-
PDF
- Split View
-
Views
-
Cite
Cite
Euna Oh, Qijing Zhang, Byeonghwa Jeon, Target optimization for peptide nucleic acid (PNA)-mediated antisense inhibition of the CmeABC multidrug efflux pump in Campylobacter jejuni, Journal of Antimicrobial Chemotherapy, Volume 69, Issue 2, February 2014, Pages 375–380, https://doi.org/10.1093/jac/dkt381
- Share Icon Share
Abstract
CmeABC is a resistance-nodulation-cell division (RND)-type multidrug efflux pump conferring resistance to clinically important antibiotics in Campylobacter. This study aimed to identify the optimal target sites for the inhibition of CmeABC with antisense peptide nucleic acid (PNA).
Eighteen PNAs were designed to bind to the translational initiation regions of cmeABC, spanning the ribosome-binding site (RBS) and the start codon of the cmeABC genes. Campylobacter jejuni was treated with CmeABC-specific PNAs (CmeABC-PNAs) at various concentrations and subjected to western blotting to measure changes in the level of CmeABC expression. The MICs of ciprofloxacin and erythromycin were measured to evaluate the impact of CmeABC knockdown on antibiotic susceptibility.
While antisense PNA significantly affected CmeA and CmeB expression, interestingly, CmeC expression was not altered by any of the CmeC-PNAs used in this study. A CmeA-PNA targeting the RBS of cmeA and its upstream region reduced CmeA expression most efficiently, and CmeB expression was most significantly decreased by PNA binding to the RBS of cmeB and its downstream region. CmeA- and CmeB-PNAs increased the susceptibility of C. jejuni to ciprofloxacin and erythromycin in proportion to the inhibition levels observed in western blotting.
The cmeA gene is the best target to knockdown CmeABC with antisense PNA. The RBS is the major target for the PNA-mediated antisense inhibition of CmeABC. However, regions in its vicinity also significantly influence the effectiveness of the PNA-based knockdown of CmeABC.
Introduction
Campylobacter jejuni is a leading foodborne pathogen resulting in ∼400 million–500 million cases of human infection around the world annually.1C. jejuni infection is usually self-limiting and does not require antibiotic treatment; however, antibiotics are prescribed for severe cases or in immunocompromised patients.2 The drugs of choice for the treatment of human campylobacteriosis include fluoroquinolones and macrolides, but C. jejuni has developed increasing resistance to these clinically important antibiotics, particularly fluoroquinolones.3
Drug efflux pumps mediate the extrusion of structurally unrelated antibiotics out of the cell and are considered to be a major mechanism responsible for multidrug resistance.4 To potentiate existing antibiotics and control antibiotic resistance associated with drug efflux pumps, numerous studies have been reported to develop efflux pump inhibitors.5 However, inhibitors of prokaryotic efflux pumps can also interfere with eukaryotic efflux systems, resulting in toxicity problems, and this is considered to be a major barrier in the development of efflux pump inhibitors.5
In Campylobacter, CmeABC is a key resistance determinant that confers both intrinsic and acquired resistance to clinically important antibiotics.6,7 In addition, CmeABC significantly affects the ability of C. jejuni to colonize chicken intestines due to its importance in resistance to bile salts.8 CmeABC is a resistance-nodulation-cell division (RND)-type efflux pump consisting of three protein components located in the inner membrane (CmeB), periplasm (CmeA) and outer membrane (CmeC), which are encoded by the polycistronic cmeABC operon.6 In our previous report, we demonstrated that an antisense peptide nucleic acid (PNA) targeting CmeA sensitized C. jejuni to ciprofloxacin and erythromycin.9 However, the targets for antisense PNA have not been optimized to maximize the susceptibility of C. jejuni to antibiotics by CmeABC knockdown. In this study, we identified the optimal target sites in cmeABC that are most susceptible to the inhibition of antisense CmeABC-specific PNAs (CmeABC-PNAs).
Materials and methods
Bacterial strains and CmeABC-PNAs
C. jejuni NCTC 11168 was the first Campylobacter to have its genome sequenced,10 and was used throughout this study. C. jejuni was grown at 42°C with Mueller–Hinton (MH) medium (Oxoid) in microaerobic conditions (5% O2, 85% N2 and 10% CO2). CmeABC-PNAs were designed based on the genome sequence of C. jejuni NCTC 11168,10 and were commercially synthesized by Panagene (Daejeon, South Korea; Table 1). To improve antisense potency, CmeABC-PNAs were conjugated with the cell-permeabilizing peptide KFFKFFKFFF, as described elsewhere.11
PNA . | Sequence (N→C) . |
---|---|
CmeA-PNA1 | KFFKFFKFFK-TGCCTTGAAAAA |
CmeA-PNA2 | KFFKFFKFFK-TTTTGCCTTGAA |
CmeA-PNA3 | KFFKFFKFFK-TGGTTTTGCCTT |
CmeA-PNA4 | KFFKFFKFFK-TCATGGTTTTGC |
CmeA-PNA5 | KFFKFFKFFK-ATTTCATGGTTT |
CmeA-PNA6 | KFFKFFKFFK-AATAATTTCATG |
CmeB-PNA1 | KFFKFFKFFK-CTCCAATTTCTT |
CmeB-PNA2 | KFFKFFKFFK-GTGCTCCAATTT |
CmeB-PNA3 | KFFKFFKFFK-ATTGTGCTCCAA |
CmeB-PNA4 | KFFKFFKFFK-ATTATTGTGCTC |
CmeB-PNA5 | KFFKFFKFFK-AAAACATTATTG |
CmeB-PNA6 | KFFKFFKFFK-TTAGAAAACATT |
CmeC-PNA1 | KFFKFFKFFK-ACCTCTTTTTTT |
CmeC-PNA2 | KFFKFFKFFK-TTACCTCTTTTT |
CmeC-PNA3 | KFFKFFKFFK-ACCTTACCTCTT |
CmeC-PNA4 | KFFKFFKFFK-ATGAACCTTACC |
CmeC-PNA5 | KFFKFFKFFK-TATTCATGAACC |
CmeC-PNA6 | KFFKFFKFFK-ATTTTATTCATG |
PNA . | Sequence (N→C) . |
---|---|
CmeA-PNA1 | KFFKFFKFFK-TGCCTTGAAAAA |
CmeA-PNA2 | KFFKFFKFFK-TTTTGCCTTGAA |
CmeA-PNA3 | KFFKFFKFFK-TGGTTTTGCCTT |
CmeA-PNA4 | KFFKFFKFFK-TCATGGTTTTGC |
CmeA-PNA5 | KFFKFFKFFK-ATTTCATGGTTT |
CmeA-PNA6 | KFFKFFKFFK-AATAATTTCATG |
CmeB-PNA1 | KFFKFFKFFK-CTCCAATTTCTT |
CmeB-PNA2 | KFFKFFKFFK-GTGCTCCAATTT |
CmeB-PNA3 | KFFKFFKFFK-ATTGTGCTCCAA |
CmeB-PNA4 | KFFKFFKFFK-ATTATTGTGCTC |
CmeB-PNA5 | KFFKFFKFFK-AAAACATTATTG |
CmeB-PNA6 | KFFKFFKFFK-TTAGAAAACATT |
CmeC-PNA1 | KFFKFFKFFK-ACCTCTTTTTTT |
CmeC-PNA2 | KFFKFFKFFK-TTACCTCTTTTT |
CmeC-PNA3 | KFFKFFKFFK-ACCTTACCTCTT |
CmeC-PNA4 | KFFKFFKFFK-ATGAACCTTACC |
CmeC-PNA5 | KFFKFFKFFK-TATTCATGAACC |
CmeC-PNA6 | KFFKFFKFFK-ATTTTATTCATG |
PNA . | Sequence (N→C) . |
---|---|
CmeA-PNA1 | KFFKFFKFFK-TGCCTTGAAAAA |
CmeA-PNA2 | KFFKFFKFFK-TTTTGCCTTGAA |
CmeA-PNA3 | KFFKFFKFFK-TGGTTTTGCCTT |
CmeA-PNA4 | KFFKFFKFFK-TCATGGTTTTGC |
CmeA-PNA5 | KFFKFFKFFK-ATTTCATGGTTT |
CmeA-PNA6 | KFFKFFKFFK-AATAATTTCATG |
CmeB-PNA1 | KFFKFFKFFK-CTCCAATTTCTT |
CmeB-PNA2 | KFFKFFKFFK-GTGCTCCAATTT |
CmeB-PNA3 | KFFKFFKFFK-ATTGTGCTCCAA |
CmeB-PNA4 | KFFKFFKFFK-ATTATTGTGCTC |
CmeB-PNA5 | KFFKFFKFFK-AAAACATTATTG |
CmeB-PNA6 | KFFKFFKFFK-TTAGAAAACATT |
CmeC-PNA1 | KFFKFFKFFK-ACCTCTTTTTTT |
CmeC-PNA2 | KFFKFFKFFK-TTACCTCTTTTT |
CmeC-PNA3 | KFFKFFKFFK-ACCTTACCTCTT |
CmeC-PNA4 | KFFKFFKFFK-ATGAACCTTACC |
CmeC-PNA5 | KFFKFFKFFK-TATTCATGAACC |
CmeC-PNA6 | KFFKFFKFFK-ATTTTATTCATG |
PNA . | Sequence (N→C) . |
---|---|
CmeA-PNA1 | KFFKFFKFFK-TGCCTTGAAAAA |
CmeA-PNA2 | KFFKFFKFFK-TTTTGCCTTGAA |
CmeA-PNA3 | KFFKFFKFFK-TGGTTTTGCCTT |
CmeA-PNA4 | KFFKFFKFFK-TCATGGTTTTGC |
CmeA-PNA5 | KFFKFFKFFK-ATTTCATGGTTT |
CmeA-PNA6 | KFFKFFKFFK-AATAATTTCATG |
CmeB-PNA1 | KFFKFFKFFK-CTCCAATTTCTT |
CmeB-PNA2 | KFFKFFKFFK-GTGCTCCAATTT |
CmeB-PNA3 | KFFKFFKFFK-ATTGTGCTCCAA |
CmeB-PNA4 | KFFKFFKFFK-ATTATTGTGCTC |
CmeB-PNA5 | KFFKFFKFFK-AAAACATTATTG |
CmeB-PNA6 | KFFKFFKFFK-TTAGAAAACATT |
CmeC-PNA1 | KFFKFFKFFK-ACCTCTTTTTTT |
CmeC-PNA2 | KFFKFFKFFK-TTACCTCTTTTT |
CmeC-PNA3 | KFFKFFKFFK-ACCTTACCTCTT |
CmeC-PNA4 | KFFKFFKFFK-ATGAACCTTACC |
CmeC-PNA5 | KFFKFFKFFK-TATTCATGAACC |
CmeC-PNA6 | KFFKFFKFFK-ATTTTATTCATG |
PNA treatment and western blot analysis
C. jejuni NCTC 11168 was grown overnight on MH agar plates and used to prepare bacterial suspensions in MH broth to an optical density at 600 nm of 0.05–0.07. Broth cultures of C. jejuni were grown in the presence of various concentrations of CmeABC-PNAs for 7 h with shaking at 200 rpm. SDS-PAGE was carried out using a 10% polyacrylamide gel for CmeA in Tris-Tricine buffer and CmeC in Tris-Glycine buffer, and a 6% polyacrylamide gel for CmeB in Tris-Glycine buffer. Western blot analysis was performed using polyclonal antibodies against CmeA, CmeB or CmeC as described previously.6
Determination of C. jejuni susceptibility to ciprofloxacin
The MICs of ciprofloxacin (Enzo Life Sciences, USA) and erythromycin (Sigma, USA) were measured with a microtitre broth dilution method in the presence or absence of CmeABC-PNAs as previously described.9
Results
DNA sequence analysis of translation initiation regions of CmeABC in C. jejuni and Campylobacter coli
PNA-mediated gene knockdown is based on blocking translation initiation in both eukaryotes and prokaryotes.12 The start codon regions, including the ribosome-binding sites (RBSs), are known to be most susceptible to antisense inhibition by PNA in Escherichia coli.13 To design PNAs targeting cmeABC, we first performed a DNA sequence analysis of the translational initiation regions of cmeABC. Since C. jejuni and C. coli are responsible for >95% of human campylobacteriosis, C. jejuni in particular accounting for >92% of cases,14,15 the sequence analysis focused on the two Campylobacter species whose genome sequences are available in the GenBank sequence database. Overall, the translation initiation regions of cmeABC are well conserved in both species (Figure 1). C. jejuni doylei has one nucleotide change from C to T at a point 2 bp upstream of the cmeA start codon (Figure 1a), and C. jejuni ICDCCJ07001 showed a nucleotide change from C to T at a point 7 bp upstream of the cmeB start codon (Figure 1b). However, all other C. jejuni strains showed identical sequences in the translation initiation regions of cmeABC (Figure 1). Relatively high sequence variation was observed between C. jejuni and C. coli in the translation initiation region of cmeA (Figure 1a), suggesting that species-specific PNAs may be required for the effective inhibition of CmeABC.
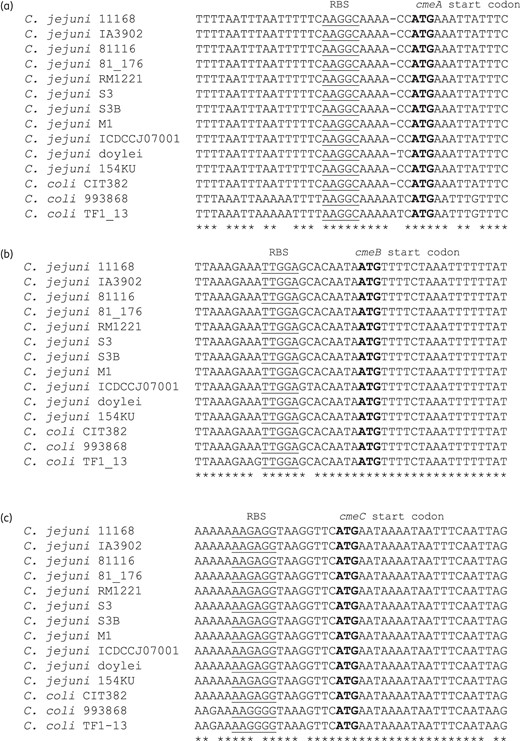
Multiple alignment of the translation initiation regions of cmeA (a), cmeB (b) and cmeC (c). The C. jejuni and C. coli DNA sequences available in the GenBank database were used for the analysis. Start codons are shown in bold and RBSs are underlined. GenBank accession numbers are AL111168 (C. jejuni NCTC 11168), CP001876 (C. jejuni IA3902), CP000814 (C. jejuni 81116), CP000538 (C. jejuni 81_176), NC_003912 (C. jejuni RM1221), CP001960 (C. jejuni S3), FJ797669 (C. jejuni S3B), CP001900 (C. jejuni M1), CP002029 (C. jejuni ICDCCJ07001), CP000768 (C. jejuni doylei 269.97), DQ333454 (C. jejuni 154KU), AY598796 (C. coli CIT382), FJ797671 (C. coli 993868) and FJ797673 (C. coli TF1_13). The alignment was performed using ClustalW2.
Knockdown efficiency of CmeABC-PNAs
Eighteen PNAs were designed to bind to various regions involved in the translation initiation of cmeA, cmeB and cmeC, primarily targeting either the RBS or the start codon (Figure 2). Since it has been reported that, due to cell permeability, a 12-mer PNA exhibits better knockdown efficiency than longer PNAs,11 we also designed 12-mer PNAs in this study. CmeA-PNAs 1, 4 and 5 significantly reduced CmeA expression at 1 μM, and CmeA-PNA1 further inhibited CmeA expression substantially even at 0.5 μM (Figure 3a). Among the three CmeA-PNAs (1, 2 and 3) binding to the RBS of cmeA, CmeA-PNA1 notably inhibited CmeA expression, while the effect of CmeA-PNAs 2 and 3 in CmeA knockdown was not significant (Figure 3a). Three CmeA-PNAs (4, 5 and 6) bind to the start codon of cmeA (Figure 2a). Treatment with 1 μM CmeA-PNAs 4 and 5 resulted in significant reductions in the level of CmeA expression (Figure 3a); however, CmeA-PNA6 had little effect on CmeA expression (Figure 3a). Among the six CmeA-PNAs, CmeA-PNA1 showed the best efficacy in CmeA knockdown (Figure 3a). CmeB-PNAs 1, 2 and 3 bind to the RBS of cmeB (Figure 2b). Compared with CmeB-PNAs 1 and 2, CmeB-PNA3, which binds to the RBS and its downstream region, was most effective in inhibiting CmeB expression (Figure 3b). Interestingly, CmeC expression was not affected by any of the six CmeC-PNAs even at high PNA concentrations, such as 4 μM (Figure 3c). Since CmeA-PNA1 and CmeB-PNA3were most effective in inhibiting CmeA and CmeB, respectively, their knockdown efficiency was further tested by exposing C. jejuni to various concentrations of PNA. CmeA-PNA1 and CmeB-PNA3 demonstrated obvious knockdown efficacy in a concentration-dependent manner (Figure 4).
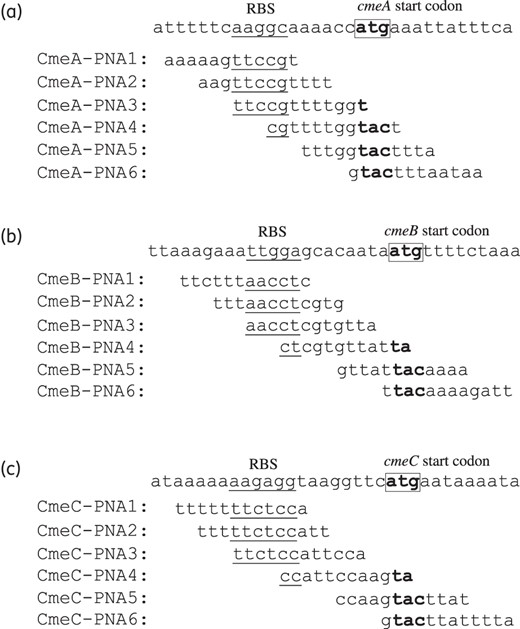
Target sequences of PNAs against cmeA (a), cmeB (b) and cmeC (c). The RBSs are underlined, and the start codon is in bold and marked with a rectangle.
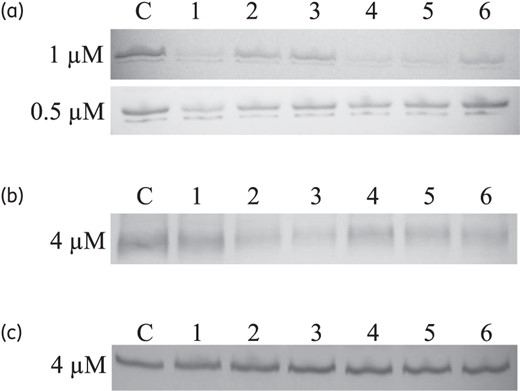
Inhibition efficiency of CmeABC-PNAs. (a) CmeA expression level visualized by western blotting following treatment with six CmeA-PNAs at two different concentrations (1 μM and 0.5 μM), as indicated on the left. CmeA is a glycosylated protein and appears as a doublet band in western blotting. C, C. jejuni NCTC 11168 without PNA; lanes 1–6, CmeA-PNA1–CmeA-PNA6, respectively. (b) Changes in the level of CmeB expression caused by 4 μM CmeB-PNAs. C, C. jejuni NCTC 11168 without PNA; lanes 1–6, CmeB-PNA1–CmeB-PNA6, respectively. (c) Protein levels of CmeC expression following treatment with 4 μM CmeC-PNAs. C, C. jejuni NCTC 11168 without PNA; lanes 1–6, CmeC-PNA1–CmeC-PNA6, respectively. An equal amount of the protein sample was used for each lane, and total proteins were visualized by SDS-PAGE and Coomassie blue staining as a control in all experiments by using the same protein amount used for western blotting (data not shown).
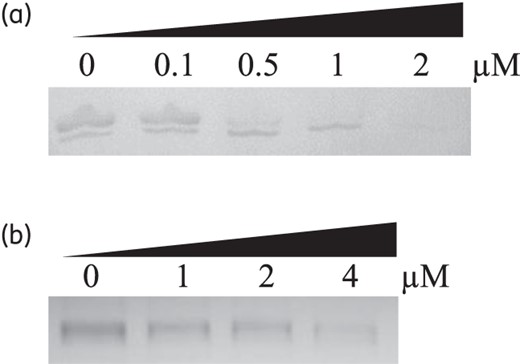
Concentration-dependent knockdown by CmeA-PNA1 (a) and CmeB-PNA3 (b). PNA concentrations are indicated at the top.
C. jejuni susceptibility to ciprofloxacin and erythromycin following treatment with CmeABC-PNAs
To evaluate the effect of CmeABC knockdown on antibiotic susceptibility, we measured the MICs of ciprofloxacin and erythromycin after PNA treatment because they are clinically important and known substrates of the CmeABC efflux pump.3,6 The changes in MIC were proportional to the levels of CmeABC inhibition observed by western blotting. Compared with PNAs targeting CmeB, most CmeA-PNAs rendered C. jejuni highly susceptible to ciprofloxacin and erythromycin (Table 2). In particular, CmeA-PNA1 decreased the MICs of ciprofloxacin and erythromycin 4-fold even at 0.5 μM (Table 2). CmeB-PNAs required higher doses than CmeA-PNAs to achieve similar MIC changes (Table 2). Consistent with the results of western blotting, CmeC-PNAs did not affect the antimicrobial susceptibility of C. jejuni (data not shown).
Changes in the MICs of ciprofloxacin and erythromycin caused by CmeA- and CmeB-PNAs
CmeA-PNAa . | CmeB-PNAa . | ||||||
---|---|---|---|---|---|---|---|
PNAs . | 0.5 μM . | 1 μM . | 2 μM . | PNAs . | 0.5 μM . | 1 μM . | 2 μM . |
Ciprofloxacin | Ciprofloxacin | ||||||
controlb | 0.5 | 0.5 | 0.5 | controlb | 0.5 | 0.5 | 0.5 |
A1 | 0.125 (4) | 0.0625 (8) | 0.0625 (8) | B1 | 0.5 | 0.5 | 0.25 (2) |
A2 | 0.5 | 0.25 (2) | 0.25 (2) | B2 | 0.5 | 0.5 | 0.25 (2) |
A3 | 0.5 | 0.25 (2) | 0.125 (4) | B3 | 0.5 | 0.25 (2) | 0.125 (4) |
A4 | 0.25 (2) | 0.125 (4) | 0.125 (4) | B4 | 0.5 | 0.5 | 0.25 (2) |
A5 | 0.5 | 0.25 (2) | 0.25 (2) | B5 | 0.5 | 0.5 | 0.5 |
A6 | 0.5 | 0.5 | 0.25 (2) | B6 | 0.5 | 0.5 | 0.5 |
Erythromycin | Erythromycin | ||||||
controlb | 0.5 | 0.5 | 0.5 | controlb | 0.5 | 0.5 | 0.5 |
A1 | 0.125 (4) | 0.0313 (16) | 0.0156 (32) | B1 | 0.5 | 0.5 | 0.25 (2) |
A2 | 0.5 | 0.125 (4) | 0.0625 (8) | B2 | 0.5 | 0.5 | 0.25 (2) |
A3 | 0.5 | 0.25 (2) | 0.125 (4) | B3 | 0.5 | 0.25 (2) | 0.25 (2) |
A4 | 0.25 (2) | 0.125 (4) | 0.0625 (8) | B4 | 0.5 | 0.25 (2) | 0.125 (4) |
A5 | 0.5 | 0.25 (2) | 0.125 (4) | B5 | 0.5 | 0.5 | 0.25 (2) |
A6 | 0.5 | 0.5 | 0.0625 (8) | B6 | 0.5 | 0.5 | 0.25 (2) |
CmeA-PNAa . | CmeB-PNAa . | ||||||
---|---|---|---|---|---|---|---|
PNAs . | 0.5 μM . | 1 μM . | 2 μM . | PNAs . | 0.5 μM . | 1 μM . | 2 μM . |
Ciprofloxacin | Ciprofloxacin | ||||||
controlb | 0.5 | 0.5 | 0.5 | controlb | 0.5 | 0.5 | 0.5 |
A1 | 0.125 (4) | 0.0625 (8) | 0.0625 (8) | B1 | 0.5 | 0.5 | 0.25 (2) |
A2 | 0.5 | 0.25 (2) | 0.25 (2) | B2 | 0.5 | 0.5 | 0.25 (2) |
A3 | 0.5 | 0.25 (2) | 0.125 (4) | B3 | 0.5 | 0.25 (2) | 0.125 (4) |
A4 | 0.25 (2) | 0.125 (4) | 0.125 (4) | B4 | 0.5 | 0.5 | 0.25 (2) |
A5 | 0.5 | 0.25 (2) | 0.25 (2) | B5 | 0.5 | 0.5 | 0.5 |
A6 | 0.5 | 0.5 | 0.25 (2) | B6 | 0.5 | 0.5 | 0.5 |
Erythromycin | Erythromycin | ||||||
controlb | 0.5 | 0.5 | 0.5 | controlb | 0.5 | 0.5 | 0.5 |
A1 | 0.125 (4) | 0.0313 (16) | 0.0156 (32) | B1 | 0.5 | 0.5 | 0.25 (2) |
A2 | 0.5 | 0.125 (4) | 0.0625 (8) | B2 | 0.5 | 0.5 | 0.25 (2) |
A3 | 0.5 | 0.25 (2) | 0.125 (4) | B3 | 0.5 | 0.25 (2) | 0.25 (2) |
A4 | 0.25 (2) | 0.125 (4) | 0.0625 (8) | B4 | 0.5 | 0.25 (2) | 0.125 (4) |
A5 | 0.5 | 0.25 (2) | 0.125 (4) | B5 | 0.5 | 0.5 | 0.25 (2) |
A6 | 0.5 | 0.5 | 0.0625 (8) | B6 | 0.5 | 0.5 | 0.25 (2) |
aMICs are expressed in mg/L. Fold changes are indicated in parentheses. The results are representative of three independent experiments that all presented similar results. All the tests were performed with C. jejuni NCTC 11168.
bA negative control tested without PNA.
Changes in the MICs of ciprofloxacin and erythromycin caused by CmeA- and CmeB-PNAs
CmeA-PNAa . | CmeB-PNAa . | ||||||
---|---|---|---|---|---|---|---|
PNAs . | 0.5 μM . | 1 μM . | 2 μM . | PNAs . | 0.5 μM . | 1 μM . | 2 μM . |
Ciprofloxacin | Ciprofloxacin | ||||||
controlb | 0.5 | 0.5 | 0.5 | controlb | 0.5 | 0.5 | 0.5 |
A1 | 0.125 (4) | 0.0625 (8) | 0.0625 (8) | B1 | 0.5 | 0.5 | 0.25 (2) |
A2 | 0.5 | 0.25 (2) | 0.25 (2) | B2 | 0.5 | 0.5 | 0.25 (2) |
A3 | 0.5 | 0.25 (2) | 0.125 (4) | B3 | 0.5 | 0.25 (2) | 0.125 (4) |
A4 | 0.25 (2) | 0.125 (4) | 0.125 (4) | B4 | 0.5 | 0.5 | 0.25 (2) |
A5 | 0.5 | 0.25 (2) | 0.25 (2) | B5 | 0.5 | 0.5 | 0.5 |
A6 | 0.5 | 0.5 | 0.25 (2) | B6 | 0.5 | 0.5 | 0.5 |
Erythromycin | Erythromycin | ||||||
controlb | 0.5 | 0.5 | 0.5 | controlb | 0.5 | 0.5 | 0.5 |
A1 | 0.125 (4) | 0.0313 (16) | 0.0156 (32) | B1 | 0.5 | 0.5 | 0.25 (2) |
A2 | 0.5 | 0.125 (4) | 0.0625 (8) | B2 | 0.5 | 0.5 | 0.25 (2) |
A3 | 0.5 | 0.25 (2) | 0.125 (4) | B3 | 0.5 | 0.25 (2) | 0.25 (2) |
A4 | 0.25 (2) | 0.125 (4) | 0.0625 (8) | B4 | 0.5 | 0.25 (2) | 0.125 (4) |
A5 | 0.5 | 0.25 (2) | 0.125 (4) | B5 | 0.5 | 0.5 | 0.25 (2) |
A6 | 0.5 | 0.5 | 0.0625 (8) | B6 | 0.5 | 0.5 | 0.25 (2) |
CmeA-PNAa . | CmeB-PNAa . | ||||||
---|---|---|---|---|---|---|---|
PNAs . | 0.5 μM . | 1 μM . | 2 μM . | PNAs . | 0.5 μM . | 1 μM . | 2 μM . |
Ciprofloxacin | Ciprofloxacin | ||||||
controlb | 0.5 | 0.5 | 0.5 | controlb | 0.5 | 0.5 | 0.5 |
A1 | 0.125 (4) | 0.0625 (8) | 0.0625 (8) | B1 | 0.5 | 0.5 | 0.25 (2) |
A2 | 0.5 | 0.25 (2) | 0.25 (2) | B2 | 0.5 | 0.5 | 0.25 (2) |
A3 | 0.5 | 0.25 (2) | 0.125 (4) | B3 | 0.5 | 0.25 (2) | 0.125 (4) |
A4 | 0.25 (2) | 0.125 (4) | 0.125 (4) | B4 | 0.5 | 0.5 | 0.25 (2) |
A5 | 0.5 | 0.25 (2) | 0.25 (2) | B5 | 0.5 | 0.5 | 0.5 |
A6 | 0.5 | 0.5 | 0.25 (2) | B6 | 0.5 | 0.5 | 0.5 |
Erythromycin | Erythromycin | ||||||
controlb | 0.5 | 0.5 | 0.5 | controlb | 0.5 | 0.5 | 0.5 |
A1 | 0.125 (4) | 0.0313 (16) | 0.0156 (32) | B1 | 0.5 | 0.5 | 0.25 (2) |
A2 | 0.5 | 0.125 (4) | 0.0625 (8) | B2 | 0.5 | 0.5 | 0.25 (2) |
A3 | 0.5 | 0.25 (2) | 0.125 (4) | B3 | 0.5 | 0.25 (2) | 0.25 (2) |
A4 | 0.25 (2) | 0.125 (4) | 0.0625 (8) | B4 | 0.5 | 0.25 (2) | 0.125 (4) |
A5 | 0.5 | 0.25 (2) | 0.125 (4) | B5 | 0.5 | 0.5 | 0.25 (2) |
A6 | 0.5 | 0.5 | 0.0625 (8) | B6 | 0.5 | 0.5 | 0.25 (2) |
aMICs are expressed in mg/L. Fold changes are indicated in parentheses. The results are representative of three independent experiments that all presented similar results. All the tests were performed with C. jejuni NCTC 11168.
bA negative control tested without PNA.
Discussion
In this study, we optimized the antisense inhibition of CmeABC by using 18 PNAs targeting the translation initiation regions of cmeABC. The cmeA gene is the first gene in the cmeABC operon and was the best target for the PNA-mediated knockdown of CmeABC (Figure 3 and Table 2). Interestingly, CmeC expression was not altered by antisense PNA even at high doses (Figure 3c), although CmeC-PNAs were extensively designed to span various regions associated with translational initiation (Figure 2c). Treatment with antisense PNAs targeting CmeA and CmeB substantially increased the susceptibility of C. jejuni to ciprofloxacin and erythromycin (Table 2). Consistent with the observations from the western blot analysis, most CmeA-PNAs significantly reduced the MIC levels (Table 2).
The inhibition efficiency of antisense PNA targeting the cmeABC operon was highest for cmeA, moderate for cmeB, and low for cmeC, suggesting that the gene organization in the cmeABC operon affects the knockdown efficiency. Similarly, Dryselius et al.16 reported that the upstream lacZ was more strongly inhibited by PNA than were the downstream lacY and lacA proteins in the lac operon of E. coli. Based on our findings and the previous report, upstream genes appear to be most susceptible to PNA-mediated antisense inhibition in a polycistronic operon.
In addition, the unresponsiveness of CmeC to antisense PNA might possibly be related to the unique organization of cmeABC in the operon. The cmeABC genes constitute a polycistronic operon, in which cmeB and cmeC overlap by 8 bp and the start codon of cmeC is embedded in the coding region of cmeB.6 The tight binding of PNA to mRNA disrupts the function of the ribosomes and interferes with translation, resulting in gene knockdown.17 While translation initiation regions are susceptible to PNA inhibition, PNA binding to a coding region does not result in knockdown effects in E. coli.13 The start codon and RBS of cmeC are located in the coding region of cmeB as the start codon of cmeC is 5 bp upstream of the stop codon of cmeB.6 Although CmeC-PNAs bind to the translation initiation regions of cmeC, which is located within the coding region of cmeB, in this case ribosomes translating cmeB may dissociate CmeC-PNAs from mRNA. This possibility is high because Dryselius et al.16 observed a fully discoordinated reduction in a gfp-DsRed operon that was artificially constructed placing RBS in an intergenic region between the stop codon of gfp and the start codon of DsRed. In their artificial operon construct, antisense PNAs directed at gfp or DsRed exhibited discoordinated inhibitory effects on both upstream and downstream genes.16
The results of western blotting demonstrated that the RBS is the major target for the PNA-mediated knockdown of CmeA and CmeB (Figure 3). However, it should be noted that the sequence in the vicinity around the RBS is also important in determining the knockdown efficiency of PNA. Although CmeA-PNA2, CmeA-PNA3 and CmeB-PNA1 harbour the whole sequence of the RBS (Figure 2a and b), their knockdown efficiency was very low (Figure 3a and b). These results suggest that targeting the RBS sequence alone is not sufficient for achieving the best knockdown efficiency and that both RBS and the sequence in its vicinity play a critical role in determining the knockdown efficacy of antisense PNA. The start codon of cmeA, but not of cmeB and cmeC, was susceptible to antisense PNA (Figure 3). Among the three CmeA-PNAs (4, 5 and 6) harbouring the cmeA start codon, only CmeA-PNAs 4 and 5 significantly reduced the level of CmeA expression, while the antisense effect of CmeA-PNA6 was small (Figure 3a). Similar to the case of targeting the RBS, the results suggest that both the start codon and its nearby sequence affect the knockdown efficiency.
In this study, we demonstrated that the target optimization of antisense PNA for CmeABC knockdown significantly affects the effectiveness of sensitizing C. jejuni to ciprofloxacin and erythromycin. Although the translation initiation regions are the known major target for antisense PNA, the knockdown efficiency of antisense PNA is still dependent on other unknown factors. Presumably, the secondary structure of mRNA may be associated with the inhibition mechanism of antisense PNA, which will be examined in future investigations.
Funding
This study was supported by the National Institutes of Health (1R21AI098742-01). The funder had no role in study design, data collection and analysis, decision to publish, or preparation of the manuscript.
Transparency declarations
None to declare.
Acknowledgements
We thank Dr Lynn McMullen and Dr Michael Gaenzle (University of Alberta) for sharing their laboratory facilities.