-
PDF
- Split View
-
Views
-
Cite
Cite
A. Petitcollin, P.-F. Dequin, F. Darrouzain, L. Vecellio, T. Boulain, D. Garot, G. Paintaud, D. Ternant, S. Ehrmann, Pharmacokinetics of high-dose nebulized amikacin in ventilated critically ill patients, Journal of Antimicrobial Chemotherapy, Volume 71, Issue 12, 1 December 2016, Pages 3482–3486, https://doi.org/10.1093/jac/dkw313
- Share Icon Share
Antibiotic nebulization theoretically allows the delivery of high doses to the lungs together with limited systemic exposure and toxicity. This study aimed to describe amikacin pharmacokinetics, and especially its absorption, in patients treated with high-dose nebulized amikacin.
Twenty critically ill patients experiencing ventilator-associated pneumonia received a 20 mg/kg infusion of amikacin, followed by either three other infusions or three nebulizations of 60 mg/kg amikacin. An extensive sampling regimen allowed measurement of amikacin serum concentrations at 0.5, 1, 1.5, 2, 3, 4, 6, 10 and 24 h after each administration. Amikacin pharmacokinetics was studied by population compartmental modelling.
Amikacin pharmacokinetics was best described using a two-compartment structural model with first-order distribution and elimination, in which lung absorption was described using a transit model. Estimated means (interindividual variability) of the main parameters were: bioavailability F = 2.65% (22.1%); transit compartments n = 1.58 (fixed); transit constant ktr = 1.38 h−1 (33.4%); central volume Vc = 10.2 L (10.5%); and elimination constant k10 = 0.488 h−1 (35.8%). The addition of interoccasion variability on F (44.0%) and k10 (41.7%) allowed the description of intraindividual variability of bioavailability and elimination. Amikacin clearance was positively correlated with baseline creatinine clearance.
Our pharmacokinetic model provided an accurate description of amikacin concentrations following nebulization. There was wide interindividual and interoccasion variability in the absorption and elimination of amikacin. Nevertheless, systemic exposure after nebulization was always much lower than after infusion, an observation suggesting that nebulized high doses are safe in this regard and may be used to treat ventilator-associated pneumonia.
Introduction
Amikacin is an aminoglycoside widely used in ICUs to treat patients with ventilator-associated pneumonia because of its activity against Gram-negative bacteria.1 Amikacin exhibits a concentration-dependent effect justifying the use of high doses to enhance efficacy; however, it is limited by its ototoxicity and nephrotoxicity.2 Aminoglycosides also exhibit relatively poor lung penetration after intravenous administration,3,4 which combined with lower peak concentrations due to an increased distribution volume in ICU patients5,6 results in low lung concentrations, even though MDR bacteria with high MIC values are increasingly common. The efficacy of aerosolized antibiotics to treat ventilator-associated pneumonia is supported by studies showing lower systemic toxicity, reduced emergence of bacterial resistance and enhanced activity against resistant bacteria.7,8 Therefore, amikacin aerosol administration theoretically combines the advantages of in situ high-dose delivery and limited absorption from the lungs.
Only a few non-compartmental studies have explored the pharmacokinetics of nebulized amikacin in ICU patients with Gram-negative pneumonia. They reported notably, aside from high intratracheal concentrations, highly variable serum concentrations.8–11 The present study aimed to describe high-dose nebulized amikacin pharmacokinetics with a population approach, especially its absorption kinetics and systemic exposure as compared with the intravenous route in ICU patients with ventilator-associated bacterial pneumonia.
Patients and methods
Ethics
This study was conducted in accordance with the Declaration of Helsinki and national and institutional standards. It was approved by the local institutional review board (Comité de Protection des Personnes, Tours, France: EudraCT 2008-000248-15, A80308-54) and all patients or their authorized representative provided informed consent (NCT00861315).
Patients and study design
The study included 20 ICU patients with ventilator-associated pneumonia. Patients ventilated through an endotracheal tube were included in case of suspicion of or confirmed amikacin-susceptible Gram-negative ventilator-associated, hospital-acquired or healthcare-associated pneumonia.12 Patients with BMI >30 kg/m2 or renal impairment (Cockcroft's creatinine clearance <30 mL/min or urine output <0.5 mL/kg/h) were not included. Each patient received either four 1 h intravenous infusions of 20 mg/kg amikacin or one infusion followed by three 60 mg/kg nebulizations at 0, 24, 48 and 72 h. Nebulization was performed using a jet nebulizer run with 6 L/min air/oxygen mixture provided by the ventilator (Atomisor NL9M; La Diffusion Technique Française, Saint-Etienne, France). Nebulization was continued until the whole dose was administered (nebulizer sputtering). Patients in whom amikacin-susceptible Gram-negative pneumonia was not confirmed or who developed acute kidney injury did not receive further amikacin within the study (analysis being restricted to less than four amikacin administrations).
Blood samples were drawn just before and at 0.5, 1, 1.5, 2, 3, 4, 6, 10 and 24 h after each amikacin administration. Additional information about inclusion criteria and nebulization setup are provided in Appendix 1 and 2, available as Supplementary data at JAC Online, respectively, and the amikacin assay is detailed in Appendix 3 (all available as Supplementary data at JAC Online).
Pharmacokinetic analysis
Population pharmacokinetic compartmental modelling was performed using Monolix 4.2.3 (Lixoft, Orsay, France).
Structural pharmacokinetic model
Concentration values lower than the lower limit of quantification (LLOQ) were censored (considered to be between 0 and the LLOQ). One-, two- and three-compartment mammillary models with first-order distribution and first-order and/or non-linear elimination were tested.
Interindividual model
Interoccasion model
Error model
Description of the absorption model
Covariates
The influence of the following covariates on amikacin pharmacokinetics was tested:
where θCAT=0 is the value of θTV in an arbitrary reference category and βCAT=i quantifies the influence of the ith category on the value of θTV.
Model comparison and covariate selection
Structural, interindividual, interoccasion, residual error and covariate models were compared using the value of the objective function (OFV) defined as −2 ln-likelihood (−2LL) and Akaike's information criterion (AIC) defined as AIC = OFV + 2p. From pairs of tested models, the one with the lowest OFV (or AIC) was chosen. This was based on the likelihood ratio test in which the difference in OFV between two models (ΔOFV) is assumed to follow a χ2 distribution. Thus, a difference in the OFV of 3.84 was considered significant at a risk α of 5%.
Evaluation of the goodness of fit and final model selection
The goodness of fit was assessed for each model by plotting population-predicted (PRED) and individually predicted (IPRED) concentrations versus observed concentrations (OBS) and by evaluating the residuals by graphical inspection of normalized prediction distribution errors (NPDE) versus time and NPDE distribution. Individual fits were also inspected. The model offering the greater reduction of AIC together with acceptable precision of the estimations of the parameters and goodness of fit was selected. A visual predictive check figure showing the 90% CI of the predictions and observations was built to ensure the predictive performance of the model (Figure S3).
Evaluation of the exposure
All AUC values reported in the article are AUC0–24, calculated from the beginning of the administration to 24 h.
Results
Characteristic . | Value . |
---|---|
Patients | |
age (years) | 57 (20–80) |
males (%) | 18 (90) |
body weight (kg) | 67 (50–84) |
serum creatinine (μmol/L) | 61 (31–122) |
creatinine clearance (mL/min) | 114 (50–254) |
SAPS II score | 42 (27–82) |
Treatments | |
total number of nebulizations (%) | 32 (51.6) |
median nebulization dose (mg) | 4560 (3250–5520) |
patients receiving nebulization | 12/20 |
3 nebulizations | 8/12 |
2 nebulizations | 4/12 |
total number of infusions (%) | 30 (48.4) |
median infusion dose (mg) | 1480 (1100–1840) |
4 infusions | 3/8 |
2 infusions | 1/8 |
1 infusion | 4/8 |
Data | |
total number of blood samples | 522 |
number of samples per patient | 33 (11–45) |
number of censored values (%) | 14 (2.5) |
Characteristic . | Value . |
---|---|
Patients | |
age (years) | 57 (20–80) |
males (%) | 18 (90) |
body weight (kg) | 67 (50–84) |
serum creatinine (μmol/L) | 61 (31–122) |
creatinine clearance (mL/min) | 114 (50–254) |
SAPS II score | 42 (27–82) |
Treatments | |
total number of nebulizations (%) | 32 (51.6) |
median nebulization dose (mg) | 4560 (3250–5520) |
patients receiving nebulization | 12/20 |
3 nebulizations | 8/12 |
2 nebulizations | 4/12 |
total number of infusions (%) | 30 (48.4) |
median infusion dose (mg) | 1480 (1100–1840) |
4 infusions | 3/8 |
2 infusions | 1/8 |
1 infusion | 4/8 |
Data | |
total number of blood samples | 522 |
number of samples per patient | 33 (11–45) |
number of censored values (%) | 14 (2.5) |
SAPS, Simplified Acute Physiology Score.
Data are expressed as median (min–max) where appropriate. Values were censored if below the LLOQ (0.3 mg/L).
Characteristic . | Value . |
---|---|
Patients | |
age (years) | 57 (20–80) |
males (%) | 18 (90) |
body weight (kg) | 67 (50–84) |
serum creatinine (μmol/L) | 61 (31–122) |
creatinine clearance (mL/min) | 114 (50–254) |
SAPS II score | 42 (27–82) |
Treatments | |
total number of nebulizations (%) | 32 (51.6) |
median nebulization dose (mg) | 4560 (3250–5520) |
patients receiving nebulization | 12/20 |
3 nebulizations | 8/12 |
2 nebulizations | 4/12 |
total number of infusions (%) | 30 (48.4) |
median infusion dose (mg) | 1480 (1100–1840) |
4 infusions | 3/8 |
2 infusions | 1/8 |
1 infusion | 4/8 |
Data | |
total number of blood samples | 522 |
number of samples per patient | 33 (11–45) |
number of censored values (%) | 14 (2.5) |
Characteristic . | Value . |
---|---|
Patients | |
age (years) | 57 (20–80) |
males (%) | 18 (90) |
body weight (kg) | 67 (50–84) |
serum creatinine (μmol/L) | 61 (31–122) |
creatinine clearance (mL/min) | 114 (50–254) |
SAPS II score | 42 (27–82) |
Treatments | |
total number of nebulizations (%) | 32 (51.6) |
median nebulization dose (mg) | 4560 (3250–5520) |
patients receiving nebulization | 12/20 |
3 nebulizations | 8/12 |
2 nebulizations | 4/12 |
total number of infusions (%) | 30 (48.4) |
median infusion dose (mg) | 1480 (1100–1840) |
4 infusions | 3/8 |
2 infusions | 1/8 |
1 infusion | 4/8 |
Data | |
total number of blood samples | 522 |
number of samples per patient | 33 (11–45) |
number of censored values (%) | 14 (2.5) |
SAPS, Simplified Acute Physiology Score.
Data are expressed as median (min–max) where appropriate. Values were censored if below the LLOQ (0.3 mg/L).
Parameter . | Value . | RSE (%) . |
---|---|---|
Fixed effects | ||
F | 0.0265 | 15 |
n | 1.58 | 4 |
ktr (h−1) | 1.38 | 10 |
Vc (L) | 10.2 | 3 |
k10 (h−1) | 0.488 | 8 |
βCL on k10 | 0.568 | 34 |
k12 (h−1) | 1.26 | 1 |
k21 (h−1) | 0.858 | 6 |
Random effects | ||
ωF (%) | 22.1 | 20 |
ωktr (%) | 33.4 | 24 |
ωVc (%) | 10.5 | 23 |
ωk10 (%) | 35.8 | 16 |
ωk21 (%) | 23.8 | 22 |
γF (%) | 44.0 | 12 |
γk10 (%) | 41.7 | 14 |
Residual error | ||
σadd (mg/L) | 0.214 | 8 |
σprop (%) | 10.9 | 5 |
Parameter . | Value . | RSE (%) . |
---|---|---|
Fixed effects | ||
F | 0.0265 | 15 |
n | 1.58 | 4 |
ktr (h−1) | 1.38 | 10 |
Vc (L) | 10.2 | 3 |
k10 (h−1) | 0.488 | 8 |
βCL on k10 | 0.568 | 34 |
k12 (h−1) | 1.26 | 1 |
k21 (h−1) | 0.858 | 6 |
Random effects | ||
ωF (%) | 22.1 | 20 |
ωktr (%) | 33.4 | 24 |
ωVc (%) | 10.5 | 23 |
ωk10 (%) | 35.8 | 16 |
ωk21 (%) | 23.8 | 22 |
γF (%) | 44.0 | 12 |
γk10 (%) | 41.7 | 14 |
Residual error | ||
σadd (mg/L) | 0.214 | 8 |
σprop (%) | 10.9 | 5 |
RSE, relative standard error; F, bioavailability; n, number of transit compartments; ktr, transit constant; Vc, central volume of distribution; k10, elimination constant; k12, transfer constant from the central to the peripheral compartment; k21, transfer constant from the peripheral to the central compartment; ω, interindividual variability; γ, interoccasion variability; σadd, additive residual error; σprop, proportional residual error. Post hoc typical values: central clearance CL = 4.98 L/h; peripheral volume V2 = 14.98 L; and peripheral clearance Q = 12.85 L/h.
Parameter . | Value . | RSE (%) . |
---|---|---|
Fixed effects | ||
F | 0.0265 | 15 |
n | 1.58 | 4 |
ktr (h−1) | 1.38 | 10 |
Vc (L) | 10.2 | 3 |
k10 (h−1) | 0.488 | 8 |
βCL on k10 | 0.568 | 34 |
k12 (h−1) | 1.26 | 1 |
k21 (h−1) | 0.858 | 6 |
Random effects | ||
ωF (%) | 22.1 | 20 |
ωktr (%) | 33.4 | 24 |
ωVc (%) | 10.5 | 23 |
ωk10 (%) | 35.8 | 16 |
ωk21 (%) | 23.8 | 22 |
γF (%) | 44.0 | 12 |
γk10 (%) | 41.7 | 14 |
Residual error | ||
σadd (mg/L) | 0.214 | 8 |
σprop (%) | 10.9 | 5 |
Parameter . | Value . | RSE (%) . |
---|---|---|
Fixed effects | ||
F | 0.0265 | 15 |
n | 1.58 | 4 |
ktr (h−1) | 1.38 | 10 |
Vc (L) | 10.2 | 3 |
k10 (h−1) | 0.488 | 8 |
βCL on k10 | 0.568 | 34 |
k12 (h−1) | 1.26 | 1 |
k21 (h−1) | 0.858 | 6 |
Random effects | ||
ωF (%) | 22.1 | 20 |
ωktr (%) | 33.4 | 24 |
ωVc (%) | 10.5 | 23 |
ωk10 (%) | 35.8 | 16 |
ωk21 (%) | 23.8 | 22 |
γF (%) | 44.0 | 12 |
γk10 (%) | 41.7 | 14 |
Residual error | ||
σadd (mg/L) | 0.214 | 8 |
σprop (%) | 10.9 | 5 |
RSE, relative standard error; F, bioavailability; n, number of transit compartments; ktr, transit constant; Vc, central volume of distribution; k10, elimination constant; k12, transfer constant from the central to the peripheral compartment; k21, transfer constant from the peripheral to the central compartment; ω, interindividual variability; γ, interoccasion variability; σadd, additive residual error; σprop, proportional residual error. Post hoc typical values: central clearance CL = 4.98 L/h; peripheral volume V2 = 14.98 L; and peripheral clearance Q = 12.85 L/h.
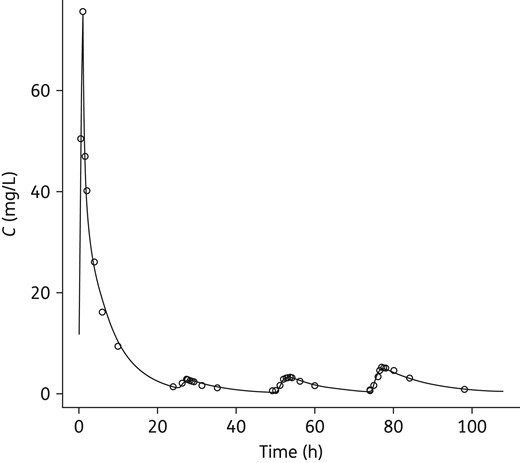
Representative individual fit. The patient received one infusion followed by three amikacin nebulizations. The line is the individually predicted amikacin concentration value and open circles are observed values. The addition of interoccasion variability on F allowed proper description of the variability of the lung absorption process. However, there was no identifiable pattern of evolution of the bioavailability during the treatment (see Figure S4).
Discussion
Amikacin pharmacokinetics was described using a two-compartment model, with a central volume of distribution of 10.2 L and clearance of 4.98 L/h, which is consistent with previously published models.13–15 The serum peak concentration after nebulization was variable between subjects, but remained low with a median (min–max) value of 3.1 (1.1–9.2) mg/L. The time to reach the peak concentration was also variable, with a value of 2.2 (1.4–4.4) h. The bioavailability was lower than that reported in healthy volunteers16 with a typical value of 2.7% and its estimated interindividual variability was 22%. This is consistent with former non-compartmental studies,9–11 which reported large standard deviations of serum concentrations of amikacin after nebulization in ICU patients. In addition, there was important intraindividual variability in the maximal concentrations after nebulization and in the elimination rate, whatever the route of administration, which were modelled by adding an interoccasion model on bioavailability and the elimination constant, respectively. Systemic exposure was consequently highly variable, but the nebulization AUC with a value of 23.2 (6.1–86.7) mg · h/L was always lower than the minimal intravenous exposure (126.4 mg · h/L). Additionally, there was no apparent accumulation of amikacin in the course of multiple nebulizations (Figure S4).
The interoccasion variability of the bioavailability may be partly explained by modifications of the airway properties and of the respiratory pattern due to progressive healing during the treatment. This is relevant because pneumonia was shown experimentally to lead to a modified absorption profile.17 Heterogeneity of lung deposition may also be an explanation. Lung deposition and consequently amikacin absorption may be influenced by the ventilator settings, which can influence nebulization, particle size and depth of pulmonary drug delivery. This study was performed with a specific jet nebulizer positioned in the inspiratory limb immediately before the Y piece and the results may therefore not be fully transposed to other settings. However, jet nebulizers remain the most frequently used device to deliver aerosols in ventilated patients.18 Of note, the value of bioavailability is influenced by the nebulizer output and thus it is underestimated, because it reflects the fraction absorbed from the total dose put in the nebulizer, not from the fraction of the dose that actually reaches the absorption site. In this regard, the estimated bioavailability might be higher with devices other than jet nebulizers due to a higher output. Heterogeneous lung delivery, combined with great variability of absorption depending both on the site of deposition (alveolar or bronchial) and its state (healthy or pathological), can probably explain the observed interoccasion variability. In addition, the time to the peak and its variability may be dependent on sputum mucin binding of amikacin, which may delay absorption and is also susceptible to variability. These elements suggest it could be interesting to improve the nebulization technology in ventilated patients, in order to ensure optimal, homogeneous drug delivery. This issue is particularly relevant considering concentration-dependent antibiotics because heterogeneity of lung deposition could lead to local areas with concentrations insufficient to reach optimal bactericidal activity.
The terminal elimination half-life was median 1.6 h (range 0.5–3.7) and did not differ whether amikacin was nebulized or infused. Amikacin clearance was linked to renal function. A decrease of 20 mL/min in creatinine clearance resulted in a 10.4% decrease in amikacin clearance. Interestingly, though baseline creatinine clearance partly explained the interindividual variability of amikacin clearance, its changes at each different occasion did not explain interoccasion variability, which may be due to other factors that were not addressed here. Modifications of the ventilation settings may also have had an influence on amikacin elimination that was not assessed, though it probably existed. For example, it is known that mechanical ventilation with positive end-expiratory pressure is associated with a decrease in the glomerular filtration rate and an increase in tubular reabsorption.19
Body weight and gender were unexpectedly not significant covariates of amikacin central volume of distribution or clearance, probably because of the small sample size and its relative homogeneity. Notably, the difficulty in relying solely on body weight to efficiently explain interindividual changes in the central volume was underlined previously.20
The low number of patients did not permit analysis of the influence of ventilator settings and other factors such as coadministration of fluids on amikacin pharmacokinetics, especially the intrapatient variability of absorption and elimination, because there were too many parameters to test. However, our results demonstrate that systemic exposure remains at acceptable limits regarding the risk of systemic toxicity with standard ventilator settings.
Conclusions
Despite wide inter- and intraindividual pharmacokinetic variability, the low bioavailability of nebulized amikacin allows multiple administrations of high doses up to 60 mg/kg with a jet nebulizer in order to increase the success rate of the treatment as compared with the intravenous route, with a controlled risk of systemic toxicity as suggested by the low systemic exposure, which is generally used as an indicator of the risk of toxicity of aminoglycosides.
Funding
This work was supported by the Association pour la Promotion à Tours de la Réanimation Médicale (APTRM).
Transparency declarations
L. V. is an employee of La Diffusion Technique Française. G. P. reports grants from Novartis, grants from Roche Pharma, grants from Genzyme, grants from Servier, grants from BMS, grants from Pfizer, grants from Chugai, outside the submitted work. S. E. reports grants and non-financial support from Aerogen, non-financial support from Axess Vision Technology, grants and non-financial support from Fisher & Paykel, non-financial support from La Diffusion Technique Française, non-financial support from Penn-Century, during the conduct of the study. All other authors have nothing to declare.
Author contributions
S. E., P.-F. D, L. V., G. P. and D. T. designed the study. S. E., T. B. and D. G. provided medical care to the study patients and were in charge of their recruitment into the study and blood sample collection. A. P. and F. D. performed amikacin concentration measurements. A. P. and D. T. performed statistical and pharmacokinetic analysis. A. P., S. E., D. T. and G. P. wrote the manuscript. All authors revised the manuscript for important intellectual content and approved the manuscript in its submitted form.
Acknowledgements
The authors sincerely thank all physicians and nurses who were involved in patient care and study recruitment. In particular, they sincerely thank Christine Mabilat, Aurélie Aubrey, Véronique Simeon-Vieules, Katty Guinoiseau, Penelope Vanault-Hodges and Chantal Brossard, research nurses of the study.
References