-
PDF
- Split View
-
Views
-
Cite
Cite
Satheesh Nair, Philip Ashton, Michel Doumith, Steve Connell, Anais Painset, Solomon Mwaigwisya, Gemma Langridge, Elizabeth de Pinna, Gauri Godbole, Martin Day, WGS for surveillance of antimicrobial resistance: a pilot study to detect the prevalence and mechanism of resistance to azithromycin in a UK population of non-typhoidal Salmonella, Journal of Antimicrobial Chemotherapy, Volume 71, Issue 12, 1 December 2016, Pages 3400–3408, https://doi.org/10.1093/jac/dkw318
- Share Icon Share
WGS and phenotypic methods were used to determine the prevalence of azithromycin resistance in Salmonella enterica isolates from the UK and to identify the underlying mechanisms of resistance.
WGS by Illumina HiSeq was carried out on 683 Salmonella spp. isolates. Known genes associated with azithromycin resistance were detected by WGS using a mapping-based approach. Macrolide resistance determinants were identified and the genomic context of these elements was assessed by various bioinformatics tools. Susceptibility testing was in accordance with EUCAST methodology (MIC ≤16 mg/L).
Fifteen isolates of non-typhoidal Salmonella enterica belonging to serovars Salmonella Blockley, Salmonella Typhimurium, Salmonella Thompson, Salmonella Ridge and Salmonella Kentucky showed resistance or decreased susceptibility to azithromycin (from 6 to >16 mg/L) due to the presence of macrolide resistance genes mphA, mphB or mefB. These genes were either plasmid or chromosomally mediated. Azithromycin-resistant Salmonella Blockley isolates harboured a macrolide inactivation gene cluster, mphA-mrx-mphr(A), within a novel Salmonella azithromycin resistance genomic island (SARGI) determined by MinION sequencing. This is the first known chromosomally mediated mphA gene cluster described in salmonellae. Phylogenetic analysis and epidemiological information showed that mphA Salmonella Blockley isolates were not derived from a single epidemiologically related event. The azithromycin MICs of the 15 Salmonella spp. isolates showed that the presence of the mphA gene was associated with MIC ≥16 mg/L, while the presence of mefB or mphB was not.
Azithromycin resistance due to acquisition of known macrolide resistance genes was seen in four different Salmonella serovars and can be either plasmid-encoded or chromosomally encoded.
Introduction
The increased resistance to a broad range of antibiotics in both Salmonella strains that cause enteric fever and non-typhoidal Salmonella (NTS) is an emerging threat.1–8 Widespread resistance to amoxicillin, chloramphenicol, trimethoprim/sulfamethoxazole and fluoroquinolones has led to azithromycin being used as the preferred antimicrobial agent to treat cases of uncomplicated enteric fever reporting travel to the Indian subcontinent and South-East Asia.4 It is also used to treat infections with MDR NTS in vulnerable patients who have prolonged or invasive infections.7 Azithromycin is an azalide that has excellent tissue penetration, concentrates in the reticuloendothelial cells and has the advantage of oral administration and a long half-life. Clinical trials have shown it to be equivalent or superior to chloramphenicol, fluoroquinolones and third-generation cephalosporins for the management of uncomplicated typhoid fever.9–11 However, reports are emerging of azithromycin resistance in cases of enteric fever as well as invasive NTS infection.1,10–12
Acquired resistance to macrolides/azalides may be caused by several different mechanisms.13 They include: (i) target site modification by methylases encoded by erm genes;14,15 (ii) modifying enzymes such as esterases encoded by ereA and B genes or phosphotransferases encoded by mphA, B and D genes;16,17 (iii) efflux pumps, e.g. mefA and msrA found mainly in Gram-positive bacteria, with mefA also identified in Gram-negative strains;15 (iv) mutations in the rrl and rpl genes encoding ribosomal proteins L22, L4 and 23S rRNA, which also confer resistance in Gram-positive bacteria.18 The presence of more than one of the above mentioned genes can confer full cross-resistance between erythromycin and azithromycin.14
The Gastrointestinal Bacteria Reference Unit (GBRU) of PHE is the national reference laboratory for Salmonella in England and Wales. Each year, ∼10 000 isolates are referred to the Salmonella Reference Service (SRS). WGS is currently used as the primary test for identification and typing of isolates received by SRS (http://biorxiv.org/content/early/2015/11/29/033225.abstract).19 These isolates are also tested phenotypically for resistance to a wide range of antimicrobial agents.
EUCAST is responsible for defining clinical breakpoints for new and existing drugs within the European Union and affiliated nations. Currently, no clinical breakpoints for azithromycin have been defined for Enterobacteriaceae, including Salmonella, by either the CLSI or EUCAST, leading to delays in early detection of azithromycin resistance. However, the epidemiological cut-off for azithromycin has been accepted as ≤16 mg/L for Salmonella enterica.11,20
The recent advancement in WGS technologies for routine microbiology is well documented.21 Sequence data allow rapid identification of Salmonella serotypes by MLST, as proposed by Achtman et al. in 2012.22 In addition, availability of the whole genome sequences allows in silico prediction of antimicrobial resistance, but the prediction should be validated by phenotypic antimicrobial testing prior to being applied.23–25 Here, we used available WGS data to determine the prevalence and underlying mechanisms of azithromycin resistance among Salmonella in the UK.
Methods
Bacterial isolates and phenotypic typing
Six hundred and sixty-seven Salmonella isolates from 2012 that were part of a 6 month (April–September 2013) WGS validation project were selected for this retrospective study (nine isolates shown in Table 1). A further 16 Salmonella Blockley isolates from 2012–15 were used as comparators for phylogenetic analysis (Table S1, available as Supplementary data at JAC Online). Selected isolates were identified and confirmed by serotyping and/or phage typing.26,27
Epidemiological features and analysis of azithromycin resistance mechanisms detected by phenotypic (MIC), genotypic (WGS) and molecular (PCR) methods in nine non-typhoidal Salmonella enterica isolates
ID . | Age . | Sex . | Travel . | Origin of isolate . | Serotype . | MLST . | Azithromycin resistance gene (WGS) . | Other resistance genes (WGS) . | Phenotypic resistance profile . | . | |||
---|---|---|---|---|---|---|---|---|---|---|---|---|---|
. | . | . | . | . | . | . | . | . | AZM MIC . | CIP MIC >0.064 mg/L . | CIP MIC >0.5 mg/L . | other antibiotics . | β-Lactamase (PCR) . |
SAL1 | 28 | M | nil | faeces | Thompson | 26 | mphA | aac-lb-cr; aac-ly; aadA16; qnrB6; arr-3; sul3; dfrA27; sul1 | 16 | >0.064 | SUL-TMP | ||
SAL2 | 67 | M | nil | urine | Typhimurium | 19 | mphB | aac-Iaa; aph-Id; TEM-98,TEM-1; sul3; sul2; tet(A)-1; dfrA1 | 6 | <0.064 | AMP-SUL-STR-TET-TMP | ||
SAL3 | 58 | F | nil | faeces | Typhimurium | 34 | mphA | aac-IId; aac-Iaa; aadA17; aph-ld; TEM-98, TEM-1; qnrS1; lnu(F); arr-2; sul2; tet(A); dfrA5; dfrA14; dfrA25 | 96 | >0.064 | >0.5 | AMP-SUL-GEN-TOB-STR-TET-TMP-NAL-FOX | |
SAL4 | 79 | M | nil | faeces | Typhimurium | 34 | mefB | aac-Iaa; aadA2; aph-Id; TEM-98, TEM-1; sul2; tet(A); dfrA12 | 8 | <0.064 | AMP-SUL-STR-TET-TMP | ||
SAL5 | 58 | M | nil | faeces | Ridge | novel ST | mphA | aac-IIa; aac-Iy; aph-Id; TEM-98, TEM-1; sul2; dfrA14; tet(A)-1 | 16 | <0.064 | AMP-SUL-GEN-TOB-STR-TET-TMP | ||
H123740558 | 5 | M | nil | faeces | Blockley | 52 | mphA | aac-Iy; aph-Id; tet(A)-1 | 24 | >0.064 | >0.5 | STR-TET-NAL | |
H123780513 | 78 | F | not known | faeces | Blockley | 52 | mphA | aac-Iy; tet(A)-1 | 24 | >0.064 | >0.5 | TET-NAL | |
H124040535 | 59 | F | nil | faeces | Blockley | 52 | mphA | aac-Iy; aph-Id; tet(A)-1 | 48 | >0.064 | STR-TET-NAL | ||
SAL6 | 38 | F | Egypt | faeces | Kentucky | 198 | mphB | aac-Ie; aac-Ib; aac(6′)-Iaa; aadA7; aph-Id; CMY-2; XA-10; TEM-98, TEM-1; cmlA1; sul3; tet(A)-1; aadA1; floR | 8 | >0.064 | >0.5 | AMP-SUL-GEN-TOB-STR-TET-NAL-CAZ2-CTX1-FOX | AmpC blaCMY-2 |
ID . | Age . | Sex . | Travel . | Origin of isolate . | Serotype . | MLST . | Azithromycin resistance gene (WGS) . | Other resistance genes (WGS) . | Phenotypic resistance profile . | . | |||
---|---|---|---|---|---|---|---|---|---|---|---|---|---|
. | . | . | . | . | . | . | . | . | AZM MIC . | CIP MIC >0.064 mg/L . | CIP MIC >0.5 mg/L . | other antibiotics . | β-Lactamase (PCR) . |
SAL1 | 28 | M | nil | faeces | Thompson | 26 | mphA | aac-lb-cr; aac-ly; aadA16; qnrB6; arr-3; sul3; dfrA27; sul1 | 16 | >0.064 | SUL-TMP | ||
SAL2 | 67 | M | nil | urine | Typhimurium | 19 | mphB | aac-Iaa; aph-Id; TEM-98,TEM-1; sul3; sul2; tet(A)-1; dfrA1 | 6 | <0.064 | AMP-SUL-STR-TET-TMP | ||
SAL3 | 58 | F | nil | faeces | Typhimurium | 34 | mphA | aac-IId; aac-Iaa; aadA17; aph-ld; TEM-98, TEM-1; qnrS1; lnu(F); arr-2; sul2; tet(A); dfrA5; dfrA14; dfrA25 | 96 | >0.064 | >0.5 | AMP-SUL-GEN-TOB-STR-TET-TMP-NAL-FOX | |
SAL4 | 79 | M | nil | faeces | Typhimurium | 34 | mefB | aac-Iaa; aadA2; aph-Id; TEM-98, TEM-1; sul2; tet(A); dfrA12 | 8 | <0.064 | AMP-SUL-STR-TET-TMP | ||
SAL5 | 58 | M | nil | faeces | Ridge | novel ST | mphA | aac-IIa; aac-Iy; aph-Id; TEM-98, TEM-1; sul2; dfrA14; tet(A)-1 | 16 | <0.064 | AMP-SUL-GEN-TOB-STR-TET-TMP | ||
H123740558 | 5 | M | nil | faeces | Blockley | 52 | mphA | aac-Iy; aph-Id; tet(A)-1 | 24 | >0.064 | >0.5 | STR-TET-NAL | |
H123780513 | 78 | F | not known | faeces | Blockley | 52 | mphA | aac-Iy; tet(A)-1 | 24 | >0.064 | >0.5 | TET-NAL | |
H124040535 | 59 | F | nil | faeces | Blockley | 52 | mphA | aac-Iy; aph-Id; tet(A)-1 | 48 | >0.064 | STR-TET-NAL | ||
SAL6 | 38 | F | Egypt | faeces | Kentucky | 198 | mphB | aac-Ie; aac-Ib; aac(6′)-Iaa; aadA7; aph-Id; CMY-2; XA-10; TEM-98, TEM-1; cmlA1; sul3; tet(A)-1; aadA1; floR | 8 | >0.064 | >0.5 | AMP-SUL-GEN-TOB-STR-TET-NAL-CAZ2-CTX1-FOX | AmpC blaCMY-2 |
AMP, ampicillin; CHL, chloramphenicol; SUL, sulphonamide; GEN, gentamicin; TOB, tobramycin; STR, streptomycin; TET, tetracycline; TMP, trimethoprim; NAL, nalidixic acid; CIP, ciprofloxacin; CAZ, ceftazidime; CTX, cefotaxime; FOX, cefoxitin; AZM, azithromycin.
Breakpoints for antibiotics: ampicillin 8 mg/L, chloramphenicol 8 and 16 mg/L, sulphonamide 256 mg/L, gentamicin 2 mg/L, tobramycin 8 mg/L, streptomycin 16 mg/L, tetracycline 8 mg/L, trimethoprim 2 mg/L, nalidixic acid 16 mg/L, ciprofloxacin 0.064 and 0.5 mg/L, ceftazidime 1 and 2 mg/L, cefotaxime 0.5 and 1 mg/L, cefoxitin 8 mg/L, azithromycin 16 mg/L.
Resistance genes: aac, aph, aad (aminoglycosides), qnrB6/S1 (quinolone), arr (rifampicin), sul (sulphonamide), dfr (trimethoprim), TEM-1 (β-lactam, ampicillin), TEM-98 (β-lactam, ampicillin), tet (tetracycline), lnu (oxazolidinone), CMY-2 (Amp C β-lactam), OXA-10 (β-lactam), cmlA1 (chloramphenicol), floR (chloramphenicol), mph, mef (macrolide).
Epidemiological features and analysis of azithromycin resistance mechanisms detected by phenotypic (MIC), genotypic (WGS) and molecular (PCR) methods in nine non-typhoidal Salmonella enterica isolates
ID . | Age . | Sex . | Travel . | Origin of isolate . | Serotype . | MLST . | Azithromycin resistance gene (WGS) . | Other resistance genes (WGS) . | Phenotypic resistance profile . | . | |||
---|---|---|---|---|---|---|---|---|---|---|---|---|---|
. | . | . | . | . | . | . | . | . | AZM MIC . | CIP MIC >0.064 mg/L . | CIP MIC >0.5 mg/L . | other antibiotics . | β-Lactamase (PCR) . |
SAL1 | 28 | M | nil | faeces | Thompson | 26 | mphA | aac-lb-cr; aac-ly; aadA16; qnrB6; arr-3; sul3; dfrA27; sul1 | 16 | >0.064 | SUL-TMP | ||
SAL2 | 67 | M | nil | urine | Typhimurium | 19 | mphB | aac-Iaa; aph-Id; TEM-98,TEM-1; sul3; sul2; tet(A)-1; dfrA1 | 6 | <0.064 | AMP-SUL-STR-TET-TMP | ||
SAL3 | 58 | F | nil | faeces | Typhimurium | 34 | mphA | aac-IId; aac-Iaa; aadA17; aph-ld; TEM-98, TEM-1; qnrS1; lnu(F); arr-2; sul2; tet(A); dfrA5; dfrA14; dfrA25 | 96 | >0.064 | >0.5 | AMP-SUL-GEN-TOB-STR-TET-TMP-NAL-FOX | |
SAL4 | 79 | M | nil | faeces | Typhimurium | 34 | mefB | aac-Iaa; aadA2; aph-Id; TEM-98, TEM-1; sul2; tet(A); dfrA12 | 8 | <0.064 | AMP-SUL-STR-TET-TMP | ||
SAL5 | 58 | M | nil | faeces | Ridge | novel ST | mphA | aac-IIa; aac-Iy; aph-Id; TEM-98, TEM-1; sul2; dfrA14; tet(A)-1 | 16 | <0.064 | AMP-SUL-GEN-TOB-STR-TET-TMP | ||
H123740558 | 5 | M | nil | faeces | Blockley | 52 | mphA | aac-Iy; aph-Id; tet(A)-1 | 24 | >0.064 | >0.5 | STR-TET-NAL | |
H123780513 | 78 | F | not known | faeces | Blockley | 52 | mphA | aac-Iy; tet(A)-1 | 24 | >0.064 | >0.5 | TET-NAL | |
H124040535 | 59 | F | nil | faeces | Blockley | 52 | mphA | aac-Iy; aph-Id; tet(A)-1 | 48 | >0.064 | STR-TET-NAL | ||
SAL6 | 38 | F | Egypt | faeces | Kentucky | 198 | mphB | aac-Ie; aac-Ib; aac(6′)-Iaa; aadA7; aph-Id; CMY-2; XA-10; TEM-98, TEM-1; cmlA1; sul3; tet(A)-1; aadA1; floR | 8 | >0.064 | >0.5 | AMP-SUL-GEN-TOB-STR-TET-NAL-CAZ2-CTX1-FOX | AmpC blaCMY-2 |
ID . | Age . | Sex . | Travel . | Origin of isolate . | Serotype . | MLST . | Azithromycin resistance gene (WGS) . | Other resistance genes (WGS) . | Phenotypic resistance profile . | . | |||
---|---|---|---|---|---|---|---|---|---|---|---|---|---|
. | . | . | . | . | . | . | . | . | AZM MIC . | CIP MIC >0.064 mg/L . | CIP MIC >0.5 mg/L . | other antibiotics . | β-Lactamase (PCR) . |
SAL1 | 28 | M | nil | faeces | Thompson | 26 | mphA | aac-lb-cr; aac-ly; aadA16; qnrB6; arr-3; sul3; dfrA27; sul1 | 16 | >0.064 | SUL-TMP | ||
SAL2 | 67 | M | nil | urine | Typhimurium | 19 | mphB | aac-Iaa; aph-Id; TEM-98,TEM-1; sul3; sul2; tet(A)-1; dfrA1 | 6 | <0.064 | AMP-SUL-STR-TET-TMP | ||
SAL3 | 58 | F | nil | faeces | Typhimurium | 34 | mphA | aac-IId; aac-Iaa; aadA17; aph-ld; TEM-98, TEM-1; qnrS1; lnu(F); arr-2; sul2; tet(A); dfrA5; dfrA14; dfrA25 | 96 | >0.064 | >0.5 | AMP-SUL-GEN-TOB-STR-TET-TMP-NAL-FOX | |
SAL4 | 79 | M | nil | faeces | Typhimurium | 34 | mefB | aac-Iaa; aadA2; aph-Id; TEM-98, TEM-1; sul2; tet(A); dfrA12 | 8 | <0.064 | AMP-SUL-STR-TET-TMP | ||
SAL5 | 58 | M | nil | faeces | Ridge | novel ST | mphA | aac-IIa; aac-Iy; aph-Id; TEM-98, TEM-1; sul2; dfrA14; tet(A)-1 | 16 | <0.064 | AMP-SUL-GEN-TOB-STR-TET-TMP | ||
H123740558 | 5 | M | nil | faeces | Blockley | 52 | mphA | aac-Iy; aph-Id; tet(A)-1 | 24 | >0.064 | >0.5 | STR-TET-NAL | |
H123780513 | 78 | F | not known | faeces | Blockley | 52 | mphA | aac-Iy; tet(A)-1 | 24 | >0.064 | >0.5 | TET-NAL | |
H124040535 | 59 | F | nil | faeces | Blockley | 52 | mphA | aac-Iy; aph-Id; tet(A)-1 | 48 | >0.064 | STR-TET-NAL | ||
SAL6 | 38 | F | Egypt | faeces | Kentucky | 198 | mphB | aac-Ie; aac-Ib; aac(6′)-Iaa; aadA7; aph-Id; CMY-2; XA-10; TEM-98, TEM-1; cmlA1; sul3; tet(A)-1; aadA1; floR | 8 | >0.064 | >0.5 | AMP-SUL-GEN-TOB-STR-TET-NAL-CAZ2-CTX1-FOX | AmpC blaCMY-2 |
AMP, ampicillin; CHL, chloramphenicol; SUL, sulphonamide; GEN, gentamicin; TOB, tobramycin; STR, streptomycin; TET, tetracycline; TMP, trimethoprim; NAL, nalidixic acid; CIP, ciprofloxacin; CAZ, ceftazidime; CTX, cefotaxime; FOX, cefoxitin; AZM, azithromycin.
Breakpoints for antibiotics: ampicillin 8 mg/L, chloramphenicol 8 and 16 mg/L, sulphonamide 256 mg/L, gentamicin 2 mg/L, tobramycin 8 mg/L, streptomycin 16 mg/L, tetracycline 8 mg/L, trimethoprim 2 mg/L, nalidixic acid 16 mg/L, ciprofloxacin 0.064 and 0.5 mg/L, ceftazidime 1 and 2 mg/L, cefotaxime 0.5 and 1 mg/L, cefoxitin 8 mg/L, azithromycin 16 mg/L.
Resistance genes: aac, aph, aad (aminoglycosides), qnrB6/S1 (quinolone), arr (rifampicin), sul (sulphonamide), dfr (trimethoprim), TEM-1 (β-lactam, ampicillin), TEM-98 (β-lactam, ampicillin), tet (tetracycline), lnu (oxazolidinone), CMY-2 (Amp C β-lactam), OXA-10 (β-lactam), cmlA1 (chloramphenicol), floR (chloramphenicol), mph, mef (macrolide).
DNA extraction for WGS
DNA extraction of Salmonella isolates was carried out using a modified protocol of the Qiasymphony DSP DNA Midi Kit (Qiagen). In brief, 0.7 mL of overnight Salmonella broth culture was harvested. Bacterial cells were pre-lysed in 220 μL of ATL buffer (Qiagen) and 20 μL of Proteinase K (Qiagen), and incubated with shaking for 30 min at 56°C. Four microlitres of RNase at 100 mg/mL (Qiagen) was added to the lysed cells, which were then re-incubated for a further 15 min at 37°C. This step increases the purity of the DNA for downstream sequencing.
DNA from the treated cells was then extracted on the Qiasymphony SP platform (Qiagen) and eluted in 100 μL of water.
DNA concentration was determined using the GloMax system (Promega) and quality (optimal OD260/230 = 1.8–2.0) was determined using the LabChip DX system (Perkin Elmer) for the following sequencing steps.
DNA sequencing
Extracted DNA was then prepared using the NexteraXT sample preparation method and sequenced with a standard 2 × 101 base protocol on a HiSeq 2500 instrument (Illumina, San Diego).
MinION sequencing was also carried out to define the complete structure of the genomic drug island in the Salmonella Blockley isolate H123780513. A library was prepared using the Genomic DNA Sequencing Kit SQK–MAP006 according to the protocol from Oxford Nanopore Technologies (Version MN006_1115_revC_14Aug2015) and following the same principles as described in Ip et al.,28 except that sheared DNA was repaired using FFP Repair Mix (New England Biolabs, Ipswich, MA, USA) and then prepared using the NEBNext Ultra II End-Repair/dA-tailing Module (New England Biolabs). The final ligation of adapter and hairpin was performed using adapters and tethers from the SQK–MAP006 sequencing kit (Oxford Nanopore Technologies, Oxford, UK) followed by purification of the adapted and tethered DNA using MyOne C1 beads (Life Technologies). Purified DNA was loaded for sequencing into the flow cell (R9 chemistry) by Oxford Nanopore Technologies (Oxford, UK).
Sequence assembly and detection of resistance genes
Genome assembly was carried out using Spades v.3.7.0 (http://www.ncbi.nlm.nih.gov/pmc/articles/PMC3342519/) with the command line options ‘–k 21, 33, 55, 77’ and ‘–careful’. Detection of resistance genes was as described by Doumith et al. (2015).29 Briefly, reads were mapped to a reference database of acquired genes including those conferring resistance to macrolides that were collated from the Comprehensive Antibiotic Resistance Database (http://arpcard.mcmaster.ca).
Spades v.3.7.0 hybrid assembly was used to combine the MinION reads with the Illumina reads. MinION reads were mapped back to the hybrid assembly and this mapping was used to confirm the contiguity of key parts of the hybrid assembly.
Phylogenetic analysis
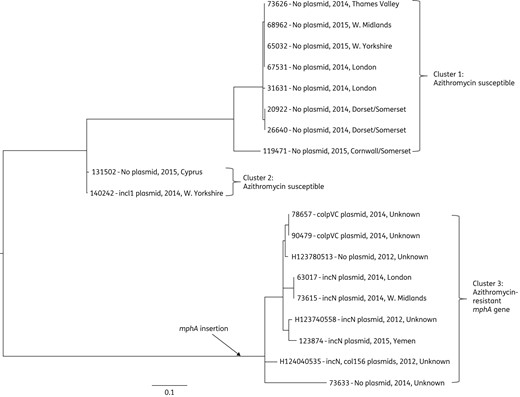
Phylogenetic relationship between azithromycin-resistant and -susceptible Salmonella Blockley isolates circulating in the UK between 2012 and 2015. The phylogenetic tree was generated by SNP analysis. 73 626 was the reference strain used for de novo assembly for SNP detection. The insertion point of mphA into Salmonella Blockley population is indicated. Presence (inc group)/absence of plasmid, year of isolation and location are shown beside the isolate number.
Location and characterization of drug resistance region
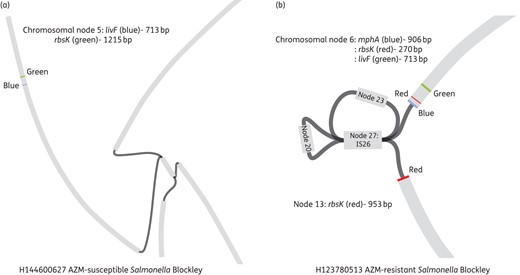
Bandage assembly of nodes (contigs) from susceptible and resistant Salmonella Blockley isolates. Bandage allows visualization of how contigs (in grey) are possibly connected (in black) to each other. The genes of interest (in this case livF, rbsK and mphA) are then blasted against all the assembled contigs and their locations determined. Regions around the genes of interest can then be determined using Artemis. (a) livF and rbsK are located on chromosomal node 5 in an azithromycin-susceptible Salmonella Blockley isolate. mphA is not present. (b) The mphA gene (azithromycin resistance) and the other regions associated with resistance (node 27, 23, 20) are inserted between rbsK in an azithromycin-resistant Salmonella Blockley isolate. AZM, azithromycin. This figure appears in colour in the online version of JAC and in black and white in the print version of JAC.
Nucleotide sequence accession number
The nucleotide sequence of the Salmonella azithromycin resistance genomic island (SARGI) was assigned GenBank accession number KX237654.
In silico plasmid detection
PlasmidFinder (http://cge.cbs.dtu.dk/services/PlasmidFinder/) was used to detect the presence of known replicon types of plasmids in the isolates studied.36
Plasmid extraction
Plasmid DNA was isolated as previously described37 in accordance with the methods of Kado and Liu (1981).38
Phenotypic and PCR susceptibility testing
Susceptibility testing for isolates harbouring azithromycin resistance determinants was performed using a well-established breakpoint agar dilution method using Iso-Sensitest agar or Mueller–Hinton agar to determine whether the isolate was susceptible or resistant to a known concentration of the antimicrobial.1 The antimicrobial concentrations used for screening of resistance were: ampicillin 8 mg/L; chloramphenicol 8 and 16 mg/L; colomycin 2 mg/L; sulphonamide 256 mg/L; gentamicin 2 mg/L; tobramycin 8 mg/L; amikacin 8 mg/L; streptomycin 16 mg/L; tetracycline 8 mg/L; trimethoprim 2 mg/L; nalidixic acid 16 mg/L; ciprofloxacin 0.064 and 0.5 mg/L; ceftazidime 1 and 2 mg/L; cefotaxime 0.5 and 1 mg/L; cefoxitin 8 mg/L; cefpirome 8 mg/L; ertapenem 0.064 and 0.5 mg/L; and temocillin 128 mg/L.1,20,39 Azithromycin susceptibility testing was performed using Etests (bioMérieux, France) and MIC ≤16 mg/L according to the EUCAST guidelines was used for interpretation of resistance.20 Antimicrobial susceptibility testing was subjected to internal quality assurance in accordance with published methods and to external quality assurance in collaboration with laboratories within the European Union Reference Laboratory Antimicrobial Resistance (EURL-AMR).1,39
NTS isolates were classified as MDR if they were resistant to three or more antimicrobial agents.6 Isolates that were resistant to cefotaxime at 1 mg/L were subjected to an in-house PCR assay to detect mechanisms of β-lactam resistance (CTX-M ESBLs, and genes encoding AmpC, SHV, TEM, GES, VEB and PER β-lactamases3).
Results
Genomes of 667 Salmonella isolates were screened for known acquired resistance genes, including those previously associated with resistance to azithromycin in Enterobacteriaceae. The presence of azithromycin resistance determinants mphA (n = 6), mphB (n = 2) and mefB (n = 1), amongst other resistance determinants conferring resistance to β-lactams, aminoglycosides, quinolones, tetracycline and sulphonamides, was identified in only nine genomes, as detailed in Table 1. Phenotypic susceptibility testing confirmed the multidrug resistance phenotypes of the corresponding nine isolates, which had MICs of azithromycin from 6 to ≥16 mg/L (Table 1).
Strains were confirmed to be NTS by MLST (MLST database: http://mlst.warwick.ac.uk/mlst/) and classical serology; there were three Salmonella Typhimurium (ST19 and ST34), three Salmonella Blockley (ST52) and one each of Salmonella Thompson (ST26), Salmonella Ridge (novel ST not found in the MLST database) and Salmonella Kentucky (ST198) (Table 1).
Table 1 shows the epidemiological data and analysis of the mechanism of drug resistance, in particular the azithromycin resistance detected by phenotypic (MIC), genetic (WGS) and molecular (PCR) methods in the nine NTS isolates studied.
In addition to the sequence type, we also investigated the whole-genome similarity between the three Salmonella Typhimurium and three Salmonella Blockley isolates. The phylogenetic analysis and metadata of the Salmonella Typhimurium isolates indicated that they were not closely related (data not shown) and different genes (mphA, mphB and mefB) associated with azithromycin resistance/partial resistance were involved, suggesting it had been acquired following separate events. In addition to the three Salmonella Blockley isolates harbouring mphA, a further 16 Salmonella Blockley isolates not included in the initial screening process were used as background isolates in the Salmonella Blockley phylogenetic analysis (Table S1 and Figure 1). Six of the additional 16 Salmonella Blockley isolates harboured the mphA gene. The resulting phylogeny separated the azithromycin-resistant Salmonella Blockley isolates harbouring mphA (cluster 3) from the azithromycin-susceptible isolates (clusters 1 and 2) (Figure 1). The diversity between the azithromycin-resistant isolates was not consistent with them being derived from a single epidemiologically related event, with SNP differences ranging from 0 to 50 (Figure 1). The inferred point of insertion of the mphA gene in the azithromycin-resistant Salmonella Blockley population is indicated in Figure 1.
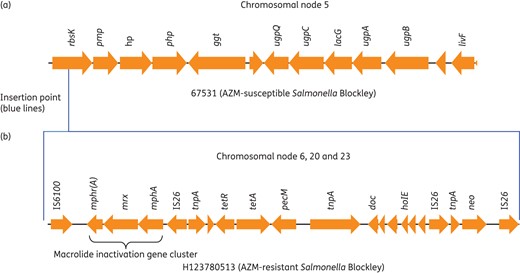
Chromosomal insertion site of the azithromycin resistance gene (mphA) and possible structure of the Salmonella azithromycin resistance genomic island (SARGI). Chromosomal nodes are based on Bandage assembly (Figure 2). Insertion site of drug island in rbsK is depicted by blue lines. Postulated structure of drug island: neo, aminoglycoside; tet, tetracycline; mphA, macrolide (azithromycin); mrx, major facilitator protein; mphr(A), macrolide repressor A; tnp, transposase; AZM, azithromycin. This figure appears in colour in the online version of JAC and in black and white in the print version of JAC.
We generated a total of 10 913 2D MinION reads (both pass and fail were used) with a mean length of 3133 bp. When mapped using BWA-MEM, 9076 reads (83%) mapped back to the Illumina-only assembly of H123780513, giving an average depth of 5.8×. This depth of coverage is not sufficient for de novo assembly, so a hybrid assembly approach was used. The hybrid Illumina–MinION assembly resolved the complete structure of the ∼17 kb SARGI (Figure 3). The island harboured tetracycline and aminoglycoside resistance genes as well as phage and plasmid remnants.
There are various ways of detecting plasmids from WGS sequence data using bioinformatic tools,40,41 but in this study we used classical plasmid extraction analysis to show that all the azithromycin-susceptible isolates (except for 140242, which is an MDR isolate) were plasmid free (Figure 1 and Figure S1). PlasmidFinder confirmed the absence of known replicon sequence types in the respective genomes. Two of the mphA-positive Salmonella Blockley isolates (H123780513 and 73633) did not harbour any plasmids while the other isolates had an incN, colpVC or col156 plasmid that did not seem to be associated with azithromycin resistance (Figure 1). Preliminary Bandage, Blast, Prokka, Artemis and PlasmidFinder analysis also suggests that the mphA gene is present on an incFIB(K) plasmid in Salmonella Thompson, an incA/C2 plasmid in Salmonella Ridge and either an incQ1 or an incH12 plasmid in Salmonella Typhimurium (data not shown). Characterization of the complete resistance regions in each of the plasmids belonging to the different serovars was not carried out as it was beyond the remit of this study.
The ages of the nine cases from whom the isolates were acquired ranged from 5 to 79 years and five were males (Table 1). The nine isolates were recovered from urine (n = 1) and stool (n = 8) and were MDR. One isolate was acquired from a case with a history of recent travel to Egypt and was identified as a Salmonella Kentucky. The isolate was confirmed to be an AmpC producer by both phenotypic (cefotaxime MIC >1 mg/L and cefoxitin MIC >8 mg/L) and molecular methods (PCR demonstrated the blaCMY-2 gene). Another MDR isolate, identified as Salmonella Ridge, was acquired from a case with underlying immunosuppression (after bone marrow transplantation) and had recent exposure to antibiotics.
The azithromycin MICs of the six non-Salmonella Blockley and nine Salmonella Blockley isolates showed that the presence of the mphA gene was associated with MIC ≥16 mg/L, while the presence of mefB or mphB was not associated with MIC ≥16 mg/L (Table 1 and Table S1).
Discussion
Multidrug resistance in typhoidal and non-typhoidal Salmonella is an emerging threat to public health.3–6,8 Azithromycin is being used as the preferred antimicrobial agent to treat cases of uncomplicated enteric fever from Asia and MDR NTS in immunosuppressed patients or those with invasive infections. However, there are emerging reports of azithromycin resistance in cases of enteric fever as well as invasive NTS infection.9,10,12,42,43
The incidence of azithromycin resistance is increasing in Escherichia coli, Klebsiella and Shigella. Azithromycin-resistant Shigella spp. isolated from MSM who had previous multiple exposures to azithromycin have been reported.44 Decreased susceptibility to azithromycin (DSA) is defined as a strain of Shigella with azithromycin MIC >16 mg/L; such strains often harbour genes ermB and mphA, which are plasmid encoded and associated with clinical failure.44–46 A Canadian study showed that strains of Shigella flexneri isolated from MSM harboured the mphA gene and had azithromycin MIC >64 mg/L.45
This study identified the presence of known azithromycin resistance determinants in 15 Salmonella isolates. Twelve out of the 15 isolates encoded the mphA gene and these isolates had azithromycin MICs between 16 and 96 mg/L; none of these isolates carried ermB (Table 1). Two isolates encoded only mphB while one isolate encoded only mefB; these three isolates all had azithromycin MICs <16 mg/L. These results indicate that carriage of only mphB or mefB may not lead to azithromycin resistance in Salmonella, as described previously in Shigella flexneri,44 and that the presence of other genes, such as the erm cluster/genes, or chromosomal mutations in the rrl ribosomal genes, may be required for a synergistic effect to produce higher resistance to azithromycin (or the azalide group).14 However, larger studies with a more diverse set of salmonellae, and more in-depth functional characterizations, are needed to understand the resistance mechanisms associated with these genes.
The single isolate of Salmonella Kentucky, which was an AmpC producer and carried the blacmy-2 gene, was associated with travel to Egypt, a finding consistent with previous studies.3 This isolate was typed as ST198 Kentucky and this serovar has been reported to be an ESBL producer.47
It is interesting to note that the 15 Salmonella isolates were MDR and the presence of plasmids and mobile genetic elements may have played a crucial role in acquisition of resistance to multiple agents. There were various mechanisms involved in high-level azithromycin resistance in the different Salmonella serovars studied, conferred either on the chromosome or plasmid. In Salmonella Blockley azithromycin resistance was not associated with the presence of a plasmid but rather a chromosomally mediated macrolide inactivation gene cluster, mphA-mrx-mphr(A). The macrolide inactivation gene cluster was part of a novel SARGI which was inserted in the same chromosomal rbsK gene in all the azithromycin-resistant Salmonella Blockley isolates (Figure 3). This chromosomal mphA-mrx-mphr(A) gene cluster has not been described previously in salmonellae but has been recently characterized in a genomic island in Proteus mirabilis.48 The complete structure of the azithromycin drug island in Salmonella Blockley (Figure 3) was deduced by hybrid genome assembly of long MinION reads and short Illumina reads. Recently the mphA gene was shown to be present on a plasmid-encoded drug island in Salmonella Corvallis.12 This plasmid drug island differed from the one described in the current study as it did not have the same macrolide inactivation cluster. Azithromycin resistance in Salmonella Typhimurium, Salmonella Thompson and Salmonella Ridge was associated with the presence of the mphA gene located on a plasmid. The plasmids associated with the mphA gene in each of these serovars—Salmonella Typhimurium (incQ1 or incHI2), Salmonella Ridge (incA/C2), Salmonella Thompson [incFIB(K)]—differed from the one described by Villa et al.12 in 2015 for Salmonella Corvallis as well as the incFII plasmid associated with azithromycin resistance in Shigella,46 thus providing further evidence for multiple modes of transmission for azithromycin resistance.
The presence of Salmonella Blockley isolates with chromosomally mediated high levels of azithromycin resistance in the UK population is a cause for concern. This stable chromosomal resistance may lead to the dissemination of resistant clones that can cause outbreaks. Phylogenetic analysis of the 9 azithromycin-resistant and 10 background-susceptible Salmonella Blockley isolates studied indicated that the majority of the resistant isolates are not clonally related, and the probable point of insertion of the mphA gene in the population is indicated on the phylogenetic tree (Figure 1). Clonal relatedness was observed for two resistant isolates (78657 and 90479), which were isolated from the same patient a few months apart (probable treatment failure), as well as for 63017 and 73615, isolated from two separate cases in London and the West Midlands (possible undetected outbreak clone) (Table S1 and Figure 1). Screening for azithromycin resistance is not conducted routinely but should be encouraged as in the past 3 years there have been 16–26 Salmonella Blockley isolates submitted to GBRU each year, with most being domestically acquired (PHE data).
There is also a cause for concern as plasmid-mediated resistance to azithromycin is arising in multiple Salmonella serovars in the UK and may lead to easier and widespread onward transmission of resistance. This rise of both chromosomally mediated and plasmid-mediated azithromycin resistance may be due to the increase in azithromycin usage and increase in azithromycin resistance in other Enterobacteriaceae populations.7,14,49
This study demonstrated the utility of WGS data as a rapid screening tool allowing many hundreds of isolates to be investigated for antimicrobial resistance determinants not routinely assayed using phenotypic tests. The availability of WGS data as well as phenotypic and epidemiological investigations allows emerging threats, such as azithromycin resistance in Enterobacteriaceae, to be monitored in a cost-effective and timely manner. High-throughput screening for surveillance is beneficial for public health purposes as it not only allows detection of azithromycin resistance in the population but also allows enhanced surveillance of patients to be carried out, which will improve our understanding of onward transmission. Such data in turn can be used to inform clinicians to enable them to administer appropriate treatment. At present no clinical breakpoints for azithromycin have been defined for Enterobacteriaceae by EUCAST or CLSI. However, further work on strains of NTS and those causing enteric fever needs to be undertaken to establish whether 16 mg/L is the relevant clinical MIC for azithromycin in Salmonella spp. This epidemiological cut-off established from WT strains seems very high compared with clinical MICs for other Gram-positive and Gram-negative bacteria; however, azithromycin has a very high tissue:serum concentration ratio.50
Using WGS for detection of antibiotic resistance also lends itself to data sharing, enabling international collaboration in the monitoring of this global threat. As part of this approach, continued phenotypic characterization of antimicrobial resistance for a subset of isolates is vital to ensure that novel resistance mechanisms are discovered.
One of the limitations of this study is the absence of a complete clinical history of each case. This prevents us correlating our work with clinical outcome. Moreover, most of the isolates were from stool specimens and the cases may not have received antimicrobials if they had self-limiting infections.
Conclusions
Azithromycin resistance is probably under-reported in the UK and globally as frontline laboratories do not test for azithromycin resistance in NTS due to the cost. This study has shown that WGS is an effective method for screening large numbers of isolates for known resistance determinants. Further clinical studies are needed to establish the role of various resistance genes in determination of clinical MIC in conjunction with WGS. Even though the numbers of azithromycin-resistant Salmonella spp. from the UK remained low (15/683 isolates studied), the detection of azithromycin resistance in multiple serovars of Salmonella is a matter of concern and regular monitoring and surveillance should be a priority.
Funding
This study was supported by internal funding.
Transparency declarations
None to declare.
Acknowledgements
We thank Professor Neil Woodford from the Bacteriology Reference Department, Public Health England, for support during this pilot project, as well as colleagues in the Salmonella Reference Service, Genome Sequencing and Development Unit and Infectious Disease Informatics at Colindale for their contribution to this work. We also thank Dr Kathie Grant for critically reading the manuscript.
References