-
PDF
- Split View
-
Views
-
Cite
Cite
Ezechiel Ngoufack Jagni Semengue, Daniele Armenia, Seth Inzaule, Maria Mercedes Santoro, Béatrice Dambaya, Désiré Takou, Georges Teto, Alex Durand Nka, Bouba Yagai, Lavinia Fabeni, Collins Chenwi, Grâce Angong Beloumou, Sandrine Claire Djupsa Ndjeyep, Vittorio Colizzi, Carlo-Federico Perno, Francesca Ceccherini-Silberstein, Joseph Fokam, Baseline integrase drug resistance mutations and conserved regions across HIV-1 clades in Cameroon: implications for transition to dolutegravir in resource-limited settings, Journal of Antimicrobial Chemotherapy, Volume 76, Issue 5, May 2021, Pages 1277–1285, https://doi.org/10.1093/jac/dkab004
- Share Icon Share
Abstract
Transition to dolutegravir-based regimens in resource-limited settings (RLS) requires prior understanding of HIV-1 integrase variants and conserved regions. Therefore, we evaluated integrase drug resistance mutations (DRMs) and conserved regions amongst integrase strand transfer inhibitor (INSTI)-naive patients harbouring diverse HIV-1 clades in Cameroon.
A cross-sectional study was conducted amongst 918 INSTI-naive patients from Cameroon (89 ART-naive and 829 ART-experienced patients). HIV-1 sequences were interpreted regarding INSTI-DRMs using the Stanford HIVdb v8.9-1 and the 2019 IAS–USA list. Amino acid positions with <1% variability were considered as highly conserved. Subtyping was performed by phylogeny.
Overall prevalence (95% CI) of INSTI-DRMs was 0.8% (0.4–1.7), with 0.0% (0.0–4.0) amongst ART-naive versus 0.9% (0.5–1.9) amongst ART-experienced patients; P = 0.44. Accessory mutations (95% CI) were found in 33.8% (30.9–37.0), with 38.2% (28.1–49.1) amongst ART-naive versus 33.4% (30.4–36.7) amongst ART-experienced patients; P = 0.21. Of 288 HIV-1 integrase amino acid positions, 58.3% were highly conserved across subtypes in the following major regions: V75–G82, E85–P90, H114–G118, K127–W132, E138–G149, Q168–L172, T174–V180, W235–A239 and L241–D253. Wide genetic diversity was found (37 clades), including groups M (92.3%), N (1.4%), O (6.2%) and P (0.1%). Amongst group M, CRF02_AG was predominant (47.4%), with a significantly higher frequency (95% CI) of accessory mutations compared with non-AG [41.4% (36.8–46.0) versus 27.1% (23.3–31.2) respectively; P < 0.001].
The low baseline of INSTI-DRMs (<1%) in Cameroon suggests effectiveness of dolutegravir-based regimens. In spite of high conservation across clades, the variability of accessory mutations between major circulating strains underscores the need for monitoring the selection of INSTI-DRMs while scaling up dolutegravir-based regimens in RLS.
Introduction
Sub-Saharan Africa (SSA) alone carries 70% of global HIV epidemics and about one-third of people living with HIV harbour drug-resistant viruses in this geographical setting.1,2 Moreover, SSA has a wide diversity of HIV (including both HIV types 1 and 2), with an important predominance of HIV-1 non-B subtype viruses that might have clinical significance in terms of viral pathogenesis and treatment response.3 This is particularly true for West and Central Africa, where several clades have been described, with anecdotal clinical implications.4–6
Due to increasing trends of efavirenz/nevirapine pre-treatment drug resistance (above 10%) in several SSA countries, the WHO recommends transitioning from first-generation NNRTI-based regimens to a dolutegravir-based regimen as preferred initial ART and as a possible alternative in managing cases of multiresistance in resource-limited settings (RLS).1,7–10 Integrase strand transfer inhibitors (INSTIs) are the latest approved drug class to treat HIV infection. Specifically, INSTIs stop antiretroviral activity by blocking the integration of HIV proviral DNA into the genetic material of host cells. There are currently four approved drugs belonging to this therapeutic class: raltegravir, elvitegravir, dolutegravir and bictegravir.3,11–16 These INSTIs are known to be highly effective in both treatment-naive and treatment-experienced individuals who may harbour multidrug resistance to other drug classes, with better drug tolerance, fewer drug interactions, higher potency and a genetic barrier to resistance reported with second-generation INSTIs (dolutegravir and bictegravir), both in vitro and in vivo, as supported by the recent WHO guidelines.3,8,12,15–17
As the selection mechanism of drug resistance mutations (DRMs) is known to be subtype-dependent,3,18 HIV-1 integrase resistance patterns, as well as natural polymorphisms, may display distinct pathways in the frame of a broad diversity of HIV-1 clades.3,19,20 Of note, more than 40 substitutions have been associated with the development of resistance to INSTIs in HIV-1 B subtypes.3,21 The most prevalent major mutations are at six key positions, namely T66, E92, Y143, S147, Q148 and N155 in the integrase coding region of HIV-1 across subtypes.3,17 Nonetheless, other INSTI major mutations have been described at lower frequencies and in specific viral strains. Of note, G118R is a mutation that has rarely been observed in subtype B viruses and it has a preferential pathway in selecting dolutegravir resistance in non-B subtype viruses.3,22,23 Also, R263K (which particularly impairs response to dolutegravir-containing regimens) is known to be preferentially selected amongst subtype B viruses compared with other viral subtypes.3,24–29 A few studies have attempted similar analysis on subtype C in South Africa, without exploring viral clades circulating in other African countries.22,30–32 Thus, translating evidence on INSTI-DRMs from settings with B to those with non-B viruses might first require a preliminary assessment of the latter setting for optimal monitoring and shaping of treatment strategies.3,24–29
An in-depth understanding of INSTI-resistance patterns requires informed data across genotypes from real-world evidence. In light of this, the Stanford HIV algorithm for drug resistance interpretation (Stanford HIVdb) compared the variations observed at key positions associated with major and accessory DRMs amongst INSTI-naive versus INSTI-treated patients in 2016 (https://hivdb.stanford.edu/pages/SDRM.worksheet.INI.html). More recently, Tzou et al.33 updated this worksheet by developing a standardized list of INSTI-resistance mutations suitable for surveillance of transmitted INSTI resistance. Similar to the Stanford HIVdb worksheet, the comparison in the Tzou et al. study does not give clear indications for all subtypes and most especially for circulating recombinant forms (CRFs); only CRF01_AE and CRF02_AG are considered. In the meantime, recent viral strains have been identified and new sequences have been generated, revealing an increasing HIV genetic diversity in West and Central African settings.34 Furthermore, in this same setting, Cameroon has been described as an epicentre for HIV in Africa,35 characterized by a very diversified molecular epidemiology.20,36–39 Because knowledge on INSTI-DRMs and polymorphisms remains very limited in this geographical setting, Cameroon henceforth stands as a unique context for a baseline analysis of integrase mutational variations in preparation for a wide scale-up of a tenofovir/lamivudine/dolutegravir regimen in SSA countries.9,10,40–43 Such findings would provide a benchmark for INSTI-DRMs and polymorphisms for optimal surveillance of selected integrase mutations, considering viral diversity, before transitioning to INSTI-based regimens. In this study, we therefore sought to characterize the HIV-1 integrase genotypic profile of INSTI-naive Cameroonian patients and to determine integrase-conserved regions among viral clades circulating locally.
Materials and methods
Design and setting
This was an analytical study conducted from July 2019 to February 2020 amongst people living with HIV-1 residing in Cameroon. Analysis included samples from routine clinical monitoring of HIV genotypic drug resistance at the Virology Laboratory of the Chantal BIYA International Reference Centre for Research on HIV/AIDS Prevention and Management (CIRCB) in Yaoundé, Cameroon and data retrieved from the Los Alamos National Laboratory (LANL) HIV Sequence Database (HIVdb) (https://www.hiv.lanl.gov/components/sequence/HIV/search/search.html).
Ethics
Ethics clearance was obtained from the Cameroon National Ethics Committee for research on human health, under the authorization number 2019/06/34/CE/CNERSH/SP. Given the use of de-identified data that were unlinked from the respective individuals, as well as the retrospective design of the study consisting of stored specimens in the biobank or sequences available in the public repository, informed consent was not applicable, as per local regulations.
Patients
Specimens used for analysis were collected either from stored patient samples received throughout the year 2019 for HIV-1 protease and reverse transcriptase genotyping in routine practice, or from patients’ sequences that were published in the LANL HIVdb. These patients were either naive to ART or experiencing failure to NNRTI- and/or PI-based ART regimens. Data collected from medical records, and further abstracted into the study data collection tool, revealed no cases of patients previously exposed to INSTIs. Sociodemographic (sex, age) and biological (CD4 count and plasma HIV-RNA) parameters at genotyping were obtained from the laboratory database.
All articles related to the LANL HIVdb sequences were searched to ensure that these sequences were obtained from plasma aliquots of INSTI-naive patients. Sequences from other cellular compartments and/or from INSTI-experienced patients were systematically excluded, as well as sequences from patients with unknown INSTI exposure. To exclude the presence of any further duplicates, remaining sequences were checked by using the unique identifiers of each patient and by performing multiple alignments of sequences for the detection of any monophyletic clustering. Demographic data of these sequences were present as complementary information retrieved while downloading some sequences. Furthermore, to ensure representativeness of findings for the study geographical settings, all sequences of HIV-1 subtypes B and C were excluded.
Amplification and sequencing of HIV-1 integrase region
For the sequences obtained from our laboratory, HIV-1 integrase genotyping was performed as previously described by Nanfack et al.44 Briefly, after viral RNA extraction from plasma samples, RNA was reverse-transcribed and amplified. From positive amplicons, DNA sequencing was performed using four overlapping sense and antisense sequence-specific primers. Sequences were obtained after capillary electrophoresis on an Applied Biosystems™ 3500 Genetic Analyzer (Applied Biosystems™, USA) and sequences of at least 864 nucleotides of the integrase region were assembled and manually edited using RECall (CDC, Atlanta, GA, USA).
Interpretation of integrase DRMs
HIV-1 integrase sequences, obtained either from our laboratory routine clinical practice or from the LANL HIVdb, were all combined in one batch and analysed for interpretation of DRMs using both the Stanford HIVdb v8.9-1 (https://hivdb.stanford.edu/dr-summary/comments/INSTI/; last updated 25 October 2019) and the 2019 IAS–USA drug resistance mutations list45 using consensus B (HXB2 from LANL HIVdb; GenBank accession number K03455) as the reference strain for defining DRMs. All variants at amino acid positions associated with decreased INSTI susceptibility were considered as resistant variants. Samples with a resistant mutant or a mixture of WT and mutant at an amino acid position were considered as resistant.
Analysis of polymorphisms and conserved regions
Within the 288 amino acids of HIV-1 integrase sequences generated, the detection of polymorphisms, defined as mutations occurring naturally and frequently in viruses without any selective drug pressure, was set at a threshold of ≥5% variability. Analysis of polymorphic positions was performed by calculating the frequency of mutations and compared statistically by using the chi-squared test. Analysis of conserved regions was based on consensus B as reference strain, with <1% variability defined as highly conserved positions.21
Subtyping of HIV-1 integrase sequences
Subtypes were obtained as previously described.46 Briefly, subtypes were first obtained from the Stanford HIVdb algorithm and then assessed using rapid subtyping tools available online: COMET HIV-1 (https://comet.lih.lu/) and REGA HIV-1 Subtyping Tool v3.0 (http://dbpartners.stanford.edu:8080/RegaSubtyping/stanford-hiv/typingtool/). Subtyping of each individual sequence was confirmed following molecular phylogeny by using MEGA v7 with HIV-1 integrase reference sequences downloaded from LANL.
Statistical analysis
Descriptive statistics were performed for sociodemographic data and biological parameters wherever available. Median and IQR were reported for continuous variables. The chi-squared test and the Fisher’s exact test were used to compare variables where appropriate. P values of ≤0.05 were considered statistically significant. All the analyses were performed using Epi Info v7.
Sequence data
GenBank accession numbers for 73 integrase sequences are MW328641–713.
Results
Patient characteristics
A total of 918 INSTI-naive patients with available HIV-1 integrase sequences from Cameroon were considered within a sampling period of 1994–2019, of which 740 (80.6%) were generated after the year 2000; in particular, 294 (32.03%) were generated after 2007. Seventy-eight were obtained from genotyping of plasma samples at CIRCB and 840 sequences were retrieved from the LANL database. Based on available data, 59.1% (94/159) of our study population were female; the median (IQR) age was 44 (35–49) years; the median (IQR) CD4 cell count at genotyping was 121 (43–353) cells/mm3 and median (IQR) plasma viraemia at genotyping was 99 003 (15 892–411 646) copies/mL. Regarding treatment exposure, all patients were naive to INSTIs, stratified into 89 who had never been exposed to any ART (ART-naive) and 829 who had been exposed to ART for therapeutic management (ART-experienced).
Integrase resistance-associated mutations
Overall prevalence (95% CI) of major INSTI-DRMs was 0.8% (8/918) (0.4–1.7) in the entire study population. According to ART exposure, distribution of INSTI-DRMs was not statistically significant for ART-naive [0.0% (0.0–4.0)] versus ART-experienced [0.9% (0.5–1.9)] patients; P = 0.44. Major INSTI-DRMs were predominantly R263K (2/918), T66A (2/918), E92Q (1/918), G140R (1/918), N155T (1/918), P145S (1/918), Q148H (1/918) and Q148R (1/918). Distribution across patients is summarized in Table 1. The overall prevalence (95% CI) of accessory mutations was 33.8% (311/918) (30.9–37.0), with a rate of 38.2% (34/89) (28.1–49.1) amongst ART-naive versus 33.4% (277/829) (30.4–36.7) amongst ART-experienced patients; P = 0.21. Accessory mutations found were L74I (204/918), L74M (55/918), T97A (48/918), E157Q (30/918), A128T (3/918), Q95K (2/918), V151M (2/918) and V151A (1/918).
Patients . | Treatment exposure . | Major INSTI-DRMs . | Drugs affected . | Viral clade . |
---|---|---|---|---|
1 | ART-experienced | P145PS | EVG | CRF02_AG |
2 | ART-experienced | N155T | EVG, RAL | CRF02_AG |
3 | ART-experienced | T66A | EVG, RAL | CRF02_AG |
4 | ART-experienced | T66TA | EVG, RAL | A1 |
5 | ART-experienced | Q148QHLPR, R263RIKT | BIC, DTG, EVG, RAL | CRF02_AG |
6 | ART-experienced | G140GR | CABa | HIV-1 group O |
7 | ART-experienced | R263RK | BIC, DTG, EVG, RAL | URF |
8 | ART-experienced | E92EKQ | BIC, DTG, EVG, RAL | A1/F2 |
Patients . | Treatment exposure . | Major INSTI-DRMs . | Drugs affected . | Viral clade . |
---|---|---|---|---|
1 | ART-experienced | P145PS | EVG | CRF02_AG |
2 | ART-experienced | N155T | EVG, RAL | CRF02_AG |
3 | ART-experienced | T66A | EVG, RAL | CRF02_AG |
4 | ART-experienced | T66TA | EVG, RAL | A1 |
5 | ART-experienced | Q148QHLPR, R263RIKT | BIC, DTG, EVG, RAL | CRF02_AG |
6 | ART-experienced | G140GR | CABa | HIV-1 group O |
7 | ART-experienced | R263RK | BIC, DTG, EVG, RAL | URF |
8 | ART-experienced | E92EKQ | BIC, DTG, EVG, RAL | A1/F2 |
BIC, bictegravir; CAB, cabotegravir; DTG, dolutegravir; EVG, elvitegravir; RAL, raltegravir; URF, unique recombinant form. Bold represents the resistant variant present at the amino acid position in the sample. Patient numbers (1–8) represent individual patients harbouring the resistant mutations.
CAB is still in Phase 3.
Patients . | Treatment exposure . | Major INSTI-DRMs . | Drugs affected . | Viral clade . |
---|---|---|---|---|
1 | ART-experienced | P145PS | EVG | CRF02_AG |
2 | ART-experienced | N155T | EVG, RAL | CRF02_AG |
3 | ART-experienced | T66A | EVG, RAL | CRF02_AG |
4 | ART-experienced | T66TA | EVG, RAL | A1 |
5 | ART-experienced | Q148QHLPR, R263RIKT | BIC, DTG, EVG, RAL | CRF02_AG |
6 | ART-experienced | G140GR | CABa | HIV-1 group O |
7 | ART-experienced | R263RK | BIC, DTG, EVG, RAL | URF |
8 | ART-experienced | E92EKQ | BIC, DTG, EVG, RAL | A1/F2 |
Patients . | Treatment exposure . | Major INSTI-DRMs . | Drugs affected . | Viral clade . |
---|---|---|---|---|
1 | ART-experienced | P145PS | EVG | CRF02_AG |
2 | ART-experienced | N155T | EVG, RAL | CRF02_AG |
3 | ART-experienced | T66A | EVG, RAL | CRF02_AG |
4 | ART-experienced | T66TA | EVG, RAL | A1 |
5 | ART-experienced | Q148QHLPR, R263RIKT | BIC, DTG, EVG, RAL | CRF02_AG |
6 | ART-experienced | G140GR | CABa | HIV-1 group O |
7 | ART-experienced | R263RK | BIC, DTG, EVG, RAL | URF |
8 | ART-experienced | E92EKQ | BIC, DTG, EVG, RAL | A1/F2 |
BIC, bictegravir; CAB, cabotegravir; DTG, dolutegravir; EVG, elvitegravir; RAL, raltegravir; URF, unique recombinant form. Bold represents the resistant variant present at the amino acid position in the sample. Patient numbers (1–8) represent individual patients harbouring the resistant mutations.
CAB is still in Phase 3.
Polymorphisms and conservation analysis
Overall, polymorphisms (≥5% variability) were found amongst 75/288 (26.0%) amino acid positions in the entire study population. Highly polymorphic positions (≥20% variability) were found predominantly at L234, T112, V201, T124, T125, K136, G134, L101, R283, K14, T206, V31, I135, E11, I72, M50, L74, D167, S255, S119 and D256 (listed from the most to the least polymorphic position). Interestingly, we found many polymorphisms at key resistance positions across subtypes. Table 2 gives a breakdown of all these non-documented variants at resistance positions.
Distribution of amino acid variants at major drug resistance positions according to viral clade
WT amino acids and their positions . | Resistant variantsa . | Most prevalent HIV-1 group M subtypes . | HIV-1 group O (57) . | Other viral clades (183) . | ||||
---|---|---|---|---|---|---|---|---|
CRF02_ AG (435) . | A1 (76) . | G (58) . | F2 (56) . | CRF22_ 01A1 (53) . | ||||
T66 | A, I, K | A(1), E(1) | A(1), S(1) | |||||
E92 | G, Q, V | K(1), S(1) | K(1), Q(1) | |||||
G118 | R | |||||||
F121 | Y | |||||||
E138 | A, K, T | D(3) | D(3) | D(3) | ||||
G140 | A, C, R, S | E(1) | R(1) | |||||
Y143 | A, C, G, H, K, R, S | |||||||
P145 | S | S(1) | ||||||
Q146 | P | L(1) | R(1) | |||||
S147 | G | |||||||
Q148 | H, K, N, R | V(1) | ||||||
V151 | L | A(1), M(1) | I(1), M(1) | P(1) | I(1) | |||
N155 | H, T | T(1) | K(1) | |||||
R263 | K | I(1), K(1), T(1) | K(1) |
WT amino acids and their positions . | Resistant variantsa . | Most prevalent HIV-1 group M subtypes . | HIV-1 group O (57) . | Other viral clades (183) . | ||||
---|---|---|---|---|---|---|---|---|
CRF02_ AG (435) . | A1 (76) . | G (58) . | F2 (56) . | CRF22_ 01A1 (53) . | ||||
T66 | A, I, K | A(1), E(1) | A(1), S(1) | |||||
E92 | G, Q, V | K(1), S(1) | K(1), Q(1) | |||||
G118 | R | |||||||
F121 | Y | |||||||
E138 | A, K, T | D(3) | D(3) | D(3) | ||||
G140 | A, C, R, S | E(1) | R(1) | |||||
Y143 | A, C, G, H, K, R, S | |||||||
P145 | S | S(1) | ||||||
Q146 | P | L(1) | R(1) | |||||
S147 | G | |||||||
Q148 | H, K, N, R | V(1) | ||||||
V151 | L | A(1), M(1) | I(1), M(1) | P(1) | I(1) | |||
N155 | H, T | T(1) | K(1) | |||||
R263 | K | I(1), K(1), T(1) | K(1) |
Other viral clades refer to HIV-1 group N, group P and non-prevalent HIV-1 group M subtypes [pure subtypes (A2, A3, D, F1, H, J, K; CRFs CRF01_AE, CRF06_cpx, CRF09_cpx, CRF11_cpx, CRF13_cpx, CRF18_cpx, CRF19_cpx, CRF25_cpx, CRF26_AU, CRF36_cpx, CRF37_cpx, CRF45_cpx) and unique recombinants (CRF02_AG/A3/A1, CRF09_cpx/H, D/O, F2O, CRF13_cpx/CRF18_cpx etc.)].
Number of patients harbouring the variant is shown in brackets.
Resistant variants were defined according to the Stanford HIVdb algorithm v.8.9-1 and the 2019 IAS–USA drug resistance list.
Distribution of amino acid variants at major drug resistance positions according to viral clade
WT amino acids and their positions . | Resistant variantsa . | Most prevalent HIV-1 group M subtypes . | HIV-1 group O (57) . | Other viral clades (183) . | ||||
---|---|---|---|---|---|---|---|---|
CRF02_ AG (435) . | A1 (76) . | G (58) . | F2 (56) . | CRF22_ 01A1 (53) . | ||||
T66 | A, I, K | A(1), E(1) | A(1), S(1) | |||||
E92 | G, Q, V | K(1), S(1) | K(1), Q(1) | |||||
G118 | R | |||||||
F121 | Y | |||||||
E138 | A, K, T | D(3) | D(3) | D(3) | ||||
G140 | A, C, R, S | E(1) | R(1) | |||||
Y143 | A, C, G, H, K, R, S | |||||||
P145 | S | S(1) | ||||||
Q146 | P | L(1) | R(1) | |||||
S147 | G | |||||||
Q148 | H, K, N, R | V(1) | ||||||
V151 | L | A(1), M(1) | I(1), M(1) | P(1) | I(1) | |||
N155 | H, T | T(1) | K(1) | |||||
R263 | K | I(1), K(1), T(1) | K(1) |
WT amino acids and their positions . | Resistant variantsa . | Most prevalent HIV-1 group M subtypes . | HIV-1 group O (57) . | Other viral clades (183) . | ||||
---|---|---|---|---|---|---|---|---|
CRF02_ AG (435) . | A1 (76) . | G (58) . | F2 (56) . | CRF22_ 01A1 (53) . | ||||
T66 | A, I, K | A(1), E(1) | A(1), S(1) | |||||
E92 | G, Q, V | K(1), S(1) | K(1), Q(1) | |||||
G118 | R | |||||||
F121 | Y | |||||||
E138 | A, K, T | D(3) | D(3) | D(3) | ||||
G140 | A, C, R, S | E(1) | R(1) | |||||
Y143 | A, C, G, H, K, R, S | |||||||
P145 | S | S(1) | ||||||
Q146 | P | L(1) | R(1) | |||||
S147 | G | |||||||
Q148 | H, K, N, R | V(1) | ||||||
V151 | L | A(1), M(1) | I(1), M(1) | P(1) | I(1) | |||
N155 | H, T | T(1) | K(1) | |||||
R263 | K | I(1), K(1), T(1) | K(1) |
Other viral clades refer to HIV-1 group N, group P and non-prevalent HIV-1 group M subtypes [pure subtypes (A2, A3, D, F1, H, J, K; CRFs CRF01_AE, CRF06_cpx, CRF09_cpx, CRF11_cpx, CRF13_cpx, CRF18_cpx, CRF19_cpx, CRF25_cpx, CRF26_AU, CRF36_cpx, CRF37_cpx, CRF45_cpx) and unique recombinants (CRF02_AG/A3/A1, CRF09_cpx/H, D/O, F2O, CRF13_cpx/CRF18_cpx etc.)].
Number of patients harbouring the variant is shown in brackets.
Resistant variants were defined according to the Stanford HIVdb algorithm v.8.9-1 and the 2019 IAS–USA drug resistance list.
Conservation analysis revealed highly conserved amino acid positions (<1% variability) accounting for 168/288 (58.3%) conserved codons and these invariant residues were scattered throughout the entire sequence either individually, in pairs, triplets or quadruplets or within invariant regions. The nine longest invariant regions made up of 5 to 15 consecutive amino acids were I (V75–G82), II (E85–P90), III (H114–G118), IV (K127–W132), V (E138–G149), VI (Q168–L172), VII (T174–V180), VIII (W235–A239) and IX (L241–D253), as shown in Figure 1.
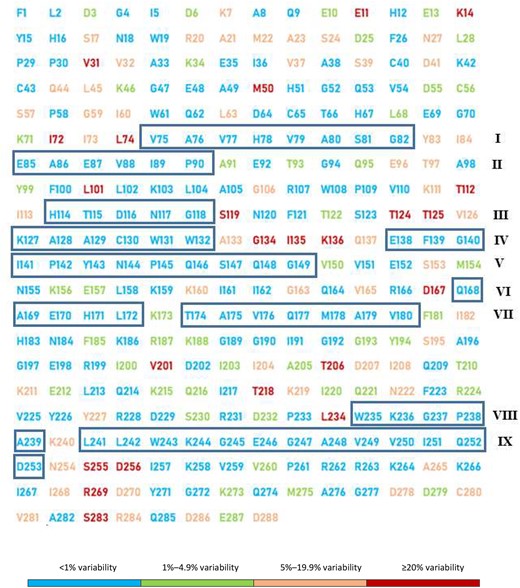
Conservation degree of integrase amongst the 37 HIV-1 clades found locally. Sequences containing the 288 amino acids of HIV-1 integrase were aligned and compared with the reference strain B (HXB2) for the entire study population. Depiction of HIV-1 integrase is coloured according to the frequency of each mutation such that blue corresponds to highly conserved amino acids (<1% variability), green corresponds to weakly conserved amino acids (1%–4.9% variability), orange corresponds to non-conserved amino acids or polymorphisms (5%–19.9% variability) and red represents highly polymorphic positions (≥20% variability).This figure appears in colour in the online version of JAC and in black and white in the printed version of JAC.
Genetic diversity
Following molecular phylogeny, 37 HIV-1 clades were found, which included groups M (92.3%), N (1.4%), O (6.2%) and P (0.1%). HIV-1 group M subtypes included pure subtypes (A1, A2, A3, D, F1, F2, G, H, J and K), CRFs (CRF01_AE, CRF02_AG, CRF06_cpx, CRF09_cpx, CRF11_cpx, CRF13_cpx, CRF18_cpx, CRF19_cpx, CRF22_01A1, CRF25_cpx, CRF26_AU, CRF36_cpx, CRF37_cpx and CRF45_cpx) and unique recombinants (CRF02_AG/A3/A1, CRF09_cpx/H, D/O, F2O, CRF13_cpx/CRF18_cpx etc.). The most predominant clades were CRF02_AG (47.4%), A1 (8.3%), G (6.3%), F2 (6.1%) and CRF22_01A1 (5.8%).
According to national HIV-1 molecular epidemiology (CRF02_AG versus non-02_AG), the overall distribution (95% CI) of both major and accessory DRMs combined was significantly higher amongst CRF02_AG (42.1%) (37.5–46.7) compared with non-CRF02_AG (28.1%) (24.3–32.3); P < 0.0001. This mutational variability was driven by accessory DRMs [41.4% (36.8–46.0) for CRF02_AG versus 27.1% (23.3–31.2) for non-CRF02_AG; P < 0.0001]. However, following stratification by ART exposure, the distribution of accessory mutations amongst CRF02_AG versus non-CRF02_AG was found to be similar in both ART-naive (42.8% versus 32.5%) and ART-experienced (41.2% versus 26.6%) patients; P = 0.42. Detailed distribution of these accessory mutations across subtypes is presented in Table 3. Interestingly, fewer polymorphisms were found amongst CRF02_AG (14.2%) compared with non-CRF02_AG (27.8%), with a statistically significant difference (P = 0.027). Table S1, available as Supplementary data at JAC Online, gives a breakdown of all mutational variants encountered at each of the 288 amino acid positions of HIV-1 integrase, according to subtypes and treatment exposure.
Viral clade (n) . | Frequency of accessory DRMs, n (%) . | 95% CI . | P valuea . |
---|---|---|---|
CRF02_AG (435) | 180 (41.4) | 36.8–46.1 | — |
A1 (76) | 14 (18.4) | 10.4–28.9 | <0.0001 |
G (58) | 12 (20.7) | 11.2–33.3 | 0.0012 |
F2 (56) | 8 (14.3) | 6.4–26.2 | <0.0001 |
CRF22_01A1 (53) | 1 (1.89) | 0.05–10.1 | <0.0001 |
CRF11_cpx (45) | 7 (15.5) | 6.5–29.4 | 0.0003 |
CRF13_cpx (20) | 7 (35.0) | 15.4–59.2 | 0.37 |
Other HIV-1 M subtypes (104) | 23 (22.1) | 14.6–31.3 | 0.0001 |
HIV-1 O (57) | 57 (100.0) | 93.7–100.0 | <0.0001 |
HIV-1 N (13) | 1 (7.7) | 0.2–36.0 | 0.01 |
HIV-1 P (1) | 1 (100.0) | 2.5–100.0 | 0.41 |
Viral clade (n) . | Frequency of accessory DRMs, n (%) . | 95% CI . | P valuea . |
---|---|---|---|
CRF02_AG (435) | 180 (41.4) | 36.8–46.1 | — |
A1 (76) | 14 (18.4) | 10.4–28.9 | <0.0001 |
G (58) | 12 (20.7) | 11.2–33.3 | 0.0012 |
F2 (56) | 8 (14.3) | 6.4–26.2 | <0.0001 |
CRF22_01A1 (53) | 1 (1.89) | 0.05–10.1 | <0.0001 |
CRF11_cpx (45) | 7 (15.5) | 6.5–29.4 | 0.0003 |
CRF13_cpx (20) | 7 (35.0) | 15.4–59.2 | 0.37 |
Other HIV-1 M subtypes (104) | 23 (22.1) | 14.6–31.3 | 0.0001 |
HIV-1 O (57) | 57 (100.0) | 93.7–100.0 | <0.0001 |
HIV-1 N (13) | 1 (7.7) | 0.2–36.0 | 0.01 |
HIV-1 P (1) | 1 (100.0) | 2.5–100.0 | 0.41 |
P values were obtained by comparing estimates of each subtype with CRF02_AG. The table highlights the significant association of low prevalence of accessory DRMs within all subtypes compared with CRF02_AG except for CRF13_cpx and HIV-1 group P.
Viral clade (n) . | Frequency of accessory DRMs, n (%) . | 95% CI . | P valuea . |
---|---|---|---|
CRF02_AG (435) | 180 (41.4) | 36.8–46.1 | — |
A1 (76) | 14 (18.4) | 10.4–28.9 | <0.0001 |
G (58) | 12 (20.7) | 11.2–33.3 | 0.0012 |
F2 (56) | 8 (14.3) | 6.4–26.2 | <0.0001 |
CRF22_01A1 (53) | 1 (1.89) | 0.05–10.1 | <0.0001 |
CRF11_cpx (45) | 7 (15.5) | 6.5–29.4 | 0.0003 |
CRF13_cpx (20) | 7 (35.0) | 15.4–59.2 | 0.37 |
Other HIV-1 M subtypes (104) | 23 (22.1) | 14.6–31.3 | 0.0001 |
HIV-1 O (57) | 57 (100.0) | 93.7–100.0 | <0.0001 |
HIV-1 N (13) | 1 (7.7) | 0.2–36.0 | 0.01 |
HIV-1 P (1) | 1 (100.0) | 2.5–100.0 | 0.41 |
Viral clade (n) . | Frequency of accessory DRMs, n (%) . | 95% CI . | P valuea . |
---|---|---|---|
CRF02_AG (435) | 180 (41.4) | 36.8–46.1 | — |
A1 (76) | 14 (18.4) | 10.4–28.9 | <0.0001 |
G (58) | 12 (20.7) | 11.2–33.3 | 0.0012 |
F2 (56) | 8 (14.3) | 6.4–26.2 | <0.0001 |
CRF22_01A1 (53) | 1 (1.89) | 0.05–10.1 | <0.0001 |
CRF11_cpx (45) | 7 (15.5) | 6.5–29.4 | 0.0003 |
CRF13_cpx (20) | 7 (35.0) | 15.4–59.2 | 0.37 |
Other HIV-1 M subtypes (104) | 23 (22.1) | 14.6–31.3 | 0.0001 |
HIV-1 O (57) | 57 (100.0) | 93.7–100.0 | <0.0001 |
HIV-1 N (13) | 1 (7.7) | 0.2–36.0 | 0.01 |
HIV-1 P (1) | 1 (100.0) | 2.5–100.0 | 0.41 |
P values were obtained by comparing estimates of each subtype with CRF02_AG. The table highlights the significant association of low prevalence of accessory DRMs within all subtypes compared with CRF02_AG except for CRF13_cpx and HIV-1 group P.
Discussion
Before transitioning to dolutegravir-based ART for treating HIV infection in SSA,1,7–10 it is essential to understand the baseline data of INSTI-DRMs and polymorphisms, as well as the variability of the mutations in the frame of the broad viral diversity.19,31,32 At the moment, a detailed characterization of INSTI mutational patterns would serve as a benchmark in monitoring the dynamics and evolutionary trends of INSTI-DRMs, therefore giving a unique opportunity to detect potentially selected novel INSTI mutations while scaling up dolutegravir-based regimens across non-B clades circulating in RLS.20,36–39
In Cameroon, transitioning to tenofovir/lamivudine/dolutegravir as the first-line regimen for treatment initiation was launched as of 1 January 2020 (2019 World AIDS Day activities, Cameroon). Additionally, the country recommends dolutegravir and ritonavir-boosted darunavir in combination with two optimized NRTIs (selection guided by genotyping) for a third-line ART regimen after confirmed failure to second-line therapy. We report here a very low level of INSTI-DRMs; the HIV-1 integrase region across viral clades showed relatively high conservation and the disparity of mutational patterns appeared significant with broad genetic diversity.
Our study population, potentially made of adults with immunological and virological failure, underscores late clinical detection of treatment failure in our context, as previously reported in Cameroon.47,48 The current data therefore reflect the real-life situation of people living with HIV in Cameroon, suggesting the representativeness and programmatic relevance of our findings.
Specifically, we found that in patients who had never been exposed to INSTIs, major DRMs were found in only 0.8% of patients. This expected low level of major INSTI-DRMs is similar to that found worldwide and confirms the limited current exposure to INSTIs and the relevance of exploiting these findings for a rationale including monitoring of emerging INSTI-DRMs over time.21,26,30,41,49–56 However, an intriguing finding is the fact that a dolutegravir-resistance signature, through R263K mutation,3,24–29,33 was found in two patients infected with recombinant viruses (CRF02_AG and URF). This calls for long-term clinical monitoring of patients with such pre-existing DRMs, in order to ascertain the likelihood of failure to dolutegravir-based regimens during routine clinical care.
Regarding accessory mutations, more than 33% of the study population harboured at least one mutation. Some of these mutations (L74I/M, T97A and E157Q), classified as polymorphic mutations by the Stanford HIVdb algorithm, are well documented in the population of INSTI-naive patients; of note, these accessory mutations may contribute to drug resistance21,28,57–62 and affect viral replicative fitness,59 if in combination with major DRMs. On the other hand, non-polymorphic mutations (such as Q95K, A128T and V151A/M), though not well documented, were also found in this population of INSTI-naive patients. Importantly, these non-polymorphisms are reported to have minimal effect on dolutegravir when present alone and a more clinically important effect when present with major DRMs.28,57,58,63,64 However, understanding their significance after failure on INSTI-based regimens in RLS like Cameroon would serve to inform policies in future ART programmes in RLS.28,57,58,63,64
This study also enables the identification of conserved HIV-1 integrase across non-B and non-C subtypes circulating in Cameroon. Interestingly, genetically conserved regions were significantly lower than those reported for B subtypes by Ceccherini-Silberstein et al.21 (58.3% versus 65%; P = 0.028) and those found in HIV-1 group M subtypes by Rhee et al.54 (58.3% versus 65.3%; P = 0.024). This lower conservation in our study could be related to the wider or broad viral diversity, thus underlining the need for close monitoring while scaling up dolutegravir-based regimens. In general, the majority of amino acids involved in key functions of the enzyme often appeared to be highly conserved (<1% variability) within the N-terminal domain [i.e. the zinc-binding HHCC motif (H12, H16, C40, C43) involved in the multimerization of integrase subunits and interaction with LEDGF/p75],21 in the catalytic core domain [i.e. the catalytic triade DDE (D64, D116, E152) involved in all catalytic processes of the enzyme]21 and the C-terminal domain (i.e. regions C235WKGPAKLLWKGEGAVV250 and N259VVPRRK264, which are conserved in all retroviruses and are essential for viral replication).21 Exceptions were made for two regions of the catalytic core domain (I161–K173 and K186–K188) and two residues of the C-terminal domain (K240 and V260). The I161–K173 region, known to be involved in the non-canonical nuclear localization signal, appeared to be polymorphic (≥5% variability) in its residues G163, V165 and D167 (the first two being associated with in vitro and/or in vivo resistance to different INSTIs)21 and weakly conserved (1%–4.9% variability) in its residue K173. On the other hand, in the KRK motif (K186, R187, K188), important for the integrase:integrase oligomerization at the dimer:dimer interface, residues R187 and K188 were weakly conserved, but with no or minimal effect on viral infectivity.21,54 Residues K240 and V260, respectively, appeared to be highly variable and weakly conserved in our dataset, but their effect on viral replication is still to be studied. Overall, polymorphisms and conservation analysis in our dataset revealed that previously described major INSTI (including dolutegravir)-resistance patterns appeared to be conserved, henceforth postulating an in silico similarity of integrase-resistance pathways with observations in B subtypes.21In vivo studies, following transition to tenofovir/lamivudine/dolutegravir, would be highly informative for shaping INSTI-ART strategy in RLS.
Finally, we obtained 37 different HIV clades in the integrase regions, with CRF02_AG (47.4%) being predominant. Though these results are concordant with the broad HIV genetic variability in Cameroon,1,7–10 the significantly higher frequency of accessory DRMs in AG subtypes (41.1%, P < 0.0001) suggests a rationale involving careful therapeutic monitoring of patients, according to subtypes after transitioning to dolutegravir-based regimens, in these settings.
The study design does not give room for understanding the phenotypic effect of the described mutational variants. This warrants further assessments of recently described INSTI mutations (https://hivdb.stanford.edu/dr-summary/comments/INSTI/), in terms of the clinical outcomes, as tenofovir/lamivudine/dolutegravir is rolled out in RLS. However, these findings serve as a local baseline database for the monitoring of INSTI-based regimens prior to any change within conserved residues under this new ART paradigm.
In conclusion, the low level of HIV-1 INSTI-DRMs (<1%) and the consistent conservation of the integrase region in this Cameroonian population suggest effectiveness of dolutegravir-based regimens within the national ART programme. However, transitioning to this new regimen, in the context of diverse viral strains, requires monitoring of the dynamics of selected DRMs for possible updates and considerations in future treatment paradigms.
Acknowledgements
We thank the CIRCB for hosting the present study and for all the facilitations. We are also very appreciative to the support team from LANL HIVdb for their technical assistance in the early phase of this study while retrieving sequences from the database.
Funding
The study was supported technically and financially by the Chantal BIYA International Reference Centre for Research on HIV/AIDS Prevention and Management (CIRCB) and the Chair of Virology of the Faculty of Medicine and Surgery of the University of Rome “Tor Vergata”.
Transparency declarations
None to declare.
Supplementary data
Table S1 is available as Supplementary data at JAC Online.
References
WHO. Updated Recommendations on First-Line and Second-Line Antiretroviral Regimens and Post-Exposure Prophylaxis and Recommendations on Early Infant Diagnosis of HIV - Interim Guidance.
WHO. HIV Drug Resistance Report 2017.
WHO. Consolidated Guidelines on the use of Antiretroviral Drugs for Treating and Preventing HIV infection.
Republic of Cameroon, Ministry of Public Health, Chantal BIYA International Reference Centre. New HIV guidelines and strategies against drug resistance. 2019 World AIDS Day activities; 21–22 November
Stanford University. INSTI Resistance Comments - HIV Drug Resistance Database.