumps in Mycoplasma and Ureaplasma spp. was not commonly reported. Instructively, Pandelidis et al. (2013) reported biofilm formation in Ureaplasma spp. with no significant effect on antibiotic susceptibility in the isolates. Hence, the rising resistance rates in Ureaplasma spp. should be given more attention. Moreover, a possible ABC-type efflux pump implicated in ciprofloxacin resistance has been detected and reported in MH (Kolesnikova et al. 2020). MH harbors macrolide-specific efflux pump genes. Although resistance through an active efflux pump in MP is not fully understood, Li et al. (2017) reported a possible ABC-type efflux pump that is associated with macrolide resistance in MP. Biofilm formation for antibiotic resistance purposes has been reported in MG strains (Pandelidis et al. 2013, Feng et al. 2015, Daubenspeck et al. 2020) and MP strains (Yan et al. 2020).
Molecular typing of MP is paramount for the surveillance and epidemiology of respiratory infections caused by this species (Dou et al. 2020). This is more useful during MP outbreaks, which are epidemics that repeatedly occur every 4–7 years (Dumke et al. 2015, Diaz et al. 2016, Parrott et al. 2016, Loconsole et al. 2019). This is most likely related to the changes in the p1 gene, which change predominance with time (Loconsole et al. 2019). The p1 gene was analyzed for this study. P1 type 1 was more common in children than in adults (Diaz et al. 2015). A prevalence of 84.4% of P1 type 1 was detected in children, while 12.5% was detected in adults (Diaz et al. 2015). A decrease in MP and MRMP infections between 2012 (n = 628) and 2016 (n = 225) was reported (Nakamura et al. 2021). In addition to the level of antibiotic usage, it was suggested that the decrease in infection rate may be because of the P1 genotype distribution among isolates (Nakamura et al. 2021). Although the P1 subtypes change predominance with MP epidemics, type 2 strains are not as common as the type 1 strains, which are implicated in high levels of MP infection and resistance (Hubert et al. 2021). Macrolide resistance is found in both type 1 and 2 MP strains. A high resistance rate of 82.4% was reported in P1 type 1 strains and 6.5% in other types (Nakamura et al. 2021). Antibiotic resistance through biofilm production differs between P1 types 1 and 2. Type 2 strains produce and express more biofilms as an important virulence factor when in distress (Simmons et al. 2013). This is most likely related to the changes in the attachment organelle, the P1 protein, which changes predominance with time (Loconsole et al. 2019).
This study encountered a few challenges. First, the review was based on the availability of data from published studies, and that may have a possible impact on the overall data analysis. Most of these studies did not provide information on resistance profiles at the species level; therefore, the studies focused more on the presence of detected mutations associated with resistance against macrolides, fluoroquinolones, and tetracyclines. Even so, the data were sufficient to explore the prevalence and resistance profiles of Mycoplasma and Ureaplasma spp. in different countries. Another limitation was that most studies were based more on in vitro analyses, which may need substantial phenotypic tests to support the available data, particularly on the resistance profiles.
The findings from this study paint a concerning picture of widespread antibiotic resistance, with significant geographical and clinical variations that reflect complex patterns of evolution and adaptation. Japan and China are at the forefront of mycoplasmas’ infections and resistance rates. These countries also reported co-infections among urogenital mycoplasmas and the emergence of multi-drug resistance in those infections. The most common resistance mechanisms reported were point mutations for macrolides and fluoroquinolones and the acquisition of the tet(M) gene for tetracycline resistance. The detection of mycoplasma infections is already hard to treat due to their lack of cell walls as one of the antibiotics' targets and rising antibiotic resistance. The detection of the ABC-type efflux pump and biofilm formation as resistance mechanisms in mycoplasmas is a serious concern. Therefore, there is an urgent need for new antimicrobials for the successful treatment of such infections. In addition, surveillance of macrolide, fluoroquinolone, and tetracycline usage is vital to reduce resistant infections and enhance treatment success. The findings from this study have profound implications for clinical practice and public health policy. The high prevalence of resistant Mycoplasma and Ureaplasma strains necessitates a re-evaluation of current treatment guidelines. Moreover, the integration of genomic technologies in routine clinical practice could facilitate more precise and rapid identification of resistant strains, enabling targeted therapies that mitigate the spread of resistance.
Author contributions
Winnie Thabisa Ramaloko (Formal analysis, Investigation, Visualization, Writing––original draft, Writing––review & editing), Nontuthuko Excellent Maningi (Conceptualization, Supervision, Writing––review & editing), and John Osei Sekyere (Conceptualization, Supervision, Writing––review & editing)
Conflict of interest
None declared.
Funding
None.