-
PDF
- Split View
-
Views
-
Cite
Cite
Stefan Riedel, Divya Vijayakumar, Gretchen Berg, Anthony D Kang, Kenneth P Smith, James E Kirby, Evaluation of apramycin against spectinomycin-resistant and -susceptible strains of Neisseria gonorrhoeae, Journal of Antimicrobial Chemotherapy, Volume 74, Issue 5, May 2019, Pages 1311–1316, https://doi.org/10.1093/jac/dkz012
- Share Icon Share
Abstract
The emergence of Neisseria gonorrhoeae resistant to all currently available antimicrobial therapies poses a dire public health threat. New antimicrobial agents with activity against N. gonorrhoeae are urgently needed. Apramycin is an aminocyclitol aminoglycoside with broad-spectrum in vitro activity against MDR Gram-negative pathogens and Staphylococcus aureus. However, its activity against N. gonorrhoeae has not been described.
The activity spectrum of apramycin against a collection of MDR N. gonorrhoeae was assessed. Isolates tested included those susceptible and resistant to the structurally distinct aminocyclitol, spectinomycin.
The modal MICs for apramycin and spectinomycin were 16 mg/L and 32 mg/L, respectively. The epidemiological cut-off (ECOFF) for apramycin was 64 mg/L. No strains among 77 tested had an MIC above this ECOFF, suggesting very low levels of acquired apramycin resistance. In time–kill analysis, apramycin demonstrated rapid bactericidal activity comparable to that of spectinomycin.
Apramycin has broad-spectrum, rapidly bactericidal activity against N. gonorrhoeae. Future pharmacokinetic and pharmacodynamic studies will be needed to determine whether apramycin and/or apramycin derivatives hold promise as new therapeutics for N. gonorrhoeae infection.
Introduction
Neisseria gonorrhoeae is a sexually transmitted pathogen that continues to present a significant and global public health challenge. According to the data from global sexually transmitted infection surveillance networks, an estimated 78 million cases of gonorrhoea are diagnosed each year.1 With the introduction of effective antimicrobial agents in the 1940s, gonorrhoea could be reliably treated; however, during the past few decades, successful treatment has become significantly more difficult due to the organism’s propensity to develop resistance to the antimicrobial agents typically used for treatment.2–4
Antimicrobial resistance (AMR) in N. gonorrhoeae occurs by several mechanisms: drug inactivation, alteration of antimicrobial targets, efflux pumps and/or decreased antimicrobial uptake. Several regional and global surveillance networks for AMR in N. gonorrhoeae have raised concerns regarding emerging MDR based on these mechanisms that will ultimately lead to infection that is effectively untreatable with currently available agents.4–6
In 2012, the WHO published its ‘Global Action Plan to Control the Spread and Impact of Antimicrobial Resistance in Neisseria gonorrhoeae’.7,8 This plan contains three important core components: rigorous AMR surveillance; early detection of AMR and treatment failures in individual patients; and development of antimicrobials with unique mechanisms of action. In response to this threat, several new antimicrobial agents, such as solithromycin (a fluoroketolide), eravacycline (a glycylcycline) and zoliflodacin (a spiropyrimidinetrione), are in development.9–14 However, their potential contribution to treatment shortfalls and staying power against emerging resistance in N. gonorrhoeae is not yet established. Several studies have suggested that further evaluation of existing antimicrobial agents such as ertapenem, fosfomycin and gentamicin may be warranted.15,16
Aminoglycosides are potent Gram-negative agents with potential activity against N. gonorrhoeae.17 Concerns about treatment-associated ototoxicity and nephrotoxicity have generally precluded their use in N. gonorrhoeae treatment. However, gentamicin is the first line of treatment in Malawi, based on cost, proven efficacy and lack of obvious toxic effects after a single intramuscular injection.18,19 Gentamicin has been used either alone or in combination with doxycycline.18,19 The emergence of isolates with reduced susceptibility, but not resistance, has been variably observed in different longitudinal studies.18–22 Interestingly, the structurally distinct aminocyclitols, spectinomycin and apramycin, are known or believed to have significantly lower risk of these side effects.23,24 Spectinomycin is an approved agent for N. gonorrhoeae treatment via intramuscular injection and resistance is rarely observed.25 However, this agent is neither routinely available nor routinely used for human therapy.25 It is unavailable in 30 of 38 European countries and in the USA.26
Apramycin is currently available as a veterinary treatment for bovine mastitis and diarrhoeal disease in farm animals.27–30 It possesses an unusual bicyclic octadiose aminosugar linked to a monosubstituted 4-O-deoxystreptamine moiety. Apramycin was originally isolated in 1967 from Streptomyces tenebrarius obtained from a soil sample from Sonora, Mexico.31–33 Apramycin is believed to bind to the 16S rRNA A-decoding site of the 30S ribosomal subunit and thereby inhibit peptide chain elongation and also lead to incorporation of non-cognate amino acids through induced miscoding activity.34 Resistance is primarily conferred by a single aminoglycoside modifying enzyme, AAC(3)-IV, which circulates at very low frequency in Gram-negative pathogens.35,36 Importantly, in contrast to other aminoglycosides, apramycin’s activity is not blocked by circulating G1405 rRNA methylases which are found with increasing frequency in NDM-1-carbapenemase-producing Enterobacteriaceae.27,37–40
Apramycin demonstrates broad-spectrum in vitro activity against human isolates of MDR Acinetobacter baumannii, Pseudomonas aeruginosa, carbapenem-resistant Enterobacteriaceae and Staphylococcus aureus,15,16,35,36,41,42 and rapid in vitro bactericidal activity.43 It has also demonstrated in vivo activity against A. baumannii, S. aureus and Mycobacterium tuberculosis in murine models.43,44 Therefore, based on previously demonstrated broad-spectrum activity and other compelling properties, we evaluated in vitro activity of apramycin against contemporary clinical strains of N. gonorrhoeae as a first step in assessing whether apramycin or potential derivatives of apramycin might serve as future therapeutics against this problematic pathogen.
Materials and methods
Bacterial isolates
A total of 72 clinical isolates of N. gonorrhoeae were tested against spectinomycin and apramycin. Forty-nine isolates were obtained from the FDA-CDC Antimicrobial Resistance Isolate Bank (https://www.cdc.gov/drugresistance/resistance-bank/). Twenty-one isolates were from the CDC Gonococcal Isolate Surveillance Program (GISP) Bank at Beth Israel Deaconess Medical Center (BIDMC) and were obtained from several locations in the USA (Chicago, IL; Minneapolis, MN; New York, NY; Boston, MA; and Erie, PA). Testing of de-identified GISP isolates was approved by the Institution Review Board at BIDMC. Three spectinomycin-resistant isolates were obtained from the Culture Collection University of Gothenburg (CCUG): CCUG 15821 (WHO-A); CCUG 57601 (WHO-O) and CCUG 41811. ATCC 49226/F-18 and CDC F-28 are spectinomycin-susceptible and -resistant quality control strains, respectively. These quality control strains were tested for spectinomycin susceptibility in duplicate on each day of testing and results were consistently within the acceptable range. Among the FDA-CDC Antimicrobial Resistance Isolate Bank isolates, 100%, 82%, 100%, 2%, 80% and 0% were non-susceptible to penicillin, ciprofloxacin, tetracycline, ceftriaxone, cefpodoxime and spectinomycin, respectively, based on CLSI susceptibility criteria (i.e. MIC >0.06, >0.06, >0.25, >0.25, >0.5 and >32 mg/L, respectively) and strain MIC data.
Agar dilution (AD) antimicrobial susceptibility testing (AST)
Spectinomycin was obtained from Sigma–Aldrich (St Louis, MO, USA) or Alfa Aesar (Tewksbury, MA, USA), and apramycin was obtained from Alfa Aesar. AST was performed using the AD method following CLSI guidelines and the CDC’s GISP protocol for AST of N. gonorrhoeae.45,46 From spectinomycin stock solutions, appropriate working concentrations were prepared to achieve a range of test concentrations from 0.5 to 1024 mg/L. Similarly, from apramycin stock solutions, appropriate working concentrations were prepared to achieve a range of test concentrations from 0.5 to 256 mg/L.
For inoculum preparation, colonies of all N. gonorrhoeae isolates (including the quality control strains) from a chocolate agar plate (20–24 h of incubation) were suspended in Mueller–Hinton broth to prepare a solution adjusted to a 0.5 McFarland standard density. The agar plates were inoculated with 1–2 μL of each suspension using a Steers inoculum-replicating apparatus. Agar growth control plates (no antimicrobial agent added) were inoculated at the beginning and end of every test run to ensure that there was no contamination or antimicrobial carry-over during inoculation. The endpoints for determining the MIC by AD testing were interpreted as no visible growth on an agar plate for a specific antimicrobial concentration. The CLSI categorical interpretive criteria of ≤32 mg/L, susceptible; 64 mg/L, intermediate; and ≥128 mg/L, resistant were applied for spectinomycin.24
Time–kill studies
Time–kill studies were performed according to CLSI recommendations47,48 with substitution of Wade–Graver liquid medium (WGM), as previously described,17,49 to permit robust growth of N. gonorrhoeae. Antibiotic stocks were diluted in 10 mL of WGM in 25 × 150 mm glass round-bottom tubes to achieve multiples of the MIC for each strain tested. To prepare the inoculum, 100 μL of a 0.5 McFarland suspension of colonies from an overnight chocolate agar plate (Remel, Lenexa, KS, USA) were added to 5 mL of WGM and incubated at 35°C in a 5% CO2 incubator for 8–10 h until log phase (i.e. 1.0–1.5 McFarland). The culture was then adjusted to a turbidity of 1.0 McFarland and 200 μL was inoculated into each growth tube containing antibiotic dilutions.
During incubation of tube cultures on a shaking platform at 35°C, 5% CO2 atmosphere, aliquots were removed at indicated timepoints and 10-fold serial dilutions prepared in 0.9% sodium chloride. A 10 μL drop from each dilution was spotted on a chocolate agar plate and incubated overnight. Drops containing 3 to 30 colonies were considered ‘countable’ and used for cfu determination. If more than one dilution was countable, the cfu of the two dilutions was averaged. If no drops were countable, consecutive drops above and below the countable range were averaged. The limit of detection was 300 cfu/mL. Antibiotic carry-over effect was not observed. Bactericidal activity was defined as a ≥ 3 log10 cfu/mL reduction sustained at 24 h of incubation at ≤4× the MIC determined by AD.
Genomic analysis
We queried the AAC(3)-IV and ApmA protein sequence against all predicted proteins from N. gonorrhoeae available at the NCBI using the BlastP50 algorithm with an expect value (e-value) cut-off of <10−10. All N. gonorrhoeae protein sequences available in the CARD Prevalence, Resistomes, & Variants database (https://card.mcmaster.ca/download), which uses a more conservative e-value threshold of <10−30, were also screened for matches to all known apramycin resistance determinants.51,52
Results and discussion
A total of 72 strains of N. gonorrhoeae were tested. MIC distributions for apramycin and spectinomycin are shown in Figure 1(a and b), respectively. The modal MICs for apramycin and spectinomycin were 16 mg/L and 32 mg/L, respectively. No categorical interpretive breakpoints are available for apramycin from either EUCAST or CLSI and therefore categorical assessment was not made. An apramycin epidemiological cut-off value (ECOFF) of 64 mg/L was assigned based on visual inspection.53 There were no strains with an apramycin MIC above this value, suggesting absence of acquired resistance in the tested strain set.
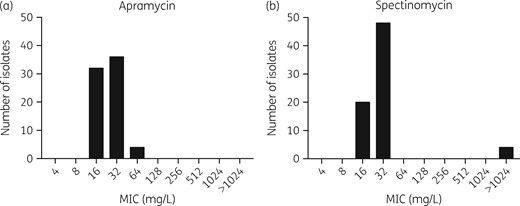
Apramycin and spectinomycin MIC distribution for N. gonorrhoeae.
For the 68 spectinomycin-susceptible strains of N. gonorrhoeae, 56 isolates (82%) had identical apramycin and spectinomycin MIC values; 13 isolates (19%) had a 2-fold dilution lower apramycin MIC; and 3 isolates (4%) and 4 isolates (6%) had a 2-fold and 4-fold dilution higher apramycin MIC, respectively. Four known spectinomycin-resistant isolates were tested and confirmed to be spectinomycin resistant (MIC >1024 mg/L). WHO-O contains the C1192T spectinomycin resistance mutation in the 16S rRNA gene.25 WHO-A contains the T22P spectinomycin resistance mutation in the ribosomal S5 protein (encoded by the rpsE gene).25 The mutations in F-28 and CCUG 41811 have not yet been characterized. Notably, high-level spectinomycin resistance in these strains did not confer detectable cross-resistance to apramycin. Two of the spectinomycin-resistant isolates had an apramycin MIC of 16 mg/L and two had an apramycin MIC of 32 mg/L, consistent with findings in spectinomycin-susceptible strains.
Four representative strains were tested in time–kill analysis including the ATCC type strain F-18; spectinomycin-resistant F-28; and FDA-CDC Isolate Bank strains 193 and 200. Rapid, sustained bactericidal activity was observed for both apramycin and spectinomycin within 4 h, with the exception, as expected, for spectinomycin in the spectinomycin-resistant strain, F-28 (Figure 2). Time–kill results were consistent with prior observations of rapid bactericidal activity of spectinomycin17,54 and gentamicin17 for N. gonorrhoeae. Our data suggest that apramycin also exhibits similar bactericidal activity.
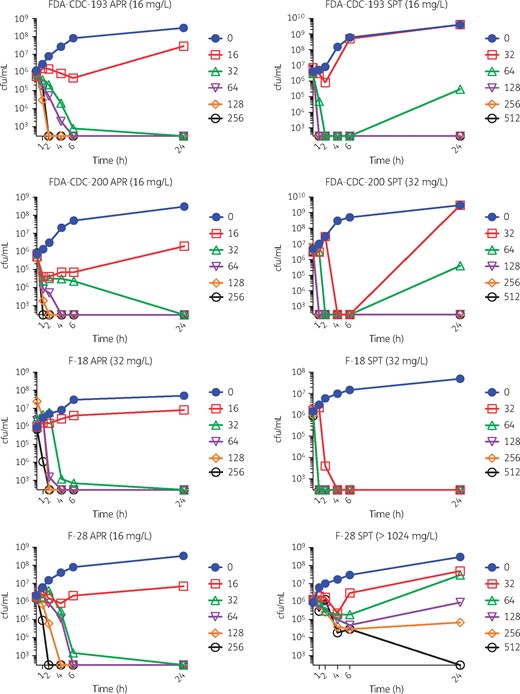
Time–kill analysis. Apramycin (APR) and spectinomycin (SPT) were tested against various strains (MIC values are indicated in parentheses in panel titles). A ‘no antibiotic’ control and a doubling dilution series of increasing concentrations tested (mg/L) are indicated, respectively, by filled circles, open squares, open triangles, open inverted triangles, open diamonds and open circles. Specific concentrations tested (mg/L) are indicated in respective panel legends. Both apramycin and spectinomycin were bactericidal. This figure appears in colour in the online version of JAC and in black and white in the print version of JAC.
In Gram-negative organisms, a single aminoglycoside-modifying enzyme, AAC(3)-IV, has been described that inactivates apramycin through acetylation of the C-3 amine on the deoxystreptamine ring.55 The presence of this resistance element is rare, even in MDR organisms such as carbapenem-resistant Enterobacteriaceae and A. baumannii, consistent with the infrequency of organisms with MICs above the ECOFFs for these pathogens.36,43 A BLASTP56 search performed on 12 August 2018 for AAC(3)-IV found no matches to N. gonorrhoeae among the 451 complete genomes and other N. gonorrhoeae sequences available in the NCBI databases.51 Similarly, no significant homology was found with ApmA, an aminoglycoside-modifying enzyme, which also inactivates apramycin, and has been described recently in two staphylococcal porcine isolates.57,58
Of note, apramycin remains active in strains expressing ribosomal methylases that modify 16S rRNA at position G1405, in contrast to aminoglycosides currently used for human therapy and the novel aminoglycoside, plazomicin.59 In contrast, activity of both apramycin and the aforementioned aminoglycosides are blocked by NpmA, identified in one Escherichia coli clinical isolate, and KamB, found in aminoglycoside-producing Actinomycetales that methylate 16S rRNA at position A1408.60,61 However, again, BLASTP analysis did not identify any significant homology between these proteins and available N. gonorrhoeae sequences. Therefore, our analysis also indicates that, currently, A1408 ribosomal rRNA methylases, that would undermine apramycin activity, must be extremely rare or absent in N. gonorrhoeae. Furthermore, a search of the curated CARD Prevalence, Resistomes, & Variants database (Version 3.0.2) also did not identify apramycin resistance elements in the N. gonorrhoeae genomic sequences. Only a single kanamycin aminoglycoside-modifying enzyme, APH(3′)-Ia,62 was identified at very low prevalence (0.24%) in the N. gonorrhoeae sequences available in the NCBI database.
Several limitations of our study should be noted. First, spectinomycin does not achieve sufficient pharyngeal levels for effective treatment of gonococcal pharyngitis,25 although cure of pharyngeal infection with gentamicin used in combination with azithromycin appears to occur.21 Based on these observations, it is possible that apramycin, also a highly hydrophilic aminocyclitol, may have similar limitations. Second, isolates with reduced susceptibility to gentamicin, observed in regions where gentamicin is used for primary treatment,19,22 were not available to us. It is possible such reduced susceptibility, potentially based on decreased bacterial permeability or acquisition of efflux pumps, could be the basis for cross-resistance to apramycin, an issue that warrants further study. Furthermore, it is not yet established with what frequency spontaneous apramycin resistance would arise under direct selective pressure.
Taken together, the lack of acquired resistance (i.e. strains with MIC values above the ECOFF and genetic evidence for resistance elements), rapid bactericidal activity and putative lack of typical aminoglycoside-associated toxicities24 highlight the potential of apramycin, either directly and/or after derivatization, for development as an alternative treatment of MDR N. gonorrhoeae. However, further experimental and human pharmacokinetic and pharmacodynamic studies are needed to determine whether efficacious drug levels can be obtained at sites of infection and whether compelling dosing strategies, such as single, high-dose administration for cure, can be established.
Acknowledgements
We thank Dr Elizabeth Torrone and the CDC Neisseria Reference Laboratory for the permission to include a select number of N. gonorrhoeae isolates, originally obtained through the CDC’s Gonococcal Isolate Surveillance Project.
Funding
This work was supported by a Chief Academic Officer’s Pilot Grant from Beth Israel Deaconess Medical Center and by the National Institute of Allergy and Infectious Diseases of the National Institutes of Health under R21 AI142040-01 to J. E. K. A. D. K. was supported by the Long Term Health Education and Training program from the United States Army as an American Society for Microbiology Committee on Postgraduate Educational Programs Fellow at Beth Israel Deaconess Medical Center. K. P. S. was supported by the National Institute of Allergy and Infectious Diseases of the National Institutes of Health under award number F32 AI124590. During this course of this work, J. E. K. also received ongoing support from the National Institute of Allergy and Infectious Diseases of the National Institutes of Health under award numbers R33AI119114, R01 AI099122, and R21 AI130434, and a Harvard Catalyst Pilot Grant.
Transparency declarations
None to declare.
Disclaimer
The content is solely the responsibility of the authors and does not necessarily represent the official views of the National Institutes of Health, United States Army, or Department of Defense.
References
WHO Department of Reproductive Health and Research. Global Action Plan to Control the Spread and Impact of Antimicrobial Resistance in Neisseria gonorrhoeae. http://apps.who.int/iris/bitstream/10665/44863/1/9789241503501_eng.pdf.
Centers for Disease Control and Prevention. Gonococcal Isolate Surveillance Program (GISP). https://www.cdc.gov/std/gisp/GISP-Protocol-May-2016.pdf.
Clinical and Laboratory Standards Institute.
National Committee for Clinical Laboratory Standards.