-
PDF
- Split View
-
Views
-
Cite
Cite
Felipe Fernández-Cuenca, María Tomás, Francisco-Javier Caballero-Moyano, Germán Bou, Luis Martínez-Martínez, Jordi Vila, Jerónimo Pachón, José-Miguel Cisneros, Jesús Rodríguez-Baño, Álvaro Pascual, on behalf of the Spanish Group of Nosocomial Infections (GEIH) from the Spanish Society of Clinical Microbiology and Infectious Diseases (SEIMC) and the Spanish Network for Research in Infectious Diseases (REIPI), José Garnacho, Antonio Gutierrez-Pizarraya, Juan Antonio Márquez-Vácaro, María Eliecer Cano, M. Carmen Fariñas, Antonio Sánchez-Porto, Gloria Esteban Meruendano, Luis Barbeyto-Vales, Javier Casas-Ciria, Luis Vallejo, Begona Fernández-Pérez, José Carlos Villar-Chao, Belén Padilla-Ortega, Emilia Cercenado-Mansilla, José Javier García-Irure, Alfonso del Arco Jiménez, Concepción Gimeno-Cardona, Juan Carlos Valía, Núria Tormo-Palop, Vicente Abril, Josefina Rifa, Maria Jesus Martinez-Garcia, Joseph Vilaró-Pujals, Marian Navarro Aguirre, Ana Vilamala, José Antonio Jiménez-Alfaro, Carlos Reviejo-Jaca, Pilar Marín Casanova, Francisca Guerreo, Evelyn Shaw, Virginia Plasencia, Teresa Nebreda-Mayoral, María José Fernández-Calavia, Susana García de Cruz, Carmen Aldea-Mansilla, Esperanza Merino de Lucas, Alfredo Zorraquino, Sergio Reus-Bañuls, Eugenio Garduno-Eseverri, Luis López Sánchez, Ana Fleites-Gutiérrez, Azucena Rodríguez-Guardado, Alfonso Moreno, José María García-Arenzana Anguera, Serafín López-Palmero, Manuel Rodríguez-Maresca, Fernando García-Garrote, José Varela-Otero, María del Pilar Alonso, Elisa Vidal-Verdú, Fernando Rodríguez-López, Fernanda Pardo-Sánchez, E. Ferrer-Vizoso, B. Regueiro-Garcia, Mercé Gurgui, Roser Pericas, Virginia Pomar, Pedro María Olaechea-Astigarraga, Rafael Ayarza-Igartua, María Dolores Maciá-Romero, Enrique Ruiz de Gopegui-Bordes, María Isabel Sánchez-Romero, Jesús García-Mata, María José Goyanes, Cristina Morales-Mateos, José Hernández-Quero, Trinidad Escobar-Lara, Frederic Ballester-Bastardie, Simona Iftimie, Isabel Pujol-Bajador, María Isabel Galán-Navarro, María Luz Cádiz-Gurrea, Carmen Amores-Antequera, Montserrat Gómez, Purificación Cantudo, Carmina Martí-Salas, Jordi Cuquet-Peragosa, Antonio Moreno-Flores, Luis Anibarro-García, Susana Hernando-Real, Pablo A. Carrero-González, María Angeles Pallarés-González, Sergio Rodríguez-Fernández, Miquel Pujol-Rojo, Fe Tubau, Enrique Nuno-Alvarez, María Ortega-Torres, Salvador Giner-Almaraz, María Rosa Roca-Castelló, Manuela Castillo, Elena Hortelano, Fernando Chaves-Sánchez, Ana García-Reyne, Juan Pablo Horcajada-Gallego, Concha Segura, Gema Sierra-Dorado, Raquel Yano-Escudero, María Elena Dorta-Hung, Cristóbal del Rosario Q, on behalf of the Spanish Group of Nosocomial Infections (GEIH) from the Spanish Society of Clinical Microbiology and Infectious Diseases (SEIMC) and the Spanish Network for Research in Infectious Diseases (REIPI), Reduced susceptibility to biocides in Acinetobacter baumannii: association with resistance to antimicrobials, epidemiological behaviour, biological cost and effect on the expression of genes encoding porins and efflux pumps, Journal of Antimicrobial Chemotherapy, Volume 70, Issue 12, December 2015, Pages 3222–3229, https://doi.org/10.1093/jac/dkv262
- Share Icon Share
Abstract
The objective of this study was to analyse whether there is an association between reduced susceptibility to biocides in Acinetobacter baumannii and (i) antimicrobial resistance (co-resistance), (ii) prevalent (epidemic) clones, (iii) changes in the fitness or (iv) expression of genes related to efflux pumps and porins.
Susceptibility to biocides and antimicrobials was determined in 49 clonally unrelated isolates of A. baumannii. Biological cost, in terms of mean generation time, was determined by spectrophotometry. Quantitative real-time RT–PCR was used to determine the relative expression of genes encoding several efflux pumps and porins.
Reduced susceptibility to chlorhexidine digluconate, benzalkonium chloride and Irgasan® was associated with resistance to aminoglycosides, tetracycline and ciprofloxacin (P < 0.05). The MICs of carbapenems, aminoglycosides, doxycycline and ciprofloxacin for isolate Ab70 (epidemic clone) exposed to these biocides increased by ≥2 dilutions. Reduced susceptibility to Orsan® was more frequent among prevalent clones than non-prevalent clones (P < 0.05). Mean generation times for Ab70 before and after exposure to benzalkonium chloride were 57.8 and 78.1 min, respectively (P = 0.02). Relative expression of abeS and adeB was increased in Ab46 and Ab70 after exposure to chlorhexidine digluconate, but was decreased for ompA and carO after exposure to Irgasan®.
Reduced susceptibility to biocides is associated with co-resistance to carbapenems, aminoglycosides, tetracycline and ciprofloxacin. Reduced susceptibility to Orsan® may be a marker of prevalent clones. Acquisition of reduced susceptibility to benzalkonium chloride has a biological cost. Exposure to biocides affects the relative expression of genes related to some efflux pump genes (increased expression) or porins (reduced expression).
Introduction
Acinetobacter baumannii is a nosocomial pathogen that can cause infections in humans, particularly patients with severe underlying diseases. The clinical and epidemiological relevance of this opportunistic pathogen resides in its ability to acquire MDR and to persist in the nosocomial environment for long periods of time under adverse conditions.1–3 These two factors, MDR and persistence, have been clearly associated with the epidemic behaviour of some successful clones of A. baumannii, although the role of other potential factors, such as acquisition of reduced susceptibility to biocides (RSB), remains unclear or has not been well studied.
Biocides are commonly used in healthcare institutions as antiseptics and disinfectants. Some researchers have postulated that the presence of subinhibitory concentrations or small traces of biocide could select for MDR epidemic clones of A. baumannii with RSB.4–9 Selection of MDR isolates of A. baumannii with RSB could be explained directly if MDR and RSB shared common mechanisms of resistance. Potential candidates for reducing the activity of biocides and antimicrobials include outer membrane permeability and efflux pumps, as has previously been described for other microorganisms.6,10–14 Reducing the intracellular accumulation of antimicrobial agents and biocides can be achieved by reducing outer membrane permeability, mediated by the loss of or reduced porin expression and by the overexpression of efflux pumps, since biocides and antimicrobials are good substrates of most pumps.12,15–19 The acquisition of RSB would confer a competitive advantage (favourable biological cost) for MDR A. baumannii isolates exposed to subinhibitory concentrations of biocides, although this hypothesis has not yet been proven.
The aim of the present study was to determine susceptibility to some commonly used biocides of a collection of clinical isolates representing the most prevalent clones of A. baumannii isolated in Spain and to examine whether isolates with the highest MICs (more tolerant) to biocides, compared with those with the lowest MICs (less tolerant) to biocides, were (i) more resistant to antimicrobials or (ii) more prevalent (epidemic behaviour), and to evaluate the effect of the acquisition of RSB on (iii) fitness (biological cost) and (iv) gene expression related to efflux pumps and porins.
Materials and methods
Bacterial isolates
Forty-nine A. baumannii clinical isolates were selected from a collection of 221 isolates obtained during a multicentre Spanish study performed in 2010 (REIPI-GEIH Ab-2010 project).20 These isolates were clonally unrelated (49 out of 79 different PFGE patterns) and represented the most important clones and all the antimicrobial susceptibility patterns observed in the nationwide study.
Biocides and antimicrobial agents
The biocides were tested either diluted or undiluted (Table 1). When necessary, the biocide was diluted in sterile distilled water, except for Irgasan®, which was diluted with 70% methanol (Panreac Química SAU, Barcelona, Spain).
Generic name . | Manufacturer . | Chemical composition . | Working concentration . |
---|---|---|---|
Sterillium® | Bode Chemie | 45% (w/w) isopropanol, 30% (w/w) n-propanol, 0.2% (w/w) mecetronium ethylsulphate | undiluted (8.5 × 105 mg/L) |
Orsan® | Tyco Healthcare Spain SL | 10% PVP-iodine (alcoholic solution), 1% free iodine | undiluted (1 × 104 mg iodine/L) |
Domestic bleach | Chari® | sodium hypochlorite (40 g/L) | undiluted (4 × 104 mg/L) |
Chlorhexidine digluconate | Sigma-Aldrich | 20% (w/v) chlorhexidine digluconate | 4% (4 × 104 mg/L) |
Benzalkonium chloride | Sigma-Aldrich | 50% (w/v) benzalkonium chloride | 0.1% (1 × 103 mg/L) |
Ethanol | Alcosani® | 70% (v/v) ethanol | 70% (5.7 × 102 mg/L) |
Irgasan® | Sigma-Aldrich | 2,4,4′-trichloro-2′-hydroxy-diphenyl-ether (triclosan) | 0.003% (30 mg/L) |
Generic name . | Manufacturer . | Chemical composition . | Working concentration . |
---|---|---|---|
Sterillium® | Bode Chemie | 45% (w/w) isopropanol, 30% (w/w) n-propanol, 0.2% (w/w) mecetronium ethylsulphate | undiluted (8.5 × 105 mg/L) |
Orsan® | Tyco Healthcare Spain SL | 10% PVP-iodine (alcoholic solution), 1% free iodine | undiluted (1 × 104 mg iodine/L) |
Domestic bleach | Chari® | sodium hypochlorite (40 g/L) | undiluted (4 × 104 mg/L) |
Chlorhexidine digluconate | Sigma-Aldrich | 20% (w/v) chlorhexidine digluconate | 4% (4 × 104 mg/L) |
Benzalkonium chloride | Sigma-Aldrich | 50% (w/v) benzalkonium chloride | 0.1% (1 × 103 mg/L) |
Ethanol | Alcosani® | 70% (v/v) ethanol | 70% (5.7 × 102 mg/L) |
Irgasan® | Sigma-Aldrich | 2,4,4′-trichloro-2′-hydroxy-diphenyl-ether (triclosan) | 0.003% (30 mg/L) |
Generic name . | Manufacturer . | Chemical composition . | Working concentration . |
---|---|---|---|
Sterillium® | Bode Chemie | 45% (w/w) isopropanol, 30% (w/w) n-propanol, 0.2% (w/w) mecetronium ethylsulphate | undiluted (8.5 × 105 mg/L) |
Orsan® | Tyco Healthcare Spain SL | 10% PVP-iodine (alcoholic solution), 1% free iodine | undiluted (1 × 104 mg iodine/L) |
Domestic bleach | Chari® | sodium hypochlorite (40 g/L) | undiluted (4 × 104 mg/L) |
Chlorhexidine digluconate | Sigma-Aldrich | 20% (w/v) chlorhexidine digluconate | 4% (4 × 104 mg/L) |
Benzalkonium chloride | Sigma-Aldrich | 50% (w/v) benzalkonium chloride | 0.1% (1 × 103 mg/L) |
Ethanol | Alcosani® | 70% (v/v) ethanol | 70% (5.7 × 102 mg/L) |
Irgasan® | Sigma-Aldrich | 2,4,4′-trichloro-2′-hydroxy-diphenyl-ether (triclosan) | 0.003% (30 mg/L) |
Generic name . | Manufacturer . | Chemical composition . | Working concentration . |
---|---|---|---|
Sterillium® | Bode Chemie | 45% (w/w) isopropanol, 30% (w/w) n-propanol, 0.2% (w/w) mecetronium ethylsulphate | undiluted (8.5 × 105 mg/L) |
Orsan® | Tyco Healthcare Spain SL | 10% PVP-iodine (alcoholic solution), 1% free iodine | undiluted (1 × 104 mg iodine/L) |
Domestic bleach | Chari® | sodium hypochlorite (40 g/L) | undiluted (4 × 104 mg/L) |
Chlorhexidine digluconate | Sigma-Aldrich | 20% (w/v) chlorhexidine digluconate | 4% (4 × 104 mg/L) |
Benzalkonium chloride | Sigma-Aldrich | 50% (w/v) benzalkonium chloride | 0.1% (1 × 103 mg/L) |
Ethanol | Alcosani® | 70% (v/v) ethanol | 70% (5.7 × 102 mg/L) |
Irgasan® | Sigma-Aldrich | 2,4,4′-trichloro-2′-hydroxy-diphenyl-ether (triclosan) | 0.003% (30 mg/L) |
The antimicrobial agents assayed were: ciprofloxacin, gentamicin, tobramycin, amikacin, netilmicin, ceftazidime, imipenem, colistin, minocycline, tetracycline and rifampicin, from Sigma, Madrid, Spain; sulbactam and doxycycline, from Pfizer, Madrid, Spain; meropenem, from AstraZeneca, Madrid, Spain; and tigecycline, from Wyeth, Madrid, Spain.20
Susceptibility testing
A broth microdilution assay was performed using serial 2-fold dilutions of each biocide or antimicrobial agent in Mueller–Hinton broth (Difco, Madrid, Spain). For biocides the ranges of concentrations evaluated were 208–425 000 mg/L (Sterillium®), 9.8–20 000 mg/L (domestic bleach), 2.4–5000 mg/L (Orsan®), 0.2–500 mg/L (benzalkonium chloride), 0.2–312.5 mg/L (chlorhexidine digluconate), 0.1–285 mg/L (ethanol) and 0.01–15 mg/L (Irgasan®). Breakpoints were not used for biocides, since none have been established. The range of antimicrobial concentrations used were 2–256 mg/L (amikacin), 1–128 mg/L (ceftazidime), 0.5–64 mg/L (sulbactam, imipenem, meropenem, ciprofloxacin, tetracycline, doxycycline, minocycline, gentamicin, tobramycin, netilmicin, colistin and rifampicin) and 0.25–32 mg/L (tigecycline). The breakpoints used for the antimicrobials tested were in accordance with CLSI performance standards for antimicrobial susceptibility testing.21
The MICs of biocides and antimicrobial agents were determined. The MBCs for biocides were determined by subculturing all wells with no visible growth in MacConkey agar (Oxoid).
Association between RSB and antimicrobial resistance
Two groups of isolates were defined according to their MICs of biocides (Table 2): those with the highest MICs (isolates with RSB) and those with the lowest MICs (isolates with no RSB). With respect to the antimicrobial agents tested, two further groups of isolates were considered: those for which the MICs were less than or equal to the susceptibility breakpoint, as defined by CLSI criteria (isolates susceptible to antimicrobial agents) and those for which the MICs were greater than the susceptibility breakpoint (resistant isolates, including fully resistant and intermediate resistant isolates).
Biocide . | MICs (% isolates) . | RSB . | MBCs (% isolates) . |
---|---|---|---|
Sterillium® | 10 626 (0) | not applicable | 10 626 (1) |
5313 (20) | yes | 5313 (24) | |
2656 (77) | no | 2656 (73) | |
1328 (3) | no | 1328 (2) | |
Orsan® | 625 (0) | not applicable | 625 (3) |
312.5 (43) | yes | 312.5 (43) | |
156.3 (57) | no | 156.3 (54) | |
Domestic bleach | 2500 (0) | not applicable | 2500 (1) |
1250 (7) | yes | 1250 (7) | |
625 (93) | no | 625 (92) | |
Chlorhexidine digluconate | 156.4 (0) | not applicable | 156.4 (2) |
78.2 (0) | not applicable | 78.2 (3) | |
39.1 (1) | yes | 39.1 (7) | |
19.5 (12) | yes | 19.5 (21) | |
9.8 (58) | yes | 9.8 (44) | |
4.9 (16) | no | 4.9 (12) | |
2.4 (13) | no | 2.4 (11) | |
Benzalkonium chloride | 124.8 (0) | not applicable | 124.8 (2) |
62.4 (0) | not applicable | 62.4 (0) | |
31.2 (0) | not applicable | 31.2 (2) | |
15.6 (1) | yes | 15.6 (3) | |
7.8 (28) | yes | 7.8 (36) | |
3.9 (60) | no | 3.9 (48) | |
2 (10) | no | 2 (8) | |
1 (1) | no | 1 (1) | |
Ethanol | 71.2 (0) | not applicable | 71.2 (2) |
35.6 (0) | not applicable | 35.6 (3) | |
17.8 (0) | not applicable | 17.8 (4) | |
8.9 (50) | yes | 8.9 (46) | |
4.5 (40) | yes | 4.5 (37) | |
2.2 (9) | no | 2.2 (7) | |
1.1 (1) | no | 1.1 (1) | |
Irgasan® | 7.5 (1) | yes | 7.5 (28) |
3.8 (15) | yes | 3.8 (59) | |
1.9 (12) | yes | 1.9 (2) | |
0.9 (21) | yes | 0.9 (8) | |
0.5 (19) | no | 0.5 (3) | |
0.3 (20) | no | 0.3 (0) | |
0.1 (11) | no | 0.1 (0) | |
0.05 (1) | no | 0.05 (0) |
Biocide . | MICs (% isolates) . | RSB . | MBCs (% isolates) . |
---|---|---|---|
Sterillium® | 10 626 (0) | not applicable | 10 626 (1) |
5313 (20) | yes | 5313 (24) | |
2656 (77) | no | 2656 (73) | |
1328 (3) | no | 1328 (2) | |
Orsan® | 625 (0) | not applicable | 625 (3) |
312.5 (43) | yes | 312.5 (43) | |
156.3 (57) | no | 156.3 (54) | |
Domestic bleach | 2500 (0) | not applicable | 2500 (1) |
1250 (7) | yes | 1250 (7) | |
625 (93) | no | 625 (92) | |
Chlorhexidine digluconate | 156.4 (0) | not applicable | 156.4 (2) |
78.2 (0) | not applicable | 78.2 (3) | |
39.1 (1) | yes | 39.1 (7) | |
19.5 (12) | yes | 19.5 (21) | |
9.8 (58) | yes | 9.8 (44) | |
4.9 (16) | no | 4.9 (12) | |
2.4 (13) | no | 2.4 (11) | |
Benzalkonium chloride | 124.8 (0) | not applicable | 124.8 (2) |
62.4 (0) | not applicable | 62.4 (0) | |
31.2 (0) | not applicable | 31.2 (2) | |
15.6 (1) | yes | 15.6 (3) | |
7.8 (28) | yes | 7.8 (36) | |
3.9 (60) | no | 3.9 (48) | |
2 (10) | no | 2 (8) | |
1 (1) | no | 1 (1) | |
Ethanol | 71.2 (0) | not applicable | 71.2 (2) |
35.6 (0) | not applicable | 35.6 (3) | |
17.8 (0) | not applicable | 17.8 (4) | |
8.9 (50) | yes | 8.9 (46) | |
4.5 (40) | yes | 4.5 (37) | |
2.2 (9) | no | 2.2 (7) | |
1.1 (1) | no | 1.1 (1) | |
Irgasan® | 7.5 (1) | yes | 7.5 (28) |
3.8 (15) | yes | 3.8 (59) | |
1.9 (12) | yes | 1.9 (2) | |
0.9 (21) | yes | 0.9 (8) | |
0.5 (19) | no | 0.5 (3) | |
0.3 (20) | no | 0.3 (0) | |
0.1 (11) | no | 0.1 (0) | |
0.05 (1) | no | 0.05 (0) |
Biocide . | MICs (% isolates) . | RSB . | MBCs (% isolates) . |
---|---|---|---|
Sterillium® | 10 626 (0) | not applicable | 10 626 (1) |
5313 (20) | yes | 5313 (24) | |
2656 (77) | no | 2656 (73) | |
1328 (3) | no | 1328 (2) | |
Orsan® | 625 (0) | not applicable | 625 (3) |
312.5 (43) | yes | 312.5 (43) | |
156.3 (57) | no | 156.3 (54) | |
Domestic bleach | 2500 (0) | not applicable | 2500 (1) |
1250 (7) | yes | 1250 (7) | |
625 (93) | no | 625 (92) | |
Chlorhexidine digluconate | 156.4 (0) | not applicable | 156.4 (2) |
78.2 (0) | not applicable | 78.2 (3) | |
39.1 (1) | yes | 39.1 (7) | |
19.5 (12) | yes | 19.5 (21) | |
9.8 (58) | yes | 9.8 (44) | |
4.9 (16) | no | 4.9 (12) | |
2.4 (13) | no | 2.4 (11) | |
Benzalkonium chloride | 124.8 (0) | not applicable | 124.8 (2) |
62.4 (0) | not applicable | 62.4 (0) | |
31.2 (0) | not applicable | 31.2 (2) | |
15.6 (1) | yes | 15.6 (3) | |
7.8 (28) | yes | 7.8 (36) | |
3.9 (60) | no | 3.9 (48) | |
2 (10) | no | 2 (8) | |
1 (1) | no | 1 (1) | |
Ethanol | 71.2 (0) | not applicable | 71.2 (2) |
35.6 (0) | not applicable | 35.6 (3) | |
17.8 (0) | not applicable | 17.8 (4) | |
8.9 (50) | yes | 8.9 (46) | |
4.5 (40) | yes | 4.5 (37) | |
2.2 (9) | no | 2.2 (7) | |
1.1 (1) | no | 1.1 (1) | |
Irgasan® | 7.5 (1) | yes | 7.5 (28) |
3.8 (15) | yes | 3.8 (59) | |
1.9 (12) | yes | 1.9 (2) | |
0.9 (21) | yes | 0.9 (8) | |
0.5 (19) | no | 0.5 (3) | |
0.3 (20) | no | 0.3 (0) | |
0.1 (11) | no | 0.1 (0) | |
0.05 (1) | no | 0.05 (0) |
Biocide . | MICs (% isolates) . | RSB . | MBCs (% isolates) . |
---|---|---|---|
Sterillium® | 10 626 (0) | not applicable | 10 626 (1) |
5313 (20) | yes | 5313 (24) | |
2656 (77) | no | 2656 (73) | |
1328 (3) | no | 1328 (2) | |
Orsan® | 625 (0) | not applicable | 625 (3) |
312.5 (43) | yes | 312.5 (43) | |
156.3 (57) | no | 156.3 (54) | |
Domestic bleach | 2500 (0) | not applicable | 2500 (1) |
1250 (7) | yes | 1250 (7) | |
625 (93) | no | 625 (92) | |
Chlorhexidine digluconate | 156.4 (0) | not applicable | 156.4 (2) |
78.2 (0) | not applicable | 78.2 (3) | |
39.1 (1) | yes | 39.1 (7) | |
19.5 (12) | yes | 19.5 (21) | |
9.8 (58) | yes | 9.8 (44) | |
4.9 (16) | no | 4.9 (12) | |
2.4 (13) | no | 2.4 (11) | |
Benzalkonium chloride | 124.8 (0) | not applicable | 124.8 (2) |
62.4 (0) | not applicable | 62.4 (0) | |
31.2 (0) | not applicable | 31.2 (2) | |
15.6 (1) | yes | 15.6 (3) | |
7.8 (28) | yes | 7.8 (36) | |
3.9 (60) | no | 3.9 (48) | |
2 (10) | no | 2 (8) | |
1 (1) | no | 1 (1) | |
Ethanol | 71.2 (0) | not applicable | 71.2 (2) |
35.6 (0) | not applicable | 35.6 (3) | |
17.8 (0) | not applicable | 17.8 (4) | |
8.9 (50) | yes | 8.9 (46) | |
4.5 (40) | yes | 4.5 (37) | |
2.2 (9) | no | 2.2 (7) | |
1.1 (1) | no | 1.1 (1) | |
Irgasan® | 7.5 (1) | yes | 7.5 (28) |
3.8 (15) | yes | 3.8 (59) | |
1.9 (12) | yes | 1.9 (2) | |
0.9 (21) | yes | 0.9 (8) | |
0.5 (19) | no | 0.5 (3) | |
0.3 (20) | no | 0.3 (0) | |
0.1 (11) | no | 0.1 (0) | |
0.05 (1) | no | 0.05 (0) |
To determine whether or not the potential association between RSB and resistance to antimicrobials was clonally dependent, two clonally related clinical isolates of A. baumannii (Ab46 and Ab70) were selected from the REIPI-GEIH Ab-2010 project. They differed in their susceptibility to carbapenems, ciprofloxacin and aminoglycosides (Table 3). These two isolates were subcultured weekly in Mueller–Hinton broth for 30 days in the presence of subinhibitory concentrations (0.25 × MIC) of Irgasan®, benzalkonium chloride or chlorhexidine digluconate. The MICs of biocides and antimicrobials before exposure to the biocides were determined and compared with those determined after exposure to them.
MICs (mg/L) of 15 antimicrobials for Ab46 and Ab70 isolates exposed to subinhibitory concentrations (0.25× MIC) of chlorhexidine digluconate (CHX), benzalkonium chloride (BZK) and Irgasan® (IRG)
Isolate . | Distribution of MICs . | ||||||||||||||
---|---|---|---|---|---|---|---|---|---|---|---|---|---|---|---|
SUL . | CAZ . | IPM . | MEM . | CIP . | GEN . | TOB . | AMK . | MIN . | NET . | TET . | DOX . | CST . | RIF . | TGC . | |
Ab46 | 64 | >128 | 64 | 32 | 32 | 2 | 32 | 32 | <0.5 | >64 | >64 | 16 | <0.5 | 1 | 0.5 |
Ab46 exposed to CHX | 32 | >128 | 64 | 32 | 32 | 2 | 32 | 16 | <0.5 | 64 | >64 | 16 | <0.5 | 1 | 0.5 |
Ab46 exposed to BZK | 64 | >128 | 64 | 32 | >64 | 4 | 32 | 16 | <0.5 | >64 | >64 | 8 | 1 | 1 | 1 |
Ab46 exposed to IRG | 64 | >128 | 32 | 32 | >64 | 2 | 32 | 64 | 2 | >64 | >64 | 64 | <0.5 | 2 | <0.25 |
Ab70 | 4 | >128 | 8 | 2 | 4 | 2 | <0.5 | <2 | <0.5 | <0.5 | >64 | 2 | <0.5 | 2 | <0.25 |
Ab70 exposed to CHX | 32 | >128 | 64 | 32 | 64 | <0.5 | 8 | 4 | 2 | 64 | >64 | 32 | <0.5 | 1 | <0.25 |
Ab70 exposed to BZK | 32 | >128 | 64 | 32 | 64 | 8 | 64 | 32 | <0.5 | >64 | >64 | 16 | <0.5 | 1 | 0.5 |
Ab70 exposed to IRG | 4 | >128 | 8 | 4 | >64 | 32 | <0.5 | 16 | 4 | 64 | >64 | 32 | <0.5 | 2 | <0.25 |
Isolate . | Distribution of MICs . | ||||||||||||||
---|---|---|---|---|---|---|---|---|---|---|---|---|---|---|---|
SUL . | CAZ . | IPM . | MEM . | CIP . | GEN . | TOB . | AMK . | MIN . | NET . | TET . | DOX . | CST . | RIF . | TGC . | |
Ab46 | 64 | >128 | 64 | 32 | 32 | 2 | 32 | 32 | <0.5 | >64 | >64 | 16 | <0.5 | 1 | 0.5 |
Ab46 exposed to CHX | 32 | >128 | 64 | 32 | 32 | 2 | 32 | 16 | <0.5 | 64 | >64 | 16 | <0.5 | 1 | 0.5 |
Ab46 exposed to BZK | 64 | >128 | 64 | 32 | >64 | 4 | 32 | 16 | <0.5 | >64 | >64 | 8 | 1 | 1 | 1 |
Ab46 exposed to IRG | 64 | >128 | 32 | 32 | >64 | 2 | 32 | 64 | 2 | >64 | >64 | 64 | <0.5 | 2 | <0.25 |
Ab70 | 4 | >128 | 8 | 2 | 4 | 2 | <0.5 | <2 | <0.5 | <0.5 | >64 | 2 | <0.5 | 2 | <0.25 |
Ab70 exposed to CHX | 32 | >128 | 64 | 32 | 64 | <0.5 | 8 | 4 | 2 | 64 | >64 | 32 | <0.5 | 1 | <0.25 |
Ab70 exposed to BZK | 32 | >128 | 64 | 32 | 64 | 8 | 64 | 32 | <0.5 | >64 | >64 | 16 | <0.5 | 1 | 0.5 |
Ab70 exposed to IRG | 4 | >128 | 8 | 4 | >64 | 32 | <0.5 | 16 | 4 | 64 | >64 | 32 | <0.5 | 2 | <0.25 |
SUL, sulbactam; CAZ, ceftazidime; IPM, imipenem; MEM, meropenem; CIP, ciprofloxacin; GEN, gentamicin; TOB, tobramycin; AMK, amikacin; MIN, minocycline; NET, netilmicin; TET, tetracycline; DOX, doxycycline; CST, colistin; RIF, rifampicin; TGC, tigecycline.
Greater than or equal to 2-fold changes in MICs between exposed and non-exposed isolates appear in bold.
MICs (mg/L) of 15 antimicrobials for Ab46 and Ab70 isolates exposed to subinhibitory concentrations (0.25× MIC) of chlorhexidine digluconate (CHX), benzalkonium chloride (BZK) and Irgasan® (IRG)
Isolate . | Distribution of MICs . | ||||||||||||||
---|---|---|---|---|---|---|---|---|---|---|---|---|---|---|---|
SUL . | CAZ . | IPM . | MEM . | CIP . | GEN . | TOB . | AMK . | MIN . | NET . | TET . | DOX . | CST . | RIF . | TGC . | |
Ab46 | 64 | >128 | 64 | 32 | 32 | 2 | 32 | 32 | <0.5 | >64 | >64 | 16 | <0.5 | 1 | 0.5 |
Ab46 exposed to CHX | 32 | >128 | 64 | 32 | 32 | 2 | 32 | 16 | <0.5 | 64 | >64 | 16 | <0.5 | 1 | 0.5 |
Ab46 exposed to BZK | 64 | >128 | 64 | 32 | >64 | 4 | 32 | 16 | <0.5 | >64 | >64 | 8 | 1 | 1 | 1 |
Ab46 exposed to IRG | 64 | >128 | 32 | 32 | >64 | 2 | 32 | 64 | 2 | >64 | >64 | 64 | <0.5 | 2 | <0.25 |
Ab70 | 4 | >128 | 8 | 2 | 4 | 2 | <0.5 | <2 | <0.5 | <0.5 | >64 | 2 | <0.5 | 2 | <0.25 |
Ab70 exposed to CHX | 32 | >128 | 64 | 32 | 64 | <0.5 | 8 | 4 | 2 | 64 | >64 | 32 | <0.5 | 1 | <0.25 |
Ab70 exposed to BZK | 32 | >128 | 64 | 32 | 64 | 8 | 64 | 32 | <0.5 | >64 | >64 | 16 | <0.5 | 1 | 0.5 |
Ab70 exposed to IRG | 4 | >128 | 8 | 4 | >64 | 32 | <0.5 | 16 | 4 | 64 | >64 | 32 | <0.5 | 2 | <0.25 |
Isolate . | Distribution of MICs . | ||||||||||||||
---|---|---|---|---|---|---|---|---|---|---|---|---|---|---|---|
SUL . | CAZ . | IPM . | MEM . | CIP . | GEN . | TOB . | AMK . | MIN . | NET . | TET . | DOX . | CST . | RIF . | TGC . | |
Ab46 | 64 | >128 | 64 | 32 | 32 | 2 | 32 | 32 | <0.5 | >64 | >64 | 16 | <0.5 | 1 | 0.5 |
Ab46 exposed to CHX | 32 | >128 | 64 | 32 | 32 | 2 | 32 | 16 | <0.5 | 64 | >64 | 16 | <0.5 | 1 | 0.5 |
Ab46 exposed to BZK | 64 | >128 | 64 | 32 | >64 | 4 | 32 | 16 | <0.5 | >64 | >64 | 8 | 1 | 1 | 1 |
Ab46 exposed to IRG | 64 | >128 | 32 | 32 | >64 | 2 | 32 | 64 | 2 | >64 | >64 | 64 | <0.5 | 2 | <0.25 |
Ab70 | 4 | >128 | 8 | 2 | 4 | 2 | <0.5 | <2 | <0.5 | <0.5 | >64 | 2 | <0.5 | 2 | <0.25 |
Ab70 exposed to CHX | 32 | >128 | 64 | 32 | 64 | <0.5 | 8 | 4 | 2 | 64 | >64 | 32 | <0.5 | 1 | <0.25 |
Ab70 exposed to BZK | 32 | >128 | 64 | 32 | 64 | 8 | 64 | 32 | <0.5 | >64 | >64 | 16 | <0.5 | 1 | 0.5 |
Ab70 exposed to IRG | 4 | >128 | 8 | 4 | >64 | 32 | <0.5 | 16 | 4 | 64 | >64 | 32 | <0.5 | 2 | <0.25 |
SUL, sulbactam; CAZ, ceftazidime; IPM, imipenem; MEM, meropenem; CIP, ciprofloxacin; GEN, gentamicin; TOB, tobramycin; AMK, amikacin; MIN, minocycline; NET, netilmicin; TET, tetracycline; DOX, doxycycline; CST, colistin; RIF, rifampicin; TGC, tigecycline.
Greater than or equal to 2-fold changes in MICs between exposed and non-exposed isolates appear in bold.
Association between RSB and epidemiological behaviour
To evaluate this association, a further 9 clones were added to the original 49. Of these 58 clones (selected from 34 of 37 participating hospitals), 33 were prevalent clones and 25 were non-prevalent. Each hospital used the following definition for a prevalent clone: (i) it was the only clone and was represented by three or more isolates; or (ii) there were two or more different clones (coexistence of prevalent with non-prevalent clones) and one of them was represented by >75% of all isolates at that hospital.
Biological cost of RSB
The biological cost associated with the acquisition of RSB was determined using growth rates, as previously described.22 OD at 600 nm was measured every 5 min for 24 h, using an Infinite M200 (Tecan Austria GmbH) spectrometer reader. Maximum growth rates were determined in the presence of biocides at 0.25 × MIC and in the absence of biocides (control). Doubling time or mean generation time was calculated as log10 2 divided by the maximal growth rate. These experiments were performed in triplicate (mean growth rate ± SD).
Effect of RSB on gene expression
The relative expression of genes encoding efflux pumps (AdeB, AdeJ, AbeM, AbeS and AmvA) and porins (OmpA, CarO, OprD-like and Omp 33–36 kDa) was determined by quantitative real-time RT–PCR using a LightCycler® 2.0 instrument (Roche, Barcelona, Spain). Total RNA was treated with DNAase (TURBO DNA-free™; Ambion, Madrid, Spain) and retrotranscribed into cDNA (Transcriptor First Strand cDNA Synthesis Kit; Roche), which was amplified using specific oligonucleotides (Table 4) and LightCycler® FastStart DNA MasterPLUS SYBR Green I (Roche). RNA samples amplified without reverse transcription were used to control for possible DNA contamination. Relative gene expression was determined using the 2−ΔΔCt method.23 The housekeeping gene rpoB was used as a reference endogenous gene for normalization of gene expression and isolates Ab46 and Ab70 not exposed to biocides were used as calibrators or reference samples (expression set to 1). All experiments were performed in triplicate.
Primer . | Nucleotide sequence (5′-3′) . | Target . | Reference . |
---|---|---|---|
Omp33-36-RT-F | TCCAACTCCTTACTTGCCAGTTTA | omp 33-36 | 33 |
Omp33-36-RT-R | GCACCAGCTTCTAATGCATAACG | ||
CarO-RT-F | GGCGGATGAAGCTGTTGTTC | carO | 33 |
CarO-RT-R | CACGAGCGCCTACTGGAATT | ||
OprD-RT-F | CGCCCGGCCATGTATCA | oprD | 33 |
OprD-FT-R | GCGACACCGCGTTTCCATAT | ||
OmpA-RT-F | AGCTCTTGCTGGCTTAAACG | ompA | 34 |
OmpA-RT-R | GAGCAACTGGAGTTGGTTCA | ||
AdeB-RT-F | GGATTATGGCGACAGAAGGA | adeB | 35 |
AdeB-RT-R | AATACTGCCGCCAATACCAG | ||
AdeJ-RT-F | GGTCATTAATATCTTTGGC | adeJ | 16 |
AdeJ-RT-R | GGTACGAATACCGCTGTCA | ||
AbeM-RT-F | GCTATTCCGAAGCATTAGGC | abeM | 34 |
AbeM-RT-R | CCAAAGCAGGTATTGGTCCT | ||
AbeS-RT-F | CGTGGCGTGGGATATAGACT | abeS | 34 |
AbeS-RT-R | AGGAAAATGCCACAAAATGG | ||
AmvA-RT1 | TGTATTCCTCATTAATATCCC | amvA | 17 |
AmvA-RT2 | TTACTTTCTTCGGAAAACTAAACC |
Primer . | Nucleotide sequence (5′-3′) . | Target . | Reference . |
---|---|---|---|
Omp33-36-RT-F | TCCAACTCCTTACTTGCCAGTTTA | omp 33-36 | 33 |
Omp33-36-RT-R | GCACCAGCTTCTAATGCATAACG | ||
CarO-RT-F | GGCGGATGAAGCTGTTGTTC | carO | 33 |
CarO-RT-R | CACGAGCGCCTACTGGAATT | ||
OprD-RT-F | CGCCCGGCCATGTATCA | oprD | 33 |
OprD-FT-R | GCGACACCGCGTTTCCATAT | ||
OmpA-RT-F | AGCTCTTGCTGGCTTAAACG | ompA | 34 |
OmpA-RT-R | GAGCAACTGGAGTTGGTTCA | ||
AdeB-RT-F | GGATTATGGCGACAGAAGGA | adeB | 35 |
AdeB-RT-R | AATACTGCCGCCAATACCAG | ||
AdeJ-RT-F | GGTCATTAATATCTTTGGC | adeJ | 16 |
AdeJ-RT-R | GGTACGAATACCGCTGTCA | ||
AbeM-RT-F | GCTATTCCGAAGCATTAGGC | abeM | 34 |
AbeM-RT-R | CCAAAGCAGGTATTGGTCCT | ||
AbeS-RT-F | CGTGGCGTGGGATATAGACT | abeS | 34 |
AbeS-RT-R | AGGAAAATGCCACAAAATGG | ||
AmvA-RT1 | TGTATTCCTCATTAATATCCC | amvA | 17 |
AmvA-RT2 | TTACTTTCTTCGGAAAACTAAACC |
Primer . | Nucleotide sequence (5′-3′) . | Target . | Reference . |
---|---|---|---|
Omp33-36-RT-F | TCCAACTCCTTACTTGCCAGTTTA | omp 33-36 | 33 |
Omp33-36-RT-R | GCACCAGCTTCTAATGCATAACG | ||
CarO-RT-F | GGCGGATGAAGCTGTTGTTC | carO | 33 |
CarO-RT-R | CACGAGCGCCTACTGGAATT | ||
OprD-RT-F | CGCCCGGCCATGTATCA | oprD | 33 |
OprD-FT-R | GCGACACCGCGTTTCCATAT | ||
OmpA-RT-F | AGCTCTTGCTGGCTTAAACG | ompA | 34 |
OmpA-RT-R | GAGCAACTGGAGTTGGTTCA | ||
AdeB-RT-F | GGATTATGGCGACAGAAGGA | adeB | 35 |
AdeB-RT-R | AATACTGCCGCCAATACCAG | ||
AdeJ-RT-F | GGTCATTAATATCTTTGGC | adeJ | 16 |
AdeJ-RT-R | GGTACGAATACCGCTGTCA | ||
AbeM-RT-F | GCTATTCCGAAGCATTAGGC | abeM | 34 |
AbeM-RT-R | CCAAAGCAGGTATTGGTCCT | ||
AbeS-RT-F | CGTGGCGTGGGATATAGACT | abeS | 34 |
AbeS-RT-R | AGGAAAATGCCACAAAATGG | ||
AmvA-RT1 | TGTATTCCTCATTAATATCCC | amvA | 17 |
AmvA-RT2 | TTACTTTCTTCGGAAAACTAAACC |
Primer . | Nucleotide sequence (5′-3′) . | Target . | Reference . |
---|---|---|---|
Omp33-36-RT-F | TCCAACTCCTTACTTGCCAGTTTA | omp 33-36 | 33 |
Omp33-36-RT-R | GCACCAGCTTCTAATGCATAACG | ||
CarO-RT-F | GGCGGATGAAGCTGTTGTTC | carO | 33 |
CarO-RT-R | CACGAGCGCCTACTGGAATT | ||
OprD-RT-F | CGCCCGGCCATGTATCA | oprD | 33 |
OprD-FT-R | GCGACACCGCGTTTCCATAT | ||
OmpA-RT-F | AGCTCTTGCTGGCTTAAACG | ompA | 34 |
OmpA-RT-R | GAGCAACTGGAGTTGGTTCA | ||
AdeB-RT-F | GGATTATGGCGACAGAAGGA | adeB | 35 |
AdeB-RT-R | AATACTGCCGCCAATACCAG | ||
AdeJ-RT-F | GGTCATTAATATCTTTGGC | adeJ | 16 |
AdeJ-RT-R | GGTACGAATACCGCTGTCA | ||
AbeM-RT-F | GCTATTCCGAAGCATTAGGC | abeM | 34 |
AbeM-RT-R | CCAAAGCAGGTATTGGTCCT | ||
AbeS-RT-F | CGTGGCGTGGGATATAGACT | abeS | 34 |
AbeS-RT-R | AGGAAAATGCCACAAAATGG | ||
AmvA-RT1 | TGTATTCCTCATTAATATCCC | amvA | 17 |
AmvA-RT2 | TTACTTTCTTCGGAAAACTAAACC |
Statistical analysis
Qualitative variables were compared using the χ2 test or Fisher's exact test, as appropriate. Quantitative variables were compared using Student's t-test. Differences with P < 0.05 were considered statistically significant.
Results
Susceptibility to biocides
As Table 2 shows, the MIC values of the biocides tested were quite variable and in the following ranges: 1328–5313 mg/L (Sterillium®); 156.3–312.5 mg/L (Orsan®); 625–1250 mg/L (domestic bleach); 2.4–39.1 mg/L (chlorhexidine digluconate); 1–15.6 mg/L (benzalkonium chloride); 1.1–8.9 mg/L (ethanol); and 0.05–7.5 mg/L (Irgasan®). The MBC values were also variable (Table 2): 1328–10 626 mg/L (Sterillium®); 156.3–625 mg/L (Orsan®); 625–2500 mg/L (domestic bleach); 2.4–156.4 mg/L (chlorhexidine digluconate); 1–124.8 mg/L (benzalkonium chloride); 1.1–71.2 mg/L (ethanol); and 0.05–7.5 mg/L (Irgasan®).
Association between RSB and antimicrobial resistance
Reduced susceptibility to chlorhexidine digluconate was associated with resistance to carbapenems (imipenem, P = 0.002; meropenem, P = 0.002), aminoglycosides (gentamicin, P = 0.007; tobramycin, P = 0.05; netilmicin, P < 0.001), tetracycline (P = 0.02) and doxycycline (P = 0.005) (Table S1 and Table S2, available as Supplementary data at JAC Online). Reduced susceptibility to Irgasan® was associated with resistance to sulbactam (P = 0.004), ceftazidime (P = 0.04), carbapenems (imipenem, P = 0.006; meropenem, P = 0.006), aminoglycosides (gentamicin, P < 0.001; tobramycin, P < 0.001; amikacin, P = 0.03; netilmicin, P = 0.001), tetracycline (P = 0.002) and ciprofloxacin (P < 0.001). Reduced susceptibility to benzalkonium chloride was associated with resistance to aminoglycosides (gentamicin, P = 0.01; netilmicin, P = 0.04), tetracycline (P = 0.01), doxycycline (P = 0.003) and ciprofloxacin (P = 0.02). In contrast, resistance to Sterillium®, ethanol, domestic bleach or Orsan® was not associated with resistance to the antimicrobial agents assayed (Supplementary Data).
The MICs of antimicrobial agents for isolates Ab46 and Ab70 exposed to biocides are shown in Table 3. For isolate Ab46, the MICs of antimicrobial agents were the same, irrespective of previous exposure to chlorhexidine digluconate. In contrast, when exposed to benzalkonium chloride, the MICs of ciprofloxacin and colistin (but not other antimicrobials) increased by ≥2 dilutions (from 32 to >64 mg/L for ciprofloxacin and from <0.5 to 1 mg/L for colistin). For isolate Ab46 exposed to Irgasan®, only the MICs of ciprofloxacin, minocycline and doxycycline increased (>64, 2 and 64 mg/L, respectively), with respect to isolate Ab46 not exposed to Irgasan® (32, <0.5 and 16 mg/L, respectively).
The effect of previous exposure to biocides on the increase in the MICs of antimicrobial agents was more evident for isolate Ab70 than for isolate Ab46 (Table 3). For isolate Ab70 exposed to chlorhexidine digluconate, there was a ≥2-fold increase in the MICs of sulbactam, imipenem, meropenem, ciprofloxacin, tobramycin, amikacin, minocycline, netilmicin and doxycycline, compared with isolate Ab70 not exposed to chlorhexidine digluconate. In contrast, there was a ≥2-fold decrease in the MIC of gentamicin. Exposure of isolate Ab70 to benzalkonium chloride produced a ≥2-fold increase in the MICs of sulbactam, imipenem, meropenem, ciprofloxacin, gentamicin, tobramycin, amikacin, netilmicin, doxycycline and tigecycline. Exposure of isolate Ab70 to Irgasan® increased ≥2-fold only the MICs of ciprofloxacin, gentamicin, amikacin, minocycline, netilmicin and doxycycline.
In order to determine the ability of biocides to select for subpopulations with higher MICs of biocides than the parent strain, the following study was carried out. One colony was selected from isolates Ab46 and Ab70 after exposure to chlorhexidine digluconate, benzalkonium chloride or Irgasan®. The MICs of these biocides after seven subcultures in Mueller–Hinton agar were the same as or 1 dilution lower than those observed before subculture (Figure 1).
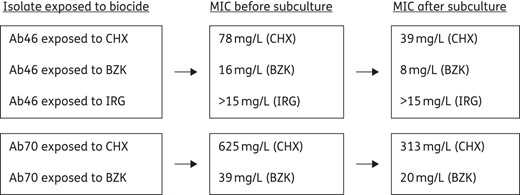
MICs (mg/L) of biocides before and after subculture in Mueller–Hinton agar of isolates Ab46 and Ab70 exposed to chlorhexidine digluconate (CHX), benzalkonium chloride (BZK) or Irgasan® (IRG).
Association between RSB and epidemiological behaviour
The association between reduced susceptibility to Orsan® and epidemiological behaviour was the only statistically significant association. Reduced susceptibility to Orsan® was found in 23/33 (69.7%) of prevalent clones and 7/25 (28.0%) of non-prevalent clones (P = 0.002).
The MIC of Orsan® was 313 mg/L for 69.7% of epidemic clones and 28.0% of sporadic clones and 156 mg/L for 30.3% of epidemic clones and 72.0% of sporadic clones.
Biological cost associated with the acquisition of RSB
Mean generation times (min) were 57.8 ± 2.1 (isolate Ab70), 67.9 ± 5.3 (Ab70 exposed to Irgasan®), 63.4 ± 8.2 (Ab70 exposed to chlorhexidine digluconate) and 78.1 ± 5.7 (Ab70 exposed to benzalkonium chloride, P = 0.02).
Effect of RSB on gene expression
Exposure of isolate Ab46 to chlorhexidine digluconate was associated with a significant increase (>2-fold) in the relative gene expression of adeB, abeS and amvA, whereas exposure to benzalkonium chloride was associated with an increase in the relative expression of abeS (Table 5). Exposure to Irgasan® was associated with an increase in the expression of adeJ, but a decrease in the expression of abeM and amvA. For isolate Ab70, exposure to chlorhexidine digluconate was associated with a significant increase in the expression of adeB and abeS. Exposure to benzalkonium chloride was associated with an increase in the expression of adeB and abeS, and a decrease in the expression of abeM. Exposure to Irgasan® was associated with an increase in the expression of abeS and a decrease in the expression of abeM and amvA.
Relative gene expression (mean ± SD) for Ab46 and Ab70 isolates exposed and not exposed to biocides
Gene . | Ab46 exposed to . | Ab70 exposed to . | ||||
---|---|---|---|---|---|---|
chlorhexidine digluconate . | benzalkonium chloride . | Irgasan® . | chlorhexidine digluconate . | benzalkonium chloride . | Irgasan® . | |
adeB | 2.4 ± 0.9 | 1.2 ± 0.1 | 0.6 ± 0.1 | 34.7 ± 6.7 | 13.3 ± 0.8 | 0.6 ± 0.1 |
adeJ | 1.5 ± 0.1 | 0.7 ± 0.1 | 3.4 ± 0.1 | 1.1 ± 0.0 | 1.0 ± 0.3 | 0.9 ± 0.1 |
abeM | 0.7 ± 0.4 | 0.5 ± 0.1 | 0.4 ± 0.1 | 0.6 ± 0.2 | 0.4 ± 0.1 | 0.3 ± 0.1 |
abeS | 3.5 ± 0.3 | 2.2 ± 1.4 | 2.0 ± 0.5 | 2.2 ± 1.3 | 4.0 ± 0.8 | 2.2 ± 0.6 |
amvA | 14.2 ± 3.9 | 0.6 ± 0.1 | 0.3 ± 0.1 | 0.8 ± 0.3 | 0.5 ± 0.1 | 0.3 ± 0.1 |
ompA | 0.8 ± 0.5 | 0.5 ± 0.1 | 0.2 ± 0.0 | 0.4 ± 0.1 | 0.4 ± 0.1 | 0.2 ± 0.0 |
carO | 0.5 ± 0.0 | 0.3 ± 0.1 | 0.2 ± 0.0 | 0.8 ± 0.4 | 0.2 ± 0.0 | 0.0 ± 0.0 |
omp 33-36 | 1.5 ± 0.4 | 1.3 ± 0.6 | 1.5 ± 0.6 | 1.1 ± 0.3 | 1.5 ± 0.5 | 0.3 ± 0.2 |
oprD | ND | ND | ND | ND | ND | ND |
Gene . | Ab46 exposed to . | Ab70 exposed to . | ||||
---|---|---|---|---|---|---|
chlorhexidine digluconate . | benzalkonium chloride . | Irgasan® . | chlorhexidine digluconate . | benzalkonium chloride . | Irgasan® . | |
adeB | 2.4 ± 0.9 | 1.2 ± 0.1 | 0.6 ± 0.1 | 34.7 ± 6.7 | 13.3 ± 0.8 | 0.6 ± 0.1 |
adeJ | 1.5 ± 0.1 | 0.7 ± 0.1 | 3.4 ± 0.1 | 1.1 ± 0.0 | 1.0 ± 0.3 | 0.9 ± 0.1 |
abeM | 0.7 ± 0.4 | 0.5 ± 0.1 | 0.4 ± 0.1 | 0.6 ± 0.2 | 0.4 ± 0.1 | 0.3 ± 0.1 |
abeS | 3.5 ± 0.3 | 2.2 ± 1.4 | 2.0 ± 0.5 | 2.2 ± 1.3 | 4.0 ± 0.8 | 2.2 ± 0.6 |
amvA | 14.2 ± 3.9 | 0.6 ± 0.1 | 0.3 ± 0.1 | 0.8 ± 0.3 | 0.5 ± 0.1 | 0.3 ± 0.1 |
ompA | 0.8 ± 0.5 | 0.5 ± 0.1 | 0.2 ± 0.0 | 0.4 ± 0.1 | 0.4 ± 0.1 | 0.2 ± 0.0 |
carO | 0.5 ± 0.0 | 0.3 ± 0.1 | 0.2 ± 0.0 | 0.8 ± 0.4 | 0.2 ± 0.0 | 0.0 ± 0.0 |
omp 33-36 | 1.5 ± 0.4 | 1.3 ± 0.6 | 1.5 ± 0.6 | 1.1 ± 0.3 | 1.5 ± 0.5 | 0.3 ± 0.2 |
oprD | ND | ND | ND | ND | ND | ND |
ND, not detected.
Changes in gene expression >2-fold appear in bold.
Relative gene expression (mean ± SD) for Ab46 and Ab70 isolates exposed and not exposed to biocides
Gene . | Ab46 exposed to . | Ab70 exposed to . | ||||
---|---|---|---|---|---|---|
chlorhexidine digluconate . | benzalkonium chloride . | Irgasan® . | chlorhexidine digluconate . | benzalkonium chloride . | Irgasan® . | |
adeB | 2.4 ± 0.9 | 1.2 ± 0.1 | 0.6 ± 0.1 | 34.7 ± 6.7 | 13.3 ± 0.8 | 0.6 ± 0.1 |
adeJ | 1.5 ± 0.1 | 0.7 ± 0.1 | 3.4 ± 0.1 | 1.1 ± 0.0 | 1.0 ± 0.3 | 0.9 ± 0.1 |
abeM | 0.7 ± 0.4 | 0.5 ± 0.1 | 0.4 ± 0.1 | 0.6 ± 0.2 | 0.4 ± 0.1 | 0.3 ± 0.1 |
abeS | 3.5 ± 0.3 | 2.2 ± 1.4 | 2.0 ± 0.5 | 2.2 ± 1.3 | 4.0 ± 0.8 | 2.2 ± 0.6 |
amvA | 14.2 ± 3.9 | 0.6 ± 0.1 | 0.3 ± 0.1 | 0.8 ± 0.3 | 0.5 ± 0.1 | 0.3 ± 0.1 |
ompA | 0.8 ± 0.5 | 0.5 ± 0.1 | 0.2 ± 0.0 | 0.4 ± 0.1 | 0.4 ± 0.1 | 0.2 ± 0.0 |
carO | 0.5 ± 0.0 | 0.3 ± 0.1 | 0.2 ± 0.0 | 0.8 ± 0.4 | 0.2 ± 0.0 | 0.0 ± 0.0 |
omp 33-36 | 1.5 ± 0.4 | 1.3 ± 0.6 | 1.5 ± 0.6 | 1.1 ± 0.3 | 1.5 ± 0.5 | 0.3 ± 0.2 |
oprD | ND | ND | ND | ND | ND | ND |
Gene . | Ab46 exposed to . | Ab70 exposed to . | ||||
---|---|---|---|---|---|---|
chlorhexidine digluconate . | benzalkonium chloride . | Irgasan® . | chlorhexidine digluconate . | benzalkonium chloride . | Irgasan® . | |
adeB | 2.4 ± 0.9 | 1.2 ± 0.1 | 0.6 ± 0.1 | 34.7 ± 6.7 | 13.3 ± 0.8 | 0.6 ± 0.1 |
adeJ | 1.5 ± 0.1 | 0.7 ± 0.1 | 3.4 ± 0.1 | 1.1 ± 0.0 | 1.0 ± 0.3 | 0.9 ± 0.1 |
abeM | 0.7 ± 0.4 | 0.5 ± 0.1 | 0.4 ± 0.1 | 0.6 ± 0.2 | 0.4 ± 0.1 | 0.3 ± 0.1 |
abeS | 3.5 ± 0.3 | 2.2 ± 1.4 | 2.0 ± 0.5 | 2.2 ± 1.3 | 4.0 ± 0.8 | 2.2 ± 0.6 |
amvA | 14.2 ± 3.9 | 0.6 ± 0.1 | 0.3 ± 0.1 | 0.8 ± 0.3 | 0.5 ± 0.1 | 0.3 ± 0.1 |
ompA | 0.8 ± 0.5 | 0.5 ± 0.1 | 0.2 ± 0.0 | 0.4 ± 0.1 | 0.4 ± 0.1 | 0.2 ± 0.0 |
carO | 0.5 ± 0.0 | 0.3 ± 0.1 | 0.2 ± 0.0 | 0.8 ± 0.4 | 0.2 ± 0.0 | 0.0 ± 0.0 |
omp 33-36 | 1.5 ± 0.4 | 1.3 ± 0.6 | 1.5 ± 0.6 | 1.1 ± 0.3 | 1.5 ± 0.5 | 0.3 ± 0.2 |
oprD | ND | ND | ND | ND | ND | ND |
ND, not detected.
Changes in gene expression >2-fold appear in bold.
The relative expression of oprD was very low and it was not possible to determine the effect of exposure to biocides on the expression of this gene. For isolate Ab46, exposure to chlorhexidine digluconate had no significant effect on the relative expression of ompA, carO and omp 33-36 genes (Table 5). In contrast, exposure to benzalkonium chloride was associated with a decrease in the relative expression of carO, whereas exposure to Irgasan® was associated with a decreased expression of ompA and carO. For isolate Ab70, exposure to chlorhexidine digluconate was associated with a decrease in the relative expression of the ompA gene, whereas exposure to benzalkonium chloride was associated with decreased relative expression of the ompA and carO genes. Exposure to Irgasan® was associated with decreased expression of the ompA, carO and omp 33-36 genes.
Discussion
The success of A. baumannii in adapting to hospital environments has been attributed to its great capacity for acquiring multi-resistance to antimicrobials and for surviving in the hospital environment. The persistence of A. baumannii in this environment could potentially be favoured by reduced susceptibility to commonly used biocides. However, the role of reduced susceptibility to biocides such as antiseptics and disinfectants that are commonly used in hospital settings is controversial; this is probably the result of the very few studies performed, the different methodologies used to test susceptibility to biocides, the criteria and terminology used to define resistance or tolerance to biocides, and the small number of isolates and biocides analysed.
Several studies have demonstrated that improper use of biocides can lead to the ineffective eradication of A. baumannii from the hospital environment due to the selection of isolates with RSB.24,25 The A. baumannii strains included in this study showed variable susceptibility to biocides, particularly those that are good substrates of efflux pumps, such as chlorhexidine digluconate or benzalkonium chloride. This variability may imply a competitive advantage in isolates that are more resistant to biocides, since they may be more easily selected for in the presence of biocides than those that are more susceptible to them.26
In order to evaluate whether acquisition of RSB was reversible (tolerance) or not (mutational resistance), we performed several subcultures of Ab46 and Ab70 isolates exposed to chlorhexidine digluconate, benzalkonium chloride or Irgasan® in a biocide-free medium. The MICs obtained after subculture were equal to or differed by 1 dilution from those obtained before exposure, suggesting that tolerance or adaptation to growth in the presence of biocides was more probably related to acquisition of RSB than to selection of resistant mutants. The role of tolerance is also supported by the results of MBC experiments, since most of the MBC values were 1 or 2 dilutions lower than the MIC. Isolates recovered from wells corresponding to the MBC showed no change in MIC, which indicates that these A. baumannii isolates are able to support the inhibitory effect of biocides at slightly higher concentrations than the respective MIC. It is not likely that this represents a potential problem from an epidemiological point of view, due to the high in-house or recommended concentrations used in hospitals. Nevertheless, it is possible that inefficient cleaning out with biocides facilitates the selection and dissemination of A. baumannii, as has been suggested.4,6,7,9
There is a general hypothesis that acquisition of RSB may be linked to antimicrobial resistance (co-resistance).12,27 Thus, the presence of subinhibitory concentrations of biocides could select for strains with decreased susceptibility to biocides and thereafter to antimicrobials. This association has been studied in A. baumannii, although no clear association between resistance to biocides and antimicrobials has been demonstrated.28–30 In contrast, we observed a statistically significant association between decreased susceptibility to some biocides and resistance to antimicrobials. Isolates that showed reduced susceptibility to chlorhexidine digluconate, benzalkonium chloride or Irgasan® were also more resistant to some antimicrobials, particularly ciprofloxacin, carbapenems, aminoglycosides and/or tetracyclines. This finding was confirmed by the results obtained with Ab46 isolates and, in particular, with Ab70 exposed to chlorhexidine digluconate, benzalkonium chloride or Irgasan®.
Acquisition of RSB means a competitive advantage that may be associated with a biological cost, although there are no data in the literature supporting this hypothesis in A. baumannii. The acquisition of reduced susceptibility to chlorhexidine digluconate or Irgasan® by the isolate Ab70 had no impact on the generation time, whereas against benzalkonium chloride it was associated with a significant increase in the generation time. These data suggest that biological cost is dependent on the biocide and the strain, although this statement should be interpreted with caution and more studies including more isolates and other more appropriate assays are required to confirm these results.
Acquisition of reduced susceptibility to Orsan® was more frequently observed among prevalent clones than non-prevalent clones, so that this biocide would be expected to play some role in the development of the epidemic potential of A. baumannii clones. This finding should be interpreted with caution since the difference between what is defined as RSB and no RSB is only a 2-fold change in MIC and this small difference could not be reliably associated with prevalent or non-prevalent clones. It would, in any case, be of interest to analyse whether reduced susceptibility to Orsan® is associated with certain efflux pumps, although there is no information on this.
The action of biocides is usually non-specific, although some of them, as well as some antimicrobials, may be good substrates of some efflux pumps.12 The acquisition of RSB and antimicrobial resistance may be achieved by reduced outer membrane permeability (mediated by reduced porin expression) and/or by overexpression of one or several efflux pumps, as has been observed in other microorganisms.6,10,14,31 In A. baumannii it has been observed that the RND-type efflux pump AdeABC and the non-RND efflux pump AmvA are able to extrude biocides out of the cell. Rajamohan et al.18 observed that inactivation of adeB or adeJ genes led to increased susceptibility to biocides. Our results for expression showed that exposure to chlorhexidine digluconate or benzalkonium chloride increased the expression of adeB and/or amvA, and these results are in agreement with a recent study performed on ATCC strain 17978, where transcriptome analysis showed that exposure to chlorhexidine digluconate induced the up-regulation of several genes, including adeB and aceI, coding for an active chlorhexidine efflux protein.32
Chlorhexidine digluconate and benzalkonium chloride were also associated with decreased expression of the genes encoding OmpA and/or CarO porins. Additional experiments are required to resolve the question of whether the effect of biocides is a consequence of the induction (direct or indirect) of the expression of these efflux pumps and porins or the result of selecting subpopulations with RSB-containing mutations that affect the level of resistance to biocides.
In conclusion, A. baumannii shows variable susceptibility to commonly used biocides (chlorhexidine digluconate, benzalkonium chloride and Irgasan®) and reduced susceptibility to these biocides seems to induce co-resistance to some antimicrobial agents (quinolones, aminoglycosides, carbapenems and tetracyclines). The biological cost associated with the acquisition of RSB depends on the type of isolate and the biocide. Epidemic clones are more resistant than sporadic clones to Orsan®. Exposure to chlorhexidine digluconate and benzalkonium chloride is associated with overexpression of genes encoding some efflux pumps (AdeB and AmvA) and reduced expression of genes coding for some porins (OmpA and CarO).
Funding
This work was supported by the Ministerio de Sanidad y Consumo, Instituto de Salud Carlos III (projects PI11-02046 and PI10/00056) and the Consejería de Innovación Ciencia y Empresa, Junta de Andalucía (P11-CTS-7730), Spain, by the Plan Nacional de I+D+i 2008-2011 and the Instituto de Salud Carlos III, Subdirección General de Redes y Centros de Investigación Cooperativa, Ministerio de Economía y Competitividad, the Spanish Network for Research in Infectious Diseases (REIPI RD12/0015)—co-financed by European Development Regional Fund ‘A way to achieve Europe’ ERDF.
Transparency declarations
None to declare.
Acknowledgements
We would like to thank the following organizations and researchers who participated in the study: Virgen Rocío (José Garnacho, Antonio Gutierrez-Pizarraya, Juan Antonio Márquez-Vácaro); Hospital Marqués de Valdecilla (María Eliecer Cano, M. Carmen Fariñas); Hospital SAS La Línea (Antonio Sánchez-Porto, Gloria Esteban Meruendano, Luis Barbeyto-Vales, Javier Casas-Ciria, Luis Vallejo); Complejo hospitalario de Ourense (Begona Fernández-Pérez, José Carlos Villar-Chao); Hospital Gregorio Maranón (Belén Padilla-Ortega, Emilia Cercenado-Mansilla); Hospital de Navarra (José Javier García-Irure); Hospital Costa del Sol-Marbella (Alfonso del Arco Jiménez); Hospital General de Valencia (Concepción Gimeno-Cardona, Juan Carlos Valía, Núria Tormo-Palop, Vicente Abril, Josefina Rifa, Maria Jesus Martinez-Garcia); Consorci Hospitalari de Vic (Joseph Vilaró-Pujals, Marian Navarro Aguirre, Ana Vilamala); Policlínica Guipúzkoa (José Antonio Jiménez-Alfaro, Carlos Reviejo-Jaca); Hospital Puerta del Mar (Pilar Marín Casanova, Francisca Guerreo, Evelyn Shaw, Virginia Plasencia); Complejo Hospitalario de Soria (Teresa Nebreda-Mayoral, María José Fernández-Calavia, Susana García de Cruz, Carmen Aldea-Mansilla); Hospital Universitario de Alicante (Esperanza Merino de Lucas, Alfredo Zorraquino, Sergio Reus-Bañuls); Hospital Infanta Cristina (Eugenio Garduno-Eseverri, Luis López Sánchez); Hospital Universitario Central de Asturias (Ana Fleites-Gutiérrez, Azucena Rodríguez-Guardado, Alfonso Moreno); Hospital Donostia (José María García-Arenzana Anguera); Complejo Hospitalario Torrecárdenas (Serafín López-Palmero, Manuel Rodríguez-Maresca); Complejo Hospitalario Xeral-Calde Lugo (Fernando García-Garrote, José Varela-Otero, María del Pilar Alonso); Hospital Universitario Reina Sofía de Córdoba (Elisa Vidal-Verdú, Fernando Rodríguez-López); Hospital Universitario Santiago Compostela (Fernanda Pardo-Sánchez, E. Ferrer-Vizoso, B. Regueiro-Garcia); Hospital Sant Pau (Mercé Gurgui, Roser Pericas, Virginia Pomar); Hospital Galdakao-Usansolo (Pedro María Olaechea-Astigarraga, Rafael Ayarza-Igartua); Hospital Son Dureta (María Dolores Maciá-Romero, Enrique Ruiz de Gopegui-Bordes); Hospital Puerta de Hierro (María Isabel Sánchez-Romero); Hospital Juan Grande (Jesús García-Mata, María José Goyanes, Cristina Morales-Mateos); Hospital San Cecilio (José Hernández-Quero, Trinidad Escobar-Lara); Hospital Sant Joan de Reus (Frederic Ballester-Bastardie, Simona Iftimie, Isabel Pujol-Bajador); Hospital de Motril (María Isabel Galán-Navarro, María Luz Cádiz-Gurrea); Hospital San Agustín (Carmen Amores-Antequera, Montserrat Gómez, Purificación Cantudo); Hospital de Granollers (Carmina Martí-Salas, Jordi Cuquet-Peragosa, Antonio Moreno-Flores, Luis Anibarro-García); Hospital de Segovia (Susana Hernando-Real, Pablo A. Carrero-González); Complejo Hospitalario de Pontevedra (María Angeles Pallarés-González, Sergio Rodríguez-Fernández); Hospital de Bellvitge (Miquel Pujol-Rojo, Fe Tubau); Hospital Virgen de la Victoria de Málaga (Enrique Nuno-Alvarez, María Ortega-Torres); Hospital Doctor Moliner (Salvador Giner-Almaraz, María Rosa Roca-Castelló, Manuela Castillo, Elena Hortelano); Hospital 12 de Octubre (Fernando Chaves-Sánchez, Ana García-Reyne); Hospital del Mar (Juan Pablo Horcajada-Gallego, Concha Segura); Hospital San Agustín de Avilés (Gema Sierra-Dorado, Raquel Yano-Escudero); Complejo Hospitalario Materno Insular de Gran Canaria (María Elena Dorta-Hung, Cristóbal del Rosario Q).
References
Author notes
Other members are listed in the Acknowledgements section.