-
PDF
- Split View
-
Views
-
Cite
Cite
Gloria Wong, Scott Briscoe, Brett McWhinney, Mumtaz Ally, Jacobus Ungerer, Jeffrey Lipman, Jason A Roberts, Therapeutic drug monitoring of β-lactam antibiotics in the critically ill: direct measurement of unbound drug concentrations to achieve appropriate drug exposures, Journal of Antimicrobial Chemotherapy, Volume 73, Issue 11, November 2018, Pages 3087–3094, https://doi.org/10.1093/jac/dky314
- Share Icon Share
Abstract
To describe the achievement of unbound β-lactam antibiotic concentration targets in a therapeutic drug monitoring (TDM) programme in critically ill patients, and the factors associated with failure to achieve a target concentration.
Plasma samples and clinical data were obtained for analysis from a single centre prospectively. Unbound concentrations of ceftriaxone, cefazolin, meropenem, ampicillin, benzylpenicillin, flucloxacillin and piperacillin were directly measured using ultracentrifugation. Factors associated with the achievement of pharmacokinetic/pharmacodynamic (PK/PD) targets or negative clinical outcomes were evaluated with binomial logistic regression.
TDM data from 330 patients, and 369 infection episodes, were included. The range of doses administered was 99.4% ± 45.1% relative to a standard daily dose. Dose increases were indicated in 33.1% and 63.4% of cases to achieve PK/PD targets of 100% fT>MIC and 100% fT>4×MIC, respectively. Dose reduction was indicated in 17.3% of cases for an upper PK/PD threshold of 100% fT>10×MIC. Higher protein bound β-lactams (ceftriaxone and benzylpenicillin) had better therapeutic target attainment (P < 0.01), but were prone to excessive dosing. Augmented renal clearance (calculated CLCR >130 mL/min) increased the odds of failure to achieve 100% fT>MIC and 100% fT>4×MIC (OR 2.47 and 3.05, respectively; P < 0.01).
Measuring unbound concentrations of β-lactams as part of a routine TDM programme is feasible and demonstrates that a large number of critically ill patients do not achieve predefined PK/PD targets. The clinical significance of this finding is unknown due to the lack of correlation between PK/PD findings and clinical outcomes.
Introduction
Mortality and morbidity associated with severe infections remain high among the critically ill despite advances in modern medicine.1,2 Early and targeted antibiotic treatment is the cornerstone of managing infections in critically ill patients, particularly those with sepsis.3 However, achieving adequate antibiotic exposure in the critically ill through using conventional dosing regimens is challenging. Significantly altered β-lactam antibiotic pharmacokinetics (PK) in the critically ill can result from patients’ dynamic and deranged pathophysiology such as altered fluid balance and augmented renal clearance (ARC).4–6 On the other hand, patients with organ dysfunction and associated impaired drug clearance are prone to inappropriately high drug exposures and possible drug toxicities.7–9 Thus, standard antibiotic dosing regimens derived from healthy volunteers and non-critically ill patients may not be appropriate. Strategies that aim to optimize antibiotic exposure using PK/pharmacodynamic (PK/PD) data have been proposed to improve clinical outcomes in infected critically ill patients.9–12
β-Lactams are the most frequently used antibiotics for treatment of severe infections in the critically ill. Efficacy of β-lactams depends on the percentage of the dosing period that the unbound concentration of the antibiotic is maintained above the MIC of targeted pathogen (%fT>MIC). Early in vitro and animal in vivo studies demonstrated that 40%–70% fT>MIC is associated with bactericidal effect,13,14 while clinical data suggest a higher threshold of 50% fT>MIC up to 100% fT>4×MIC is associated with positive clinical outcomes.15 In view of the extremely deranged and unpredictable β-lactam PK in the critically ill, therapeutic drug monitoring (TDM) has been proposed as a strategy to optimize %fT>MIC and to improve potentially the clinical outcomes. However, currently available TDM reports have not directly accounted for altered protein binding, and the associated change in unbound β-lactam concentrations,16 despite the high prevalence of hypoalbuminaemia causing altered protein binding in the critically ill.17 Given that efficacy is dependent on the unbound rather than total plasma concentration of antibiotic, knowledge of unbound concentrations when applying TDM should be considered essential.
The primary aim of this study was to describe the achievement of unbound β-lactam antibiotic concentration targets in a TDM programme in critically ill patients. Furthermore, we sought to describe the factors associated with target achievement. The secondary aims were to identify factors associated with failure to achieving PK/PD targets and negative clinical outcomes.
Patients and methods
Patient selection
This prospective observational study was conducted as part of a β-lactam TDM programme in critically ill patients at a 27 bed tertiary referral ICU. β-Lactam TDM is provided as a part of routine clinical care in this unit. Approval to collect these data was granted by the local institutional review board (Royal Brisbane and Women’s Hospital, Human Research Ethics Committee). Data were collected over the period between 1 January 2012 and 19 December 2013.
Ten antibiotics are included in the routine TDM service: ampicillin, benzylpenicillin, dicloxacillin, flucloxacillin, piperacillin (co-administered with tazobactam), ceftriaxone, cefalotin, cefazolin, meropenem and ertapenem. Patients were eligible for inclusion in this analysis if they were ≥18 years old, receiving one of the selected study antibiotic(s), would have received at least four doses of antibiotic by the time of sampling and were expected to remain on the treatment for the next 24 h.
Demographic and clinical data were collected to describe the patient sample including age, sex, weight, serum albumin and creatinine concentrations, indications for antibiotic treatment, antibiotic dosing data (including dose, administration frequency, duration of infusion, dosing time and duration of antibiotic therapy), admission diagnosis and clinical outcome of infection treatment. Microbiological data collected include identity of cultured bacteria and its corresponding MIC, if available. CLCR on the sampling day was estimated from serum creatinine concentrations using the Cockcroft–Gault equation.18
Initial dose selection and dose adjustment strategy
The treating physician in consultation with the clinical pharmacist determined the empirical dosing regimen and performed subsequent dose adjustment. Empirical doses were prescribed based on the patients’ clinical condition (including body size, haemodynamic status, renal and hepatic function) and known or likely pathogen. Loading doses were given when continuous infusion was used. Subsequent dose adjustment was made according to the approach described in Table 1.
Unbound concentration . | Dose adjustment . |
---|---|
<100% fT>MIC | 25%–50% increased frequency of same dose; change of administration to extended infusion (infused over one-half of the dosing interval) if TDM concentration within 20% of target concentration; or change to continuous infusion (same day dose) when intermittent dosing at maximum dose in line with the antibiotic’s product information |
>100% fT>10×MIC | 50% decreased dose with same dosing frequency; or 25%–50% decreased frequency with same dose |
Unbound concentration . | Dose adjustment . |
---|---|
<100% fT>MIC | 25%–50% increased frequency of same dose; change of administration to extended infusion (infused over one-half of the dosing interval) if TDM concentration within 20% of target concentration; or change to continuous infusion (same day dose) when intermittent dosing at maximum dose in line with the antibiotic’s product information |
>100% fT>10×MIC | 50% decreased dose with same dosing frequency; or 25%–50% decreased frequency with same dose |
%fT>N×MIC, percentage of dosing period where unbound concentration of β-lactam antibiotic is above N times the MIC for respective β-lactam antibiotics.
Unbound concentration . | Dose adjustment . |
---|---|
<100% fT>MIC | 25%–50% increased frequency of same dose; change of administration to extended infusion (infused over one-half of the dosing interval) if TDM concentration within 20% of target concentration; or change to continuous infusion (same day dose) when intermittent dosing at maximum dose in line with the antibiotic’s product information |
>100% fT>10×MIC | 50% decreased dose with same dosing frequency; or 25%–50% decreased frequency with same dose |
Unbound concentration . | Dose adjustment . |
---|---|
<100% fT>MIC | 25%–50% increased frequency of same dose; change of administration to extended infusion (infused over one-half of the dosing interval) if TDM concentration within 20% of target concentration; or change to continuous infusion (same day dose) when intermittent dosing at maximum dose in line with the antibiotic’s product information |
>100% fT>10×MIC | 50% decreased dose with same dosing frequency; or 25%–50% decreased frequency with same dose |
%fT>N×MIC, percentage of dosing period where unbound concentration of β-lactam antibiotic is above N times the MIC for respective β-lactam antibiotics.
Sampling
As per the TDM protocol, blood samples were obtained at assumed PK ‘steady-state’, defined as sampling after administration of at least four prior doses. For intermittent dosing, two plasma samples were taken, first at the mid-point of the dosing interval and secondly, immediately prior to re-dosing (trough concentration). For continuous infusion, plasma samples were taken after at least four half-lives. Patients treated with more than one study antibiotic were eligible to provide more than one set of blood samples on the same day.
β-Lactam assay
Plasma unbound concentration of β-lactams were measured directly using a validated and previously published HPLC assay.19 Briefly, plasma samples were filtered by an Amicon Ultra-0.5 mL 30 000 molecular weight cut-off centrifugal filter device. The ultrafiltrate were mixed with MES buffer (pH 6.6) and analysed using HPLC. The concentration ranges of the standard curves were 0.1–50 mg/L for all antibiotics (except piperacillin 0.1–100 mg/L). The coefficients of variation for inter-assay and intra-assay precision were <10%, and the accuracy was within 10% for all antibiotics.
β-Lactam TDM service
The TDM service was provided two to four times weekly by the in-house pathology laboratory. Typically results were available within 6 h (maximally within 24 h) when samples were taken on the morning of a scheduled service day.
PK/PD targets for dose adjustment
The PK/PD target of 100% fT>MIC was chosen for dose adjustment in this study. The upper limit of 100% fT>10×MIC was arbitrarily selected for dose reduction as indicative of an exposure beyond which no therapeutic value is likely, but for which there may be an increased possibility of toxicity. The EUCAST clinical breakpoint specific for the targeted (or suspected) bacteria was used (http://www.eucast.org/clinical_breakpoints) when a measured MIC was not available. The highest MIC for a suspected bacterium susceptible to the antibiotic was used in cases where no pathogen was cultured (e.g. 16 mg/L for anti-pseudomonal activity for piperacillin/tazobactam).
Clinical endpoints definitions
Clinical outcomes are defined in Table 2. Data were extracted from patients’ medical notes where clinical progress was documented by independent treating physicians.
Clinical response . | Definition . |
---|---|
Positive clinical outcome | Resolution (disappearance of all signs and symptoms related to the infection) or improvement (marked or moderate reduction in the severity and/or number of signs and symptoms of infection) clinically as documented by independent clinicians in patients’ progress notes. |
Negative clinical outcome | Any outcome other than positive outcomes, which includes insufficient lessening of the signs and symptoms of infection to qualify as improvement, including death or indeterminate (no evaluation possible, for any reason). |
Clinical response . | Definition . |
---|---|
Positive clinical outcome | Resolution (disappearance of all signs and symptoms related to the infection) or improvement (marked or moderate reduction in the severity and/or number of signs and symptoms of infection) clinically as documented by independent clinicians in patients’ progress notes. |
Negative clinical outcome | Any outcome other than positive outcomes, which includes insufficient lessening of the signs and symptoms of infection to qualify as improvement, including death or indeterminate (no evaluation possible, for any reason). |
Clinical response . | Definition . |
---|---|
Positive clinical outcome | Resolution (disappearance of all signs and symptoms related to the infection) or improvement (marked or moderate reduction in the severity and/or number of signs and symptoms of infection) clinically as documented by independent clinicians in patients’ progress notes. |
Negative clinical outcome | Any outcome other than positive outcomes, which includes insufficient lessening of the signs and symptoms of infection to qualify as improvement, including death or indeterminate (no evaluation possible, for any reason). |
Clinical response . | Definition . |
---|---|
Positive clinical outcome | Resolution (disappearance of all signs and symptoms related to the infection) or improvement (marked or moderate reduction in the severity and/or number of signs and symptoms of infection) clinically as documented by independent clinicians in patients’ progress notes. |
Negative clinical outcome | Any outcome other than positive outcomes, which includes insufficient lessening of the signs and symptoms of infection to qualify as improvement, including death or indeterminate (no evaluation possible, for any reason). |
Safety analysis
Data were extracted from patients’ medical notes where probable adverse drug reactions related to studies β-lactams were documented by independent treating physicians.
Statistics
All continuous data are reported as number (percentages), or medians (IQR) as appropriate. Achievement of various PK/PD targets [50% fT>MIC (most conservative), 50% fT>4×MIC, 100% fT>MIC and 100% fT>4×MIC] are reported as percentages. For patients with two or more TDM occasions during a single course of antibiotic therapy, achievement of PK/PD targets among first and subsequent TDM were compared using McNemar’s test. The association of patient demographic and clinical factors with achievement of PK/PD targets was evaluated with binomial logistic regression using SPSS (version 22.0; IBM Corp., Armonk, NY, USA) for all patients. Factors associated with negative clinical outcome were analysed for cases where respiratory, abdominal or bloodstream infections were being treated, and analysed relative to the outcome associated with bloodstream infections. Cases where there was more than one identified source of infection were not included in the analysis. ORs and 95% CIs were obtained. P ≤ 0.05 was considered significant.
Results
Three hundred and seventy-three patients were included in the study and the demographic and clinical characteristics of 330 evaluable patients (369 cases of infection) are shown in Table 3. Patients were typically male and with a high mean BMI (29.0 kg/m2). Serum albumin concentrations were consistently below the normal range on the day of sampling and renal function was highly variable, as described by serum creatinine concentrations. Sixty-eight patients (13.8%) were receiving continuous renal replacement therapy on the day of sampling. In 192 cases (39.1%), patients manifested ARC (calculated CLCR >130 mL/min). One hundred and three patients (31.2%) had TDM performed on more than one occasion. Four hundred and thirty-eight and 491 mid-dosing and trough samples were measured, respectively. MIC data were available for 12 culture-positive samples. Treatment was initiated for respiratory infection in the majority of cases (35.0%), followed by bloodstream and intra-abdominal infections (16.8% and 9.2%, respectively). In 10.3% of cases, more than one source of infection was identified. The dosing ranges for the prescribed antibiotics are shown in Figure 1. The range of doses administered was wide with an observed variation relative to a standard daily dose of 99.4% ± 45.1%. Among patients with manifested ARC, doses administered were above the standard daily dose (range 133.3%–400% of standard daily dose) in 53 cases (27.6%). None of the study participants received dicloxacillin, cefalotin or ertapenem during the data collection period.
Characteristic . | Value . |
---|---|
Males | 66% |
Age (years) | 53.4 ± 17.7 |
Serum albumin concentration (g/L)a | 24.2 ± 5.6 |
Serum creatinine concentration (μmol/L)a | 76 (53–129) |
Calculated CLCR (mL/min)b | 101.5 (59.1–163.0) |
Renal replacement therapy | 68 (13.8) |
BMI (kg/m2) | 29.0 ± 8.9 |
APACHE II score | 22 (16–27) |
Repeated sampling (2nd or subsequent) | 122 (24.8) |
Duration of β-lactam therapy (days) | 5 (3–7) |
Antibiotic administered as continuous infusion | 21 (4.3) |
Percentage of standard daily dose | 99.4% ± 45.1% |
Characteristic . | Value . |
---|---|
Males | 66% |
Age (years) | 53.4 ± 17.7 |
Serum albumin concentration (g/L)a | 24.2 ± 5.6 |
Serum creatinine concentration (μmol/L)a | 76 (53–129) |
Calculated CLCR (mL/min)b | 101.5 (59.1–163.0) |
Renal replacement therapy | 68 (13.8) |
BMI (kg/m2) | 29.0 ± 8.9 |
APACHE II score | 22 (16–27) |
Repeated sampling (2nd or subsequent) | 122 (24.8) |
Duration of β-lactam therapy (days) | 5 (3–7) |
Antibiotic administered as continuous infusion | 21 (4.3) |
Percentage of standard daily dose | 99.4% ± 45.1% |
Data shown are n (%), mean ± SD, or median (IQR).
Serum creatinine and serum albumin concentrations were measured on the day of sampling; other parameters were measured upon admission.
CLCR was estimated using the Cockcroft–Gault formula.
Characteristic . | Value . |
---|---|
Males | 66% |
Age (years) | 53.4 ± 17.7 |
Serum albumin concentration (g/L)a | 24.2 ± 5.6 |
Serum creatinine concentration (μmol/L)a | 76 (53–129) |
Calculated CLCR (mL/min)b | 101.5 (59.1–163.0) |
Renal replacement therapy | 68 (13.8) |
BMI (kg/m2) | 29.0 ± 8.9 |
APACHE II score | 22 (16–27) |
Repeated sampling (2nd or subsequent) | 122 (24.8) |
Duration of β-lactam therapy (days) | 5 (3–7) |
Antibiotic administered as continuous infusion | 21 (4.3) |
Percentage of standard daily dose | 99.4% ± 45.1% |
Characteristic . | Value . |
---|---|
Males | 66% |
Age (years) | 53.4 ± 17.7 |
Serum albumin concentration (g/L)a | 24.2 ± 5.6 |
Serum creatinine concentration (μmol/L)a | 76 (53–129) |
Calculated CLCR (mL/min)b | 101.5 (59.1–163.0) |
Renal replacement therapy | 68 (13.8) |
BMI (kg/m2) | 29.0 ± 8.9 |
APACHE II score | 22 (16–27) |
Repeated sampling (2nd or subsequent) | 122 (24.8) |
Duration of β-lactam therapy (days) | 5 (3–7) |
Antibiotic administered as continuous infusion | 21 (4.3) |
Percentage of standard daily dose | 99.4% ± 45.1% |
Data shown are n (%), mean ± SD, or median (IQR).
Serum creatinine and serum albumin concentrations were measured on the day of sampling; other parameters were measured upon admission.
CLCR was estimated using the Cockcroft–Gault formula.
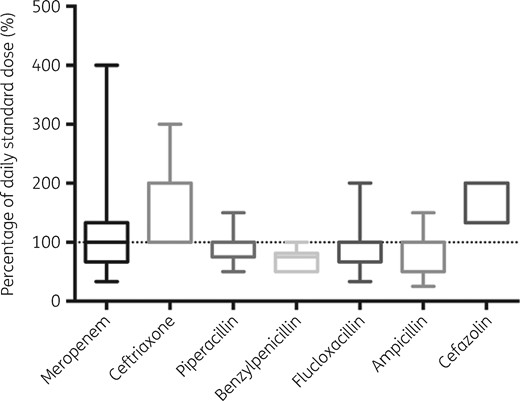
Range of β-lactam doses administered relative to standard daily dose (meropenem 1000 mg q8h; piperacillin/tazobactam 4500 mg q8h; ampicillin 2000 mg q6h; ceftriaxone 1000 mg q12h; cefazolin 1000 mg q8h; benzylpenicillin 2400 mg q4h; and flucloxacillin 2000 mg q4h).
The overall achievements of predefined PK/PD targets for dose increases are shown in Table 4 and Figure 2. The majority of cases (90.1%) achieved the conservative target of 50% fT>MIC, with the exception of ampicillin where attainment of this target was significantly lower (60.0%). Success in target achievement decreased considerably as the magnitude of the PK/PD target increased, with only 36.6% cases achieving the target of 100% fT>4×MIC. For cefazolin, no patients achieved this target. Among the tested β-lactams, benzylpenicillin and ceftriaxone achieved the upper target of 100% fT>4×MIC in 80.0% and 71.4% of the cases, respectively. On the other hand, there was high proportion of cases (80.0% and 49.3% among patients received benzylpenicillin and ceftriaxone, respectively) where dose reduction was required because of a high PK/PD index (100% fT>10×MIC).
Achievement of predefined PK/PD dose adjustment targets for first TDM of studied β-lactams
. | Percentage of episodes where predefined targets were reached (within each β-lactam group) . | Total . | ||||||
---|---|---|---|---|---|---|---|---|
ampicillin . | benzylpenicillin . | cefazolin . | ceftriaxone . | flucloxacillin . | meropenem . | piperacillin . | ||
Standard dose | 2000 mg q6h | 2400 mg q4h | 1000 mg q8h | 1000 mg q12h | 2000 mg q4h | 1000 mg q8h | 4500 mg q8h | |
Dosage range | 1000 mg q12h–2000 mg q4h | 1200 mg q4h–2400 mg q4h | 1000 mg q6h–2000 mg q8h | 1000 mg q12h–2000 mg q8h | 2000 mg q12h–2000 mg q2h | 500 mg q12h–2000 mg q6h | 4500 mg q12h–4500 mg q4h | |
50% fT>MIC | 60.0%a | 100.0% | 100.0% | 96.2% | 83.3% | 92.1% | 90.4% | 90.1% |
50% fT>4×MIC | 53.3% | 91.7%a | 28.6% | 96.2%a | 44.4% | 68.5% | 53.2% | 61.3% |
Total (n) | 15 | 15 | 7 | 26 | 18 | 89 | 156 | 323 |
100% fT>MIC | 53.3% | 93.3%a | 57.1% | 96.4%a | 52.0% | 72.2% | 61.0% | 66.9% |
100% fT>4×MIC | 33.3% | 80.0%a | 0.0% | 71.4%a | 32.0% | 29.9% | 33.5% | 36.6% |
100% fT>10×MIC | 13.3% | 80.0%a | 0.0% | 39.3% | 16.0% | 11.3% | 13.2% | 17.3% |
Total (n) | 15 | 15 | 7 | 28 | 25 | 97 | 182 | 369 |
. | Percentage of episodes where predefined targets were reached (within each β-lactam group) . | Total . | ||||||
---|---|---|---|---|---|---|---|---|
ampicillin . | benzylpenicillin . | cefazolin . | ceftriaxone . | flucloxacillin . | meropenem . | piperacillin . | ||
Standard dose | 2000 mg q6h | 2400 mg q4h | 1000 mg q8h | 1000 mg q12h | 2000 mg q4h | 1000 mg q8h | 4500 mg q8h | |
Dosage range | 1000 mg q12h–2000 mg q4h | 1200 mg q4h–2400 mg q4h | 1000 mg q6h–2000 mg q8h | 1000 mg q12h–2000 mg q8h | 2000 mg q12h–2000 mg q2h | 500 mg q12h–2000 mg q6h | 4500 mg q12h–4500 mg q4h | |
50% fT>MIC | 60.0%a | 100.0% | 100.0% | 96.2% | 83.3% | 92.1% | 90.4% | 90.1% |
50% fT>4×MIC | 53.3% | 91.7%a | 28.6% | 96.2%a | 44.4% | 68.5% | 53.2% | 61.3% |
Total (n) | 15 | 15 | 7 | 26 | 18 | 89 | 156 | 323 |
100% fT>MIC | 53.3% | 93.3%a | 57.1% | 96.4%a | 52.0% | 72.2% | 61.0% | 66.9% |
100% fT>4×MIC | 33.3% | 80.0%a | 0.0% | 71.4%a | 32.0% | 29.9% | 33.5% | 36.6% |
100% fT>10×MIC | 13.3% | 80.0%a | 0.0% | 39.3% | 16.0% | 11.3% | 13.2% | 17.3% |
Total (n) | 15 | 15 | 7 | 28 | 25 | 97 | 182 | 369 |
%fT>N×MIC, percentage of dosing period where unbound concentration of β-lactam antibiotic is above N times the MIC for the respective β-lactam antibiotics.
Percentage of episodes achieving corresponding PK/PD targets significantly different from other β-lactam antibiotics (P < 0.05).
Achievement of predefined PK/PD dose adjustment targets for first TDM of studied β-lactams
. | Percentage of episodes where predefined targets were reached (within each β-lactam group) . | Total . | ||||||
---|---|---|---|---|---|---|---|---|
ampicillin . | benzylpenicillin . | cefazolin . | ceftriaxone . | flucloxacillin . | meropenem . | piperacillin . | ||
Standard dose | 2000 mg q6h | 2400 mg q4h | 1000 mg q8h | 1000 mg q12h | 2000 mg q4h | 1000 mg q8h | 4500 mg q8h | |
Dosage range | 1000 mg q12h–2000 mg q4h | 1200 mg q4h–2400 mg q4h | 1000 mg q6h–2000 mg q8h | 1000 mg q12h–2000 mg q8h | 2000 mg q12h–2000 mg q2h | 500 mg q12h–2000 mg q6h | 4500 mg q12h–4500 mg q4h | |
50% fT>MIC | 60.0%a | 100.0% | 100.0% | 96.2% | 83.3% | 92.1% | 90.4% | 90.1% |
50% fT>4×MIC | 53.3% | 91.7%a | 28.6% | 96.2%a | 44.4% | 68.5% | 53.2% | 61.3% |
Total (n) | 15 | 15 | 7 | 26 | 18 | 89 | 156 | 323 |
100% fT>MIC | 53.3% | 93.3%a | 57.1% | 96.4%a | 52.0% | 72.2% | 61.0% | 66.9% |
100% fT>4×MIC | 33.3% | 80.0%a | 0.0% | 71.4%a | 32.0% | 29.9% | 33.5% | 36.6% |
100% fT>10×MIC | 13.3% | 80.0%a | 0.0% | 39.3% | 16.0% | 11.3% | 13.2% | 17.3% |
Total (n) | 15 | 15 | 7 | 28 | 25 | 97 | 182 | 369 |
. | Percentage of episodes where predefined targets were reached (within each β-lactam group) . | Total . | ||||||
---|---|---|---|---|---|---|---|---|
ampicillin . | benzylpenicillin . | cefazolin . | ceftriaxone . | flucloxacillin . | meropenem . | piperacillin . | ||
Standard dose | 2000 mg q6h | 2400 mg q4h | 1000 mg q8h | 1000 mg q12h | 2000 mg q4h | 1000 mg q8h | 4500 mg q8h | |
Dosage range | 1000 mg q12h–2000 mg q4h | 1200 mg q4h–2400 mg q4h | 1000 mg q6h–2000 mg q8h | 1000 mg q12h–2000 mg q8h | 2000 mg q12h–2000 mg q2h | 500 mg q12h–2000 mg q6h | 4500 mg q12h–4500 mg q4h | |
50% fT>MIC | 60.0%a | 100.0% | 100.0% | 96.2% | 83.3% | 92.1% | 90.4% | 90.1% |
50% fT>4×MIC | 53.3% | 91.7%a | 28.6% | 96.2%a | 44.4% | 68.5% | 53.2% | 61.3% |
Total (n) | 15 | 15 | 7 | 26 | 18 | 89 | 156 | 323 |
100% fT>MIC | 53.3% | 93.3%a | 57.1% | 96.4%a | 52.0% | 72.2% | 61.0% | 66.9% |
100% fT>4×MIC | 33.3% | 80.0%a | 0.0% | 71.4%a | 32.0% | 29.9% | 33.5% | 36.6% |
100% fT>10×MIC | 13.3% | 80.0%a | 0.0% | 39.3% | 16.0% | 11.3% | 13.2% | 17.3% |
Total (n) | 15 | 15 | 7 | 28 | 25 | 97 | 182 | 369 |
%fT>N×MIC, percentage of dosing period where unbound concentration of β-lactam antibiotic is above N times the MIC for the respective β-lactam antibiotics.
Percentage of episodes achieving corresponding PK/PD targets significantly different from other β-lactam antibiotics (P < 0.05).
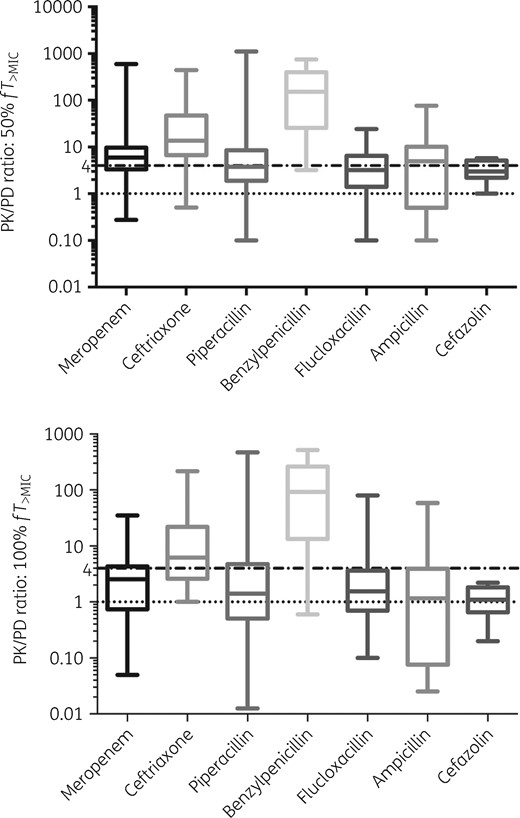
PK/PD ratios (as unbound concentrations divided by MICs) at 50% and 100% dosing intervals. Dotted lines denote predefined targets of fT>MIC and fT>4×MIC at respective dosing intervals.
Paired comparison of PK/PD targets achievement between first and subsequent TDM measurements were performed for patients with two or more TDM measurements (n = 84 at mid-dosing interval, n = 94 at trough). No significant difference was found in PK/PD ratios achieved and percentage of cases achieving the predefined PK/PD targets in first TDM as compared with subsequent TDM indicating that the dose adjustment schedule was not efficient (Table 5).
Achievement of PK/PD dose adjustment targets for first versus subsequent TDM for patients that had sampling on more than one occasion (n = 84 at mid-dosing interval, n = 94 at trough). PK/PD ratios and percentage of cases achieving the predefined PK/PD targets in first TDM as compared with subsequent TDM were not significantly different
Measure . | First TDM . | Subsequent TDM . |
---|---|---|
PK/PD ratio (at mid-dosing interval) | 4.0 (2.3–8.5) | 4.3 (2.3–8.5) |
PK/PD ratio (at trough) | 1.8 (0.6–4.7) | 1.6 (0.7–3.4) |
Achievement of PK/PD targets | ||
50% fT>MIC | ||
yes | 76 (90.5%) | 76 (90.5%) |
no | 8 (9.5%) | 8 (9.5%) |
100% fT>MIC | ||
yes | 59 (62.8%) | 61 (64.9%) |
no | 35 (37.2%) | 33 (35.1%) |
50% fT>4×MIC | ||
yes | 42 (49.4%) | 45 (52.9%) |
no | 43 (50.6%) | 40 (47.1%) |
100% fT>4×MIC | ||
yes | 25 (26.6%) | 21 (22.3%) |
no | 69 (73.4%) | 73 (77.7%) |
>100% fT>10×MIC | ||
yes | 11 (11.7%) | 8 (8.5%) |
no | 83 (88.3%) | 86 (91.5%) |
Measure . | First TDM . | Subsequent TDM . |
---|---|---|
PK/PD ratio (at mid-dosing interval) | 4.0 (2.3–8.5) | 4.3 (2.3–8.5) |
PK/PD ratio (at trough) | 1.8 (0.6–4.7) | 1.6 (0.7–3.4) |
Achievement of PK/PD targets | ||
50% fT>MIC | ||
yes | 76 (90.5%) | 76 (90.5%) |
no | 8 (9.5%) | 8 (9.5%) |
100% fT>MIC | ||
yes | 59 (62.8%) | 61 (64.9%) |
no | 35 (37.2%) | 33 (35.1%) |
50% fT>4×MIC | ||
yes | 42 (49.4%) | 45 (52.9%) |
no | 43 (50.6%) | 40 (47.1%) |
100% fT>4×MIC | ||
yes | 25 (26.6%) | 21 (22.3%) |
no | 69 (73.4%) | 73 (77.7%) |
>100% fT>10×MIC | ||
yes | 11 (11.7%) | 8 (8.5%) |
no | 83 (88.3%) | 86 (91.5%) |
Achievement of PK/PD dose adjustment targets for first versus subsequent TDM for patients that had sampling on more than one occasion (n = 84 at mid-dosing interval, n = 94 at trough). PK/PD ratios and percentage of cases achieving the predefined PK/PD targets in first TDM as compared with subsequent TDM were not significantly different
Measure . | First TDM . | Subsequent TDM . |
---|---|---|
PK/PD ratio (at mid-dosing interval) | 4.0 (2.3–8.5) | 4.3 (2.3–8.5) |
PK/PD ratio (at trough) | 1.8 (0.6–4.7) | 1.6 (0.7–3.4) |
Achievement of PK/PD targets | ||
50% fT>MIC | ||
yes | 76 (90.5%) | 76 (90.5%) |
no | 8 (9.5%) | 8 (9.5%) |
100% fT>MIC | ||
yes | 59 (62.8%) | 61 (64.9%) |
no | 35 (37.2%) | 33 (35.1%) |
50% fT>4×MIC | ||
yes | 42 (49.4%) | 45 (52.9%) |
no | 43 (50.6%) | 40 (47.1%) |
100% fT>4×MIC | ||
yes | 25 (26.6%) | 21 (22.3%) |
no | 69 (73.4%) | 73 (77.7%) |
>100% fT>10×MIC | ||
yes | 11 (11.7%) | 8 (8.5%) |
no | 83 (88.3%) | 86 (91.5%) |
Measure . | First TDM . | Subsequent TDM . |
---|---|---|
PK/PD ratio (at mid-dosing interval) | 4.0 (2.3–8.5) | 4.3 (2.3–8.5) |
PK/PD ratio (at trough) | 1.8 (0.6–4.7) | 1.6 (0.7–3.4) |
Achievement of PK/PD targets | ||
50% fT>MIC | ||
yes | 76 (90.5%) | 76 (90.5%) |
no | 8 (9.5%) | 8 (9.5%) |
100% fT>MIC | ||
yes | 59 (62.8%) | 61 (64.9%) |
no | 35 (37.2%) | 33 (35.1%) |
50% fT>4×MIC | ||
yes | 42 (49.4%) | 45 (52.9%) |
no | 43 (50.6%) | 40 (47.1%) |
100% fT>4×MIC | ||
yes | 25 (26.6%) | 21 (22.3%) |
no | 69 (73.4%) | 73 (77.7%) |
>100% fT>10×MIC | ||
yes | 11 (11.7%) | 8 (8.5%) |
no | 83 (88.3%) | 86 (91.5%) |
Factors that predicted a failure to achieve the various unbound PK/PD targets (for first TDM) were explored. ARC (calculated CLCR >130 mL/min) was significantly associated with not achieving all predefined PK/PD targets (OR 2.47–3.33, P < 0.05). Administration by prolonged infusion (continuous or extended infusion) was associated with a decreased likelihood of achieving 100% fT>MIC [OR 0.28 (95% CI 0.09–0.86); P = 0.026]. The type of β-lactam used was also significantly associated with the successful achievement of all PK/PD targets except 50% fT>MIC (P < 0.01).
Overall, dose reduction was indicated in 17.3% of cases. Excessive drug exposure was associated with impaired renal clearance for a calculated CLCR <50 mL/min [OR 9.12 (95% CI 3.05–27.25); P < 0.01] and calculated CLCR 51–90 mL/min [OR 3.21 (95% CI 1.10–9.41); P = 0.03].
Two hundred and seventy-five cases (71.2%) had a positive clinical outcome. There were 41 deaths among the studied patients. The percentage of positive clinical outcome in cases where respiratory, abdominal or bloodstream infections were being treated (73.6%, n = 220) was comparable to the overall studied population (71.2%). An abdominal source of infection significantly increased the odds of negative clinical outcome [OR 7.60 (95% CI 2.39–24.17); P = 0.001]. Overall, failure to achieve PK/PD targets was not found to be independently associated with negative clinical outcomes in this patient sample with positive microbiological cultures [OR 0.88 (95% CI 0.40–1.91); P = 0.74, OR 0.67 (95% CI 0.29–1.55); P = 0.35, for 100% fT>MIC and 100% fT>4×MIC, respectively].
Adverse effects deemed causally related to β-lactam antibiotic therapy were reported in five cases. Neurotoxicity and nephrotoxicity were reported in one patient where trough free benzylpenicillin concentration reached 31 mg/L (beyond 10 times of the targeted MIC). Mild hepatotoxicity was reported in the other four cases, where none of them reached the predefined target for dose reduction.
Discussion
This study has uniquely evaluated achievement of PK/PD targets of directly measured unbound antibiotic concentrations for a large number of β-lactam antibiotics, which, to the best of our knowledge, has not been previously reported. Hypoalbuminaemia in the critically ill is common, and a previous study demonstrated poor predictive performance of calculated unbound drug concentration using conventional method.16 Given the variability of unbound drug fraction in the setting of hypoalbuminaemia, calculation of the unbound β-lactam antibiotics concentration using published protein binding data tends to underestimate the true unbound concentration of highly protein bound β-lactams, while these overestimate the unbound concentrations of some important β-lactams such as meropenem and piperacillin/tazobactam. Using a simple HPLC/UV-based method in the clinical setting, we aim to characterize better the β-lactam antibiotic exposures in the critically ill. We found that for the majority of tested β-lactams, attainment of PK/PD targets was suboptimal, with the exception of drugs with relatively high protein binding, benzylpenicillin and ceftriaxone. Even with the predefined empirical dose adjustment strategy, target attainment at subsequent TDM measurements was not high, although it appeared to reduce the odds of excessive dosing.
Our study echoes the concerns raised in other β-lactam PK/PD studies in the critically ill where significant numbers of patients did not achieve empirical PK/PD targets.10,20 Despite the fact that a large proportion of our studied population received β-lactams at a non-standard dose that was adjusted according to their clinical condition as shown in Table 4, almost half of the cases did not achieve 100% fT>MIC. Achievement of 50% fT>MIC was significantly lower for ampicillin compared with the other tested β-lactams suggesting that empirical dosing for this drug requires urgent revision. Interestingly, administration by prolonged infusion (continuous or extended infusion) was associated with a decreased likelihood of achieving 100% fT>MIC. This finding is unexpected, given the theoretical advantage of continuous infusion over short infusion in attaining PK/PD targets for β-lactams, as previously demonstrated in critically ill patients with severe sepsis21 and in those subjected to continuous renal replacement therapy.22,23 However, using a population PK model, Dhaese et al.24 demonstrated even a high-dose continuous infusion regimen (4 g loading dose followed by continuous infusion of 24 g/24 h) was inadequate to achieve a targeted exposure of 100% fT>4×MIC against susceptible Pseudomonas aeruginosa isolates (MIC ≤16 mg/L) in critically ill patients with normal renal clearance or ARC (>90 mL/min/1.73 m2). Similar analysis for unbound flucloxacillin also showed continuous infusion at a higher than standard dose (12 g/24 h) is needed to achieve a more aggressive PK/PD target.25 Our observations reflect the difficulty in determining dosing regimen in this group of patients, where high renal clearance was anticipated and continuous infusion was used pre-emptively. Relatively highly protein bound β-lactams such as ceftriaxone (83%–95% protein bound) and benzylpenicillin (65%) had better PK/PD target attainment. This may be explained by the higher unbound fraction of drug in the setting of hypoalbuminaemia in the critically ill, in combination with relatively lower MIC targets for these antibiotics (e.g. Streptococcus pneumoniae 0.125 mg/L for both antibiotics). Interestingly, the same results were not observed for flucloxacillin, which is also highly protein bound (95%).
Although it is important to maintain adequate β-lactam exposure when treating the critically ill, the risk of unnecessarily excessive drug exposure should not be overlooked. In this study, dose reduction was indicated in 17.3% of cases (first TDM) when trough concentrations reached >10 times the targeted MIC. Although there is limited evidence that this target is an indicator of toxicity, it was chosen for dose reduction arbitrarily in this study, based on the likelihood of extra therapeutic value beyond that level is limited. Patients administered the relatively highly protein bound benzylpenicillin (65%) or ceftriaxone (83%–95%) and/or patients with significantly impaired renal function (CLCR <50 mL/min) were most susceptible to overexposure of antibiotics but those with relatively normal renal function (CLCR 51–90 mL/min) were also at risk. β-Lactams are considered generally safe at high doses and have a wide therapeutic window. However, neurological adverse events associated with cephalosporins and carbapenems in particular have been widely reported in critically ill patients and those with renal impairment in general.7,8,26 Clinicians should be vigilant about the potential risk of excessive β-lactam concentrations. Indeed, fluctuations of patient’s renal function should prompt closer monitoring of drug concentrations and associated hidden complications such as encephalopathy and non-convulsive epilepsy. Practically, assays that directly measure unbound β-lactam should be used, particularly for highly protein bound antibiotics. In the setting of hypoalbuminaemia in the critically ill, there is a risk of underestimating unbound antibiotic concentrations when correcting total measured concentrations with published protein-binding data.16 This can lead to inappropriate overexposure of drug and potential dose-related adverse events.
Not only were the observed PK/PD ratios from the first TDM measurement highly variable, achieving the desirable level of drug exposure in subsequent TDM appeared difficult. Paired comparison of first and subsequent TDM measurements in patients who had more than one TDM did not show any significant improvement in the percentage of cases achieving the predefined PK/PD targets. These observations were likely secondary to intra-subject variability of PK throughout the antibiotic course27 and the less precise dose adjustment strategy we adopted in this study. On the other hand, excessive β-lactam dosing (as measured by upper dose reduction threshold of 100% fT>10×MIC) reduced with subsequent TDM. Nevertheless, given only a small percentage of our cohort had a second TDM performed, the value of TDM in improving target attainment might not be reflected here. Better re-dosing strategies are needed in future studies to optimize the potential benefit β-lactam TDM could offer.28–30
The secondary aim of this study was to describe factors associated with failure to achieve PK/PD targets. ARC (calculated CLCR >130 mL/min) was found to be the strongest predicting factor for subtherapeutic β-lactam exposure in our cohort; despite the fact that the empirical dosing regimen had already been adjusted for a substantial proportion of patients with suspected ARC. The underlying mechanism of ARC in the critically ill is unclear; however, there is emerging evidence that shows high prevalence of the phenomenon and its association with subthreshold β-lactam concentrations in the severely ill.6,31,32 In an observational study by Huttner et al.,31 in which the prevalence of ARC and subtherapeutic β-lactam concentrations in 100 critically ill patients treated with imipenem, meropenem, piperacillin/tazobactam or cefepime were evaluated, ARC was significantly associated with undetectable trough β-lactam concentrations (OR = 3.3). Although we were unable to confirm the association of ARC with negative clinical outcome in this study, and the correlation of ARC and outcome is still yet to be clarified,33 adjusted β-lactam dosage and utilization of TDM is probably indicated in this group of patients who are theoretically at higher risk of clinical failure secondary to suboptimal antibiotic exposure.
The association of clinical factors, in particular achievement of predefined PK/PD targets, with clinical failure were also explored in this analysis. In the DALI study, a large multinational β-lactam point-prevalence PK study, increasing 50% fT>MIC and 100% fT>MIC ratios were associated with increased odds of positive clinical outcomes, but the effect was shown to be more significant in those with lower disease severity as measured by APACHE II score (of 0–14).20 Our patient population, however, falls into the more critically ill category with a median APACHE II score of 22 (IQR = 16–27). Whether this explains the lack of association found between respective predefined PK/PD targets and clinical outcome in this analysis, and the appropriate PK/PD target for this group of more critically ill patients would need to be clarified.
Another notable finding was the significantly increased odds in negative clinical outcome associated with abdominal infection. In a recent large multicentre study, abdominal infection was found to be associated with a significantly higher mortality as compared with other types of infection.34 Deranged PK of β-lactams have been noted in patients with intra-abdominal infections;35 however, information on optimizing β-lactam PK/PD in this subgroup of patients was very limited. Only 4 of 41 deaths (9.8%) in this study were of intra-abdominal cause, yet our observation of increased treatment failure risk warrants further research to individualize better the antibiotic dosing in this group of patients.
Limitations of the study
First, this is a single-centre study in which the wider generalizability of the results might be limited. However, we have reported data from a relatively large cohort of patients enrolled with very broad inclusion criteria, and to the best of our knowledge this is the largest dataset of directly measured unbound concentrations’ data of the studied drugs. Second, use of data from only selected indications for therapy (respiratory, abdominal and bloodstream infections) for defining associations between PK/PD target attainment and outcome may not be optimal for application to other indications. However, no significant association was found either for patients treated for other indications or for the overall studied population (results not presented). Third, CLCR to describe renal function was estimated using the Cockcroft–Gault formula, which can underestimate actual CLCR in the critically ill population particularly for those with ARC.36,37 However, estimation of renal function using measured CLCR has its own practical issues in terms of urine sample collection and accessibility of the test. Our findings highlight the risk of under-dosing in patients with calculated ARC, which is practically relevant in clinical practice where only estimated CLCR is available. In addition, the dose adjustment strategy used in this study appears to have been suboptimal and may have contributed to poor PK/PD target attainment with subsequent TDM measurements, which could be improved with the use of dosing software based on Bayesian forecasting.38 The PK/PD targets were selected based on preclinical data and limited number of clinical studies as discussed above. To date, there is still no validated unifying PK/PD target for β-lactams in the critically ill population; however, the PK/PD targets selected for this analysis, cover the most conservative to most aggressive antibiotic exposures likely necessary for adequate clinical response. Our results reinforce the suboptimal antibiotic exposure observed across these target ranges. The clinical significance of this finding needs to be delineated further, as this study was not specifically designed, and therefore might not be adequately powered to detect difference in outcome of patients who did or did not attain PK/PD targets. Furthermore, we did not take into account the synergistic effect of other co-administered antibiotics in this study, which potentially introduce bias in our analysis. Lastly, in the majority of the cases, measured MICs of the targeted pathogens were not available, or culture was negative. Therefore, the PK/PD ratios and associated results presented represent the worst-case scenario where the presence of the least susceptible pathogen was assumed. Yet, this assumption is acceptable and closely resembles clinical practice, where most MIC data are not available. The use of EUCAST clinical breakpoints, which were derived to predict likelihood of therapeutic success, is clinically relevant and appropriate in practice. Nevertheless, this highlights the importance of developing methods that allow timely determination and application of MIC data in clinical settings in the future.39,40
In conclusion, our study indicates that optimal exposure of unbound β-lactams, in particular, for drugs with lower protein binding, is not achieved in a significant proportion of critically ill patients using conventional or empirically adjusted dosing regimens. Dosing strategies need to be redefined, particularly for patients with ARC and intra-abdominal infections, who are at risk of subtherapeutic exposure of β-lactams and negative clinical outcome respectively. Further clinical studies are needed to define better re-dosing methods as well as the implications of achieving targeted β-lactam exposure with TDM on clinical outcomes.
Acknowledgements
J. A. R. received salary funding from the National Health and Medical Research Council of Australia; Practitioner Fellowship (APP1117065). We wish to acknowledge institutional and salary funding from the Australian National Health and Medical Research Council for Centre of Research Excellence (APP1099452).
Funding
This study was carried out as part of our routine clinical work.
Transparency declarations
None to declare.