-
PDF
- Split View
-
Views
-
Cite
Cite
K. Kletenkov, D. Hoffmann, J. Böni, S. Yerly, V. Aubert, F. Schöni-Affolter, D. Struck, J. Verheyen, T. Klimkait, on behalf of the Swiss HIV Cohort Study, Role of Gag mutations in PI resistance in the Swiss HIV cohort study: bystanders or contributors?, Journal of Antimicrobial Chemotherapy, Volume 72, Issue 3, March 2017, Pages 866–875, https://doi.org/10.1093/jac/dkw493
- Share Icon Share
Background: HIV Gag mutations have been reported to confer PI drug resistance. However, clinical implications are still controversial and most current genotyping algorithms consider solely the protease gene for assessing PI resistance.
Objectives: Our goal was to describe for HIV infections in Switzerland the potential role of the C-terminus of Gag (NC–p6) in PI resistance. We aimed to characterize resistance-relevant mutational patterns in Gag and protease and their possible interactions.
Methods: Resistance information on plasma samples from 2004–12 was collected for patients treated by two diagnostic centres of the Swiss HIV Cohort Study. Sequence information on protease and the C-terminal Gag region was paired with the corresponding patient treatment history. The prevalence of Gag and protease mutations was analysed for PI treatment-experienced patients versus PI treatment-naive patients. In addition, we modelled multiple paths of an assumed ordered accumulation of genetic changes using random tree mixture models.
Results: More than half of all PI treatment-experienced patients in our sample set carried HIV variants with at least one of the known Gag mutations, and 17.9% (66/369) carried at least one Gag mutation for which a phenotypic proof of PI resistance by in vitro mutagenesis has been reported. We were able to identify several novel Gag mutations that are associated with PI exposure and therapy failure.
Conclusions: Our analysis confirmed the association of Gag mutations, well known and new, with PI exposure. This could have clinical implications, since the level of potential PI drug resistance might be underestimated.
Introduction
Although the main molecular features of HIV pathogenesis seem well understood,1–3 mechanisms of viral escape continue to be the subject of intense research and might even become more challenging in increasingly long-term therapy-experienced populations, where treatment histories may cover several decades and multiple drug classes.4–6 PIs, as an example, initially used as part of second-line regimens, have also widely been used in first-line regimens in recent years.7,8 Although in the Swiss HIV Cohort Study (SHCS) most patients with antiretroviral therapy have suppressed viral loads,9 one out of three patients has been affected by HIV drug resistances over time.10 Along with poor therapy adherence, the development of resistance-associated HIV mutations represents the primary cause of treatment failure.3,11,12
Mechanistically, resistance of HIV enzymes to targeting drugs is based on mutations in the respective gene, leading to structural alterations in the viral drug target.13–15 Despite this seemingly strict correspondence, cases of clinical failure under PI treatment have been reported in which genetic changes in the protease (PR) gene fail to provide a sufficient explanation.16,17 In addition to amino acid changes in the viral enzyme, Gag mutations have been associated with PI drug resistance by various methods, such as statistical analysis,18–20 examination of patient-derived samples after PI exposure and failure,20–26 or directly in phenotypic HIV assays in vitro.27–30 However, most current genotyping algorithms for PI failure still consider solely the PR gene for predicting HIV drug resistance.
This study set out to further investigate the potential role of the C-terminal region of Gag (NC–p6) in PI resistance and to characterize resistance-relevant mutational patterns in Gag-PR.
Methods
Study population
The SHCS is a large, prospective, nationwide, clinic-based cohort study enrolling patients prospectively since 1988. As of December 2014, a total of 18 850 persons have been enrolled in the SHCS. Including at least 53% of all HIV cases ever diagnosed in Switzerland, 72% of all patients receiving ART and 69% of the nationwide registered AIDS cases, it is very representative of HIV epidemiology in Switzerland. The largest proportion of patients was recruited in Swiss University Hospital infectious disease outpatient clinics in Zurich (37.3%). The centres of Lausanne, Geneva and Berne each contribute about 15% of patients and Basel 10%.31,32
Resistance information for plasma samples was collected between 2004 and 2012 from patients in two centres of the SHCS: Basel (n = 2022) and Zurich (n = 773).
Sequences and treatment information
The ViroSeq HIV-1 Genotyping System (Abbott Molecular, IL, USA) was used for Sanger sequencing of the HIV-1 pol region in the routine diagnostics setting of an accredited laboratory. Only the main virus population in each analysis (>70% of the viral pool) was considered in this study. The pol sequences were assembled and edited using the ViroSeq Genotyping software (Abbott Molecular). Details of the sequencing procedure have been described elsewhere.33 As a variable performance of primers of the ViroSeq system had to be anticipated for the various HIV-1 clades, which could lead to biased results, the entire study was limited to the analysis of subtype B viruses.
The F-primer, an integral part of the system, produces a read that extends in reverse orientation from PR into the C-terminal Gag region. Although not accessible with the standard ViroSeq software, this information was manually extracted from the raw sequencing data in the form of .ab1 files and analysed to obtain available Gag C-terminal sequences of NC to p6, which are disregarded in the standard setting. The corresponding Gag region spans from bases 790 to 2292 in the reference HXB2 genome. Alignment and amino acid translation were performed with RegaDB Sequence Analysis Tools34,35 and the Stanford HIVdb Program.36,37 We used the list and definitions of the Stanford HIV resistance database (HIVdb Program, Genotypic Resistance Interpretation Algorithm Version 7.0; 02/27/14) for major and minor PI resistance mutations in the PR gene.36,37
Sequence information on PR and the C-terminal Gag region (NC–p6) was paired with the corresponding patient treatment history. Treatment information came from the records on the diagnostic order forms, on which the indication for a resistance test is provided, or directly from the SHCS database. Also, the status of the treatment history is categorized for each drug as ‘current’ or ‘previous’.
Every sample with a status ‘current’ or ‘previous’ for at least one PI was considered PI treatment experienced (henceforth referred to as ‘PI-TE’; n = 515). No explicit statement was available indicating that a specific PI-TE patient had never been treated with any other PI. The samples from untreated patients formed the treatment-naive group (henceforth referred to as ‘PI-TN’, n = 825).
Statistical analysis
Data analysis was performed using R language, version 3.2.4 Revised (2016-03-16 r70336).38 Statistical associations were assessed using Fisher’s exact test with significance level 0.05. Prevalences of Gag and PR mutations in the viral sequences of PI-TE patients versus PI-TN patients were assessed. We defined and analysed the following distinct types of mutations: major PI resistance mutations in PR; minor PI resistance mutations in PR; other PR mutations; and treatment-associated Gag NC–p6 mutations. Analyses were limited to the available subtype B viruses (n = 890; of these PI-TE n = 369 and PI-TN n = 521; Table S1, available as Supplementary data at JAC Online).
Construction of mutagenetic trees
The mtreemix method39,40 as implemented in the R package Rtreemix, version 1.32.0,41 makes it possible to model multiple paths of an ordered accumulation of genetic changes from cross-sectional data. Assuming that all observed mutations are permanent, it estimates local maximum likelihood mutagenetic tree models. These models have been successfully used to scrutinize the development of HIV resistance as characterized by an ordered accumulation of resistance mutations in the viral genome under drug pressure.39 For estimating the stability of fitted models, we performed 1000 rounds of bootstrapping and selected tree branches by the number of supporting bootstraps.
Ethics
Project and data analysis had been approved by the Scientific Board of the SHCS prior to analysis. All specimens were from the SHCS and for each patient written informed consent as well as ethical clearance from the respective local Ethics boards had been obtained for anonymized data analysis as performed in this study.
Results
Validating the approach using the prevalence of resistance mutations in the PR gene
In order to verify the validity of our analytical approach, the prevalence of well-established PI resistance mutations in the PR gene was examined for PI-TE and PI-TN samples. In the overall collection of PI-TE viruses 24.9% (92/369) of all sequence samples carried at least one of the known major resistance mutations in the PR gene. This was found to be in good agreement with published data.42,43 As a further confirmation, mutations D30N, V32I, M46L/I, I54V, V82A, I84V and L90M (Table S2) were found to be significantly overrepresented in the PI-TE group compared with the PI-TN group. Nine minor PI mutations in the PR gene were preferably detected in the PI-TE group: L10F/I, L33F, Q58E, L63P, A71V/I, 73S and N88D (Table S3).
Demonstration of established PI-associated mutations in Gag
In the next step of verifying our analytical approach, we determined the prevalences of those Gag mutations in the analysed NC–p6 region that have previously been reported to associate with PI exposure and/or resistance to PIs (Table S4). Among the PI-TE samples in our study, 56.4% (208/369) carried at least one of the known Gag mutations. For the PI-TE HIV variants carrying at least one major PR mutation, Verheyen et al.44 have reported rates of known Gag mutations to be as high as 65.3%. Our study also found the known A431V, I437V and P453L mutations, for which a phenotypic proof of PI resistance by in vitro mutagenesis has been provided,24,30,45 and mutation L363M. In 17.9% (66/369) of the PI-TE sequences of our cohort, at least one of the former three was present, compared with 8.3% (43/521) in the PI-TN samples of the set. Furthermore, in our dataset 93.8% (15/16) of all PI-TE samples with A431V carried also at least one major resistance mutation in PR. Mutations P453L and I437V occurred along with major PI resistances in 60.5% (26/43) and 42.9% (6/14) of cases, respectively.
In a next step, the data set was examined for the presence of Gag alterations that by themselves lead to therapy failure. In the total pool of PI-TE samples, 86.4% (319/369) had viral loads of at least 200 copies/mL, which this study used as an indicator for possible treatment failure. In this group alone, 43.9% (140/319) of all samples also carried resistance mutations for NNRTIs or NRTIs, and 21.9% (70/319) carried known resistance mutations in the pr gene. At the same time, 11.3% (36/319) of samples in the group carried known resistance-associated mutations in Gag, and NNRTI or NRTI resistances but no major resistance mutations in PR. Gag mutation A431V was detected in combination with at least one reverse transcriptase inhibitor (RTI) resistance mutation and one major PI resistance mutation in 92.3% (12/13). For sequences with I437V this rate was 33.3% (4/12). P453L was detected in combination with RTI resistances and major PI resistance mutations in 53.9% of cases (21/39).
Novel Gag mutations associated with PI exposure
In this study we were able to identify several novel Gag mutations that emerge during PI exposure and failure: T427D/N and E467V/K were found to prominently associate with PI treatment in our study set (Table 1). Moreover, mutation Q474H correlated with the simultaneous occurrence of T427N (13.3%; OR = 24.4) in our dataset. Most of the sequences with T427D/N (79%; 15/19) carried none of the known PI or RTI resistance mutations.
Mutation . | Protein . | Cleavage site . | PI-TE . | PI-TN . | P . | OR (95% CI) . |
---|---|---|---|---|---|---|
A360S/P | p24 CA | p24 CA/p2 SP1 | 7% (6/86) | 1.2% (3/255) | 0.01 | 6.30 (1.36–32.62) |
Q369L | p2 SP1 | non-CS | 8.1% (7/86) | 1.6% (4/255) | 0.007 | 5.50 (1.36–26.31) |
T427D/N | p7 NC | non-CS | 10.4% (19/182) | 3.9% (13/338) | 0.004 | 2.91 (1.33–6.43) |
E467V/K | p6 | non-CS | 3.6% (13/356) | 0.8% (4/512) | 0.005 | 4.81 (1.45–17.63) |
Mutation . | Protein . | Cleavage site . | PI-TE . | PI-TN . | P . | OR (95% CI) . |
---|---|---|---|---|---|---|
A360S/P | p24 CA | p24 CA/p2 SP1 | 7% (6/86) | 1.2% (3/255) | 0.01 | 6.30 (1.36–32.62) |
Q369L | p2 SP1 | non-CS | 8.1% (7/86) | 1.6% (4/255) | 0.007 | 5.50 (1.36–26.31) |
T427D/N | p7 NC | non-CS | 10.4% (19/182) | 3.9% (13/338) | 0.004 | 2.91 (1.33–6.43) |
E467V/K | p6 | non-CS | 3.6% (13/356) | 0.8% (4/512) | 0.005 | 4.81 (1.45–17.63) |
CA, capsid; NC, nucleocapsid; CS, cleavage site.
Mutation . | Protein . | Cleavage site . | PI-TE . | PI-TN . | P . | OR (95% CI) . |
---|---|---|---|---|---|---|
A360S/P | p24 CA | p24 CA/p2 SP1 | 7% (6/86) | 1.2% (3/255) | 0.01 | 6.30 (1.36–32.62) |
Q369L | p2 SP1 | non-CS | 8.1% (7/86) | 1.6% (4/255) | 0.007 | 5.50 (1.36–26.31) |
T427D/N | p7 NC | non-CS | 10.4% (19/182) | 3.9% (13/338) | 0.004 | 2.91 (1.33–6.43) |
E467V/K | p6 | non-CS | 3.6% (13/356) | 0.8% (4/512) | 0.005 | 4.81 (1.45–17.63) |
Mutation . | Protein . | Cleavage site . | PI-TE . | PI-TN . | P . | OR (95% CI) . |
---|---|---|---|---|---|---|
A360S/P | p24 CA | p24 CA/p2 SP1 | 7% (6/86) | 1.2% (3/255) | 0.01 | 6.30 (1.36–32.62) |
Q369L | p2 SP1 | non-CS | 8.1% (7/86) | 1.6% (4/255) | 0.007 | 5.50 (1.36–26.31) |
T427D/N | p7 NC | non-CS | 10.4% (19/182) | 3.9% (13/338) | 0.004 | 2.91 (1.33–6.43) |
E467V/K | p6 | non-CS | 3.6% (13/356) | 0.8% (4/512) | 0.005 | 4.81 (1.45–17.63) |
CA, capsid; NC, nucleocapsid; CS, cleavage site.
For a subset of samples from the Basel site of the study, longer Gag sequences were available, also covering the region of the p24/p2 cleavage site. In this set of PI-TE samples two additional novel alterations were identified: A360S/P and Q369L (Table 1). Mutation Q369L was preferentially linked with either one of three other, established correlates of PI exposure: K418R (42.9%; OR = 5.8), I437V (28.6%; OR = 10.9) and P453T (42.9%; OR = 15.0). A360S/P was in no case accompanied by major resistances in PR, and in only one sample was A360S linked to RTI resistance.
In order to address a possible bias that could arise from different available read lengths, PI-TE samples with longer gag sequences were compared with PI-TE samples with shorter gag reads for: (i) major resistance mutations in PR; (ii) minor resistance mutations in PR; and (iii) known mutations in gag. Only a single major resistance mutation in PR, V32I, occurred more often in PI-TE samples with shorter gag (4.6%, 13/283 versus 0%, 0/86; OR = 1.1). Conversely, the major resistance mutation in PR, I50L (3.5%, 3/86 versus 0%, 0/283; OR = 1.4), and the minor resistance mutations in PR, G73T (3.5%, 3/86 versus 0.4%, 1/283; OR = 10.1), as well as two known gag treatment-associated mutations, K436R (11.6%, 10/86 versus 3.1%, 4/129; OR = 4.1) and S451N (24.4%, 21/86 versus 12.1%, 29/239; OR = 2.3), were more frequent in PI-TE samples with longer gag sequences.
Beyond a principal linkage with treatment, some of the new mutations tended to associate with exposure to certain drugs: T427D/N with lopinavir, E467K with nelfinavir, and Q474H or Y484P with darunavir (Table 2). Five out of six cases with E467K also carried resistance mutations in PR and RT. None of the four darunavir-experienced viruses with both Gag mutations Q474H and Y484P carried any known PI or RTI resistance mutation.
Mutation . | Protein . | Cleavage site . | PI . | PI-TE . | PI-TN . | P . | OR (95% CI) . |
---|---|---|---|---|---|---|---|
T427D/N | p7 NC | non-CS | lopinavir | 13.2% (12/91) | 4.7% (20/429) | 0.006 | 3.12 (1.37–7.00) |
E467K | p6 | non-CS | nelfinavir | 3.8% (6/157) | 1.1% (8/711) | 0.029 | 3.49 (0.98–11.65) |
Q474H | p6 | non-CS | darunavir | 5% (4/80) | 1.5% (12/802) | 0.049 | 3.46 (0.79–11.77) |
Mutation . | Protein . | Cleavage site . | PI . | PI-TE . | PI-TN . | P . | OR (95% CI) . |
---|---|---|---|---|---|---|---|
T427D/N | p7 NC | non-CS | lopinavir | 13.2% (12/91) | 4.7% (20/429) | 0.006 | 3.12 (1.37–7.00) |
E467K | p6 | non-CS | nelfinavir | 3.8% (6/157) | 1.1% (8/711) | 0.029 | 3.49 (0.98–11.65) |
Q474H | p6 | non-CS | darunavir | 5% (4/80) | 1.5% (12/802) | 0.049 | 3.46 (0.79–11.77) |
Mutation . | Protein . | Cleavage site . | PI . | PI-TE . | PI-TN . | P . | OR (95% CI) . |
---|---|---|---|---|---|---|---|
T427D/N | p7 NC | non-CS | lopinavir | 13.2% (12/91) | 4.7% (20/429) | 0.006 | 3.12 (1.37–7.00) |
E467K | p6 | non-CS | nelfinavir | 3.8% (6/157) | 1.1% (8/711) | 0.029 | 3.49 (0.98–11.65) |
Q474H | p6 | non-CS | darunavir | 5% (4/80) | 1.5% (12/802) | 0.049 | 3.46 (0.79–11.77) |
Mutation . | Protein . | Cleavage site . | PI . | PI-TE . | PI-TN . | P . | OR (95% CI) . |
---|---|---|---|---|---|---|---|
T427D/N | p7 NC | non-CS | lopinavir | 13.2% (12/91) | 4.7% (20/429) | 0.006 | 3.12 (1.37–7.00) |
E467K | p6 | non-CS | nelfinavir | 3.8% (6/157) | 1.1% (8/711) | 0.029 | 3.49 (0.98–11.65) |
Q474H | p6 | non-CS | darunavir | 5% (4/80) | 1.5% (12/802) | 0.049 | 3.46 (0.79–11.77) |
Pairwise associations of Gag and PR mutations after drug exposure
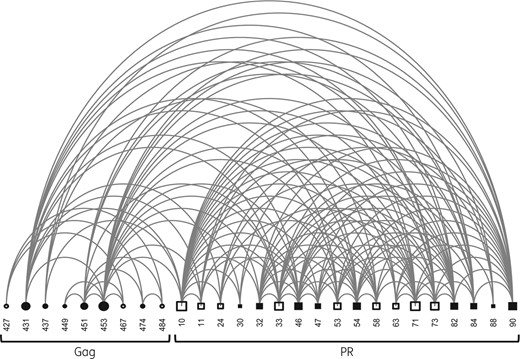
Arch diagram of covariation between amino acids at the Gag C-terminus and in PR. A connector between two nodes indicates a verified covariation. Node sizes are proportional to the degree of interconnectivity of a given residue. Filled squares indicate positions linked to major PI resistance; open squares indicate minor resistance; filled circles indicate known Gag resistance- or treatment-associated mutation; and open circles indicate newly identified treatment-associated mutations in Gag.
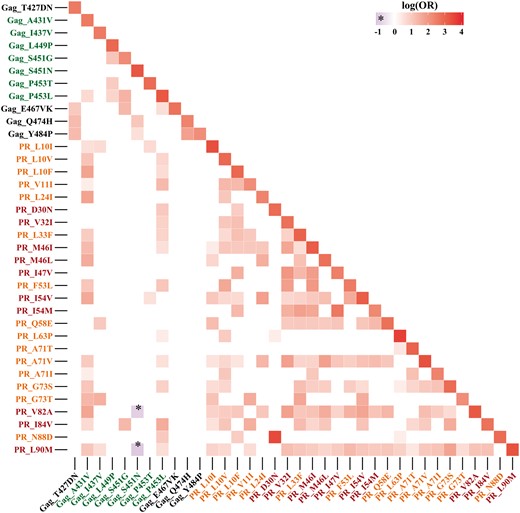
Heat map representing pairwise correlations in the Gag–PR region, ordered according to amino acid positions. Red labels indicate major PI resistance codons in PR, orange labels indicate minor PI resistance mutations, green labels indicate Gag positions correlated with PI exposure and black labels indicate novel Gag codons linked to PI exposure. Only significant associations are shown as coloured squares; a more intense colour reflects higher significance, with OR plotted on a logarithmic scale. This figure appears in colour in the online version of JAC and in black and white in the print version of JAC.
Among the minor PI resistance mutations in PR mutations, A71V and L33F had the widest interconnection, each with 19 associations with other changes in PR or Gag. In Gag, P453L and A431V were the most interconnected. Both were located at the p7/p1 and p1/p6 cleavage sites; they had 18 significant links in our TE data set. The polymorphic position L449P had three connections.
The correlates of PI exposure newly observed in this study turned out to be poorly interconnected. Their linkages were exclusively restricted to other Gag alterations: T427D/N was linked to E467V/K, Q474H and Y484P. For E467V/K we observed an association with T427D/N, S451G and P453L. In association with each other, Q474H and Y484P were linked to T427D/N and S451N.
Patterns and predicted order of accumulation of mutations
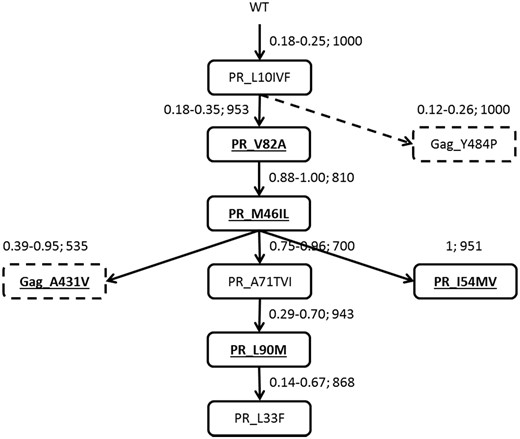
Mutagenetic tree illustrating the mutational pathways for Gag and PR mutations observed in the dataset. Bold and underlined font in an unbroken box represents major resistance mutations in PR; ordinary font in an unbroken box represents minor resistance mutations in PR; bold and underlined font in a broken box represents known treatment- and resistance-associated mutations in Gag; ordinary font in broken box represents novel treatment-associated mutations in Gag. Arrows indicate the order of appearance. The first two numbers next to an arrow represent the 95% CI for the conditional probability of occurrence of the next respective genetic event. The third number indicates the bootstrap support of a given element. The broken arrow indicates a branch with no support from the analysis of pairwise associations.
Discussion
This study is the first in the SHCS to systematically investigate potential PI resistance residing in Gag as the PR substrate and their possible role during PI treatment.
Beyond established Gag resistances: novel PI-associated mutations
Most of the currently known mutations in Gag relevant for PI resistance reside in or near the p7/p1 and p1/p6 cleavage sites. Our study attempted to complement this information by including a longer C-terminal region of Gag for 58.4% (520/890) of our subtype B sequences. As a result, a few additional mutations could be associated with PI exposure, which are mainly located at functionally important points of the Gag polyprotein: positions A360 and Q369 are both well conserved among M-group isolates of HIV-1, and alterations at position 360 directly affect p24/p2 cleavage, a function that has been associated with virion assembly and release.46 Residue 369 belongs to the binding sequence of the maturation inhibitor bevirimat and is required for proper virion formation and maturation, a step that critically depends on the conformation of spacer peptide 1 (p2).47 The folding of this spacer could be affected by the observed exchange of the hydrophilic glutamine with the rather hydrophobic leucine. Furthermore, the observed association of Q369L with other known Gag mutations, such as K418R, I437V and P453T, further supports a crucial role in viral functioning.
Although PI-TE samples with longer or shorter Gag sequences tended to yield some heterogeneity in terms of prevalence of mutations, this could also be associated with the respective study site and selection effects, as more samples with longer Gag sequences were available from the Basel site.
Mutation T427N has previously been recognized as a CTL escape mutation affecting viral replication capacity.48 Changing the conserved polar threonine in HIV-1 group M viruses to asparagine or aspartic acid greatly increases the hydrophilicity, which, in the immediate vicinity of a PR cleavage site, could affect PR recognition and cleavage.
Of interest, p6 contains several predicted ERK-2 phosphorylation sites, i.e. at positions T456, S462, T469, T471, S473, Y484 and S499,49 and the incorporation of ERK-2 into the HIV particle is thought to regulate the L-domain function of p6.50 Since mono-ubiquitination can be regulated via phosphorylation through cellular kinases, mutations might directly affect the structure of p6,49 while the function of p6 is thought to require the hydrophobic characteristics.51 Hence the mutations E467V/K, 474H and Y484P described in this study are positioned in this seemingly crucial p6 region near the phosphorylation motifs and L-domains.
Gag amino acid 467 marks another potentially critical and conserved position. It resides between the proline-rich region, responsible for interaction with TSG101, and the ALIX interaction motif and is flanked by Vpr binding sequences. Glutamic acid at this position is the inter-subtype consensus. Therefore, the observed change to valine would change the character from hydrophilic to hydrophobic, while lysine would reverse the negative charge in the WT to a positive charge. It is not known what the precise contact points in the reaction partners are, but it is likely that either one of these drastic mutations would have implications for protein–protein interaction.
Mutation Q474L has, at least in vitro, been found to be deleterious for viral replication, while with the simultaneous introduction of corresponding Gag cleavage-site mutations the phenotype could be rescued.28 For some HIV-1 subtypes it is linked to PI resistance when combined with P453L/T.52 The latter mutation has been associated with a loss of an ERK-2 phosphorylation site at T471.52 In our dataset mutation Q474H was linked with the putative CTL epitope at T427N (13%; OR = 24.4). This supports a connection of the p6 phosphorylation motifs with the sequence surrounding the p7/p1 cleavage site. As the amino acid histidine can serve as substrate for mammalian protein kinases, mutation Q474H might provide a phosphorylation site alternative to T471, suggesting a potential link between the loss of phosphorylation at T471 and mutation Q474H.
Tyr at position 484, the WT amino acid for HIV-1 subtype B, belongs to the ALIX recognition motif. Various mutations at residues 482–484 have been described to affect ALIX binding, leading to the accumulation of the Gag processing intermediates p41 and p25.53,54 This observation highlights an additional connection between the p6 protein and processing efficiency at the cleavage sites for p17/p24 and p24/p2. Moreover, residues 483 and 484 have been identified as subtype-dependent CTL epitopes,55 and mutation L483M has been selected in vitro in the presence of darunavir.56
In line with previous studies,28,52,57 our data support the claim that p6 alterations have a role in the development of PR resistance. Phosphorylation and folding can affect the efficiency of Gag processing and might hence change viral fitness and PI susceptibility.
Frequency of known Gag treatment-associated mutations in the SHCS
The frequent occurrence of mutations I437V and P453L among the PI-TE samples in the absence of any accompanying major PR mutations or RTI resistances might indicate the influence of several mechanisms. They could either have an independent role in resistance or they might prime, as initial steps further mutations in PR, or they could directly cooperate with minor PR mutations. Mutation P453L has been shown earlier to confer resistance only with accompanying major PR mutations.30 This led us to speculate that in our dataset I437V is the most likely candidate (of the observed known PI treatment- and resistance-associated mutations in Gag) to contribute to the failure of PI-containing regimens in the absence of major resistances. In the standardized in vitro background it decreased susceptibilities to lopinavir, tipranavir, atazanavir and amprenavir.24
The frequent identification of at least one known PI treatment- and resistance-associated mutation in Gag in the PI-TE set of SHCS samples is in good agreement with a report by Malet et al.,58 who report the presence of at least one mutation at the p2/NC cleavage site alone in 91% of PI-exposed subtype B viruses. In 17.9% (66/369) of all PI-TE samples the examined Gag region carried mutations capable of decreasing PI susceptibility either alone or in combination with PR resistances, compared with only 8.3% (43/521) in the PI-TN group. These figures are in good agreement with the 19.1% for PI-TE and 12.1% for PI-TN samples reported by Verheyen et al.44 As the Gag region has not been considered in routine HIV resistance genotyping in diagnostic settings until now, the overall level of PI resistance may be higher than reported. Although this study could not sufficiently discriminate whether a test request was based on ‘suspected failure’ versus ‘consequence of therapy failure’, this finding demonstrates that a significant number of sequence analyses did indeed occur at times of virological failure.
Validity of the approach and outlook
The pilot steps of our analysis validated the analytical approach, since the observed rates of major and minor resistances in PR in the PI-TE and PI-TN groups matched previously published data.36,37,59–62 The fraction of PI-TE patients affected by any major resistance in PR is in agreement with previous reports as well.42,43
This study suggests that mutations in Gag such as I437V (this mutation was frequently found in the absence of major PR resistances and was experimentally shown to independently confer a degree of PI resistance) might be independently responsible for the development of PI resistance. Or, similar to minor PR alterations, other mutations could act indirectly by compensating for fitness loss and/or by cooperatively decreasing PI susceptibility.
Overall, this analysis of data from the SHCS is in good agreement with previously published data. In addition, our analysis has revealed new, potentially important mutations in Gag that could have clinical implications. However, further phenotypic analyses and clinical correlates of drug failure will be needed before such information is suitable for amending existing resistance algorithms that are used for genotypic HIV resistance testing.
Acknowledgements
We express our sincere gratitude to Sarah Wagner, Joelle Bader and Alexandra Haas for critical review and valuable input to the article. We appreciate the expert technical help of Sabrina Steiner and Adelaide Loureiro in the laboratory.
This study was conducted as a research project approved by the Scientific Board of the Swiss HIV Cohort Study with approval of the ethics committees of all seven participating study sites (Kantonale Ethikkommission Bern, Ethikkommission beider Basel, Ethikkommission des Kantons St Gallen, Kantonale Ethikkommission Zürich, Comitato etico cantonale del Ticino, Commission d'éthique de la recherche clinique de la faculté de biologie et de medicine de l'université de Lausanne, Comité d'éthique du département de médicine des hôpitaux universitaires de Genève). Written consent has been obtained from all participants.
Members of the Swiss HIV cohort study
V. Aubert, M. Battegay, E. Bernasconi, J. Böni, D. L. Braun, H. C. Bucher, A. Calmy, M. Cavassini, A. Ciuffi, G. Dollenmaier, M. Egger, L. Elzi, J. Fehr, J. Fellay, H. Furrer (Chairman of the Clinical and Laboratory Committee), C. A. Fux, H. F. Günthard (President of the SHCS), D. Haerry (deputy of ‘Positive Council’), B. Hasse, H. H. Hirsch, M. Hoffmann, I. Hösli, C. Kahlert, L. Kaiser, O. Keiser, T. Klimkait, R. D. Kouyos, H. Kovari, B. Ledergerber, G. Martinetti, B. Martinez de Tejada, C. Marzolini, K. J. Metzner, N. Müller, D. Nicca, G. Pantaleo, P. Paioni, A. Rauch (Chairman of the Scientific Board), C. Rudin (Chairman of the Mother & Child Substudy), A. U. Scherrer (Head of Data Centre), P. Schmid, R. Speck, M. Stöckle, P. Tarr, A. Trkola, P. Vernazza, G. Wandeler, R. Weber, S. Yerly.
Funding
This study has been financed within the framework of the Swiss HIV Cohort Study, supported by the Swiss National Science Foundation (grant #148522), by SHCS project #720 and by the SHCS research foundation. The data are gathered by the 5 Swiss University Hospitals, 2 Cantonal Hospitals, 15 affiliated hospitals and 36 private physicians (listed in http://www.shcs.ch/180-health-care-providers). K. K. received a travel grant from Boehringer Ingelheim. D. H. gratefully acknowledges funding by Deutsche Forschungsgemeinschaft, grant TRR60/B1. None of the funding institutions had any influence on the conduct, analysis or publication of the work.
Transparency declarations
None to declare.
Supplementary data
Tables S1 to S4 are available as Supplementary data at JAC Online (http://jac.oxfordjournals.org/).
References
Author notes
†Present address: Office for Public Health, Berne, Switzerland.
‡Members of the Swiss HIV Cohort Study are listed in the Acknowledgements section.