-
PDF
- Split View
-
Views
-
Cite
Cite
Praveen Papareddy, Gopinath Kasetty, Martina Kalle, Ravi K. V. Bhongir, Matthias Mörgelin, Artur Schmidtchen, Martin Malmsten, NLF20: an antimicrobial peptide with therapeutic potential against invasive Pseudomonas aeruginosa infection, Journal of Antimicrobial Chemotherapy, Volume 71, Issue 1, January 2016, Pages 170–180, https://doi.org/10.1093/jac/dkv322
- Share Icon Share
Abstract
Increasing resistance to antibiotics makes antimicrobial peptides interesting as novel therapeutics. Here, we report on studies of the peptide NLF20 (NLFRKLTHRLFRRNFGYTLR), corresponding to an epitope of the D helix of heparin cofactor II (HCII), a plasma protein mediating bacterial clearance.
Peptide effects were evaluated by a combination of in vitro and in vivo methods, including antibacterial, anti-inflammatory and cytotoxicity assays, fluorescence and electron microscopy, and experimental models of endotoxin shock and Pseudomonas aeruginosa sepsis.
The results showed that NLF20 displayed potent antimicrobial effects against the Gram-negative bacteria Escherichia coli and P. aeruginosa, the Gram-positive Bacillus subtilis and Staphylococcus aureus and the fungi Candida albicans and Candida parapsilosis. Importantly, this antimicrobial effect was retained in human blood, particularly for P. aeruginosa. Fluorescence and electron microscopy studies showed that the peptide exerted membrane-breaking effects. In an animal model of P. aeruginosa sepsis, NLF20 reduced bacterial levels, resulting in improved survival. Reduced mortality was also observed in experimental animal models of endotoxin shock, which was paralleled with modulated IFN-γ, IL-10 and coagulation responses.
Together, these results indicate that functional epitopes of HCII may have therapeutic potential against bacterial infection.
Introduction
The human pathogen Pseudomonas aeruginosa causes and/or aggravates a spectrum of diseases, including bacterial conjunctivitis and keratitis, otitis, postoperative and burn wound infections, chronic leg ulcers, pneumonia and cystic fibrosis.1–3 Considering also growing problems with resistance development against conventional antibiotics, new bactericidal agents against P. aeruginosa are needed and there is significant current interest in the potential use of antimicrobial peptides as novel treatment modalities.4 From a therapeutic perspective, antimicrobial peptides should display high bactericidal potency, but low toxicity against (human) eukaryotic cells. Combinational library approaches,5 use of stereoisomers composed of d-amino acids,6 or cyclic d,l-α-peptides,7 high-throughput-based screening assays,8,9 quantitative structure–activity relationship (QSAR) approaches4,8,10,11 and identification of endogenous peptides12–17 have all been used for identifying selective and therapeutically interesting antimicrobial peptides.4,18 In addition to their direct antimicrobial effects, antimicrobial peptides have recently been found to exhibit multifaceted immunomodulatory activities, such as those observed for LL-3719,20 and peptides derived from thrombin,12,21,22 including functions within angiogenesis, chemotaxis and wound healing.19 These biological properties indicate that antimicrobial peptides may also have a clinical potential in disorders where targeting of inflammatory pathways is beneficial, such as in sepsis.19,23
The serine proteinase inhibitor heparin cofactor II (HCII), found in plasma, was recently shown to mediate bacterial clearance upon proteolytic activation.24 Mice deficient in HCII displayed increased susceptibility to infection by P. aeruginosa. Correspondingly, decreased levels of HCII were observed in WT animals challenged with bacteria. Since the antibacterial activities were mapped to the A and D helices of HCII, supplementation with these functional epitopes could be an attractive strategy to target bacterial infection. In this work, we therefore explored whether NLF20 (NLFRKLTHRLFRRNFGYTLR), an antimicrobial peptide derived from helix D of HCII, may have therapeutic potential against invasive P. aeruginosa infection.
Materials and methods
Peptides
The peptides NLF20 (NLFRKLTHRLFRRNFGYTLR) and omiganan (ILRWPWWPWRRK-NH2) were synthesized by Biopeptide Co., San Diego, USA, while LL-37 (LLGDFFRKSKEKIGKEFKRIVQRIKDFLRNLVPRTES) was from Innovagen AB, Lund, Sweden. The two latter peptides were included as biological effect benchmark peptides. The purity (>95%) of these peptides was confirmed by mass spectral analysis (MALDI-TOF Voyager).
Microorganisms
The bacterial and fungal isolates Escherichia coli ATCC 25922, P. aeruginosa ATCC 27853, Staphylococcus aureus ATCC 29213, Streptococcus pyogenes AP1, Bacillus subtilis ATCC 6633, Candida albicans ATCC 90028 and Candida parapsilosis ATCC 90018 were all obtained from the Department of Bacteriology, Lund University Hospital. The P. aeruginosa strain PA01 was a generous gift from Dr B. Iglewski (University of Rochester). The clinical isolate P. aeruginosa 15159 was originally derived from a patient with a chronic leg ulcer and obtained from the Department of Bacteriology, Lund University Hospital, Sweden.
Viable count analysis
E. coli ATCC 25922, P. aeruginosa ATCC 27853 or S. aureus ATCC 29213 bacteria were grown to mid-logarithmic phase in Todd–Hewitt (TH) medium (Becton Dickinson, MD, USA). The microorganisms were then washed and diluted in 10 mM Tris, pH 7.4, containing 5 mM glucose. Following this, bacteria (50 μL; 2 × 106 cfu/mL) were incubated at 37°C for 2 h with NLF20 or LL-37 (at 0.03, 0.06, 0.3, 0.6, 3, 6, 30 and 60 μM) in 10 mM Tris/0.15 M NaCl, with or without 20% human citrate plasma. In the experiments using 50% whole blood, S. aureus ATCC 29213 and P. aeruginosa ATCC 27853 bacteria (50 μL; 2 × 108 cfu/mL) were incubated at 37°C for 1 h in the presence of peptide at 60 (for P. aeruginosa) and 120 μM (P. aeruginosa and S. aureus) (the high concentrations were used to achieve high bactericidal effect). To quantify bactericidal activity, serial dilutions of the incubation mixtures were plated on TH agar, followed by incubation at 37°C overnight and determination of the number of cfu. One-hundred percent survival was defined as total survival of bacteria in the same buffer and under the same condition in the absence of peptide. Significance was determined using the statistical software SigmaStat (SPSS Inc., Chicago, IL, USA).
MIC determination
The MIC assay was carried out using a microtitre broth dilution method as previously described in the CSLI guidelines.25 In brief, fresh overnight colonies were suspended to a turbidity equivalent to that of a 0.5 McFarland standard and further diluted in Mueller–Hinton broth (Becton Dickinson). For determination of MIC, peptides were dissolved in water at a concentration 10-fold higher than the required range by serial dilutions from a stock solution. Ten microlitres of each concentration was added to each corresponding well of a 96-well microtitre plate (polypropylene, Costar Corp.) and 90 μL of bacterial suspension (1.1 × 106/mL) in Mueller–Hinton broth was added. The plate was incubated at 37°C for 16–18 h. The MIC was taken as the lowest concentration where no visual growth of bacteria was detected.
Radial diffusion assay
Essentially as described previously,26,27 bacteria were grown to mid-logarithmic phase in 10 mL of full-strength (3% w/v) trypticase soy broth (TSB) (Becton Dickinson). The microorganisms were then washed once with 10 mM Tris, pH 7.4. Subsequently, 4 × 106 cfu were added to 15 mL of the underlay agarose gel, consisting of 0.03% (w/v) TSB, 1% (w/v) low-electroendosmosis-type agarose (Sigma-Aldrich, St Louis, MO, USA) and 0.02% (v/v) Tween 20 (Sigma-Aldrich). The underlay was poured into a 144 mm diameter Petri dish. After agarose solidification, 4 mm diameter wells were punched and 6 μL of peptide solution of the required concentration was added to each well. Plates were incubated at 37°C for 3 h to allow peptide diffusion. The underlay gel was then covered with 15 mL of molten overlay (6% TSB and 1% low-electroendosmosis-type agarose in distilled H2O). Antimicrobial activity of a peptide was visualized as a clearing zone around each well after 18–24 h of incubation at 37°C.
Fluorescence microscopy
The impermeant probe FITC (Sigma-Aldrich) was used for monitoring bacterial membrane permeabilization. E. coli ATCC 25922 bacteria were grown to mid-logarithmic phase in TSB medium. Bacteria were washed and re-suspended in buffer (10 mM Tris, pH 7.4/0.15 M NaCl/5 mM glucose) to yield a suspension of 1 × 107 cfu/mL. One-hundred microlitres of the bacterial suspension was incubated with 30 μM of the respective peptides at 30°C for 30 min. Microorganisms were then immobilized on poly(l-lysine)-coated glass slides by incubation for 45 min at 30°C, followed by addition to the slides of 200 μL of FITC (6 μg/mL) in buffer and a final incubation for 30 min at 30°C. The slides were washed and bacteria fixed by incubation, first on ice for 15 min, then at room temperature for 45 min in 4% paraformaldehyde. The glass slides were subsequently mounted on slides using Prolong Gold antifade reagent mounting medium (Invitrogen, Eugene, USA). Bacteria were visualized using a Nikon Eclipse TE300 (Nikon, Melville, USA) inverted fluorescence microscope equipped with a Hamamatsu C4742-95 cooled CCD camera (Hamamatsu, Bridgewater, USA) and a Plan Apochromat ×100 objective (Olympus, Orangeburg, USA). Differential interference contrast (Nomarski) imaging was used for visualization of the microbes themselves.
Electron microscopy
For transmission electron microscopy and visualization of peptide effects on bacteria, P. aeruginosa ATCC 27853 and S. aureus ATCC 29213 (1–2 × 106 cfu/sample) were incubated for 2 h at 37°C with the peptides (30 μM). Samples of P. aeruginosa and S. aureus suspensions were adsorbed onto carbon-coated copper grids for 2 min, washed briefly with two drops of water, and negatively stained with two drops of 0.75% uranyl formate. The grids were rendered hydrophilic by glow discharge at low pressure in air. All samples were examined with a Jeol JEM 1230 electron microscope operated at 80 kV accelerating voltage. Images were recorded with a Gatan Multiscan 791 charge-coupled device camera.
Haemolysis assay
EDTA blood was centrifuged at 800 g for 10 min, after which plasma and buffy coat were removed. The erythrocytes were washed three times and re-suspended in PBS, pH 7.4, to a 5% suspension. The cells were then incubated with end-over-end rotation for 1 h at 37°C in the presence of peptides (3–60 μM). Triton X-100 (2%) (Sigma-Aldrich) served as positive control. Following this, the samples were centrifuged at 800 g for 10 min and the supernatant was transferred to a 96-well microtitre plate. In the experiments with blood infected by bacteria, citrate blood was diluted (1:1) with PBS. The cells were then incubated with end-over-end rotation for 1 h at 37°C in the presence of peptides (30, 60 or 120 μM) and E. coli, P. aeruginosa, S. pyogenes or S. aureus bacteria or the fungus C. albicans (2 × 108 cfu/mL). In another experiment, designed to evaluate peptide-induced haemolysis over a longer time, whole blood diluted in 50% RPMI or erythrocytes diluted in 50% PBS were incubated with NLF20 for 18 h at 37°C. In all cases, the absorbance of haemoglobin release was measured at λ 540 nm and was expressed as percentage of Triton X-100-induced haemolysis.
Lactate dehydrogenase (LDH) assay
HaCaT keratinocytes were grown to confluency in 96-well plates (3000 cells/well) in serum-free keratinocyte medium (SFM) supplemented with bovine pituitary extract and recombinant EGF (BPE–rEGF) (Invitrogen, Eugene, USA). The medium was then removed and 100 μL of the peptide added (at 3–60 μM, diluted in SFM/BPE–rEGF or in keratinocyte–SFM supplemented with 20% human serum). The LDH-based TOX-7 kit (Sigma-Aldrich) was used for quantification of LDH release from the cells. Results represent mean values from triplicate measurements, and are given as fractional LDH release compared with the positive control, consisting of 1% Triton X-100 (yielding 100% LDH release). In another set of experiments, LDH release in mouse plasma was analysed according to the manufacturer's instructions (USCN Life Science Inc., Wuhan, China). The data are represented as LDH release (in units) from three mice.
MTT assay
Sterile filtered MTT solution (5 mg/mL in PBS) was stored protected from light at −20°C until use. HaCaT keratinocytes, 3000 cells/well, were seeded in 96-well plates and grown in serum-free keratinocyte–SFM/BPE–rEGF medium to confluency. Peptides investigated were then added at 3–60 μM. After incubation overnight, 20 μL of the MTT solution was added to each well and the plates were incubated for 1 h in CO2 at 37°C. The MTT-containing medium was then removed by aspiration. The blue formazan product generated was dissolved by the addition of 100 μL of 100% DMSO per well, and the plates were gently swirled for 10 min at room temperature to dissolve the precipitate. The absorbance was monitored at 550 nm, and the results given represent mean values from triplicate measurements.
LPS effects on macrophages in vitro
Cells (3.5 × 105) (RAW264.7 macrophages) were seeded in 96-well tissue culture plates (Nunc, 167008) in phenol red-free DMEM (Gibco) supplemented with 10% FBS and antibiotics. Following 6 h of incubation to permit adherence, cells were stimulated with 10 ng/mL E. coli LPS (0111:B4) or P. aeruginosa LPS (Sigma), with and without peptide at various doses. The levels of NO in culture supernatants were determined 24 h after stimulation using the Griess reaction.28 Phenol red-free DMEM with FBS and antibiotics were used as a blank. A standard curve was prepared using 0–80 μM sodium nitrite solutions in double-distilled H2O.
Cytokine assay
The cytokines IL-6, IL-10, MCP-1, IFN-γ and TNF-α were measured in plasma from mice injected with LPS or P. aeruginosa (with or without peptide treatment), or alternatively with NLF20 alone (1 mg/mouse), using the Cytometric Bead Array Mouse Inflammation Kit (Becton Dickinson) according to the manufacturer's instructions. All plasma samples were stored at −20°C before analysis.
Clotting assays
Clotting times were analysed using a coagulometer (Amelung, Lemgo, Germany). The prothrombin time (PT) and the thrombin clotting time (TCT) were measured as follows: 100 μL of fresh human citrate plasma together with indicated concentrations of NLF20 were pre-warmed for 1 min at 37°C before clot formation was initiated by adding 100 μL of a clotting reagent [thromboplastin reagent (Trinity Biotech) for PT; thrombin reagent (Technoclone) for TCT]. To record the activated partial thromboplastin time (aPTT), 100 μL of a kaolin-containing solution (Technoclone) was added to the plasma–peptide mix and incubated for 200 s before clot formation was initiated by adding 100 μL of 30 mM fresh CaCl2 solution.
LPS model in vivo
Male C57BL/6 mice (8–10 weeks, 22 ± 5 g), were injected intraperitoneally with 200 μL of 18 mg of E. coli 0111:B4 LPS (Sigma) per kg of body weight. Thirty minutes after LPS injection, 0.2 or 0.5 mg of NLF20, or buffer alone, was injected intraperitoneally into the mice. Survival and status were followed for 7 days. For blood collection, mice were sacrificed 20 h after LPS challenge and lungs were removed and fixed. These experiments were conducted according to national guidelines and were approved by the Laboratory Animal Ethics Committee of Malmö/Lund (M228-10).
P. aeruginosa infection model
The animal experiments were conducted according to national guidelines and were approved by the Laboratory Animal Ethics Committee of Malmö/Lund (M226-12 and M228-10). Animals were housed under standard conditions of light and temperature and had free access to standard laboratory chow and water. P. aeruginosa 15159 bacteria were grown to logarithmic phase (OD620 ∼0.5), harvested, washed in PBS, diluted in the same buffer to either 2 × 108 cfu/mL (high levels) or 2 × 104 cfu/mL (low levels), and kept on ice until injection. One-hundred microlitres of the bacterial suspension was injected intraperitoneally into C57BL/6 mice. Immediately or 60 min after bacterial injection, 0.5 mg of NLF20 (in 10 mM Tris, pH 7.4) or buffer alone was injected intraperitoneally or subcutaneously into the mice. (The purpose of injecting NLF20 peptide immediately after bacterial injection was to compare NLF20 peptide activity with that of classical antibiotics.) Survival data were obtained by following the animals daily for up to 7 days. Mice reaching the predefined endpoint criteria were sacrificed and counted as non-survivors. In another experiment, mice were treated intraperitoneally or subcutaneously with 0.5 mg of NLF20 or 300 mg/kg ceftazidime or 100 mg/kg levofloxacin injected 0 h or 1 h after bacterial infection. In order to study bacterial dissemination to target organs, spleen, liver and kidney were harvested, placed on ice and homogenized and the number of cfu was determined. The P value was determined using the Mann–Whitney U-test. Data from three independent experiments were pooled. In another experiment, as previously described,29P. aeruginosa Xen41 bacteria were grown to mid-exponential phase (OD620 ∼0.5), harvested, washed in PBS, diluted in the same buffer to 2–3 × 108 cfu/mL and kept on ice until injection. One-hundred microlitres of the bacterial suspension was injected intraperitoneally into male BALB/c mice. One hour and 4 h after bacterial injection, 0.5 mg of NLF20 peptide or buffer alone was administrated subcutaneously. Mice were anaesthetized immediately or 12 h after bacterial infection, followed by data acquisition and analysis using a Spectrum three-dimensional imaging system with Living Image®. For evaluation of animal survival, mice showing the defined and approved endpoint criteria (immobilization and shaking) were sacrificed by an overdose of isoflurane (Abbott) and counted as non-survivors.
Histochemistry
Lung biopsies were fixed in 10% formalin, rehydrated and embedded in paraffin. Sections of 5 μm thickness were placed on polylysine-coated glass slides, deparaffinized in xylene, rehydrated in graded alcohols and stained with haematoxylin and eosin by routine procedures.
Statistical analysis
Values are shown as mean with SEM. For statistical evaluation of two experimental groups, the Mann–Whitney U-test was used and for comparison of survival curves the log-rank test was used. To compare more than two groups, one-way or two-way ANOVA with the Bonferroni post-test was used. Viable count data are presented as mean with standard deviation. All statistical evaluations were performed using GraphPad Prism software 6.0 with *P < 0.005, **P < 0.001 and ***P < 0.0001 and ns = not significant.
Results
To explore the antimicrobial spectrum of NLF20, we first investigated the effects in radial diffusion assays against Gram-negative E. coli and P. aeruginosa, Gram-positive B. subtilis and S. aureus and the fungi C. albicans and C. parapsilosis (Figure 1a). As can be seen, NLF20 activities well exceeded those observed for the human cathelicidin LL-37, a potent benchmark antimicrobial peptide. The antibacterial results were further substantiated by matrix-free viable count assay. Results from these dose–response experiments utilizing E. coli, P. aeruginosa and S. aureus confirmed that NLF20 displays significant antibacterial activity also in the presence of human citrated plasma (Figure 1b). In addition, kinetic studies demonstrated fast bacterial killing, indicating a direct bactericidal action compatible with many antimicrobial peptides (Figure 1c). MIC analyses according to CSLI against the above and other pathogens are presented in Supplementary Data (available as Supplementary data at JAC Online). Overall, NLF20 showed comparable activities to those observed for the two benchmarks omiganan30 and LL-37.31 It was also noted that NLF20 displayed low MIC levels, particularly for P. aeruginosa.
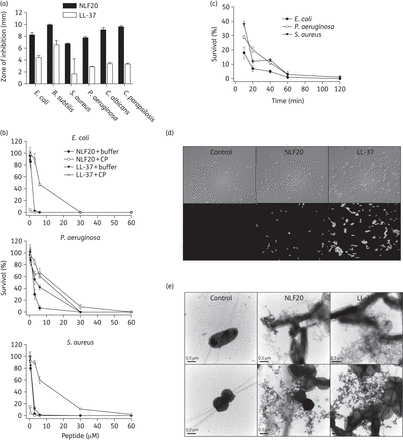
(a) Antimicrobial activity (using radial diffusion arrays) of NLF20 against the indicated microbes. For determination of antimicrobial activities, E. coli ATCC 25922, P. aeruginosa ATCC 27853, S. aureus ATCC 29213 and B. subtilis ATCC 6633 isolates (4 × 106 cfu) and C. albicans ATCC 90028 and C. parapsilosis ATCC 90018 (1 × 105 cfu) were inoculated into 0.1% TSB agarose gel (mean values are presented, n = 3). LL-37 is shown for comparison. (b) Antibacterial effects of NLF20 and LL-37 against E. coli ATCC 25922, P. aeruginosa ATCC 27853 and S. aureus ATCC 29213 in viable count assays at the indicated concentrations in 0.15 M NaCl/10 mM Tris, pH 7.4 (buffer), or in 0.15 M NaCl/10 mM Tris, pH 7.4, containing 20% human citrate plasma (CP) (n = 3, SD is indicated). (c) The time dependence of bacterial killing by NLF20 (at 6 µM) in 0.15 M NaCl/10 mM Tris, pH 7.4, containing 20% plasma was analysed by viable count assays using the indicated bacteria. (d) Permeabilizing effects of peptides on E. coli assessed using the impermeant probe FITC. (e) Electron microscopy analysis. P. aeruginosa and S. aureus bacteria were incubated for 2 h at 37°C with 6 µM NLF20 and visualized by negative staining. LL-37 is shown for comparison. Scale bar represents 0.5 µm. Control = buffer control.
Next, studies employing the impermeant probe FITC showed that NLF20 permeabilized bacterial membranes of E. coli similarly to LL-37 (Figure 1d). Electron microscopy utilizing P. aeruginosa demonstrated extensive membrane damage, with cell envelopes devoid of their cytoplasmic contents and intracellular material found extracellularly (Figure 1e). Again, similar findings were obtained with LL-37. These data indicate that NLF20 acts on bacterial membranes.
Frequently, antimicrobial peptides efficient in killing bacteria also exhibit haemolytic and membrane-permeabilizing activities against eukaryotic cells, which risks translating into toxicity issues. Importantly, however, NLF20 exerted only minor haemolytic activity at 30–60 μM and standard incubation times, and displayed less permeabilization than LL-37 (Figure 2a). Similar findings were observed with respect to permeabilization of HaCaT cells (Figure 2b), as well as effects on viability as monitored by the MTT assay (Figure 2c), further demonstrating this selectivity between bacteria and human cell membranes under physiological conditions, of importance for subsequent in vivo studies. Next, NLF20 was added to human blood infected by various Gram-positive and Gram-negative pathogens, as well as fungi. In such experiments, NLF20 caused almost complete eradication of P. aeruginosa, E. coli and S. pyogenes, with little (∼2% or less) accompanying haemolysis at a peptide concentration of 30–60 μM (Figure 2d). (The lower haemolysis in blood is due to anionic serum proteins competing with erythrocyte membranes for NLF20 binding.31) The observed low simultaneous haemolysis mediated by NLF20 under these conditions indicated a high selectivity of the peptide for bacteria (Figure 2e). In contrast, NLF20 was not particularly active in human blood against the Gram-positive S. aureus, as well as the fungus C. albicans, although the latter showed a minor reduction at 120 μM NLF20. The reduced activity against some bacteria is due to the presence of a high content of anionic serum proteins, able to bind cationic peptides such as NLF20 and reduce their antimicrobial effect through competition for peptide binding with bacterial membranes. This effect is widely observed for antimicrobial peptides,32 and is analogous to the reduced antimicrobial effects displayed by most antimicrobial peptides in the presence of growth media rich in anionic scavengers, such as in standardized MIC determinations.31
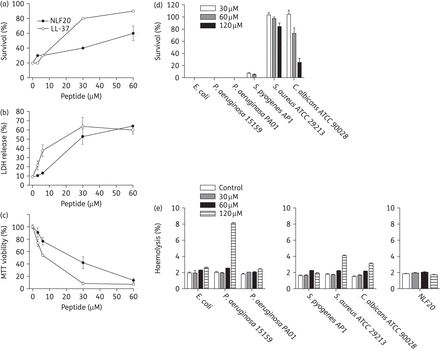
(a) Haemolytic effects of the indicated peptides. The cells were incubated with different concentrations of the peptides and haemolysis is expressed as the percentage of Triton X-100-induced haemolysis (note the scale of the y-axis). Effects of LL-37 are shown for comparison. (b) HaCaT keratinocytes were exposed to NLF20 or LL-37, and LDH release was plotted as a percentage of total LDH release. (c) The MTT assay was used to measure the viability of HaCaT keratinocytes in the presence of NLF20 or LL-37. (d) Antibacterial effects of NLF20 in the presence of the indicated bacteria in human blood (50% in PBS). The indicated microbes (2 × 108 cfu/mL) were added to 50% citrate blood, followed by peptide addition. The number of cfu was determined after an incubation period of 1 h. (e) In an identical set-up, haemolytic effects were studied. The bacteria were added to 50% citrate blood, followed by addition of peptide. Haemolysis was assessed after 1 h and is expressed as a percentage of Triton X-100-induced haemolysis (note the scale of the y-axis). The rightmost panel shows the effects of NLF20 only incubated with blood.
In another set of experiments, NLF20 toxicity was further evaluated following treatment of mice with NLF20. As shown in Supplementary Data, haemolysis after 18 h increased over time. At the highest peptide concentration used in the antimicrobial assays (313 μg/mL), however, it was only 5% in 50% blood. Furthermore, LDH release after treatment with 1 mg/mL was 4800 U, which is low compared with LL-37 treatment.33 Similarly, cytokine levels after peptide treatment at the same concentration are low,34 and also histology of lung tissue indicates no visible toxic effects of peptide treatment.
Having demonstrated the antimicrobial effects of NLF20, as well as its relatively limited toxicity, we next investigated possible anti-endotoxic effects of the peptide. As seen in Figure 3(a), NLF20 inhibited LPS-induced NO responses at 20–40 μM. In addition, measurements of the aPTT showed that NLF20 impaired the intrinsic pathway of coagulation in normal human plasma. Other parts of the coagulation system, as judged by PT (monitoring the extrinsic pathway of coagulation) and TCT (measuring thrombin-induced fibrin network formation), were not significantly affected (Figure 3b).
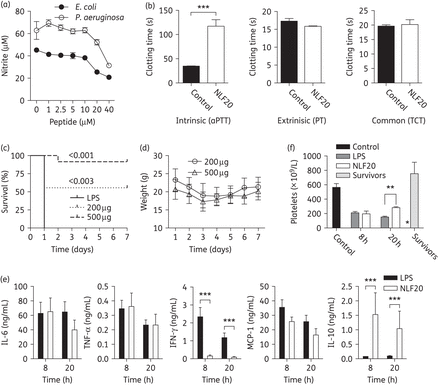
(a) NLF20 significantly blocked nitrite production. RAW264.7 macrophages were stimulated with 10 ng/mL of E. coli or P. aeruginosa LPS in combination with the indicated concentrations of NLF20. (b) NLF20 significantly prolonged aPTT in vitro. Clotting times were determined without (control) or with 20 µM of the peptide after addition of CaCl2 solution. Results illustrating PT and TCT are also presented. (c) Septic shock in C57BL6 mice was induced by intraperitoneal injection of 18 mg/kg E. coli LPS. NLF20 treatment significantly increased survival when compared with control mice. Controls, n = 10; and NLF20, n = 9 (0 h) and 1 h (n = 12) (P < 0.001 and 0.003, respectively). (d) Weight was followed for 7 days. (e) Administration of NLF20 (0.5 mg) selectively modulated the cytokine response compared with control mice. 8 h: control, n = 10; NLF20, n = 10. 20 h: control, n = 14; NLF20, n = 12. Cytokines were measured in blood from animals sacrificed 8 or 20 h after LPS injection. (f) Peptide treatment significantly increased the number of platelets in the LPS-induced shock model. In separate experiments mice were injected with LPS followed by administration of 0.5 mg of NLF20, or buffer. Animals were sacrificed at 8 and 20 h and survivors after 7 days and the number of platelets in blood were counted. *P < 0.005, **P < 0.001; ***P < 0.0001.
Given the observed dual effects on cytokine responses and coagulation, the in vivo efficiency of NLF20 was next evaluated in a standardized mouse model of endotoxin-induced shock.22 A dramatic improvement in survival rate of the animals was seen after treatment with the peptide (Figure 3c). NLF20-treated animals fully regained their weight after 7 days (Figure 3d). Analyses of cytokines 8 and 20 h after LPS injection showed no notable, or only minor, alterations of IL-6 and TNF-α, as well as of the pro-inflammatory cytokines IFN-γ and MCP-1, and a strong increase in the anti-inflammatory IL-10 (Figure 3e). Previous studies have shown that thrombocytopenia is as an important indicator for the severity of sepsis and disseminated intravascular coagulation.35 Therefore, platelet numbers were measured in blood of LPS-injected mice treated with NLF20 at 8, 20 h and 7 days after LPS injection. NLF20-treated animals showed significantly increased platelet numbers at 20 h, indicating that the peptide reduced platelet consumption at later stages in this endotoxin model (Figure 3f). The levels were completely normalized in the survivors after 7 days.
In order to explore whether NLF20 could be effective against invasive P. aeruginosa infection in vivo, the peptide was injected into mice infected with P. aeruginosa. Compared with the controls, immediate treatment with NLF20 yielded significantly lower bacterial numbers in the spleen, liver and kidney of the animals infected with both a low and a high dose of bacteria (Figure 4a). Importantly, the antimicrobial effects, as evidenced by reductions in cfu, were present also after treatment was delayed and given subcutaneously 1 h after infection (Figure 4b). Similarly to the LPS model, platelet levels were increased in the peptide-treated animals, although recovery was not complete within the time-frame of the experiments (Figure 4c). It is also notable that treatment with only one dose of NLF20, particularly when given intraperitoneally, was sufficient to reduce mortality or prolong survival (Figure 4d). Finally, in a second set of experiments, the antimicrobial effects of NLF20 were compared with those of commonly used antibiotics. The results showed that the peptide was able to reduce bacterial levels by 2–3 log, which was comparable to the bacterial reductions obtained with ceftazidime and levofloxacin (Figure 5). To further investigate the potential therapeutic effect of NLF20 in a clinically relevant bacterial sepsis model, we treated P. aeruginosa-infected mice with NLF20 1 and 4 h after bacterial infection. The data showed that the two-dose treatment with NLF20 peptide prolonged mouse survival (Figure 6a), paralleled by a reduced bacterial spread (Figures 6b and Supplementary Data).
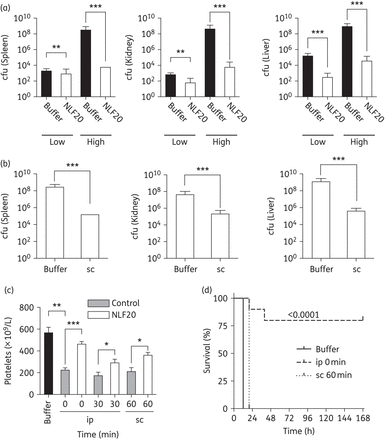
(a) One-hundred microlitres of P. aeruginosa 15159 bacteria (in PBS, ∼2 × 104 or 2 × 108 cfu/mL) was injected intraperitoneally (ip) into C57/BL6 mice. Immediately after bacterial injection, 0.5 mg of NLF20 or PBS alone was injected intraperitoneally into the mice. Liver, spleen and kidney were harvested and cfu was determined 12 h after infection. (b) In a similar infection model (using 2 × 108 cfu/mL P. aeruginosa), intraperitoneal administration, after 1 h, of 0.5 mg of NLF20 or PBS alone was injected subcutaneously (sc). (c) Treatment with NLF20 (intraperitoneally and subcutaneously) increased thrombocyte levels. (d) NLF20 significantly increased survival during P. aeruginosa sepsis. Mice were injected ip with P. aeruginosa as above followed by ip or sc administration of NLF20 (0.5 mg). Survival was followed for 7 days. Whereas sc administration only prolonged survival, ip administration significantly reduced mortality (n = 10 for controls and treated animals; P < 0.0001 for ip administration). *P < 0.005, **P < 0.001; ***P < 0.0001.
![One-hundred microlitres of P. aeruginosa 1559 bacteria (2 × 108 cfu/mL) was injected intraperitoneally into C57/BL6 mice. Treatment was given immediately after [intraperitoneally (ip)] or after 1 h [subcutaneously (sc)] by using 0.5 mg of NLF20, 300 mg/kg ceftazidime or 100 mg/kg levofloxacin. PBS alone was injected as a control. Liver, spleen and kidney were harvested and cfu was determined 12 h after infection. Controls, n = 33; NLF20, n = 14 (ip, 0 h) and n = 10 (sc, 1 h); ceftazidime, n = 12 (ip, 0 h) and n = 14 (sc,1 h); and levofloxacin, n = 2 (ip, 0 h) and n = 5 (sc, 1 h).](https://oup.silverchair-cdn.com/oup/backfile/Content_public/Journal/jac/71/1/10.1093_jac_dkv322/1/m_dkv32205.gif?Expires=1747907419&Signature=QUgl3tvmqFUAoRSZibNyy9Ti6Gx~Z-dyYX17wQe1MZ86fE1jdIC48u9IWVcU2hBRlf0HApLDFMEWWrxK42h1Cj6~0qasp~IFaQnz7GRPuc46Iqsoc3SxOeqNF6mtLvlCfrP3tAzEo4oAf6OW0l-c9bH7gYxypz6I7l9li1g-HC2Lh3zwUTWDnB~IkFtD1DlGTCAjAXetKJrDbp4ofhBDuPvHlfwNsjhZ7pfL5uc6w1azi9IvvhPBnUEOAry1OKUZ2qRSrC-cIdRORWAKcwWWjClViVUseCpBdocV~pmKqfdT3wtsbylMjUyhwMpifkgKkNVLIQUFxNR90nBarpxv-Q__&Key-Pair-Id=APKAIE5G5CRDK6RD3PGA)
One-hundred microlitres of P. aeruginosa 1559 bacteria (2 × 108 cfu/mL) was injected intraperitoneally into C57/BL6 mice. Treatment was given immediately after [intraperitoneally (ip)] or after 1 h [subcutaneously (sc)] by using 0.5 mg of NLF20, 300 mg/kg ceftazidime or 100 mg/kg levofloxacin. PBS alone was injected as a control. Liver, spleen and kidney were harvested and cfu was determined 12 h after infection. Controls, n = 33; NLF20, n = 14 (ip, 0 h) and n = 10 (sc, 1 h); ceftazidime, n = 12 (ip, 0 h) and n = 14 (sc,1 h); and levofloxacin, n = 2 (ip, 0 h) and n = 5 (sc, 1 h).
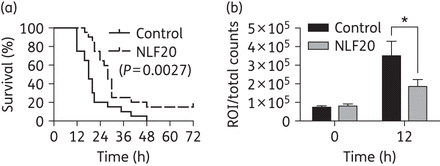
(a) One-hundred microlitres of P. aeruginosa Xen41 bacteria (2–3 × 108 cfu/mL) was injected intraperitoneally (ip) into BALB/c mice. NLF20 peptide (0.5 mg) was administered subcutaneously (sc) 60 min and 4 h after injection of bacteria (n = 20 in each group). (b) The bioluminescence signal of whole mice was measured using regions of interest (ROI) total count analysis at 0 and 12 h after bacterial injection. *P < 0.005.
Discussion
Infections caused by Gram-negative bacteria are increasingly contributing to problems associated with antibiotic resistance and remain a major cause of sepsis.36 During sepsis, multiple proinflammatory cascades, as well as tissue-factor-related coagulative responses, are activated.36 Several clinical trials aimed at inhibiting these coagulative and pro-inflammatory responses have been conducted, including administration of several modulatory substances along with the traditional antibiotic regimen. Examples include antibodies against TNF-α37–39 or endotoxins,40 antagonists of the IL-1 receptor,41,42 IL-640 or platelet-activating factor (PAF) receptors,43 or antithrombin III,44–46 and other agents.36,47–49 Despite promising preclinical results, these drug candidates have all failed when tested in the clinic. While activated protein C (APC) has shown promise in preclinical and clinical studies, clinical trials were recently terminated due to lack of clinical efficiency (http://lillytrials.com/results/Xigris.pdf). Hence the lack of dedicated therapeutics against sepsis remains, and current treatment is largely based on antibiotics in combination with supportive measures.
The capacity of proteolytically activated HCII to target bacteria and bind LPS, as well as its dramatic decrease in plasma during endotoxin shock and bacterial sepsis,24 indicates a therapeutic potential of this protein. In an attempt to increase ‘drugability’, the present study aimed at characterizing the antimicrobial, immunomodulatory and therapeutic potential of an HCII epitope. Thus, like the holoprotein, NLF20 was found to exert antimicrobial effects, particularly against P. aeruginosa, both in standard MIC assays and in physiological buffers, including human plasma. The finding that NLF20 assumes a helical conformation upon LPS binding, as well as interaction with LPS-coated or anionic liposomes,50 further indicates a parallelism between NLF20 and other helical antimicrobial peptides. In relation to its LPS-binding capacity, NLF20 showed anti-inflammatory effects in vitro as well as in vivo, further illustrating the multifunctionality of antimicrobial peptides.
It is also interesting to compare the effects observed for NLF20 (NLFRKLTHRLFRRNFGYTLR) with those of the longer epitope KYE28 (KYEITTIHNLFRKLTHRLFRRNFGYTLR), containing the NLF20 sequence, as well as those of the corresponding truncation at the other end of KYE28 (KYE21; KYEITTIHNLFRKLTHRLFR). While both KYE21 and NLF20 display antimicrobial and anti-inflammatory effects qualitatively similar to those displayed by KYE28, the relative importances of the antimicrobial and anti-inflammatory effects are shifted, such that the main effect of KYE21 is anti-inflammatory, while NLF20 displays more potent antimicrobial effects, along with partly retained anti-endotoxic effects, as shown here. The more potent antimicrobial activity of NLF20 was previously demonstrated to be due to potent membrane destabilization of the highly charged NLF20, in turn facilitated by higher electrostatically and hydrophobically driven adsorption to anionic lipid membranes. In contrast, NLF20 displays somewhat poorer capacity than KYE28 and KYE21 to provide multiple scavenging alternatives to LPS-induced NF-κB activation, including peptide-induced scavenging of LPS at human cell surfaces (mediated by lower adsorption and positive potential build-up at zwitterionic membranes for NLF20 than for KYE21 and KYE28) and LPS micelle packing disruption (mediated by a delicate balance of peptide binding to the lipid A and the polysaccharide regions of LPS, and resulting effects on packing constraints in LPS micelles).50
It must be stressed that the activities of NLF20 may not necessarily reflect all possible activities mediated by the proteolytically activated HCII molecule. Thus, it is possible that the bacterial binding and LPS interactions mediated by this helix D peptide may be complemented by other actions of distant structural motifs, potentially mediating other cell effects, phagocytosis or distinct ligand interactions. Although some evidence suggests that C-terminals of serpins, such as a1-antitrypsin and antithrombin III, may interact with cell receptors and mediate phagocytosis, little is known about such actions for HCII, and clearly this warrants further investigations. Nevertheless, the present data indicate, with these limitations, that the current strategy of selecting one functional epitope of HCII may have therapeutic implications and also benefits due to a less complex mode of action, as well as easier production and enhanced stability of the peptide, while maintaining the endogenous character of the host response.
Funding
This work was supported by grants from the Swedish Research Council (projects 2012-1842 and 2012-1883), the Knut and Alice Wallenberg Foundation, the Welander-Finsen, Thelma-Zoegas, Crafoord, Alfred Österlund, Lundgrens and Kock Foundations, The Torsten Söderberg Foundation, Swedish Foundation for Strategic Research, Ximmune AB and the Swedish Government Funds for Clinical Research.
Transparency declarations
A. S. and M. Malmsten are founders of and own shares in XImmune AB, a company developing anti-inflammatory peptides for human therapy. All other authors: none to declare.
Acknowledgements
We wish to thank Ms Lotta Wahlberg and Ms Ann-Charlotte Strömdahl for expert technical assistance.
References