-
PDF
- Split View
-
Views
-
Cite
Cite
Xiangke Duan, Xue Huang, Xiaoyu Wang, Shuangquan Yan, Siyao Guo, Abualgasim Elgaili Abdalla, Changwu Huang, Jianping Xie, l-Serine potentiates fluoroquinolone activity against Escherichia coli by enhancing endogenous reactive oxygen species production, Journal of Antimicrobial Chemotherapy, Volume 71, Issue 8, August 2016, Pages 2192–2199, https://doi.org/10.1093/jac/dkw114
- Share Icon Share
Abstract
The increase in multiple antimicrobial-resistant bacteria seriously threatens global public health. Novel effective strategies are urgently needed. l-Serine was reported as the most effective amino acid inhibitor against bacterial growth and can sensitize Escherichia coli cells to gentamicin. It is currently unknown whether l-serine affects other type of antibiotics such as β-lactams and fluoroquinolones.
Using E. coli, we studied the combination of l-serine with diverse antibiotics against laboratory and clinical E. coli cultures and persisters. The intracellular NAD+/NADH level and ROS were determined using kits. Total cellular iron was determined by using a colorimetric ferrozine-based assay.
Exogenous l-serine sensitized E. coli ATCC 25922 and clinically isolated fluoroquinolone-resistant E. coli to fluoroquinolones. This potentiation is independent of growth phase. Addition of serine increases the production of NADH. The underlying mechanism of this strategy is that the combination of serine with ofloxacin or moxifloxacin increases the NAD+/NADH ratio, disrupts the Fe-S clusters and increases the production of endogenous reactive oxygen species. Furthermore, we used a serine and ofloxacin or moxifloxacin combination in vitro to combat bacterial persister cells, compared with antibiotic treatment alone; combinational treatments of persister cells with antibiotics and l-serine resulted in a significantly greater decrease in cell viability.
To our knowledge, this is the first report that l-serine can potentiate the action of ofloxacin or moxifloxacin against Gram-negative bacteria and could constitute a new strategy for the eradication of bacterial infections.
Introduction
Bacterial antimicrobial resistance, particularly multidrug resistance, is increasingly serious and the development of new antibiotics is rather sluggish.1 The declining efficacy of currently available antibiotics2 demands novel strategies for better control of pathogen infection. The combination of compounds and antibiotics represents one promising avenue to improve the efficacy of existing antibiotics.3
It is well known that certain amino acids inhibit bacterial growth,4,5 and l-serine was the most effective inhibitor.6 The bacteriostatic effect on Escherichia coli was caused by inhibition of homoserine dehydrogenase I by l-serine.7,8 Overexpression of rhodanese-like protein enhanced the serine susceptibility in E. coli.9 Shan et al.10 found that l-serine is able to sensitize E. coli cells to gentamicin. Fluoroquinolones, one of the most successful broad-spectrum antibiotics, are active against both Gram-negative and Gram-positive bacteria.11 Quinolones can form quinolone–enzyme–DNA complexes and the subsequent release of DNA ends from such complexes results in the generation of detrimental double-stranded DNA breaks, thereby inhibiting bacterial growth.12–14 The generation of reactive oxygen species (ROS) via the Fenton reaction was proposed to contribute to fluoroquinolone-mediated bacteria cell death,15–17 although with some controversy.18,19 ROS are reactive by-products formed by the partial reduction of molecular oxygen of the respiratory chain enzymes, such as superoxide, hydrogen peroxide (H2O2) and hydroxyl radicals.20 The accumulation of ROS within bacteria can arrest cell growth or trigger death by damaging proteins, membrane lipids, DNA and RNA.15,21 Increase in the endogenous microbial ROS production can enhance killing by the antibiotics.22
In the current work, we show that a combination of ofloxacin or moxifloxacin and l-serine increases in the killing efficiency of ofloxacin and moxifloxacin toward E. coli in vitro. We demonstrate that serine can potentiate the antibacterial activity of ofloxacin and moxifloxacin by enhancing endogenous ROS production in E. coli. l-Serine can also potentiate ofloxacin and moxifloxacin against bacterial persisters. These results suggest that serine might be a potentiator of ofloxacin and moxifloxacin to eradicate Gram-negative and persister infections.
Materials and methods
Strains and growth conditions
E. coli ATCC 25922 and E. coli D137 were used in this study. Cells were cultured in LB at 37°C with aeration at 200 rpm unless specified otherwise. For all experiments performed with cells in exponential phase, E. coli overnight cultures were diluted 1: 250 in 20 mL of LB medium and grown to exponential phase in 100 mL flasks at 37°C, 200 rpm. All antimicrobial treatments were performed using 1 mL of sample in 24-well plates incubated at 37°C for 4 h. For experiments with bacterial persister cells, E. coli was grown to stationary phase for 16 h at 37°C, in a shaking incubator at 200 rpm using 20 mL of LB medium. Cells were then treated with ofloxacin (5 mg/L) for 4 h to kill non-persistent cells. The samples were then washed with PBS, suspended in M9 minimal medium and treated with different antibiotics to check the survival of persisters.
Antibiotics and chemicals
Ampicillin, ofloxacin, moxifloxacin, norfloxacin, ciprofloxacin, thiourea and 2,2′-bipyridyl (BIP) were obtained from Sangon Biotech Co. (Shanghai, China) and their stock solutions were freshly prepared, filter-sterilized and used at indicated concentrations. l-Serine was purchased from Beijing Dingguo Changsheng Biotechnology Co. Ltd (Beijing, China). The ddH2O were used to prepare stock solution of amino acid (500 mM), stored at 4°C and used at indicated concentrations. Ferrozine, neocuproine, ammonium acetate and ascorbic acid were obtained from Sigma-Aldrich Company Ltd (Poole, Dorset, UK).
MIC determination
The MICs of ofloxacin and moxifloxacin were determined using serial 2-fold dilutions of the antibiotics in LB medium and the samples were incubated for 16 h at 37°C. The MIC was determined as the concentration of antibiotics that prevented growth. All MICs were tested in duplicate at least twice.
Antibiotic survival assay
To obtain exponential- and mid-stationary-phase cultures in LB, overnight cultures (16 h) were diluted 1: 1000 in fresh medium and grown to the desired turbidity (i.e. turbidity <2 at 600 nm for the exponential phase and turbidity of 3–4 at 600 nm for the mid-stationary phase). Cultures were centrifuged at 8000 rpm for 8 min, samples were washed twice with 1 × PBS and re-suspended in M9 minimal medium supplemented with 10 mM acetate, 1 mM MgSO4 and 100 mM CaCl2 to OD600 = 0.2. Ofloxacin (0.3 mg/L) or moxifloxacin (0.3 mg/L) was added. For the amino acid supplementation experiments, the specified amino acid was added to an exponential-phase culture at indicated concentrations. After incubation, 100 μL samples were removed, serially diluted and plated (10 μL aliquots) on LB agar plates for cfu count. The survival rate of cfu at 4 h divided by cfu at 0 h was calculated. Results are averages from four biological replicates and error bars represent standard deviations.
Persister assays
For E. coli persister assays, samples were grown to log phase and stationary phase as described above. Cultures were then treated with 5 mg/L ofloxacin or moxifloxacin in the above-mentioned growth conditions and l-serine was added at different timepoints. For the isolation of persisters, ciprofloxacin (5 mg/L) was added to log-phase or overnight cultures and incubated with aeration at 37°C for 4 h. Surviving cells were pelleted and resuspended in M9 with or without l-serine and antibiotic for 4 h to determine l-serine-enabled killing of persisters. At specified timepoints, 50 μL samples were removed, serially diluted and spot-plated onto LB agar plates to determine cfu/mL. Only dilutions that yielded 10–100 colonies were counted. Survival was determined by dividing the cfu/mL of a sample at each timepoint by the initial cfu/mL for that sample.
NADH measurements
E. coli culture was diluted to OD600 = 0.2 and incubated with amino acid at 37°C for 4 h. Cell pellets were washed with 1 × PBS and re-suspended with NADH extraction buffer. Heat extracts were performed at 60°C for 5 min. Buffer and NAD extraction buffer was added to neutralize the extracts. The cells were vortexed and spun down at 14 000 rpm for 5 min. Supernatant was collected for the EnzyChrom NAD/NADH Assay Kit (BioAssay Systems).
Determination of iron concentrations
Cellular iron was determined using a colorimetric ferrozine-based assay.23 Cultures of E. coli were grown to an OD600 ≈ 0.2. After addition of the appropriate chemicals (l-serine, ofloxacin or moxifloxacin), the cultures were incubated for up to 1, 2 and 4 h and harvested by centrifugation. The cell pellets were washed twice with ice-cold PBS, resuspended in 1 mL 50 mM NaOH with vortexing. To quantify the bound iron, the lysate (100 μL) was mixed with 10 mM HCl (0.1 mL) and 0.1 mL of iron-releasing reagent [1.4 M HCl + 4.5% (w/v) aqueous solution of KMnO4; 1:1] and incubated at 60°C for 2 h.23 After the mixture was cooled to room temperature, 30 μL iron-detection reagent (6.5 mM ferrozine + 6.5 mM neocuproine + 2.5 M ammonium acetate + 1 M ascorbic acid in water) was added. After 30 min, 280 μL of the solution in each tube was transferred into a well of a 96-well plate and the sample absorbance was read at 550 nm by a SpectraMax 190 microplate reader. The iron concentrations were determined based on a standard curve obtained with increasing concentrations of ferric chloride and normalized to protein content.
ROS determination
ROS were detected by the Reactive Oxygen Species Assay Kit (Beyotime Institute of Biotechnology, China) based on 2′,7′-dichlorodihydrofluorescein diacetate (DCFH-DA). Exponential-phase cultures were washed twice with 1 × PBS and incubated with 10 mM DCFH-DA in 37°C for 20 min. They were then washed twice with 1 × PBS and re-suspended in M9 minimal medium, before treating them with ofloxacin (0.3 mg/L) or moxifloxacin (0.3 mg/L) in the presence or absence of 15 mM l-serine. Aliquots were taken at the indicated timepoints and the fluorescence intensity was monitored using a Tecan Infinite® 200 PRO microplate reader with excitation at 488 nm and emission at 525 nm. Relative ROS production was expressed as a percentage of DCF fluorescence of the untreated control.
Results
l-Serine increases the susceptibility of planktonic laboratory and clinical E. coli cells to ofloxacin and moxifloxacin
Planktonic cultures of E. coli in exponential growth phase were treated with ampicillin and ofloxacin. We found that the cell survival decreased with increasing dose of ofloxacin and moxifloxacin in the presence of l-serine (Figure 1a and b). However, no potentiation of ampicillin was spotted (Figure S1, available as Supplementary data at JAC Online). Then, we treated the bacteria with 0.3 mg/L ofloxacin or moxifloxacin in the presence of different l-serine concentrations. We observed this potentiation in an l-serine dose-dependent manner for ofloxacin and moxifloxacin (Figure 1c), but no striking differences were observed between the effects obtained using 2.5 and 10 mM concentrations for ofloxacin. Moreover, we found that the potentiation of l-serine on ofloxacin was slightly attenuated in the late-stationary phase (the survival rate was reduced 8.3- and 4.7-fold), but l-serine showed strong potentiation on moxifloxacin against different growth-phase bacteria (Figure 1d and e). Next, we wanted to know whether l-serine could potentate the bactericidal efficacy against the fluoroquinolone-resistant strains. To test this, we used E. coli D137, a clinical fluoroquinolone-resistant isolate with 80- and 256-fold higher ofloxacin and moxifloxacin MICs compared with the E. coli ATCC 25922 strain (Table 1). We observed that cell survival decreased upon treatment with ofloxacin or moxifloxacin in the presence of l-serine (Figure 1d). These data show that l-serine can enhance the antibiotic susceptibility of both E. coli ATCC 25922 and fluoroquinolone-resistant clinical isolates.
Strain . | Ofloxacin . | Moxifloxacin . |
---|---|---|
E. coli ATCC 25922 | 0.625 | 0.625 |
E. coli D137 | 320 | 80 |
Strain . | Ofloxacin . | Moxifloxacin . |
---|---|---|
E. coli ATCC 25922 | 0.625 | 0.625 |
E. coli D137 | 320 | 80 |
Strain . | Ofloxacin . | Moxifloxacin . |
---|---|---|
E. coli ATCC 25922 | 0.625 | 0.625 |
E. coli D137 | 320 | 80 |
Strain . | Ofloxacin . | Moxifloxacin . |
---|---|---|
E. coli ATCC 25922 | 0.625 | 0.625 |
E. coli D137 | 320 | 80 |
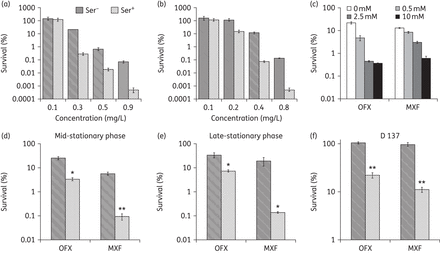
l-Serine increases the susceptibility of planktonic bacteria to ofloxacin and moxifloxacin. An exponentially growing E. coli culture was treated with different concentrations of ofloxacin (a) or moxifloxacin (b) alone and in combination with l-serine (15 mM). (c) E. coli incubated with increasing concentrations of l-serine for 4 h in the presence of ofloxacin or moxifloxacin. (d and e) Percentage survival of E. coli ATCC 25922 mid-stationary-phase (OD600 ≈ 4) and late-stationary-phase (16 h of growth) cultures. Cells were treated with 0.3 mg/L ofloxacin or 0.3 mg/L moxifloxacin. (f) An exponentially growing E. coli D137 culture was treated with 350 mg/L ofloxacin or 100 mg/L moxifloxacin in the presence of 15 mM l-serine. Values were compared with the control (without l-serine) at the same antibiotic concentration. Data are shown as mean ± SD of triplicate wells. Similar results were obtained in three independent experiments. *P < 0.05; **P < 0.01. OFX, ofloxacin; MXF, moxifloxacin.
l-Serine promotes intracellular NADH production in E. coli
In E. coli, l-serine is deaminated by three l-serine deaminases, namely SdaA, SdaB and TdcG,24–26 to form pyruvate by using the 4Fe-4S cluster.27 The pyruvate can be shunted into the tricarboxylic acid (TCA) cycle28 and generate NADH from NAD+ during the TCA cycle.29 If the exogenous l-serine could enter into the TCA cycle, as shown in Figure 2(a), then the intracellular NADH level will be elevated. Therefore, the intracellular NADH and NAD+ level was measured by the EnzyChrom NAD+/NADH Assay Kit after the bacterial cells were incubated with 15 mM serine for 4 h. Compared with untreated samples, the NADH and NAD+ concentrations increased 2.964- and 1.071-fold, respectively. The NAD+/NADH ratio increased 2.817-fold in the l-serine-treated samples owing to a larger increase in NADH concentration (Figure 2b), suggesting that the exogenous l-serine did enter into the TCA cycle and accelerate the production of NADH.
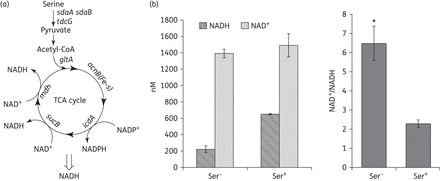
Effect of exogenous l-serine on the production of NADH and NAD+. (a) Superimposed metabolic pathways related to l-serine and the TCA cycle. (b) Intracellular NAD+ and NADH concentration were measured in E. coli treated with l-serine (15 mM) for 4 h. Values were compared with the control (without l-serine) at the same antibiotic concentration. Data are shown as mean ± SD of triplicate wells. Similar results were obtained in three independent experiments. *P < 0.01.
l-Serine enhances endogenous ROS production upon treatment with ofloxacin and moxifloxacin
It is interesting to know how l-serine mediates the potentiation of ofloxacin and moxifloxacin killing of E. coli cells. The established mechanism underlying the killing of bacteria by fluoroquinolones is predominantly via superoxide-derived oxidation of iron–sulphur clusters, which in turn stimulates the Fenton-mediated generation of highly destructive hydroxyl radicals.17 The major source of superoxide generation in E. coli is the reduction of oxygen during electron transport through the respiratory chain and the conversion of NADH into NAD+.30,31 The treatment of WT E. coli with the fluoroquinolone norfloxacin leads to an increase of the intracellular of NAD+/NADH ratio.32 To explore the effect of l-serine on the NAD+/NADH ratio when bacteria are treated with fluoroquinolones, we monitored NAD+ and NADH concentrations in WT E. coli following treatment with ofloxacin or moxifloxacin alone or in combination with l-serine. For the group treated with ofloxacin or moxifloxacin and l-serine, we observed that the NAD+/NADH ratio increased 2.005- and 1.825-fold, respectively, after 1 h of treatment when compared with the group treated with ofloxacin or moxifloxacin alone (Figure 3a). This ratio returned to the same levels of ofloxacin or moxifloxacin alone by 2 h (Figure 3a).
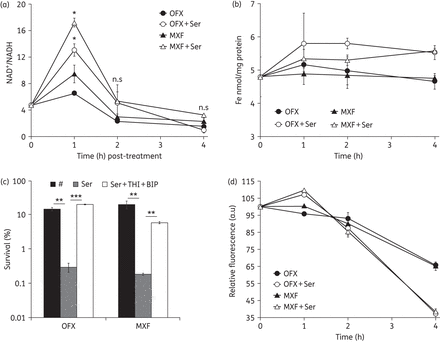
l-Serine promotes endogenous ROS production in the presence of ofloxacin or moxifloxacin. (a) Fold change in NAD+/NADH following treatment with 0.3 mg/L ofloxacin or 0.3 mg/L moxifloxacin in the presence or absence of 15 mM l-serine. (b) Iron content of E. coli in the presence of 15 mM l-serine compared with treatment with 0.3 mg/L ofloxacin or 0.3 mg/L moxifloxacin alone. (c) An exponentially growing E. coli culture was treated with 0.3 mg/L ofloxacin alone or 0.3 mg/L moxifloxacin alone, with 15 mM l-serine or with 15 mM l-serine and 100 mM thiourea plus 0.25 mM BIP for 4 h. (d) Percentages of intracellular increase in ROS generated in the presence of 15 mM l-serine compared with treatment with 0.3 mg/L ofloxacin or 0.3 mg/L moxifloxacin alone. Values were compared with the control (without l-serine) at the same antibiotic concentration. Data are shown as mean ± SD of triplicate wells. Similar results were obtained in three independent experiments. n.s indicates no significant difference (P ≥ 0.05); *P < 0.05; **P < 0.01; ***P < 0.001. OFX, ofloxacin; MXF, moxifloxacin.
The superoxide damages iron–sulphur clusters leading to the leaching of iron from iron–sulphur clusters.33 Therefore, we determined the iron concentrations in E. coli cultures treated with fluoroquinolones or fluoroquinolones plus l-serine. The group treated with antibiotics plus l-serine showed significantly higher ferric iron concentrations relative to the lysate from the group treated with antibiotics alone after 4 h of treatment (ofloxacin, P < 0.001; moxifloxacin, P < 0.01) (Figure 3b). To test whether this increased level of iron is associated with the l-serine-mediated potentiation of the bactericidal activity of ofloxacin and moxifloxacin, we pre-incubated E. coli cells with the iron chelator thiourea plus BIP before treating them with l-serine in conjunction with ofloxacin or moxifloxacin. We found that thiourea and BIP abolished the ofloxacin and moxifloxacin potentiation by l-serine (Figure 3c). These results demonstrated that addition of l-serine probably stimulated superoxide generation via the respiratory chain and destabilized iron–sulphur clusters, followed by release of iron from iron–sulphur clusters.
The reaction between free ferrous iron and hydrogen peroxide leads to the generation of highly destructive ROS (superoxide, hydrogen peroxide and hydroxyl radicals34) through the Harber–Weiss35 and Fenton reactions.21,36 It has not been widely established whether fluoroquinolones, such as ciprofloxacin,37,38 ofloxacin39 and moxifloxacin16 can induce the production of ROS, which contribute to the antimicrobial lethality.40–42 To test if l-serine enhanced fluoroquinolone activity against E. coli is a consequence of increased ROS production, ROS accumulation was measured in E. coli cultures treated with fluoroquinolones with or without l-serine using a susceptible fluorescent probe DCFH-DA.43 ROS were induced at the 1 h timepoint after the addition of l-serine and both showed a significant difference compared with the groups treated with ofloxacin or moxifloxacin alone (P < 0.05) (Figure 3d). The ROS level began to decline after 2 h and was much lower than the groups treated with ofloxacin and moxifloxacin only (Figure 3d); this might be due to the greater amount of dead bacteria after 4 h of treatment. Together, these results indicate that l-serine enhances the activity of ofloxacin and moxifloxacin against E. coli by increasing endogenous ROS production.
l-Serine potentiates ofloxacin and moxifloxacin against bacterial persister cells
Persister cells are a small fraction of a bacterial population that are extremely tolerant to antibiotics without undergoing genetic change. Unlike resistant cells, persister cells do not grow by entering into metabolically dormant states in the presence of antibiotics.44–46 Bactericidal antibiotics such as fluoroquinolones can kill slow-growing bacteria, but not persister cells.47,48 Based on the effects and mechanisms observed in normal growth E. coli cells after treatment with l-serine and drugs, we hypothesized that l-serine may also potentiate antibiotic activity against bacterial persister cells. Therefore, we explored the effect of l-serine on bacterial persisters in vitro. First, we wanted to know whether l-serine affects persister formation upon treatment with high antibiotic concentrations. To test this, l-serine was added at various timepoints during ofloxacin- and moxifloxacin-induced cell death and its killing kinetics was observed. We found that l-serine dramatically accelerated persister death regardless of the addition time (Figure 4a and b). Then, we investigated whether exposure of isolated persister cells to antimicrobials in the presence of l-serine led to a decrease in cell viability and, eventually, cell eradication. To confirm this hypothesis, we treated E. coli persister cells isolated from planktonic populations with 1 mg/L ofloxacin or 1 mg/L moxifloxacin at the concentrations indicated, in the presence or absence of 15 mM l-serine. We observed that the combination treatments exhibited greater bactericidal activity relative to the individual treatments with l-serine or either antibiotic (Figure 4c). Taken together, these results indicate that the addition of l-serine influences the number of persister cells isolated, and l-serine, in combination with ofloxacin or moxifloxacin, results in a reduction in numbers or eradication of persister cells.
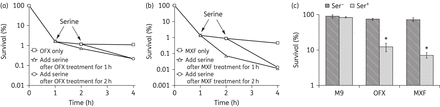
Killing persisters with the synergy of l-serine. l-Serine accelerated the persister cell death rate in stationary phase when preformed persisters were treated. Experiments were performed in the presence of 5 mg/L ofloxacin (a) or 5 mg/L moxifloxacin (b) alone and in combination with 15 mM l-serine. (c) Percentage survival of E. coli persisters in the presence or absence of l-serine plus ofloxacin or moxifloxacin. Values were compared with the control (without l-serine) at the same antibiotic concentration. Data are shown as mean ± SD of triplicate wells. Similar results were obtained in three independent experiments. *P < 0.05. OFX, ofloxacin; MXF, moxifloxacin.
Discussion
The threat of MDR bacteria demands countermeasures that are more effective. Combination of antibiotic or non-antibiotic adjuvants with antibiotics is an ideal therapeutic strategy for the treatment of bacterial infections.3,49–51 Here, we found that the combination of l-serine with fluoroquinolones can enhance the antimicrobial efficacy against E. coli by increasing the production of endogenous microbial ROS. This approach also can be used to combat MDR bacteria and eradicate persisters.
Fluoroquinolones have shown their efficacy in the treatment of Gram-positive and Gram-negative infections. The emergence of fluoroquinolone-resistant bacteria requires us to develop more judicious ways for fluoroquinolone usage to combat antibiotic resistance. We demonstrated that l-serine-mediated potentiation of ofloxacin and moxifloxacin is effective against E. coli (Figure 1). The synergistic effect between l-serine and the fluoroquinolones is attained when adding 15 mM for both metabolically active and persister cells. The bactericidal activity of some antibiotics is directly proportional to the growth rate of the bacteria and late-stationary-phase cultures are known to be more resistant.52–55 Previous study showed that ciprofloxacin can rapidly kill the organisms in both the logarithmic and stationary growth phases.56 Here, we found that l-serine potentiates ofloxacin and moxifloxacin to kill different growth-phase bacteria (Figure 1d and e). l-Serine is metabolized to pyruvate and further converted into acetyl-coenzyme A, which is the major upstream input for the TCA cycle. Addition of serine increased the NADH production, which may result from acceleration of the TCA cycle by l-serine.
In E. coli, gyrase inhibitors, such as fluoroquinolones, inducing the cell death pathway has been well established and indicates that ROS contribute to fluoroquinolone-mediated cell death.15,17 The present study demonstrates that l-serine increased the NAD+/NADH ratio (Figure 4a) and ferric iron content (Figure 4b). Directly blocking the harmful effects of iron by adding thiourea and BIP to l-serine and drug-treated cultures abolished the potentiation effects (Figure 4c). Hydroxyl radicals can be produced by the combination of the Haber–Weiss cycle35 and the Fenton reaction.21,36 In these reactions, ferrous ion reacts with oxygen to produce superoxide to produce hydrogen peroxide via the dismutation reaction. Hydrogen peroxide then reacts with ferrous ions to form hydroxyl radicals and ferric iron via the Fenton reaction. l-Serine increased ROS production in fluoroquinolone-treated cultures (Figure 4d) and improved fluoroquinolone lethality.
One of the key characteristics of persister cells is tolerance to antimicrobials, as persister cells escape killing by fluoroquinolones.47,48 Ofloxacin,57 norfloxacin58 and ciprofloxacin59 were usually used for the isolation of persisters. Moxifloxacin, one of the fourth generation of fluoroquinolones, is known to kill some subpopulations of persister cells.60,61 However, the killing kinetics in our study shows that moxifloxacin failed to eradicate persisters (Figure 4b). l-Serine dramatically reduced persistence frequency with fluoroquinolone antibiotics for E. coli (Figure 4a and b). Moreover, combination of l-serine with ofloxacin or moxifloxacin showed a better eradication of persisters (Figure 4c).
In conclusion, these results point to an original and alternative approach, which can potentiate the bactericidal effect of fluoroquinolones against drug-resistant bacteria by enhancing endogenous ROS production. Furthermore, this approach can reduce persistence during the growth of E. coli and can restore the susceptibility of isolated persister cells to antibiotics. Our findings imply that l-serine might be included as an adjuvant or potentiator of fluoroquinolones, to improve the control of chronic bacterial infections.
Funding
This work was supported by the National Natural Science Foundation (grant numbers 81511120001, 81371851, 81071316, 81271882 and 81301394), the New Century Excellent Talents in Universities (grant number NCET-11-0703), the National Megaprojects for Key Infectious Diseases (grant number 2008ZX10003-006), the Excellent PhD Thesis Fellowship of Southwest University (grant numbers kb2010017 and ky2011003), the Fundamental Research Funds for the Central Universities (grant numbers XDJK2016D025, XDJK2011D006, XDJK2012D011, XDJK2012D007, XDJK2013D003 and XDJK2014D040), the Graduate Research and Innovation Project of Graduate in Chongqing (grant number CYS14044), the Chongqing Municipal Committee of Education for Postgraduates Excellence Programme (grant number YJG123104) and the Undergraduates Teaching Reform Programme (grant number 2013JY201) to J. X.
Transparency declarations
None to declare.
Author contributions
X. D., X. H., X. W., S. Y., S. G., A. E. A. and C. H. performed the experiments. X. D. analysed the data. X. D. and J. X. designed the study and wrote the paper. All of the authors read and approved the manuscript.
Acknowledgements
We thank Dr Huzhi Zheng, School of Chemistry and Chemical Engineering, Southwest University, for providing the Tecan Infinite® 200 PRO microplate reader used in this study.
References