-
PDF
- Split View
-
Views
-
Cite
Cite
Alina Iulia Chiriac, Florian Kloss, Jonas Krämer, Cuong Vuong, Christian Hertweck, Hans-Georg Sahl, Mode of action of closthioamide: the first member of the polythioamide class of bacterial DNA gyrase inhibitors, Journal of Antimicrobial Chemotherapy, Volume 70, Issue 9, September 2015, Pages 2576–2588, https://doi.org/10.1093/jac/dkv161
- Share Icon Share
Abstract
The spread of MDR bacteria represents a serious threat to human society and novel antibiotic drugs, preferably from new chemical classes, are urgently needed. Closthioamide was isolated from the strictly anaerobic bacterium Clostridium cellulolyticum and belongs to a new class of natural products, the polythioamides. Here, we investigated the antimicrobial activity and mechanism of action of closthioamide.
For assessing the antimicrobial activity of closthioamide, MIC values and killing kinetics were determined. To identify its target pathway, whole-cell-based assays were used including analysis of macromolecular synthesis and recording the susceptibility profile of a library of clones with down-regulated potential target genes. Subsequently, the inhibitory effect of closthioamide on the activity of isolated target enzymes, e.g. DNA gyrase and topoisomerase IV, was evaluated.
Closthioamide had broad-spectrum activity against Gram-positive bacteria. Notably, closthioamide was very potent against MRSA and VRE strains. Closthioamide impaired DNA replication and inhibited DNA gyrase activity, in particular the ATPase function of gyrase and of topoisomerase IV, whereas there was little effect on the cleavage-rejoining function. Closthioamide also inhibited the relaxation activity of DNA gyrase, which does not require ATP hydrolysis, and thus may allosterically rather than directly interfere with the ATPase activity of gyrase. Cross-resistance to ciprofloxacin and novobiocin could not be detected in experimental mutants and clinical isolates.
Closthioamide, a member of an unprecedented class of antibiotics, is a potent inhibitor of bacterial DNA gyrase; however, its molecular mechanism differs from that of the quinolones and aminocoumarins.
Introduction
The healthcare sector is currently facing tremendous challenges with MDR or even pan-resistant pathogens refractory to virtually all clinically used antibiotics.1 Only very few new drugs have reached the market over the last three decades and there is an urgent need for discovery of novel antibacterial compounds, preferably from new chemical classes with new mechanisms of action.2–4
Most known antibiotics derive from natural products, which continue to be the richest source for new lead compounds with antimicrobial activity.5 Innovative screening methodologies and novel ecological niches have been explored to increase the chances of finding new structural classes. Moreover, new sequencing technologies gave unprecedented insights into the biosynthetic capacity and metabolism of new microbial isolates and entire communities with most species from such consortia being unculturable.6,7 The greatest challenge ahead is to make use of the immense biosynthetic potential, since the vast majority of natural product biosynthetic gene clusters are silent under laboratory growth conditions.8 An excellent example for the genomics-based discovery of new natural products is the recent identification of closthioamide (Figure 1) produced by Clostridium cellulolyticum. Anaerobic bacteria had been generally believed not to be able to produce secondary metabolites and thus are so far fully unexploited.9,10 Genome mining of C. cellulolyticum demonstrated the presence of putative gene clusters encoding polyketide synthases and non-ribosomal peptide synthetases;11,12 however, no bioactive compounds could be identified in culture supernatants. Addition of an aqueous soil extract to a C. cellulolyticum culture then led to the discovery of closthioamide, the first antibiotic compound isolated from an obligate anaerobe.12

Closthioamide belongs to a new class of natural products, the polythioamides. The structure of closthioamide features six thioamide groups assembled into a symmetrical molecule. Thioamides are extremely rare in nature. However, synthetic thioamides have been used in medicinal chemistry as isosters for amide bonds to improve the stability and rigidity of compounds.13,14 Closthioamide proved to have potent anti-Gram-positive activity, including against MRSA and VRE, and moderate toxicity.12
To date, the closthioamide biosynthetic route is unknown, but manipulation of the regulatory elements involved in the global activation of secondary metabolism sustained the production of closthioamide and also permitted the isolation of seven congeners of closthioamide.15 Evaluation of the antimicrobial activity of derivatives and congeners demonstrated the relevance of the number of thioamide moieties, the impact of terminal residues, the overall size of the backbone and the distinct length of spacer units for the antibacterial activity.16 Furthermore, it was found that closthioamide is a selective Cu(I) chelator.17
Most known antibiotics act on a few well-established bacterial targets, such as the ribosome, cell wall biosynthesis and DNA gyrase.18 DNA gyrase is essential in all bacteria, but absent in higher eukaryotes. Thus, DNA gyrase is considered as one of the best-validated targets in antibacterial drug development.19–22 Here, we report that closthioamide represents a novel type of DNA replication inhibitor, which acts through interfering with the activity of type II topoisomerases, DNA gyrases and topoisomerase IV.
Materials and methods
Chemicals and enzymes
Closthioamide was provided by total synthesis.23 Closthioamide stock solution was prepared at 10 mg/mL in DMSO.
All other chemicals were obtained from Sigma–Aldrich, unless specified otherwise. The radioactive reagents d-[1-3H]glucosamine hydrochloride (ART 0292A), l-[14C(U)]isoleucine (ARC 0672), [2-14C]thymidine (MC 104) and [5-3H]uridine (MT 602) were purchased from Hartmann Analytic (Braunschweig, Germany).
Escherichiacoli DNA gyrase, E. coli DNA topoisomerase IV, E. coli DNA topoisomerase I, S. aureus DNA gyrase, relaxed DNA (pHOT1) and catenated DNA were obtained from TopoGEN (TopoGEN, Port Orange, FL, USA). S. aureus topoisomerase IV, supercoiled DNA (pBR322) and the fluorescence-based assay kits for E. coli DNA ligase, E. coli DNA primase and E. coli DNA gyrase were purchased from ProFoldin (Hudson, USA). Calf thymus topoisomerase I was acquired from Invitrogen (Carlsbad, CA, USA).
MIC
The MIC was determined for the laboratory strains as follows. The assays were performed in sterile 96-well polystyrene microplates in 200 μL of cation-adjusted Mueller–Hinton (MH) broth. A serial dilution of the compound to test was made in 100 μL of medium and inoculated with 100 μL of fresh cell culture containing ∼105 cfu/mL and incubated at 37°C for 20 h. The MIC was defined as the lowest concentration of antibiotic that inhibited visible growth.
For the clinical isolates, MIC90s were determined by the broth dilution method in 96-well microtitre plates (Art. No. 650101; Greiner Bio-One, Frickenhausen, Germany) according to CLSI guideline M07-A9. For that, 90 μL of cell culture in cation-adjusted MH II broth (Becton Dickinson, Sparks, MD, USA) was used to inoculate the microtitre plates containing 10 μL of the antibiotic solutions at a 10× higher concentration of the final concentration.
Strain susceptibility
Strain susceptibility was determined by using the agar diffusion method. All strains were grown overnight in TSB broth. Then, 10 μL of the overnight culture was used to inoculate 4 mL of soft MH agar that was poured onto an MH agar plate and left to solidify. A 3 μL drop of a 1 mg/mL closthioamide solution or 3 μL of DMSO was added and the plates were incubated for 24 h at 37°C.
The inhibition zone was defined as the diameter of the clear zone (no growth) around the place where the drop was added.
Killing kinetics
Staphylococcus simulans 22 cells were grown overnight in MH broth and diluted in fresh medium to an OD600 of 0.1. One part of the culture was run as control. To the other batches, closthioamide was added in different concentrations corresponding to 2× and 5× MIC. DMSO corresponding to the highest closthioamide concentration sample was added to the control sample. Aliquots were taken at defined intervals, diluted in 10 mM potassium phosphate buffer and 100 μL of several decimal dilutions was plated in duplicate onto MH agar. The viable count was monitored up to 6 h. The plates were incubated at 37°C and the cfu/mL were counted after 24 h. Only plates containing 30–300 cfu/mL were considered for evaluation.
Macromolecule biosynthesis
Synthesis of macromolecules was followed by measuring the radioactivity in cells supplemented with the radiolabelled precursors [5-3H]uridine, d-[1-3H]glucosamine hydrochloride, l-[14C]isoleucine or [2-14C]thymidine. S. simulans 22 cells were grown in half-strength MH broth at 37°C to an OD600 of 0.5 and then diluted to an OD600 of 0.1–0.2. Subsequently, the precursors were added at final concentrations of 1.0 and 0.15 μCi/mL for the 3H- and 14C-radiolabelled precursors, respectively. After 15 min (30 min in the case of [3H]glucosamine), the culture was split: one part was run as untreated control while the other parts received closthioamide or a control antibiotic known to inhibit the pathway. Incorporation was monitored for up to 40 min and 200 μL samples were taken at different timepoints. Occasionally, only one timepoint was chosen and the concentration of closthioamide was varied. Samples were mixed with 2 mL of ice-cold precipitation buffer [10% trichloroacetic acid (TCA) and 1 M NaCl] and incubated for ≥30 min on ice before filtering through glass microfibre filters (Whatman). Filters were further washed with 5 mL of washing buffer (2.5% TCA and 1 M NaCl) and transferred to counting vials. After filters had dried, vials were filled with scintillation fluid (Filtersafe; Zinsser, Frankfurt, Germany). The radioactivity was measured in a Tri-Carb® 2810TR LSA Liquid Scintillation Analyzer (Perkin Elmer).
Fluorescence microscopy
Bacillus subtilis 168 was grown to mid-exponential phase at 37°C, diluted to an OD600 of 0.1 and treated with 3 μg/mL closthioamide, treated with 3 μg/mL ciprofloxacin or left untreated. Samples were taken at different timepoints and analysed by phase-contrast or fluorescence microscopy after staining the cells with 0.25 μg/mL DAPI (Sigma–Aldrich) in order to visualize the DNA. A 1 μL sample of the stained cells was placed onto a microscope slide covered with a thin layer of 1% agarose and analysed using a Zeiss Axiovert 200M microscope equipped with a Photometrics CoolSnap HQ CCD camera (Roper Scientific). Image acquisition was performed using the microscope software ZEN2011.
Cell-based assay for target-specific inhibitors: susceptibility profile
The susceptibility of antisense RNA down-regulated clones to closthioamide and control antibiotics was determined as described by Forsyth et al.24 The S. aureus RN4220 antisense clones were kindly provided by Merck (USA).
All S. aureus RN4220 antisense recombinant strains, each carrying a xylose-inducible plasmid expressing an antisense RNA that generates reduced expression of an essential gene, were grown overnight in LB supplemented with 34 μg/mL chloramphenicol (LB/chloramphenicol). The xylose concentration necessary for inhibiting growth was determined for each strain as described previously for the MIC determination. Exponential cultures were diluted to a final OD600 of 0.002 in the same medium in four flasks: one contained no xylose and the other three received xylose at 1×, 0.5× and 0.25× the MIC concentration in order to achieve 20%–30% growth inhibition due to down-regulation of essential genes (in the control sample). After 210 min of shaking at 37°C, the resulting cultures were diluted to an OD600 of 0.0005 in the same medium (LB/chloramphenicol) and twice the xylose concentration. Next, 75 μL aliquots of the cell suspension were dispensed into 96-well polystyrene microplates containing a serial dilution of the antibiotic compound in 75 μL of LB/chloramphenicol. All plates were made in duplicate. Seven serial dilutions with a factor of 1.5 were made starting from 2 μg/mL compound and the eighth well was left untreated as control. Plates were incubated at 37°C and cell growth was monitored (OD600) using a multichannel absorbance plate reader (Sunrise™; Tecan) for 18 h. The percentage of growth inhibition with regard to the untreated control was plotted and the IC50 values (concentration of a compound that inhibits growth by 50%) for the antisense-induced and non-induced cultures were determined in the linear range. The increase in susceptibility was calculated by dividing the IC50 for the control culture (without xylose) by the IC50 value for the down-regulated culture (with xylose) of a given antibiotic.
Fluorescence-based assays for DNA ligase, DNA primase and DNA gyrase activity
These assays were performed according to the provider's indications. Blanks without enzyme were prepared for each different sample.
Inhibition of DNA gyrase (supercoiling and relaxation) and topoisomerase IV (relaxation and decatenation) activity
DNA gyrase supercoiling and topoisomerase IV relaxation and decatenation activities were assayed following the provider's instructions. The amount of enzyme and the incubation time were adjusted in order to modify by 50%–100% the topology of 0.125 μg of relaxed DNA (gyrase supercoiling activity), 0.125 μg of supercoiled DNA (gyrase and topoisomerase IV relaxation activity) or 0.2 μg of kDNA (topoisomerase IV decatenation activity) in 20 μL of reaction volume at 37°C in the corresponding buffer: (i) E. coli DNA gyrase supercoiling buffer (35 mM Tris HCl pH 7.5, 24 mM KCl, 4 mM MgCl2, 2 mM DTT, 1.8 mM spermidine, 6.5% glycerol, 100 μg/mL BSA and 1 mM ATP); (ii) S. aureus DNA gyrase supercoiling buffer (75 mM Tris HCl pH 7.5, 30 mM KCl, 7.5 mM MgCl2, 7.5 mM DTT, 75 μg/mL BSA, 500 mM potassium glutamate and 2 mM ATP); (iii) E. coli topoisomerase IV relaxation buffer (40 mM HEPES KOH pH 8, 100 mM potassium glutamate, 10 mM magnesium acetate, 10 mM DTT, 50 μg/mL BSA and 2 mM ATP); (iv) S. aureus topoisomerase IV relaxation buffer (20 mM Tris HCl pH 8, 35 mM ammonium acetate, 8 mM MgCl2, 1 mM DTT, 4.6% glycerol, 0.005% Brij35 and 1 mM ATP); (v) E. coli topoisomerase IV decatenation buffer (idem E. coli topoisomerase IV relaxation buffer); and (vi) S. aureus topoisomerase IV decatenation buffer (50 mM Tris HCl pH 7.5, 5 mM MgCl, 5 mM DTT, 1.5 mM ATP, 350 mM potassium glutamate and 0.05 mg/mL albumin).
Gyrase relaxation activity was assayed in the corresponding supercoiling buffer without ATP and spermidine and using supercoiled DNA as substrate.
Different concentrations of closthioamide or the corresponding amount of DMSO was added to the reaction mix prior to enzyme addition. In order to facilitate a direct comparison, ciprofloxacin and novobiocin were tested in the same experiment. The reactions were stopped by the addition of stop/loading buffer (final concentration: 1% SDS, 5% glycerol and 0.025% bromophenol blue) and analysed by gel electrophoresis in a 1% agarose gel in TAE buffer (40 mM Tris-acetate and 0.01 M EDTA pH 8.3). DNA was stained with 0.5 μg/mL ethidium bromide, visualized with UV light and photodocumented. Quantification of the intensity of the bands was performed using GelQuant.NET image analysis software provided by BiochemLabSolutions.com and IC50 was determined in the linear range.
Detection of enzyme-mediated DNA cleavage
DNA cleavage was analysed by measuring the amount of linear DNA generated in the presence of the enzyme as described by Fortune and Osheroff.25 The reactions were performed as described for the E. coli gyrase supercoiling assay using 4 U of enzyme and 0.5 μg of relaxed or supercoiled DNA in the presence or absence of 1 mM ATP. The reaction was incubated at 37°C for 30 min and stopped by sequentially adding 0.5% SDS and 25 mM EDTA final concentration. Next, proteinase K (100 μg/mL) was added. The samples were incubated for 30 min at 45°C, mixed with loading dye (5% glycerol and 0.025% bromophenol blue), heated to 70°C for 3 min and analysed in a 1% agarose gel in TAE buffer containing 0.5 μg/mL ethidium bromide.
ATPase function inhibition
The ATPase activity of E. coli gyrase and topoisomerase IV was measured by quantifying the release of inorganic phosphate with a malachite green colorimetric assay as described by Singh et al.26 The amount of enzyme was adjusted to the sensitivity of the assay. For E. coli gyrase, 1 U of enzyme was tested in 50 μL of 100 mM Tris HCl buffer pH 7.4, 100 mM KCl, 5 mM MgCl2, 200 μM ATP and 0.125 μg of relaxed DNA. For E. coli topoisomerase IV, 2 U of enzyme were used in 50 μL of buffer containing 40 mM HEPES KOH pH 8, 100 mM potassium glutamate, 10 mM magnesium acetate, 200 μM ATP and 0.125 μg of supercoiled DNA. Closthioamide (100 μM) or the corresponding DMSO amount was added to the reaction mix prior to enzyme addition and ATP was added in the last instance to start the reaction. Blank samples were prepared containing enzyme dilution buffer in place of enzyme. Novobiocin and ciprofloxacin were included as positive and negative drug controls, respectively. Reactions were incubated for 60 min at 37°C, centrifuged for 3 min at 13 000 rpm and 40 μL was used for the detection of the inorganic phosphate released. The reaction was stopped by the addition of 20 μL (1/2 of the initial volume) malachite green reagent (0.016% malachite green and 0.4% polyvinyl alcohol). Colour determination was achieved with the addition of 20 μL (1/2 of the initial volume) molybdate solution (0.04 M ammonium molybdate and 2 N H2SO4). The samples were mixed thoroughly, incubated for 15 min at room temperature and the absorbance was read at 620 nm using a multichannel absorbance plate reader (Sunrise™; Tecan). The residual volume of 10 μL from the reaction mix was used to verify the supercoiling/relaxation activity in the gel assay as described before.
DNA intercalation assays (topoisomerase I DNA unwinding and topoisomerase I inhibition)
The ability of closthioamide to intercalate into DNA was determined by a topoisomerase I-catalysed unwinding assay as described by Sappal et al.27
Relaxed DNA (0.25 μg/mL) was incubated with different concentrations (25–400 μM) of closthioamide, amsacrine (positive control), etoposide (negative control), DMSO or H2O and 2 U of calf thymus topoisomerase I (Invitrogen) in 20 μL of reaction buffer (50 mM Tris HCl pH 7.5, 50 mM KCl, 10 mM MgCl2, 0.5 mM DTT, 0.1 mM EDTA and 30 μg/mL BSA). Reactions were incubated for 60 min at 37°C and stopped by adding 5 μL of stop/loading buffer. Proteinase K (100 μg/mL) was added and the reaction was further incubated for 45 min at 37°C and subsequently extracted with one volume of chloroform : isoamyl alcohol 24 : 1 (v/v). The aqueous phases of the samples were subjected to electrophoresis in a 1% agarose gel in TAE buffer. DNA bands were stained with 0.5 μg/mL ethidium bromide, visualized with UV light and photodocumented.
The ability of the above-mentioned compounds to inhibit topoisomerase I was assayed as described for the intercalation assay, although supercoiled DNA was used as a substrate instead of relaxed DNA.
Determination of resistance rate
The resistance frequency of the laboratory strains S. aureus RN4220 and S. simulans 22 was determined by spreading bacterial isolates (∼108 cfu) onto MH agar plates containing closthioamide, ciprofloxacin or novobiocin in concentrations representing 5× to 10× the MIC or left untreated. The resistant colonies were counted after incubation at 37°C for 24 h. To determine the resistance frequency of the clinical isolate S. aureus 133, an inoculum of ∼1.5 × 109 cfu was spread onto MH agar plates containing antibiotic at 4× the agar MIC concentration and the plates were incubated at 37°C for 48 h. The resistance frequency was calculated by dividing the resistant cfu/mL (treated plates) by the cfu/mL of the untreated plates.
Results
Antimicrobial activity
Closthioamide showed broad-spectrum activity against Gram-positive bacteria and limited activity against several E. coli strains in agar diffusion tests (data not shown). In microbroth dilution assays, closthioamide was highly active against Gram-positive cocci including MRSA and VRE strains (Table 1). It also showed resistance-breaking antibacterial activities against clinically isolated staphylococci, streptococci and enterococci, with MIC90 values ranging from 0.027 to 0.44 mg/L (Table 2). The data demonstrate that the natural product already exhibited superior antibacterial activity over marketed standard antibiotics. The activity against E. coli strains was intermediate (2.5–3.75 mg/L); however, susceptibility of E. coli BW25113 could be increased by 6-fold when closthioamide was used in combination with polymyxin B nonapeptide (PMBN), an indication of substantial outer membrane barrier effects. Moreover, a 12-fold increase in susceptibility was observed with a drug efflux pump-deficient mutant, E. coli JW0452 (ΔacrA). When closthioamide was used in combination with PMBN against the ΔacrA mutant, >100-fold enhanced susceptibility was achieved (Table 1). Closthioamide had a bactericidal effect as shown by the decrease of the cfu/mL within 6 h of exposure (Figure 2).
Antimicrobial activity of closthioamide compared with established drugs against laboratory strains
Strain . | . | Gram+/Gram− . | MIC (mg/L)a . | ||
---|---|---|---|---|---|
. | closthioamide . | closthioamide + PMBNb . | ciprofloxacin . | ||
MRSA | LT-1334 | Gram+ | 0.14 | NA | ND |
MRSA | USA300 | Gram+ | 0.04 | NA | >5 |
S. aureus | SG 511 | Gram+ | 0.07 | NA | ND |
S. aureus | RN4220 | Gram+ | 0.04 | NA | 0.2 |
S. simulans | 22 | Gram+ | 0.05 | NA | 0.03 |
Enterococcus faecium | I-11054 | Gram+ | 0.04 | NA | >25 |
VRE | BM 4147 | Gram+ | 0.4 | NA | 1.6 |
B. subtilis | 168 | Gram+ | 0.23 | NA | ND |
E. coli | BW25113c | Gram− | 3.75 | 0.625 | ND |
E. coli | JW0452 (ΔacrA)d | Gram− | 0.31 | 0.035 | ND |
E. coli | O-19592 | Gram− | 2.5 | ND | 12.5 |
E. coli | I-11054 | Gram− | 3.5 | ND | ND |
Strain . | . | Gram+/Gram− . | MIC (mg/L)a . | ||
---|---|---|---|---|---|
. | closthioamide . | closthioamide + PMBNb . | ciprofloxacin . | ||
MRSA | LT-1334 | Gram+ | 0.14 | NA | ND |
MRSA | USA300 | Gram+ | 0.04 | NA | >5 |
S. aureus | SG 511 | Gram+ | 0.07 | NA | ND |
S. aureus | RN4220 | Gram+ | 0.04 | NA | 0.2 |
S. simulans | 22 | Gram+ | 0.05 | NA | 0.03 |
Enterococcus faecium | I-11054 | Gram+ | 0.04 | NA | >25 |
VRE | BM 4147 | Gram+ | 0.4 | NA | 1.6 |
B. subtilis | 168 | Gram+ | 0.23 | NA | ND |
E. coli | BW25113c | Gram− | 3.75 | 0.625 | ND |
E. coli | JW0452 (ΔacrA)d | Gram− | 0.31 | 0.035 | ND |
E. coli | O-19592 | Gram− | 2.5 | ND | 12.5 |
E. coli | I-11054 | Gram− | 3.5 | ND | ND |
NA, not applicable; ND, not determined.
aAverage of two or more experiments made in duplicate.
bPMBN at 50 μg/mL; induces membrane permeabilization.
cParent strain of E. coli JW0452.
dDrug efflux pump-deficient mutant.
Antimicrobial activity of closthioamide compared with established drugs against laboratory strains
Strain . | . | Gram+/Gram− . | MIC (mg/L)a . | ||
---|---|---|---|---|---|
. | closthioamide . | closthioamide + PMBNb . | ciprofloxacin . | ||
MRSA | LT-1334 | Gram+ | 0.14 | NA | ND |
MRSA | USA300 | Gram+ | 0.04 | NA | >5 |
S. aureus | SG 511 | Gram+ | 0.07 | NA | ND |
S. aureus | RN4220 | Gram+ | 0.04 | NA | 0.2 |
S. simulans | 22 | Gram+ | 0.05 | NA | 0.03 |
Enterococcus faecium | I-11054 | Gram+ | 0.04 | NA | >25 |
VRE | BM 4147 | Gram+ | 0.4 | NA | 1.6 |
B. subtilis | 168 | Gram+ | 0.23 | NA | ND |
E. coli | BW25113c | Gram− | 3.75 | 0.625 | ND |
E. coli | JW0452 (ΔacrA)d | Gram− | 0.31 | 0.035 | ND |
E. coli | O-19592 | Gram− | 2.5 | ND | 12.5 |
E. coli | I-11054 | Gram− | 3.5 | ND | ND |
Strain . | . | Gram+/Gram− . | MIC (mg/L)a . | ||
---|---|---|---|---|---|
. | closthioamide . | closthioamide + PMBNb . | ciprofloxacin . | ||
MRSA | LT-1334 | Gram+ | 0.14 | NA | ND |
MRSA | USA300 | Gram+ | 0.04 | NA | >5 |
S. aureus | SG 511 | Gram+ | 0.07 | NA | ND |
S. aureus | RN4220 | Gram+ | 0.04 | NA | 0.2 |
S. simulans | 22 | Gram+ | 0.05 | NA | 0.03 |
Enterococcus faecium | I-11054 | Gram+ | 0.04 | NA | >25 |
VRE | BM 4147 | Gram+ | 0.4 | NA | 1.6 |
B. subtilis | 168 | Gram+ | 0.23 | NA | ND |
E. coli | BW25113c | Gram− | 3.75 | 0.625 | ND |
E. coli | JW0452 (ΔacrA)d | Gram− | 0.31 | 0.035 | ND |
E. coli | O-19592 | Gram− | 2.5 | ND | 12.5 |
E. coli | I-11054 | Gram− | 3.5 | ND | ND |
NA, not applicable; ND, not determined.
aAverage of two or more experiments made in duplicate.
bPMBN at 50 μg/mL; induces membrane permeabilization.
cParent strain of E. coli JW0452.
dDrug efflux pump-deficient mutant.
Antimicrobial activity of closthioamide compared with established drugs against clinically isolated strains
Strain . | Gram+/Gram− . | MIC90 (mg/L)a . | |||||
---|---|---|---|---|---|---|---|
closthioamide . | ciprofloxacin . | moxifloxacin . | linezolid . | vancomycin . | daptomycin . | ||
S. aureus | Gram+ | 0.434 | >17 | 20 | 4.22 | 4.54 | 2.53 |
Staphylococcus epidermidis | Gram+ | 0.438 | >8.3 | 5.02 | 2.11 | 9.06 | 2.53 |
Streptococcus pyogenes | Gram+ | 0.027 | 0.517 | 0.156 | 1.06 | 0.565 | 0.077 |
Streptococcus pneumoniae | Gram+ | 0.108 | 2.07 | 0.313 | 1.01 | 0.565 | 0.6.32 |
E. faecalis | Gram+ | 0.431 | >17 | 10 | 2.11 | >72.5 | 1.264 |
E. faecium | Gram+ | 0.435 | >17 | 20 | 2.11 | >72.5 | 2.53 |
Strain . | Gram+/Gram− . | MIC90 (mg/L)a . | |||||
---|---|---|---|---|---|---|---|
closthioamide . | ciprofloxacin . | moxifloxacin . | linezolid . | vancomycin . | daptomycin . | ||
S. aureus | Gram+ | 0.434 | >17 | 20 | 4.22 | 4.54 | 2.53 |
Staphylococcus epidermidis | Gram+ | 0.438 | >8.3 | 5.02 | 2.11 | 9.06 | 2.53 |
Streptococcus pyogenes | Gram+ | 0.027 | 0.517 | 0.156 | 1.06 | 0.565 | 0.077 |
Streptococcus pneumoniae | Gram+ | 0.108 | 2.07 | 0.313 | 1.01 | 0.565 | 0.6.32 |
E. faecalis | Gram+ | 0.431 | >17 | 10 | 2.11 | >72.5 | 1.264 |
E. faecium | Gram+ | 0.435 | >17 | 20 | 2.11 | >72.5 | 2.53 |
aAverage of two or more experiments made in duplicate.
Antimicrobial activity of closthioamide compared with established drugs against clinically isolated strains
Strain . | Gram+/Gram− . | MIC90 (mg/L)a . | |||||
---|---|---|---|---|---|---|---|
closthioamide . | ciprofloxacin . | moxifloxacin . | linezolid . | vancomycin . | daptomycin . | ||
S. aureus | Gram+ | 0.434 | >17 | 20 | 4.22 | 4.54 | 2.53 |
Staphylococcus epidermidis | Gram+ | 0.438 | >8.3 | 5.02 | 2.11 | 9.06 | 2.53 |
Streptococcus pyogenes | Gram+ | 0.027 | 0.517 | 0.156 | 1.06 | 0.565 | 0.077 |
Streptococcus pneumoniae | Gram+ | 0.108 | 2.07 | 0.313 | 1.01 | 0.565 | 0.6.32 |
E. faecalis | Gram+ | 0.431 | >17 | 10 | 2.11 | >72.5 | 1.264 |
E. faecium | Gram+ | 0.435 | >17 | 20 | 2.11 | >72.5 | 2.53 |
Strain . | Gram+/Gram− . | MIC90 (mg/L)a . | |||||
---|---|---|---|---|---|---|---|
closthioamide . | ciprofloxacin . | moxifloxacin . | linezolid . | vancomycin . | daptomycin . | ||
S. aureus | Gram+ | 0.434 | >17 | 20 | 4.22 | 4.54 | 2.53 |
Staphylococcus epidermidis | Gram+ | 0.438 | >8.3 | 5.02 | 2.11 | 9.06 | 2.53 |
Streptococcus pyogenes | Gram+ | 0.027 | 0.517 | 0.156 | 1.06 | 0.565 | 0.077 |
Streptococcus pneumoniae | Gram+ | 0.108 | 2.07 | 0.313 | 1.01 | 0.565 | 0.6.32 |
E. faecalis | Gram+ | 0.431 | >17 | 10 | 2.11 | >72.5 | 1.264 |
E. faecium | Gram+ | 0.435 | >17 | 20 | 2.11 | >72.5 | 2.53 |
aAverage of two or more experiments made in duplicate.
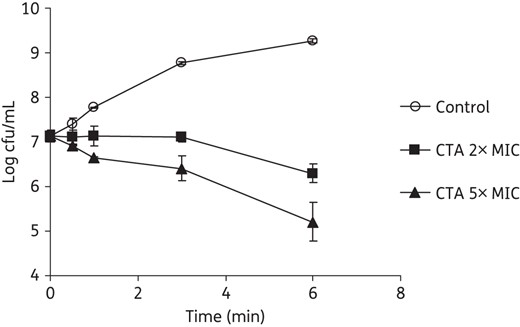
Killing kinetics of closthioamide on S. simulans 22. Closthioamide was applied at 2× and 5× MIC and the cfu/mL were determined at different timepoints. CTA, closthioamide.
Pathway identification
Macromolecular biosynthesis
Closthioamide strongly inhibited the incorporation of radioactively labelled thymidine into DNA and only moderately the incorporation of uridine into RNA, whereas no inhibition of protein and cell wall biosynthesis was observed (Figure 3a). The preferential inhibitory effect on thymidine incorporation became more obvious when different concentrations of closthioamide were compared (Figure 3b), indicating that closthioamide interferes primarily with DNA metabolism. This effect was further substantiated using fluorescence microscopy and DNA staining. Closthioamide-treated cells showed an abnormal DNA condensation pattern, which was comparable to the result obtained with the type II topoisomerase inhibitor ciprofloxacin (Figure 4).

Effect on macromolecule synthesis. (a) Incorporation of the radiolabelled precursor into DNA, RNA, proteins and peptidoglycan in S. simulans 22 whole cells treated with 5× MIC of closthioamide and known inhibitors of the pathways at different timepoints. (b) Relative inhibition of radiolabelled precursor incorporation into DNA and RNA after 20 min of exposure to different concentrations of closthioamide. CTA, closthioamide.
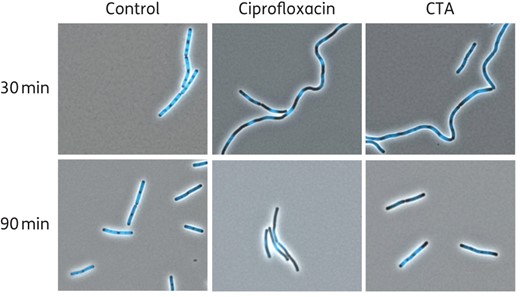
Fluorescence microscopy images of B. subtilis cells treated with closthioamide, treated with ciprofloxacin or left untreated (Control). DNA was stained with DAPI dye. CTA, closthioamide. This figure appears in colour in the online version of JAC and in black and white in the print version of JAC.
Susceptibility profile of antisense clones
To confirm DNA synthesis as the targeted pathway, we studied the susceptibility of clones in which the genes of potential target proteins are down-regulated using antisense strategies. Reduced expression of genes associated with the affected pathway induces increased susceptibility to the respective inhibitor enabling the acquisition of susceptibility profiles that are indicative of the target pathway or even the molecular target.24,28 Using the antisense library described by Donald et al.,28S. aureus RN4220 clones engineered to reduce the expression of essential genes involved mainly in DNA replication and nucleoside biosynthesis were analysed (Table 3). Moderate, but consistent, increased susceptibility to closthioamide was observed for most of the clones in which genes involved in the DNA replication and less for those involved in nucleoside biosynthesis and transcription were down-regulated. Moreover, closthioamide had no effect on the rpsE and infA antisense clones, which respond severely to DNA-intercalating agents.28 DNA primase (dnaG), DNA helicase (dnaC) and DNA ligase (dnlJ) antisense clones showed the highest susceptibility to closthioamide followed by the type II DNA topoisomerases (gyrA, gyrB, parC and parE). All these clones were also susceptible to ciprofloxacin and novobiocin in control assays.
Susceptibility of clones having essential genes down-regulated to closthioamide and the known gyrase inhibitors ciprofloxacin and novobiocin
Down-regulated gene . | Function . | Fold increase in susceptibilitya . | ||
---|---|---|---|---|
closthioamide . | ciprofloxacin . | novobiocin . | ||
DNA replication | ||||
dnaA | chromosomal replication initiator protein | 1.15 | ||
dnaG | DNA primase | 1.84 | 2.28 | 2.68 |
dnaC | replicative DNA helicase | 1.61 | 1.98 | 2.29 |
dnaE | DNA polymerase III alpha chain | 1.33 | ||
dnaK | molecular chaperone | 1.08 | ||
dnaX | DNA polymerase III gamma and tau subunits | 1.20 | ||
polC | DNA polymerase III alpha subunit | 0.95 | ||
holB | DNA polymerase III delta prime subunit | 1.04 | 2.81 | 2.74 |
polA | DNA polymerase I | 1.07 | ||
SAV1636 | single-strand DNA exonuclease | 0.97 | ||
dnlJ | DNA ligase (NAD+) | 1.88 | 1.74 | 1.32 |
hu | DNA-binding protein II | 1.33 | 2.50 | 2.70 |
ssb | single-strand DNA-binding protein | 1.21 | ||
gyrA | DNA gyrase subunit A | 1.31 | 2.47 | 1.53 |
gyrB | DNA gyrase subunit B | 1.43 | 1.68 | 1.55 |
parC | topoisomerase IV subunit A | 1.31 | 2.93 | 2.31 |
parE | topoisomerase IV subunit B | 1.36 | 2.98 | 3.77 |
topA | DNA topoisomerase I | 1.09 | ||
topB | DNA topoisomerase III | 1.08 | ||
Nucleic acid synthesis | ||||
thyA | thymidylate synthase | 0.90 | ||
adk | adenylate kinase | 1.04 | ||
tmk | thymidylate kinase | 1.31 | ||
nrdE | ribonucleotide reductase alpha subunit | 1.08 | ||
upp | uracil phosphoribosyl transferase | 1.17 | ||
smbA | uridylate kinase | 1.10 | ||
nrdF | ribonucleotide-reductase beta subunit | 1.19 | ||
tdk-prfA | thymidine kinase-peptide chain release factor RF-1 | 1.22 | ||
dfrA | dihydrofolate reductase | 1.11 | ||
folP | dihydropteroate synthase chain A | 0.94 | ||
nadE (SAV1913) | nicotinate phosphoribosyltransferase | 1.02 | ||
SAV0505 | nucleoside triphosphate pyrophosphohydrolase-like protein | 1.08 | ||
Transcription | ||||
rpoC | RNA polymerase beta subunit | 1.16 | ||
sigA | RNA polymerase sigma factor | 1.27 | ||
Translation | ||||
rpsE | 30S ribosomal protein S5 | 0.84 | ||
infA | translation initiation factor IF-1 | 0.86 |
Down-regulated gene . | Function . | Fold increase in susceptibilitya . | ||
---|---|---|---|---|
closthioamide . | ciprofloxacin . | novobiocin . | ||
DNA replication | ||||
dnaA | chromosomal replication initiator protein | 1.15 | ||
dnaG | DNA primase | 1.84 | 2.28 | 2.68 |
dnaC | replicative DNA helicase | 1.61 | 1.98 | 2.29 |
dnaE | DNA polymerase III alpha chain | 1.33 | ||
dnaK | molecular chaperone | 1.08 | ||
dnaX | DNA polymerase III gamma and tau subunits | 1.20 | ||
polC | DNA polymerase III alpha subunit | 0.95 | ||
holB | DNA polymerase III delta prime subunit | 1.04 | 2.81 | 2.74 |
polA | DNA polymerase I | 1.07 | ||
SAV1636 | single-strand DNA exonuclease | 0.97 | ||
dnlJ | DNA ligase (NAD+) | 1.88 | 1.74 | 1.32 |
hu | DNA-binding protein II | 1.33 | 2.50 | 2.70 |
ssb | single-strand DNA-binding protein | 1.21 | ||
gyrA | DNA gyrase subunit A | 1.31 | 2.47 | 1.53 |
gyrB | DNA gyrase subunit B | 1.43 | 1.68 | 1.55 |
parC | topoisomerase IV subunit A | 1.31 | 2.93 | 2.31 |
parE | topoisomerase IV subunit B | 1.36 | 2.98 | 3.77 |
topA | DNA topoisomerase I | 1.09 | ||
topB | DNA topoisomerase III | 1.08 | ||
Nucleic acid synthesis | ||||
thyA | thymidylate synthase | 0.90 | ||
adk | adenylate kinase | 1.04 | ||
tmk | thymidylate kinase | 1.31 | ||
nrdE | ribonucleotide reductase alpha subunit | 1.08 | ||
upp | uracil phosphoribosyl transferase | 1.17 | ||
smbA | uridylate kinase | 1.10 | ||
nrdF | ribonucleotide-reductase beta subunit | 1.19 | ||
tdk-prfA | thymidine kinase-peptide chain release factor RF-1 | 1.22 | ||
dfrA | dihydrofolate reductase | 1.11 | ||
folP | dihydropteroate synthase chain A | 0.94 | ||
nadE (SAV1913) | nicotinate phosphoribosyltransferase | 1.02 | ||
SAV0505 | nucleoside triphosphate pyrophosphohydrolase-like protein | 1.08 | ||
Transcription | ||||
rpoC | RNA polymerase beta subunit | 1.16 | ||
sigA | RNA polymerase sigma factor | 1.27 | ||
Translation | ||||
rpsE | 30S ribosomal protein S5 | 0.84 | ||
infA | translation initiation factor IF-1 | 0.86 |
aAverage of two or more experiments made in duplicate.
Susceptibility of clones having essential genes down-regulated to closthioamide and the known gyrase inhibitors ciprofloxacin and novobiocin
Down-regulated gene . | Function . | Fold increase in susceptibilitya . | ||
---|---|---|---|---|
closthioamide . | ciprofloxacin . | novobiocin . | ||
DNA replication | ||||
dnaA | chromosomal replication initiator protein | 1.15 | ||
dnaG | DNA primase | 1.84 | 2.28 | 2.68 |
dnaC | replicative DNA helicase | 1.61 | 1.98 | 2.29 |
dnaE | DNA polymerase III alpha chain | 1.33 | ||
dnaK | molecular chaperone | 1.08 | ||
dnaX | DNA polymerase III gamma and tau subunits | 1.20 | ||
polC | DNA polymerase III alpha subunit | 0.95 | ||
holB | DNA polymerase III delta prime subunit | 1.04 | 2.81 | 2.74 |
polA | DNA polymerase I | 1.07 | ||
SAV1636 | single-strand DNA exonuclease | 0.97 | ||
dnlJ | DNA ligase (NAD+) | 1.88 | 1.74 | 1.32 |
hu | DNA-binding protein II | 1.33 | 2.50 | 2.70 |
ssb | single-strand DNA-binding protein | 1.21 | ||
gyrA | DNA gyrase subunit A | 1.31 | 2.47 | 1.53 |
gyrB | DNA gyrase subunit B | 1.43 | 1.68 | 1.55 |
parC | topoisomerase IV subunit A | 1.31 | 2.93 | 2.31 |
parE | topoisomerase IV subunit B | 1.36 | 2.98 | 3.77 |
topA | DNA topoisomerase I | 1.09 | ||
topB | DNA topoisomerase III | 1.08 | ||
Nucleic acid synthesis | ||||
thyA | thymidylate synthase | 0.90 | ||
adk | adenylate kinase | 1.04 | ||
tmk | thymidylate kinase | 1.31 | ||
nrdE | ribonucleotide reductase alpha subunit | 1.08 | ||
upp | uracil phosphoribosyl transferase | 1.17 | ||
smbA | uridylate kinase | 1.10 | ||
nrdF | ribonucleotide-reductase beta subunit | 1.19 | ||
tdk-prfA | thymidine kinase-peptide chain release factor RF-1 | 1.22 | ||
dfrA | dihydrofolate reductase | 1.11 | ||
folP | dihydropteroate synthase chain A | 0.94 | ||
nadE (SAV1913) | nicotinate phosphoribosyltransferase | 1.02 | ||
SAV0505 | nucleoside triphosphate pyrophosphohydrolase-like protein | 1.08 | ||
Transcription | ||||
rpoC | RNA polymerase beta subunit | 1.16 | ||
sigA | RNA polymerase sigma factor | 1.27 | ||
Translation | ||||
rpsE | 30S ribosomal protein S5 | 0.84 | ||
infA | translation initiation factor IF-1 | 0.86 |
Down-regulated gene . | Function . | Fold increase in susceptibilitya . | ||
---|---|---|---|---|
closthioamide . | ciprofloxacin . | novobiocin . | ||
DNA replication | ||||
dnaA | chromosomal replication initiator protein | 1.15 | ||
dnaG | DNA primase | 1.84 | 2.28 | 2.68 |
dnaC | replicative DNA helicase | 1.61 | 1.98 | 2.29 |
dnaE | DNA polymerase III alpha chain | 1.33 | ||
dnaK | molecular chaperone | 1.08 | ||
dnaX | DNA polymerase III gamma and tau subunits | 1.20 | ||
polC | DNA polymerase III alpha subunit | 0.95 | ||
holB | DNA polymerase III delta prime subunit | 1.04 | 2.81 | 2.74 |
polA | DNA polymerase I | 1.07 | ||
SAV1636 | single-strand DNA exonuclease | 0.97 | ||
dnlJ | DNA ligase (NAD+) | 1.88 | 1.74 | 1.32 |
hu | DNA-binding protein II | 1.33 | 2.50 | 2.70 |
ssb | single-strand DNA-binding protein | 1.21 | ||
gyrA | DNA gyrase subunit A | 1.31 | 2.47 | 1.53 |
gyrB | DNA gyrase subunit B | 1.43 | 1.68 | 1.55 |
parC | topoisomerase IV subunit A | 1.31 | 2.93 | 2.31 |
parE | topoisomerase IV subunit B | 1.36 | 2.98 | 3.77 |
topA | DNA topoisomerase I | 1.09 | ||
topB | DNA topoisomerase III | 1.08 | ||
Nucleic acid synthesis | ||||
thyA | thymidylate synthase | 0.90 | ||
adk | adenylate kinase | 1.04 | ||
tmk | thymidylate kinase | 1.31 | ||
nrdE | ribonucleotide reductase alpha subunit | 1.08 | ||
upp | uracil phosphoribosyl transferase | 1.17 | ||
smbA | uridylate kinase | 1.10 | ||
nrdF | ribonucleotide-reductase beta subunit | 1.19 | ||
tdk-prfA | thymidine kinase-peptide chain release factor RF-1 | 1.22 | ||
dfrA | dihydrofolate reductase | 1.11 | ||
folP | dihydropteroate synthase chain A | 0.94 | ||
nadE (SAV1913) | nicotinate phosphoribosyltransferase | 1.02 | ||
SAV0505 | nucleoside triphosphate pyrophosphohydrolase-like protein | 1.08 | ||
Transcription | ||||
rpoC | RNA polymerase beta subunit | 1.16 | ||
sigA | RNA polymerase sigma factor | 1.27 | ||
Translation | ||||
rpsE | 30S ribosomal protein S5 | 0.84 | ||
infA | translation initiation factor IF-1 | 0.86 |
aAverage of two or more experiments made in duplicate.
Target identification
Effect on in vitro activity of DNA primase, DNA ligase and DNA gyrase
As a result of the susceptibility test, the gene products of the most susceptible antisense clones, DNA primase/helicase complex, DNA ligase and DNA gyrase were selected for in vitro enzyme inhibition studies. No inhibitory effect of closthioamide on DNA ligase could be detected and only a small effect was observed for the DNA primase/helicase complex, although the down-regulation of the respective genes induced the highest susceptibility to closthioamide in the previous experiment. In contrast, closthioamide inhibited DNA gyrase to a similar extent as the type II topoisomerase inhibitor ciprofloxacin (Figure 5).
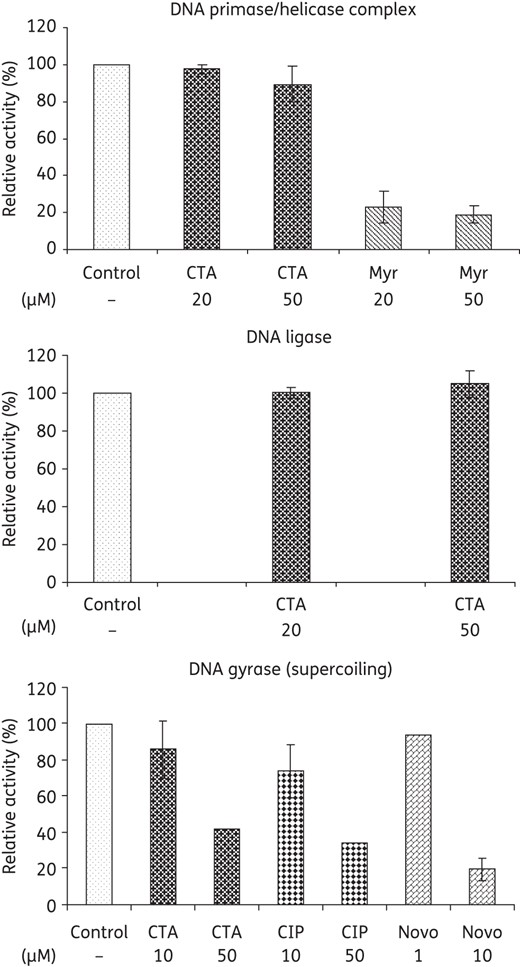
Effect of closthioamide on the in vitro activity of E. coli DNA primase/helicase complex, DNA ligase and DNA gyrase. Myricetin was used as a control inhibitor of the DNA primase/helicase complex. Ciprofloxacin and novobiocin were used as control inhibitors of gyrase supercoiling reactions. CTA, closthioamide; Myr, myricetin; CIP, ciprofloxacin; Novo, novobiocin.
Inhibition of bacterial type II topoisomerases
DNA topoisomerases are essential enzymes required for DNA strand separation during replication. The topology of DNA is modified through cleaving and rejoining of DNA double strands. All topoisomerases can relax supercoiled DNA, but only DNA gyrase can introduce negative supercoils at the expense of ATP hydrolysis. Furthermore, gyrases can relax supercoiled DNA in the absence of ATP, contrary to all other type II topoisomerases, which depend on ATP hydrolysis for activity.19,29,30 To characterize the effect of closthioamide on bacterial topoisomerases, the inhibition of DNA gyrase and topoisomerase IV from E. coli and S. aureus was studied in vitro. We analysed the capacity of the gyrase to supercoil and relax DNA and of the topoisomerase IV to relax and decatenate DNA in gel-based assays and used ciprofloxacin and novobiocin as controls (Figure 6). Closthioamide had an inhibitory effect on all these reactions, but with different potency as compared with the controls. The lowest IC50 values of closthioamide were observed for DNA gyrase and they were, with one exception, in the same order of magnitude as those of ciprofloxacin and novobiocin (Table 4). Notably, closthioamide had no effect on E. coli topoisomerase I (data not shown).
Inhibition of the in vitro activity of gyrase and topoisomerase IV by closthioamide, ciprofloxacin and novobiocin
Compound . | IC50 (μM)a . | |||||||
---|---|---|---|---|---|---|---|---|
gyrase . | topoisomerase IV . | |||||||
E. coli . | S. aureus . | E. coli . | S. aureus . | |||||
SC . | Rel . | SC . | Rel . | Dec . | Rel . | Dec . | Rel . | |
Closthioamide | 1.38 | 282 | 3.58 | 304 | 113 | 517 | 145 | 678 |
Ciprofloxacin | 0.73 | ND | 1.2 | ND | ND | 20 | ND | 0.1 |
Novobiocin | 0.15 | ND | 0.006 | ND | ND | 30 | ND | 62.42 |
Compound . | IC50 (μM)a . | |||||||
---|---|---|---|---|---|---|---|---|
gyrase . | topoisomerase IV . | |||||||
E. coli . | S. aureus . | E. coli . | S. aureus . | |||||
SC . | Rel . | SC . | Rel . | Dec . | Rel . | Dec . | Rel . | |
Closthioamide | 1.38 | 282 | 3.58 | 304 | 113 | 517 | 145 | 678 |
Ciprofloxacin | 0.73 | ND | 1.2 | ND | ND | 20 | ND | 0.1 |
Novobiocin | 0.15 | ND | 0.006 | ND | ND | 30 | ND | 62.42 |
SC, supercoiling reaction; Rel, relaxation reaction; Dec, decatenation reaction; ND, not determined.
aAverage of two or more independent determinations.
Inhibition of the in vitro activity of gyrase and topoisomerase IV by closthioamide, ciprofloxacin and novobiocin
Compound . | IC50 (μM)a . | |||||||
---|---|---|---|---|---|---|---|---|
gyrase . | topoisomerase IV . | |||||||
E. coli . | S. aureus . | E. coli . | S. aureus . | |||||
SC . | Rel . | SC . | Rel . | Dec . | Rel . | Dec . | Rel . | |
Closthioamide | 1.38 | 282 | 3.58 | 304 | 113 | 517 | 145 | 678 |
Ciprofloxacin | 0.73 | ND | 1.2 | ND | ND | 20 | ND | 0.1 |
Novobiocin | 0.15 | ND | 0.006 | ND | ND | 30 | ND | 62.42 |
Compound . | IC50 (μM)a . | |||||||
---|---|---|---|---|---|---|---|---|
gyrase . | topoisomerase IV . | |||||||
E. coli . | S. aureus . | E. coli . | S. aureus . | |||||
SC . | Rel . | SC . | Rel . | Dec . | Rel . | Dec . | Rel . | |
Closthioamide | 1.38 | 282 | 3.58 | 304 | 113 | 517 | 145 | 678 |
Ciprofloxacin | 0.73 | ND | 1.2 | ND | ND | 20 | ND | 0.1 |
Novobiocin | 0.15 | ND | 0.006 | ND | ND | 30 | ND | 62.42 |
SC, supercoiling reaction; Rel, relaxation reaction; Dec, decatenation reaction; ND, not determined.
aAverage of two or more independent determinations.
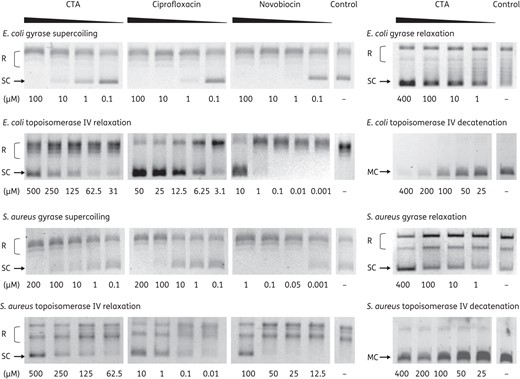
Effect of closthioamide on bacterial topoisomerase activities. E. coli and S. aureus gyrase supercoiling and relaxation and topoisomerase IV relaxation and decatenation reaction in the presence or absence (Control) of different concentrations of inhibitors. The positions of relaxed DNA, supercoiled DNA and DNA minicircles are indicated. CTA, closthioamide; R, relaxed DNA; SC, supercoiled DNA; MC, DNA minicircles.
Impact on cleavage-rejoining activity
Type II topoisomerases possess two distinct catalytic centres, the DNA cleavage-rejoining site and the ATP-binding site.31 The catalytic cycle is referred to as the ‘two-gate mechanism’. A DNA segment (G, gate segment) is bound and cleaved by the enzymatic complex whereas another segment (T, transport segment) is transported through the ‘gate’ formed by the G segment driven by hydrolysis of two ATP molecules. This event requires precise coordination between cleavage, transport and rejoining of the DNA strands and ATP hydrolysis. Topoisomerase inhibitors can interfere with one or more of these functions.
The ability of closthioamide to interfere with the cleavage-rejoining function was determined by analysing the production of linear DNA in the gyrase reaction. The assay was performed with and without ATP for both the supercoiling and relaxation activity of the E. coli DNA gyrase (Figure 7a and b). Ciprofloxacin and etoposide were included as controls since they are known to affect the cleavage–rejoining equilibrium.32 In the presence of closthioamide, linear DNA was detectable in reactions containing ATP, but only in low amounts and independent of inhibitor concentrations. The same result was obtained for etoposide, which is described to have an increased perturbing effect on human topoisomerase II-mediated DNA breaks in the presence of ATP.32 By contrast, ciprofloxacin showed this capacity also in the absence of ATP. Since no direct relation between the amount of linear DNA and the degree of inhibition was observed, the impact of closthioamide on the cleavage-rejoining activity might not be considered as its main molecular activity. To further evaluate the action of closthioamide, cooperative effects with ciprofloxacin and etoposide on the induced DNA cleavage were analysed (Figure 7c). No impact on the ciprofloxacin activity was detectable. However, a clearly enhanced effect of etoposide was noticed, which argues for non-competing binding sites.
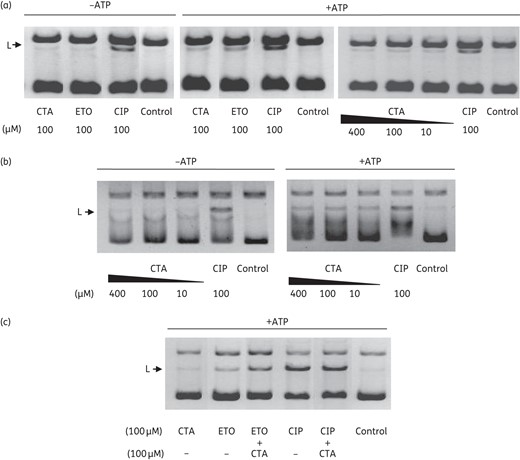
Effect of closthioamide on gyrase-induced DNA cleavage. Detection of linear DNA in the E. coli gyrase assays: (a) supercoiling and (b) relaxation reaction in the presence or absence of ATP. (c) Effect of closthioamide on ciprofloxacin- or etoposide-induced cleavage. The position of linear DNA is indicated. CTA, closthioamide; CIP, ciprofloxacin; ETO, etoposide; L, linear DNA.
Effect on ATPase function inhibition
Closthioamide inhibited the ATPase function of both gyrase and topoisomerase IV by 80% and 60%, respectively (Figure 8). In this case, a clear correlation between inhibition of ATP hydrolysis and supercoiling activity was found. However, it should be noted here that closthioamide also inhibited the DNA gyrase relaxation activity, which does not require ATP hydrolysis.
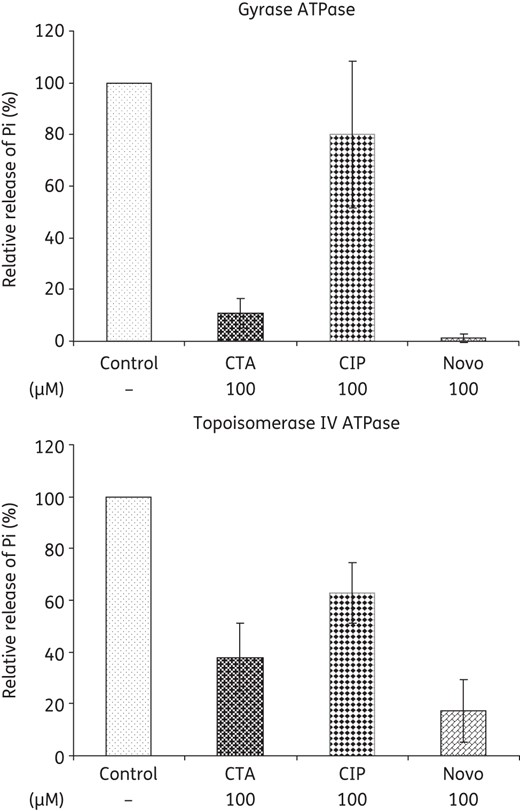
Effect of closthioamide on the ATPase function of E. coli gyrase and topoisomerase IV. Relative inhibition of inorganic phosphate release compared with the untreated control (Control). Ciprofloxacin and novobiocin were used as negative and positive inhibitor controls. CTA, closthioamide; Pi, inorganic phosphate; CIP, ciprofloxacin; Novo, novobiocin.
DNA intercalation assays
Since compounds that alter the structure of DNA through binding or intercalation can affect the function of topoisomerases, these aspects were further examined. Closthioamide did not bind to DNA in gel migration assays (data not shown). Therefore, the ability to intercalate into DNA was determined through a topoisomerase I unwinding assay as described by Sappal et al.,27 which is based on the observation that in the presence of an intercalative agent, topoisomerase I induces supercoiling of relaxed DNA. After treatment of relaxed plasmidic DNA with topoisomerase I, only samples with the intercalative agent amsacrine contained supercoiled DNA, whereas etoposide and closthioamide had no effect (Figure 9a). In order to exclude inhibitory effects on the enzyme, a control reaction using supercoiled DNA as substrate was performed. This experiment demonstrated that closthioamide and etoposide exerted no inhibition of topoisomerase I since its ability to convert supercoiled DNA into relaxed DNA remained unaffected (Figure 9b).
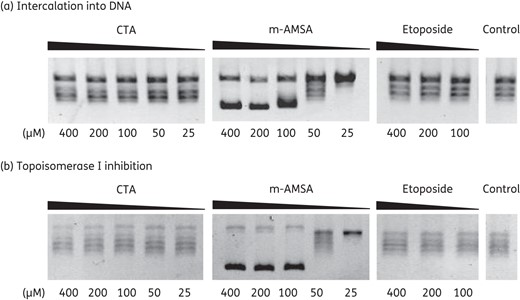
Intercalation of closthioamide into DNA. (a) DNA unwinding by calf thymus topoisomerase I in the presence or absence of an intercalating agent. The intercalative agent amsacrine was used as a positive control and etoposide as a negative control. (b) Control reaction for an inhibitory effect on the calf thymus topoisomerase I activity. CTA, closthioamide; m-AMSA, amsacrine.
Determination of resistance rate and cross-resistance
The resistance frequency of closthioamide was evaluated with two S. aureus strains and closthioamide concentrations corresponding to 4–10-fold the MIC (Table 5). Resistant mutants were obtained with low frequency rates of 10−9–10−10 on the plates containing 10× MIC of closthioamide. The resistance rates were in the same range as for ciprofloxacin and slightly higher for novobiocin. Moreover, the closthioamide-resistant colonies were small, grew very slowly, had a slimy appearance on agar and produced aggregates in liquid culture. By contrast, the ciprofloxacin- and novobiocin-resistant colonies showed no differences in phenotype, indicating that closthioamide resistance may involve restriction of cell penetration rather than protein mutation.
Resistance frequency of S. aureus exposed to closthioamide and other antibiotic drugs
. | S. aureus RN4220a . | S. simulans 22a . | S. aureus 133b . |
---|---|---|---|
Closthioamide | 1.67 × 10−10 | 5.28 × 10−9 | <3.2 × 10−10 |
Ciprofloxacin | 1.67 × 10−10 | 4.17 × 10−9 | ND |
Novobiocin | 3.83 × 10−9 | ND | ND |
Moxifloxacin | ND | ND | <3.2 × 10−10 |
Linezolid | ND | ND | <3.2 × 10−10 |
Rifampicin | ND | ND | 1.5 × 10−7 |
. | S. aureus RN4220a . | S. simulans 22a . | S. aureus 133b . |
---|---|---|---|
Closthioamide | 1.67 × 10−10 | 5.28 × 10−9 | <3.2 × 10−10 |
Ciprofloxacin | 1.67 × 10−10 | 4.17 × 10−9 | ND |
Novobiocin | 3.83 × 10−9 | ND | ND |
Moxifloxacin | ND | ND | <3.2 × 10−10 |
Linezolid | ND | ND | <3.2 × 10−10 |
Rifampicin | ND | ND | 1.5 × 10−7 |
ND, not determined.
aTen times the microbroth dilution MIC.
bFour times the soft agar MIC.
Resistance frequency of S. aureus exposed to closthioamide and other antibiotic drugs
. | S. aureus RN4220a . | S. simulans 22a . | S. aureus 133b . |
---|---|---|---|
Closthioamide | 1.67 × 10−10 | 5.28 × 10−9 | <3.2 × 10−10 |
Ciprofloxacin | 1.67 × 10−10 | 4.17 × 10−9 | ND |
Novobiocin | 3.83 × 10−9 | ND | ND |
Moxifloxacin | ND | ND | <3.2 × 10−10 |
Linezolid | ND | ND | <3.2 × 10−10 |
Rifampicin | ND | ND | 1.5 × 10−7 |
. | S. aureus RN4220a . | S. simulans 22a . | S. aureus 133b . |
---|---|---|---|
Closthioamide | 1.67 × 10−10 | 5.28 × 10−9 | <3.2 × 10−10 |
Ciprofloxacin | 1.67 × 10−10 | 4.17 × 10−9 | ND |
Novobiocin | 3.83 × 10−9 | ND | ND |
Moxifloxacin | ND | ND | <3.2 × 10−10 |
Linezolid | ND | ND | <3.2 × 10−10 |
Rifampicin | ND | ND | 1.5 × 10−7 |
ND, not determined.
aTen times the microbroth dilution MIC.
bFour times the soft agar MIC.
We further determined whether resistance to the above-mentioned topoisomerase II inhibitors triggers cross-resistance to closthioamide. Therefore, the activity of closthioamide against resistant strains with confirmed mutations was analysed. Closthioamide was equally potent against WT S. aureus RN4220, ciprofloxacin-resistant S. aureus RN4220 and novobiocin-resistant S. aureus RN4220 (Table 6).
Cross-resistance of ciprofloxacin- and novobiocin-resistant strains to closthioamide
Strain . | MIC (mg/L)a . | ||
---|---|---|---|
closthioamide . | ciprofloxacin . | novobiocin . | |
S. aureus RN4220, WT | 0.04 | 0.2 | 0.2 |
S. aureus RN4220, ciprofloxacin resistant (GyrA Ser84Leu) | 0.04 | 12.5 | 0.5 |
S. aureus RN4220, novobiocin resistant (GyrB Gly86Ser) | 0.04 | 0.2 | 6.25 |
Strain . | MIC (mg/L)a . | ||
---|---|---|---|
closthioamide . | ciprofloxacin . | novobiocin . | |
S. aureus RN4220, WT | 0.04 | 0.2 | 0.2 |
S. aureus RN4220, ciprofloxacin resistant (GyrA Ser84Leu) | 0.04 | 12.5 | 0.5 |
S. aureus RN4220, novobiocin resistant (GyrB Gly86Ser) | 0.04 | 0.2 | 6.25 |
aAverage of two or more experiments made in duplicate.
Cross-resistance of ciprofloxacin- and novobiocin-resistant strains to closthioamide
Strain . | MIC (mg/L)a . | ||
---|---|---|---|
closthioamide . | ciprofloxacin . | novobiocin . | |
S. aureus RN4220, WT | 0.04 | 0.2 | 0.2 |
S. aureus RN4220, ciprofloxacin resistant (GyrA Ser84Leu) | 0.04 | 12.5 | 0.5 |
S. aureus RN4220, novobiocin resistant (GyrB Gly86Ser) | 0.04 | 0.2 | 6.25 |
Strain . | MIC (mg/L)a . | ||
---|---|---|---|
closthioamide . | ciprofloxacin . | novobiocin . | |
S. aureus RN4220, WT | 0.04 | 0.2 | 0.2 |
S. aureus RN4220, ciprofloxacin resistant (GyrA Ser84Leu) | 0.04 | 12.5 | 0.5 |
S. aureus RN4220, novobiocin resistant (GyrB Gly86Ser) | 0.04 | 0.2 | 6.25 |
aAverage of two or more experiments made in duplicate.
Discussion
DNA gyrase is a well-validated antimicrobial target. It is affected by fluoroquinolone drugs, which are among the most important classes of broad-spectrum antibiotics, and by a class of natural products, the aminocoumarins (e.g. novobiocin), which are no longer used clinically. The attractiveness of gyrase as an antibiotic target is underlined by various development programmes in the pharmaceutical industry. A number of synthetic quinoline-based drugs are described such as GSK299423 from GlaxoSmithKline,33 NXL101 from Novexel,34 QPT-1 from Pfizer,35 a compound from AstraZeneca36 as well as the quinolone-like compounds PD0305970 and PD0326448 from Pfizer.37 Other representatives of new bacterial topoisomerase inhibitors19 are the pyrazole derivatives described by Johnson & Johnson38,39 and the cyclohexylamide derivatives developed by GlaxoSmithKline.40
Here, the antibacterial activity and the mode of action of the first member of a new class of natural products acting on bacterial gyrase are reported. Closthioamide, a polythioamide isolated from the strictly anaerobic bacterium C. cellulolyticum, is the first secondary metabolite isolated from an obligate anaerobe and also the first antibiotic derived from this group of microorganisms. Closthioamide is highly active against Gram-positive bacteria. The reduced susceptibility of Gram-negative E. coli appears to result from outer membrane barrier function and efflux pumps. The antisense susceptibility pattern resembled that of type II topoisomerase inhibitors, ciprofloxacin and novobiocin and in vitro activity assays of key enzymes pointed towards type II topoisomerases as the primary target of closthioamide. The IC50s for the gyrase supercoiling activity were in the low micromolar range and were comparable to the most frequently used gyrase inhibitor in the clinic, ciprofloxacin.
The type II topoisomerase catalytic mechanism involves double-strand DNA breakage and religation coupled with ATP hydrolysis. The catalytic cycle involves the following: (i) non-covalent binding of a DNA segment, the gate segment (G); (ii) binding of two ATP molecules that trigger the capture of another DNA segment, the transport segment (T); (iii) cleavage of both strands of the G segment and stabilization of the cleaved DNA by the formation of enzyme–DNA covalent bonds; (iv) hydrolysis of one ATP molecule that accelerates the transport of the T segment through the enzyme-stabilized break in the G segment; (v) rejoining of the cleaved segment; and (vi) hydrolysis of the second ATP, which leads to releasing of the DNA and recycling of the enzyme.19,32,41,42
Antibiotic inhibitors can affect one or several of these steps. The mechanism by which closthioamide inhibits the gyrase seems not to involve the cleavage-rejoining activity of the gyrase since only trace amounts of linear DNA, independent of the drug concentration, were detectable in the presence of ATP. Furthermore, in the drug competition assay, closthioamide did not impair the ciprofloxacin-induced DNA cleavage, but enhanced the etoposide effect. Ciprofloxacin shifts the cleavage–religation equilibrium towards cleavage independently of ATP. Etoposide is known as an inhibitor of human topoisomerase II that induces increased amounts of linear DNA in the presence of ATP through inhibiting the religation of the cleaved strand.32 Assuming that etoposide acts similarly on the bacterial gyrase, the occurrence of faint bands of linear DNA in the presence of ATP in our experiment and the enhancement of etoposide-induced cleavage suggest that closthioamide may act late in the catalytic cycle, after the strand passage triggered by the hydrolysis of one ATP molecule.
On the other hand, closthioamide inhibited the ATPase function of gyrase and topoisomerase IV by 80% and 60%, respectively, which again argues for a late cycle inhibition after the hydrolysis of the first ATP. Inhibition of the second hydrolysis step, however, appears sufficient to inhibit the overall reaction, particularly since inhibition of ATP hydrolysis correlated well with the supercoiling activity. Furthermore, closthioamide inhibited the DNA gyrase relaxation activity, which does not require ATP hydrolysis. As a consequence, closthioamide is unlikely to be a competitive inhibitor of the ATPase reaction, but may allosterically interfere with its function. Recently, a similar mechanism was described for the naphthoquinone diospyrin.29 Allosteric inhibition by reaction with cysteine residues, as reported for several type II topoisomerase inhibitors (menadione,43 etoposide quinone44 and erbstatins45), can be excluded as a mechanism. Compounds acting by this mechanism are referred to as redox-dependent poisoning agents44 and show reduced activity in the presence of reducing agents. However, DTT when applied at 1–10 mM did not affect the inhibitory activity of closthioamide (data not shown).
The present data clearly indicate that the mechanism by which closthioamide acts on DNA gyrase differs from that of the best-studied gyrase inhibitors, the quinolones and the aminocoumarins, and most probably represents a novel mechanism of action. This is also supported by the lack of cross-resistance of ciprofloxacin- and novobiocin-resistant mutants to closthioamide. Moreover, the closthioamide resistance observed after long-term training in the presence of the drug was not a result of mutations in type II topoisomerase genes, but rather of restricting access to the cell as indicated by slimy growth on plates and aggregate formation in liquid cultures.
To the best of our knowledge, closthioamide represents the third class of natural product gyrase inhibitors identified in the last 20 years. The cyclothialidine cyclic peptides produced by Streptomyces filipinensis were reported in 1993.19 After a long time gap, in 2011, kibdelomycin was isolated from a Kibdelosporangium strain crude extract46 and very recently in 2014 the cystobactamids were reported.47 Only two other natural products, simocyclinone D848 and diospyrin,29 belonging to the known classes of type II topoisomerase inhibitors of coumarins and naphthoquinones, were reported to have a novel mechanism of action. Closthioamide appears to be a valuable candidate for the pharmaceutical chemistry industry to develop a new class of resistance-breaking antibiotics. Moreover, closthioamide, being the first antibacterial agent isolated from an obligate anaerobe, strongly underlines the potential of anaerobic microorganisms as a source of novel natural product antibiotics.
Funding
This work was funded by the Ministry of Innovation, Science and Research of North Rhine-Westphalia through the BIO.NRW Cluster Biotechnologie Nordrhein-Westfalen and the European Union, ‘Ziel 2 – Programm NRW 2007-2013’ (European fund for regional development).
H.-G. S. acknowledges support from the Bonfor programme of the Medical Faculty, University of Bonn and of the German Center of Infection Research (DZIF).
Transparency declarations
C. V. is an employee of AiCuris GmbH & Co. KG with no financial conflict and availability of stock option. All other authors: none to declare.
Acknowledgements
We kindly thank Dr Terry Roemer (Merck, USA) for providing us with the antisense clones. We also thank Ute Sauer and Gisela Bukow (AiCuris, Wuppertal) for the MIC90 determination.
References
- antibiotics
- ciprofloxacin
- adenosine triphosphatases
- anaerobic bacteria
- clone cells
- clostridium
- dna
- dna gyrase
- dna ligases
- dna primase
- dna replication
- dna topoisomerase iv
- dna topoisomerases
- dna, bacterial
- gram-positive bacteria
- hydrolysis
- libraries
- novobiocin
- quinolones
- bacteria
- enzymes
- kinetics
- pharmacokinetics
- cytokinesis
- methicillin-resistant staphylococcus aureus
- macromolecular biosynthesis
- antimicrobial activity
- candidate disease gene
- natural products
- killing