-
PDF
- Split View
-
Views
-
Cite
Cite
Pierre-Antoine Dugué, Allison M Hodge, Ee Ming Wong, JiHoon E Joo, Chol-Hee Jung, John L Hopper, Dallas R English, Graham G Giles, Roger L Milne, Melissa C Southey, Methylation marks of prenatal exposure to maternal smoking and risk of cancer in adulthood, International Journal of Epidemiology, Volume 50, Issue 1, February 2021, Pages 105–115, https://doi.org/10.1093/ije/dyaa210
- Share Icon Share
Abstract
Prenatal exposure to maternal smoking is detrimental to child health but its association with risk of cancer has seldom been investigated. Maternal smoking induces widespread and long-lasting DNA methylation changes, which we study here for association with risk of cancer in adulthood.
Eight prospective case–control studies nested within the Melbourne Collaborative Cohort Study were used to assess associations between maternal-smoking-associated methylation marks in blood and risk of several cancers: breast (n = 406 cases), colorectal (n = 814), gastric (n = 166), kidney (n = 139), lung (n = 327), prostate (n = 847) and urothelial (n = 404) cancer and B-cell lymphoma (n = 426). We used conditional logistic regression models to estimate odds ratios (ORs) and 95% confidence intervals (CIs) for associations between cancer and five methylation scores calculated as weighted averages for 568, 19, 15, 28 and 17 CpG sites. Models were adjusted for confounders, including personal smoking history (smoking status, pack-years, age at starting and quitting) and methylation scores for personal smoking.
All methylation scores for maternal smoking were strongly positively associated with risk of urothelial cancer. Risk estimates were only slightly attenuated after adjustment for smoking history, other potential confounders and methylation scores for personal smoking. Potential negative associations were observed with risk of lung cancer and B-cell lymphoma. No associations were observed for other cancers.
We found that methylation marks of prenatal exposure to maternal smoking are associated with increased risk of urothelial cancer. Our study demonstrates the potential for using DNA methylation to investigate the impact of early-life, unmeasured exposures on later-life cancer risk.
Prenatal exposure to maternal smoking is detrimental to child health but its association with risk of cancer has seldom been investigated.
As maternal smoking induces widespread and long-lasting DNA methylation changes, we used methylation scores to study the association between prenatal exposure to maternal smoking and risk for eight adult cancer types, using a prospective design.
We observed a strong positive association with risk of urothelial cancer, which was robust to adjustment by questionnaire-collected smoking variables and several methylation scores for smoking in adulthood.
Our study demonstrates the potential for using DNA methylation to investigate the impact of early-life, unmeasured exposures on later-life cancer risk.
Introduction
In utero exposure to maternal smoking is detrimental to child health. In addition to adverse pregnancy and birth outcomes, it is associated with neurobehavioural disorders, reduced lung function, and metabolic conditions such as high blood pressure and obesity,1,2 but few studies have examined possible influences of maternal smoking on cancer. Some studies have examined risks for childhood cancer but these have generally been based on small samples and have not provided convincing evidence that maternal smoking is associated with increased risk.3–12 Although there are plausible mechanisms by which maternal smoking may increase the risk of cancer later in life,13–15 this hypothesis has seldom been investigated, possibly because most mature cohort studies have recruited adult participants and have not captured information on maternal smoking status. To our knowledge, very few sufficiently powered studies have assessed the association between prenatal exposure to maternal smoking and adult cancer;16 some studies have assessed the risk of breast cancer and concluded that there was no association.17 We know of no cohort study comprising mothers and their offspring (in which information on maternal smoking is likely to be accurate) with long enough follow-up and sufficient sample size to investigate the association between maternal smoking and risk of cancer in adulthood.
Recent studies have shown that prenatal exposure to maternal smoking is associated with widespread changes in DNA methylation in the blood of newborns.18–34 In the large-scale meta-analysis by Joubert et al., sustained maternal smoking during pregnancy was found to be associated with DNA methylation in the blood of newborns at over 6000 CpG sites, and a majority of these methylation changes persisted in older children.30 The study by Richmond et al. showed that associations persisted in adults (mean age 30 years), independent of personal exposure to smoking, and proposed a methylation score to serve as an enduring marker of prenatal smoke exposure.33 Tehranifar et al. also reported that exposure to maternal smoking was associated with methylation changes in the blood of adults (of mean age 43 years), independent of personal exposure to smoking.32 A methylation-based predictor of maternal smoking developed by Reese et al. was shown to be highly accurate to detect this exposure in the blood of newborns.31 A complication when studying methylation changes associated with prenatal exposure to maternal smoking is that affected CpG sites overlap with those affected by smoking in adulthood.30,34 Although studies of midlife methylation changes due to maternal smoking controlled for personal smoking history in their analysis,32,33 effects of smoking on the methylome are pervasive, with a strong possibility of residual confounding,35 and observed smoking-associated methylation patterns may vary substantially from one study to another.36 Good control for smoking history in adulthood is therefore paramount when studying the potential association between a methylation-based marker of prenatal smoke exposure and adult cancer.
In this study, we aimed to assess the association between five methylation-based predictors of maternal smoking derived from the aforementioned four studies30–33 and the risk of cancer in adulthood. We used data from eight prospective case–control studies nested within the Melbourne Collaborative Cohort Study (MCCS), including 3625 cases of breast, colorectal, gastric, kidney, lung, prostate and urothelial cancers and B-cell lymphoma.
Materials and methods
Study sample and blood collection
We used data collected from participants in the MCCS, a prospective study of 41 513 healthy adult volunteers (24 469 women) aged between 27 and 76 years (99.3% aged 40–69 years) when recruited between 1990 and 1994. More details on the cohort are available elsewhere.37,38 DNA was extracted from peripheral blood taken at the time of recruitment (1990–94) except for 151 case–control pairs of the urothelial cancer study for which blood samples were taken at a follow-up visit in 2003–07. The DNA source was dried blood spots, peripheral blood mononuclear cells or buffy coats for 70, 28 and 2% of participants, respectively. More details can be found elsewhere.39
Cancer and death ascertainment
Case–control studies of DNA methylation and risk of breast, colorectal, gastric, kidney, lung, mature B-cell lymphoma, prostate and urothelial cancer nested within the MCCS were conducted.40–44 These cancers accounted for ∼60% of new cancer cases in Victoria in 2018.45 Participants were cancer-free at blood draw and incident cancer cases were identified by linkage with the Victorian Cancer Registry and the Australian Cancer Database (Australian Institute of Health and Welfare). Cases were followed for inclusion until 2012–13 so the follow-up time was up to 23 years. Median time-to-diagnosis was 9.4 years (interquartile range: 5.2–13.3); these figures for individual cancer types are shown in Supplementary Table S1, available as Supplementary data at IJE online. For each nested case–control study, controls were individually matched to incident cases (diagnosed after blood sample collection) on age using incidence density sampling (i.e. they had to be free of the cancer of interest up to the age at diagnosis of the corresponding case), sex, country of birth (Australia/New-Zealand, Southern Europe, Northern Europe), blood DNA source (dried blood spots, peripheral blood mononuclear cells or buffy coat) and blood collection period (baseline or wave 2). Controls were also matched to cases on year of birth, except for the colorectal cancer study where controls were matched on year of baseline attendance. For the lung cancer study, controls were also matched on smoking status at the time of blood collection.
DNA extraction and bisulfite conversion, and DNA methylation data processing
Methods relating to DNA extraction and bisulfite conversion, and DNA methylation data processing have been described previously46 and are detailed in Supplementary Methods, available as Supplementary data at IJE online.
Methylation scores for maternal smoking
We calculated the following five scores, all as weighted averages of methylation beta values.
MS-568: score comprising 568 CpGs at which methylation was associated (Bonferroni P < 1.08 x 10–7) with maternal smoking in the blood of newborns;30,33 MS-19: score comprising 19 CpGs at which methylation was associated (Bonferroni P < 1.08 x 10−7) with maternal smoking in the blood of older children;30,33 MS-15: score comprising 15 CpGs at which methylation was found to be associated (FDR-adjusted P < 0.05) with maternal smoking in the blood of adults of mean age 30 years;33 MS-28: score comprising 28 CpGs resulting from the logistic Lasso regression applied to predict maternal smoking in the blood of newborns;31 MS-17: score comprising 17 CpGs at which methylation was found to be associated (FDR-adjusted P < 0.05) with maternal smoking in the blood of adults of mean age 43 years.32
The predictive ability of these scores with respect to detecting prenatal exposure to maternal smoking was reported to be good to excellent in independent samples with area under the receiver operating characteristic curve (AUC) of 0.69, 0.72 and 0.90 for MS-568, MS-19 and MS-28, respectively.31,33 The predictive ability of MS-15 and MS-17 was not evaluated.32
Methylation scores for smoking in adulthood
We also calculated scores for smoking in adulthood to control for residual confounding, i.e. reflecting different sensitivity to smoking exposure, past exposures or imperfect questionnaire-based measurement. AS-233: score comprising 233 CpGs resulting from the Lasso regression applied to predict smoking pack-years (log-transformed), using as weights the regression coefficients available from the original publication;47 AS-56: score comprising 56 CpGs resulting from the linear Lasso regression applied to predict a comprehensive smoking index in the MCCS36 (see Supplementary Methods); AS-1061: score comprising 1061 CpGs associated with the comprehensive smoking index (P < 10−7) and reported to be associated with smoking in previous studies.36
Statistical analysis
All methylation scores were rescaled to Z-scores. Correlations between methylation scores were calculated using Spearman correlations in participants who served as controls. We used conditional logistic regression to estimate odds ratios (ORs) for the association between methylation scores and the risk of cancer using the following sequential adjustments: in Model 1, no covariates were included other than blood cell composition;48,49 Model 2, further adjusted for smoking status (never; former; current), body mass index (BMI; continuous, in kg/m2) and alcohol consumption (continuous, in g/day); Model 3, further adjusted for smoking pack-years, age at starting (never smoked; ≤16 years; 17–21 years; >21 years), years since quitting (never smoked; >10 years without smoking, 5 and 10 years without smoking; <5 years without smoking), a score for physical activity,50 the alternate healthy eating index to reflect overall diet quality,51 education (score ranging from 1 to 8), socio-economic status (score ranging from 1 to 1052) and height (continuous, in meters); in Model 4, we additionally adjusted for the methylation score for smoking in adulthood AS-233; in Model 5, we additionally adjusted for AS-56. Results with additional adjustment for AS-1061 instead of, or in addition to AS-56, were virtually the same as those without, so are not presented. These models were used to analyse each cancer separately and all eight cancer types combined. For the combined analysis, where an individual was diagnosed with several cancers, we included the first diagnosis only (respecting the incidence density sampling procedure), so that participants did not contribute twice to the pooled estimates. Case–control pairs with any missing values for the confounders measured at baseline were excluded and missing values at follow-up (urothelial cancers) were replaced by baseline values; 3% of the initial sample was excluded due to missing values. Sensitivity analyses were undertaken to assess the robustness of our findings by conducting the same analyses: (i) by sample type (dried blood spots/peripheral blood mononuclear cells/buffy coats), (ii) by sample collection period (1990–94/2003–07) and (iii) restricted to never smokers. In secondary analyses, we assessed the association between methylation scores for maternal smoking and the following cancer subtypes: oestrogen-receptor positive/negative breast cancer;53 colon/rectal cancer; B-cell lymphoma subtypes: multiple myeloma/follicular lymphoma/low-grade non-Hodgkin’s lymphoma, chronic lymphocytic leukaemia/non-Hodgkin’s lymphoma,44 aggressive/non-aggressive prostate cancer42 and invasive/superficial urothelial cancers.41
Results
The characteristics of the study sample are shown in Table 1. Correlations between smoking scores are shown in Table 2: these were strong between MS-568, MS-19, MS-15 and MS-17 ranging from 0.57 to 0.87, moderate with MS-28 and equal to 0.31, 0.34, 0.39 and 0.44 for MS-568, MS-19, MS-15 and MS-17, respectively. Correlations with scores for smoking in adulthood were weak to moderate for MS-568, MS-19 and MS-15, ranging from 0.20 to 0.36, and moderate for MS-28 and MS-17, ranging from 0.34 to 0.55. Correlations between adult smoking scores were strong, ranging from 0.61 to 0.88.
Characteristics of the study sample, eight case–control studies nested within the MCCS (n = 41 513)
. | Controls . | Cases . |
---|---|---|
Cancer type (n) | ||
Breast cancer | 406 | 406 |
Colorectal cancer | 814 | 814 |
Gastric cancer | 166 | 166 |
Kidney cancer | 139 | 139 |
Lung cancer | 327 | 327 |
B-cell lymphoma | 426 | 426 |
Prostate cancer | 847 | 847 |
Urothelial cancers | 404 | 404 |
Matching variables | ||
Age at blood draw, median (IQR) | 61 (54–66) | 61 (54–66) |
Sex: male, n (%) | 2163 (61%) | 2163 (61%) |
Sex: female, n (%) | 1366 (39%) | 1366 (39%) |
Country of birth: Australia/NZ, n (%) | 2400 (68%) | 2416 (68%) |
Northern Europe, n (%) | 237 (7%) | 230 (7%) |
Southern Europe, n (%) | 892 (25%) | 883 (25%) |
Blood sample type: dried blood spots, n (%) | 2463 (70%) | 2463 (70%) |
Peripheral blood mononuclear cells, n (%) | 877 (25%) | 877 (25%) |
Buffy coats, n (%) | 189 (5%) | 189 (5%) |
Potential confounders | ||
Smoking: current, n (%) | 485 (14%) | 406 (15%) |
Smoking: former, n (%) | 1345 (38%) | 1392 (39%) |
Smoking: never, n (%) | 1699 (48%) | 1625 (46%) |
Smoking pack-years, median (IQR) | 0.6 (0–25) | 1.9 (0–29) |
Age at starting: never smoked, n (%) | 1699 (48%) | 1625 (46%) |
Age at starting: <17 years, n (%) | 682 (19%) | 697 (20%) |
Age at starting: 17–21 years, n (%) | 815 (23%) | 899 (25%) |
Age at starting: >21 years, n (%) | 333 (9%) | 308 (9%) |
Time since quitting: never smoked, n (%) | 1699 (48%) | 1625 (46%) |
Time since quitting: <5 years, n (%) | 672 (19%) | 725 (21%) |
Time since quitting: 5–10 years, n (%) | 932 (26%) | 938 (27%) |
Time since quitting: >10 years, n (%) | 226 (6%) | 241 (7%) |
Body mass index (kg/m2), median (IQR) | 27 (24–29) | 27 (24–30) |
Height (m), median (IQR) | 167 (160–174) | 167 (161–174) |
Alcohol consumption (g/day), median (IQR) | 4 (0–17) | 4 (0–18) |
Diet quality: AHEI-2010, median (IQR) | 64 (56–72) | 64 (56–73) |
Physical activity score, median (IQR) | 2 (1–4) | 2 (1–4) |
Education score, median (IQR) | 4 (4–6) | 4 (4–6) |
Socioeconomic status: SEIFA-10, median (IQR) | 5 (3–8) | 6 (3–9) |
. | Controls . | Cases . |
---|---|---|
Cancer type (n) | ||
Breast cancer | 406 | 406 |
Colorectal cancer | 814 | 814 |
Gastric cancer | 166 | 166 |
Kidney cancer | 139 | 139 |
Lung cancer | 327 | 327 |
B-cell lymphoma | 426 | 426 |
Prostate cancer | 847 | 847 |
Urothelial cancers | 404 | 404 |
Matching variables | ||
Age at blood draw, median (IQR) | 61 (54–66) | 61 (54–66) |
Sex: male, n (%) | 2163 (61%) | 2163 (61%) |
Sex: female, n (%) | 1366 (39%) | 1366 (39%) |
Country of birth: Australia/NZ, n (%) | 2400 (68%) | 2416 (68%) |
Northern Europe, n (%) | 237 (7%) | 230 (7%) |
Southern Europe, n (%) | 892 (25%) | 883 (25%) |
Blood sample type: dried blood spots, n (%) | 2463 (70%) | 2463 (70%) |
Peripheral blood mononuclear cells, n (%) | 877 (25%) | 877 (25%) |
Buffy coats, n (%) | 189 (5%) | 189 (5%) |
Potential confounders | ||
Smoking: current, n (%) | 485 (14%) | 406 (15%) |
Smoking: former, n (%) | 1345 (38%) | 1392 (39%) |
Smoking: never, n (%) | 1699 (48%) | 1625 (46%) |
Smoking pack-years, median (IQR) | 0.6 (0–25) | 1.9 (0–29) |
Age at starting: never smoked, n (%) | 1699 (48%) | 1625 (46%) |
Age at starting: <17 years, n (%) | 682 (19%) | 697 (20%) |
Age at starting: 17–21 years, n (%) | 815 (23%) | 899 (25%) |
Age at starting: >21 years, n (%) | 333 (9%) | 308 (9%) |
Time since quitting: never smoked, n (%) | 1699 (48%) | 1625 (46%) |
Time since quitting: <5 years, n (%) | 672 (19%) | 725 (21%) |
Time since quitting: 5–10 years, n (%) | 932 (26%) | 938 (27%) |
Time since quitting: >10 years, n (%) | 226 (6%) | 241 (7%) |
Body mass index (kg/m2), median (IQR) | 27 (24–29) | 27 (24–30) |
Height (m), median (IQR) | 167 (160–174) | 167 (161–174) |
Alcohol consumption (g/day), median (IQR) | 4 (0–17) | 4 (0–18) |
Diet quality: AHEI-2010, median (IQR) | 64 (56–72) | 64 (56–73) |
Physical activity score, median (IQR) | 2 (1–4) | 2 (1–4) |
Education score, median (IQR) | 4 (4–6) | 4 (4–6) |
Socioeconomic status: SEIFA-10, median (IQR) | 5 (3–8) | 6 (3–9) |
Characteristics of the study sample, eight case–control studies nested within the MCCS (n = 41 513)
. | Controls . | Cases . |
---|---|---|
Cancer type (n) | ||
Breast cancer | 406 | 406 |
Colorectal cancer | 814 | 814 |
Gastric cancer | 166 | 166 |
Kidney cancer | 139 | 139 |
Lung cancer | 327 | 327 |
B-cell lymphoma | 426 | 426 |
Prostate cancer | 847 | 847 |
Urothelial cancers | 404 | 404 |
Matching variables | ||
Age at blood draw, median (IQR) | 61 (54–66) | 61 (54–66) |
Sex: male, n (%) | 2163 (61%) | 2163 (61%) |
Sex: female, n (%) | 1366 (39%) | 1366 (39%) |
Country of birth: Australia/NZ, n (%) | 2400 (68%) | 2416 (68%) |
Northern Europe, n (%) | 237 (7%) | 230 (7%) |
Southern Europe, n (%) | 892 (25%) | 883 (25%) |
Blood sample type: dried blood spots, n (%) | 2463 (70%) | 2463 (70%) |
Peripheral blood mononuclear cells, n (%) | 877 (25%) | 877 (25%) |
Buffy coats, n (%) | 189 (5%) | 189 (5%) |
Potential confounders | ||
Smoking: current, n (%) | 485 (14%) | 406 (15%) |
Smoking: former, n (%) | 1345 (38%) | 1392 (39%) |
Smoking: never, n (%) | 1699 (48%) | 1625 (46%) |
Smoking pack-years, median (IQR) | 0.6 (0–25) | 1.9 (0–29) |
Age at starting: never smoked, n (%) | 1699 (48%) | 1625 (46%) |
Age at starting: <17 years, n (%) | 682 (19%) | 697 (20%) |
Age at starting: 17–21 years, n (%) | 815 (23%) | 899 (25%) |
Age at starting: >21 years, n (%) | 333 (9%) | 308 (9%) |
Time since quitting: never smoked, n (%) | 1699 (48%) | 1625 (46%) |
Time since quitting: <5 years, n (%) | 672 (19%) | 725 (21%) |
Time since quitting: 5–10 years, n (%) | 932 (26%) | 938 (27%) |
Time since quitting: >10 years, n (%) | 226 (6%) | 241 (7%) |
Body mass index (kg/m2), median (IQR) | 27 (24–29) | 27 (24–30) |
Height (m), median (IQR) | 167 (160–174) | 167 (161–174) |
Alcohol consumption (g/day), median (IQR) | 4 (0–17) | 4 (0–18) |
Diet quality: AHEI-2010, median (IQR) | 64 (56–72) | 64 (56–73) |
Physical activity score, median (IQR) | 2 (1–4) | 2 (1–4) |
Education score, median (IQR) | 4 (4–6) | 4 (4–6) |
Socioeconomic status: SEIFA-10, median (IQR) | 5 (3–8) | 6 (3–9) |
. | Controls . | Cases . |
---|---|---|
Cancer type (n) | ||
Breast cancer | 406 | 406 |
Colorectal cancer | 814 | 814 |
Gastric cancer | 166 | 166 |
Kidney cancer | 139 | 139 |
Lung cancer | 327 | 327 |
B-cell lymphoma | 426 | 426 |
Prostate cancer | 847 | 847 |
Urothelial cancers | 404 | 404 |
Matching variables | ||
Age at blood draw, median (IQR) | 61 (54–66) | 61 (54–66) |
Sex: male, n (%) | 2163 (61%) | 2163 (61%) |
Sex: female, n (%) | 1366 (39%) | 1366 (39%) |
Country of birth: Australia/NZ, n (%) | 2400 (68%) | 2416 (68%) |
Northern Europe, n (%) | 237 (7%) | 230 (7%) |
Southern Europe, n (%) | 892 (25%) | 883 (25%) |
Blood sample type: dried blood spots, n (%) | 2463 (70%) | 2463 (70%) |
Peripheral blood mononuclear cells, n (%) | 877 (25%) | 877 (25%) |
Buffy coats, n (%) | 189 (5%) | 189 (5%) |
Potential confounders | ||
Smoking: current, n (%) | 485 (14%) | 406 (15%) |
Smoking: former, n (%) | 1345 (38%) | 1392 (39%) |
Smoking: never, n (%) | 1699 (48%) | 1625 (46%) |
Smoking pack-years, median (IQR) | 0.6 (0–25) | 1.9 (0–29) |
Age at starting: never smoked, n (%) | 1699 (48%) | 1625 (46%) |
Age at starting: <17 years, n (%) | 682 (19%) | 697 (20%) |
Age at starting: 17–21 years, n (%) | 815 (23%) | 899 (25%) |
Age at starting: >21 years, n (%) | 333 (9%) | 308 (9%) |
Time since quitting: never smoked, n (%) | 1699 (48%) | 1625 (46%) |
Time since quitting: <5 years, n (%) | 672 (19%) | 725 (21%) |
Time since quitting: 5–10 years, n (%) | 932 (26%) | 938 (27%) |
Time since quitting: >10 years, n (%) | 226 (6%) | 241 (7%) |
Body mass index (kg/m2), median (IQR) | 27 (24–29) | 27 (24–30) |
Height (m), median (IQR) | 167 (160–174) | 167 (161–174) |
Alcohol consumption (g/day), median (IQR) | 4 (0–17) | 4 (0–18) |
Diet quality: AHEI-2010, median (IQR) | 64 (56–72) | 64 (56–73) |
Physical activity score, median (IQR) | 2 (1–4) | 2 (1–4) |
Education score, median (IQR) | 4 (4–6) | 4 (4–6) |
Socioeconomic status: SEIFA-10, median (IQR) | 5 (3–8) | 6 (3–9) |
Spearman rank correlations (and number of overlapping CpG sites) for five methylation scores for maternal smoking and three scores for smoking in adulthood
. | MS-19 . | MS-15 . | MS-28 . | MS-17 . | AS-233 . | AS-56 . | AS-1,061 . |
---|---|---|---|---|---|---|---|
MS-568 | 0.71 (19) | 0.57 (11) | 0.31 (13) | 0.77 (17) | 0.29 (3) | 0.29 (10) | 0.36 (83) |
MS-19 | 0.76 (8) | 0.34 (5) | 0.87 (8) | 0.20 (2) | 0.20 (4) | 0.21 (10) | |
MS-15 | 0.39 (3) | 0.74 (5) | 0.34 (0) | 0.30 (2) | 0.23 (7) | ||
MS-28 | 0.44 (4) | 0.53 (1) | 0.55 (1) | 0.34 (7) | |||
MS-17 | 0.41 (1) | 0.41 (2) | 0.41 (10) | ||||
AS-233 | 0.88 (7) | 0.61 (17) | |||||
AS-56 | 0.61 (43) |
. | MS-19 . | MS-15 . | MS-28 . | MS-17 . | AS-233 . | AS-56 . | AS-1,061 . |
---|---|---|---|---|---|---|---|
MS-568 | 0.71 (19) | 0.57 (11) | 0.31 (13) | 0.77 (17) | 0.29 (3) | 0.29 (10) | 0.36 (83) |
MS-19 | 0.76 (8) | 0.34 (5) | 0.87 (8) | 0.20 (2) | 0.20 (4) | 0.21 (10) | |
MS-15 | 0.39 (3) | 0.74 (5) | 0.34 (0) | 0.30 (2) | 0.23 (7) | ||
MS-28 | 0.44 (4) | 0.53 (1) | 0.55 (1) | 0.34 (7) | |||
MS-17 | 0.41 (1) | 0.41 (2) | 0.41 (10) | ||||
AS-233 | 0.88 (7) | 0.61 (17) | |||||
AS-56 | 0.61 (43) |
Spearman rank correlations (and number of overlapping CpG sites) for five methylation scores for maternal smoking and three scores for smoking in adulthood
. | MS-19 . | MS-15 . | MS-28 . | MS-17 . | AS-233 . | AS-56 . | AS-1,061 . |
---|---|---|---|---|---|---|---|
MS-568 | 0.71 (19) | 0.57 (11) | 0.31 (13) | 0.77 (17) | 0.29 (3) | 0.29 (10) | 0.36 (83) |
MS-19 | 0.76 (8) | 0.34 (5) | 0.87 (8) | 0.20 (2) | 0.20 (4) | 0.21 (10) | |
MS-15 | 0.39 (3) | 0.74 (5) | 0.34 (0) | 0.30 (2) | 0.23 (7) | ||
MS-28 | 0.44 (4) | 0.53 (1) | 0.55 (1) | 0.34 (7) | |||
MS-17 | 0.41 (1) | 0.41 (2) | 0.41 (10) | ||||
AS-233 | 0.88 (7) | 0.61 (17) | |||||
AS-56 | 0.61 (43) |
. | MS-19 . | MS-15 . | MS-28 . | MS-17 . | AS-233 . | AS-56 . | AS-1,061 . |
---|---|---|---|---|---|---|---|
MS-568 | 0.71 (19) | 0.57 (11) | 0.31 (13) | 0.77 (17) | 0.29 (3) | 0.29 (10) | 0.36 (83) |
MS-19 | 0.76 (8) | 0.34 (5) | 0.87 (8) | 0.20 (2) | 0.20 (4) | 0.21 (10) | |
MS-15 | 0.39 (3) | 0.74 (5) | 0.34 (0) | 0.30 (2) | 0.23 (7) | ||
MS-28 | 0.44 (4) | 0.53 (1) | 0.55 (1) | 0.34 (7) | |||
MS-17 | 0.41 (1) | 0.41 (2) | 0.41 (10) | ||||
AS-233 | 0.88 (7) | 0.61 (17) | |||||
AS-56 | 0.61 (43) |
Associations of the five maternal smoking methylation scores with risk of cancer after adjustment for smoking status, BMI and alcohol consumption (Model 2) are shown in Figure 1 and Supplementary Table S2, available as Supplementary data at IJE online. There was no evidence of association with risk of breast, colorectal, gastric, kidney or prostate cancer. There was weak evidence of association with decreased B-cell lymphoma risk for MS-15 [per Z-score unit: OR = 0.83, 95% confidence interval (CI) = 0.69–0.98]. We observed an association between MS-28 and the risk of lung cancer (OR = 1.28, 95%CI = 1.06–1.54). All five scores were strongly associated with risk of urothelial cancer: MS-568: per Z-score unit: OR = 1.34, 95%CI = 1.11–1.61; MS-19: OR = 1.19, 95%CI = 1.01–1.40; MS-15: OR = 1.38, 95%CI = 1.16–1.64; MS-28: OR = 1.33, 95%CI = 1.09–1.61; MS-17: OR = 1.31, 95%CI = 1.10–1.56. These corresponded to ORs comparing the highest with the lowest quartile of methylation scores of 2.11, 1.72, 2.50, 2.63 and 2.10 for MS-568, MS-19, MS-15, MS-28 and MS-17, respectively (not shown).
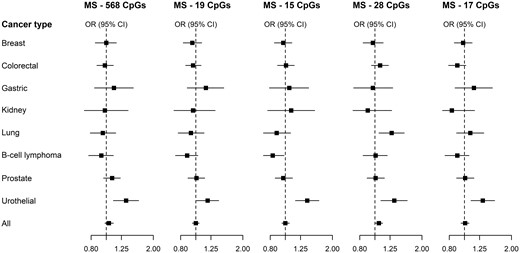
All cancers: ORs and 95% CIs for the association between five methylation scores for maternal smoking and risk of eight types of cancer in the MCCS. ORs were calculated using conditional logistic regression models, adjusting for white blood cell composition, smoking status (current/former/never), alcohol consumption (in g/day) and body mass index (in kg/m2). Cases and controls were matched on age, sex, country of birth, sample type (and smoking for the lung cancer study), and placed consecutively on the same chip of the assay
Results from analyses testing the robustness of these findings after more stringent control for confounding are shown in Figure 2 and Supplementary Table S3, available as Supplementary data at IJE online. For all maternal smoking methylation scores, the associations with risk of urothelial cancer were only slightly attenuated after more comprehensive adjustment for smoking, other confounders and methylation scores for personal smoking, with point estimates in Model 5 equal to 1.31, 1.17, 1.35, 1.30 and 1.26 for MS-568, MS-19, MS-15, MS-28 and MS-17, respectively. The overall attenuation from crude (Model 1) to fully-adjusted OR (Model 5) estimates was 24, 29, 22, 32 and 30%, respectively.
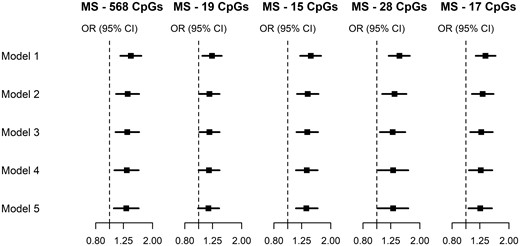
Urothelial cancers: OR and 95% CIs for the association between five methylation scores for maternal smoking and risk of urothelial cancers, using sequential adjustment to assess the robustness of our results confounding factors. ORs were calculated using conditional logistic regression models. Cases and controls were matched on age, sex, country of birth, sample type, and placed consecutively on the same chip of the assay. Model 1: adjusted for white blood cell composition. Model 2: additionally adjusted for smoking status (current/former/never), alcohol consumption (in g/day) and body mass index (in kg/m2). Model 3: additionally adjusted for smoking pack-years, age at starting (never smoked; ≤16 years; 17–21 years; >21 years); years since quitting (never smoked; >10 years without smoking, 5–10 years without smoking; <5 years without smoking), a score for physical activity,50 the alternate healthy eating index to reflect overall diet quality,51 education (score ranging from 1 to 8), socio-economic status (score ranging from 1 to 1052) and height (continuous, in meters). Model 4: additionally adjusted for AS-233: a methylation score for smoking in adulthood developed using external samples.47 Model 5: additionally adjusted for AS-56: a methylation score for smoking in adulthood developed using the MCCS data36
To further examine whether the association with risk of urothelial cancer was due to residual confounding, we used lung cancer as a negative outcome control, Figure 3 and Supplementary Table S4, available as Supplementary data at IJE online (lung cancer being strongly smoking-associated, if the association with urothelial cancer risk was driven by residual confounding from smoking in adulthood, the same should be seen with lung cancer). For MS-28, the association observed in Models 1, 2 and 3 (crude association or adjustment for traditionally measured covariates) fully disappeared after adjustment for methylation scores for smoking in adulthood (point OR = 1.02). For MS-568 and MS-15, we observed negative associations after adjustment for covariates and adult smoking methylation scores (Model 5: MS-568: OR = 0.75, 95%CI = 0.59–0.94; MS-15: OR = 0.71, 95%CI = 0.56–0.91). Consistent effect estimates were observed for MS-19 and MS-17.
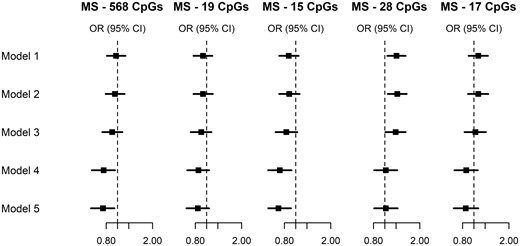
Negative outcome control. Lung cancer: OR and 95% CIs for the association between five methylation scores for maternal smoking and risk of lung cancer, using sequential adjustment to assess the robustness of our results confounding factors. ORs were calculated using conditional logistic regression models. Cases and controls were matched on age, sex, country of birth, sample type and smoking, and placed consecutively on a same chip of the assay. Model 1: adjusted for white blood cell composition. Model 2: additionally adjusted for smoking status (current/former/never), alcohol consumption (in g/day) and body mass index (in kg/m2). Model 3: additionally adjusted for smoking pack-years, age at starting (never smoked; ≤16 years; 17–21 years; >21 years); years since quitting (never smoked; >10 years without smoking, 5–10 years without smoking; <5 years without smoking), a score for physical activity,50 the alternate healthy eating index to reflect overall diet quality,51 education (score ranging from 1 to 8), socio-economic status (score ranging from 1 to 1052) and height (continuous, in meters). Model 4: additionally adjusted for AS-233: a methylation score for smoking in adulthood developed using external samples.47 Model 5: additionally adjusted for AS-56: a methylation score for smoking in adulthood developed using the ORs was calculated using conditional logistic regression models. Cases and controls were matched on age, sex, country of birth, sample type, and placed consecutively on the same chip of the assay
We then used methylation scores for smoking in adulthood as positive exposure controls, i.e. examining their association with risk of lung and urothelial cancer to confirm that they contained information about residual confounding by smoking (Table 3). Although effect estimates were attenuated after adjustment for confounders, all three scores for adult smoking still showed strong associations with lung cancer risk (AS-233: OR = 1.68, AS-56: OR = 1.59 and AS-1061: OR = 1.49, P = 0.0001) and urothelial cancer risk. (AS-233: OR = 1.19, AS-56: OR = 1.24 and AS-1061: OR = 1.32).
Positive exposure control. Lung cancer and urothelial cancers: OR and 95% CIs for the association between three methylation scores for personal smoking and risk of lung and urothelial cancersa
. | Lung cancerd . | OR . | 95% CI . | P . | Urothelial cancers . | OR . | 95% CI . | P . |
---|---|---|---|---|---|---|---|---|
Model 1b: Crude association | AS-233 | 1.84 | 1.43–2.36 | 2 x 10−6 | AS-233 | 1.35 | 1.15–1.57 | 0.0002 |
AS-56 | 1.76 | 1.37–2.27 | 1 x 10−5 | AS-56 | 1.38 | 1.17–1.61 | 9 x 10−5 | |
AS-1061 | 1.59 | 1.26–2.01 | 0.0001 | AS-1061 | 1.47 | 1.24–1.75 | 8 x 10−6 | |
Model 3c: Adjusting for all questionnaire variables | AS-233 | 1.68 | 1.29–2.19 | 0.0001 | AS-233 | 1.19 | 0.94–1.51 | 0.17 |
AS-56 | 1.59 | 1.21–2.07 | 0.0007 | AS-56 | 1.24 | 0.98–1.60 | 0.08 | |
AS-1061 | 1.49 | 1.17–1.90 | 0.001 | AS-1061 | 1.32 | 1.06–1.63 | 0.01 |
. | Lung cancerd . | OR . | 95% CI . | P . | Urothelial cancers . | OR . | 95% CI . | P . |
---|---|---|---|---|---|---|---|---|
Model 1b: Crude association | AS-233 | 1.84 | 1.43–2.36 | 2 x 10−6 | AS-233 | 1.35 | 1.15–1.57 | 0.0002 |
AS-56 | 1.76 | 1.37–2.27 | 1 x 10−5 | AS-56 | 1.38 | 1.17–1.61 | 9 x 10−5 | |
AS-1061 | 1.59 | 1.26–2.01 | 0.0001 | AS-1061 | 1.47 | 1.24–1.75 | 8 x 10−6 | |
Model 3c: Adjusting for all questionnaire variables | AS-233 | 1.68 | 1.29–2.19 | 0.0001 | AS-233 | 1.19 | 0.94–1.51 | 0.17 |
AS-56 | 1.59 | 1.21–2.07 | 0.0007 | AS-56 | 1.24 | 0.98–1.60 | 0.08 | |
AS-1061 | 1.49 | 1.17–1.90 | 0.001 | AS-1061 | 1.32 | 1.06–1.63 | 0.01 |
ORs were calculated using conditional logistic regression models. Cases and controls were matched on age, sex, country of birth and sample type, and placed consecutively on the same chip of the assay.
Model 1: adjusted for white blood cell composition.
Model 3: additionally adjusted for smoking status (current/former/never), alcohol consumption (in g/day) and BMI (in kg/m2), smoking pack-years, age at starting (never smoked; ≤16 years; 17–21 years; >21 years); years since quitting (never smoked; >10 years without smoking, 5–10 years without smoking; <5 years without smoking), a score for physical activity,44 the alternate healthy eating index to reflect overall diet quality,45 education (score ranging from 1 to 8), socio-economic status (score ranging from 1 to 1046) and height (continuous, in meters).
AS-233: a methylation score for smoking in adulthood developed using external samples47; AS-56: a methylation score for smoking in adulthood developed using the MCCS data36; AS-1061: a methylation score for smoking in adulthood developed using the MCCS data based on 1061 CpGs at which methylation was associated with smoking in the MCCS and at least another study at P < 10–7.36
Positive exposure control. Lung cancer and urothelial cancers: OR and 95% CIs for the association between three methylation scores for personal smoking and risk of lung and urothelial cancersa
. | Lung cancerd . | OR . | 95% CI . | P . | Urothelial cancers . | OR . | 95% CI . | P . |
---|---|---|---|---|---|---|---|---|
Model 1b: Crude association | AS-233 | 1.84 | 1.43–2.36 | 2 x 10−6 | AS-233 | 1.35 | 1.15–1.57 | 0.0002 |
AS-56 | 1.76 | 1.37–2.27 | 1 x 10−5 | AS-56 | 1.38 | 1.17–1.61 | 9 x 10−5 | |
AS-1061 | 1.59 | 1.26–2.01 | 0.0001 | AS-1061 | 1.47 | 1.24–1.75 | 8 x 10−6 | |
Model 3c: Adjusting for all questionnaire variables | AS-233 | 1.68 | 1.29–2.19 | 0.0001 | AS-233 | 1.19 | 0.94–1.51 | 0.17 |
AS-56 | 1.59 | 1.21–2.07 | 0.0007 | AS-56 | 1.24 | 0.98–1.60 | 0.08 | |
AS-1061 | 1.49 | 1.17–1.90 | 0.001 | AS-1061 | 1.32 | 1.06–1.63 | 0.01 |
. | Lung cancerd . | OR . | 95% CI . | P . | Urothelial cancers . | OR . | 95% CI . | P . |
---|---|---|---|---|---|---|---|---|
Model 1b: Crude association | AS-233 | 1.84 | 1.43–2.36 | 2 x 10−6 | AS-233 | 1.35 | 1.15–1.57 | 0.0002 |
AS-56 | 1.76 | 1.37–2.27 | 1 x 10−5 | AS-56 | 1.38 | 1.17–1.61 | 9 x 10−5 | |
AS-1061 | 1.59 | 1.26–2.01 | 0.0001 | AS-1061 | 1.47 | 1.24–1.75 | 8 x 10−6 | |
Model 3c: Adjusting for all questionnaire variables | AS-233 | 1.68 | 1.29–2.19 | 0.0001 | AS-233 | 1.19 | 0.94–1.51 | 0.17 |
AS-56 | 1.59 | 1.21–2.07 | 0.0007 | AS-56 | 1.24 | 0.98–1.60 | 0.08 | |
AS-1061 | 1.49 | 1.17–1.90 | 0.001 | AS-1061 | 1.32 | 1.06–1.63 | 0.01 |
ORs were calculated using conditional logistic regression models. Cases and controls were matched on age, sex, country of birth and sample type, and placed consecutively on the same chip of the assay.
Model 1: adjusted for white blood cell composition.
Model 3: additionally adjusted for smoking status (current/former/never), alcohol consumption (in g/day) and BMI (in kg/m2), smoking pack-years, age at starting (never smoked; ≤16 years; 17–21 years; >21 years); years since quitting (never smoked; >10 years without smoking, 5–10 years without smoking; <5 years without smoking), a score for physical activity,44 the alternate healthy eating index to reflect overall diet quality,45 education (score ranging from 1 to 8), socio-economic status (score ranging from 1 to 1046) and height (continuous, in meters).
AS-233: a methylation score for smoking in adulthood developed using external samples47; AS-56: a methylation score for smoking in adulthood developed using the MCCS data36; AS-1061: a methylation score for smoking in adulthood developed using the MCCS data based on 1061 CpGs at which methylation was associated with smoking in the MCCS and at least another study at P < 10–7.36
In the sensitivity analyses, there was no evidence of heterogeneity for the associations with risk of urothelial cancer by sample type and blood collection period (Supplementary Tables S5 and S6, available as Supplementary data at IJE online). The associations with risk of urothelial cancer also remained consistent for case–control pairs restricted to never smokers (Supplementary Table S7, available as Supplementary data at IJE online).
Secondary analyses of cancer subtypes are presented in Supplementary Table S8, available as Supplementary data at IJE online, and generally showed consistent associations, with no heterogeneity observed across subtypes/tumour aggressiveness for breast, colorectal, prostate and urothelial cancers. The association observed for MS-15 with risk of B-cell lymphoma appeared to vary across subtypes (P-heterogeneity = 0.008) being negative for multiple myeloma, follicular lymphoma and low-grade non-Hodgkin’s lymphoma OR = 0.75, 95%CI: 0.52–1.07, OR = 0.56, 95%CI: 0.32–0.99, and OR = 0.60, 95%CI: 0.40–0.89, respectively), and positive for high-grade non-Hodgkin’s lymphoma (OR = 1.28, 95%CI: 0.91–1.70).
Discussion
We have calculated five methylation scores for maternal smoking and three for adult smoking. The overall correlation pattern was consistent with what was expected, i.e. (i) moderate to strong correlations between scores for maternal smoking, (ii) strong to very strong correlations between scores for adult smoking and (iii) weak to moderate correlations between maternal and adult smoking scores.
A strong association was observed between maternal smoking methylation scores and risk of urothelial cancer, which was robust to comprehensive adjustment for questionnaire-collected variables relating to smoking history and methylation scores for smoking in adulthood. Because smoking in adulthood is a strong risk factor for urothelial cancers,54 we made extensive efforts to show that the observed association between urothelial cancer risk and methylation-based prediction of maternal smoking was due to residual confounding by personal smoking, but were unsuccessful. This implies that this may be either a true association, i.e. a novel environmental exposure causing urothelial cancers, or a chance finding. The associations with maternal smoking scores were only slightly attenuated (reduced by 20–30%) when comparing crude estimates with estimates adjusted for all available smoking-related variables collected via questionnaires, several other cancer risk factors and several methylation scores for personal smoking (constructed from both the MCCS and external data to control for the widespread effects of smoking on the methylome). Although not very strongly mutually correlated, maternal smoking methylation scores yielded similar associations with urothelial cancer risk, which might indicate they contained the same information relevant to urothelial cancer risk. To further assess the robustness of our results to residual confounding by smoking in adulthood, we (i) used lung cancer as a negative outcome control and did not observe the same positive associations; in fact, two methylation scores for maternal smoking showed a negative association with lung cancer risk in the same comprehensively adjusted models, and (ii) used methylation scores for smoking in adulthood as positive exposure controls and showed that these were associated with both lung and urothelial cancer risk.
The main limitation of our study was that no information on maternal smoking was collected via questionnaires to further validate the methylation signatures. This information has to our knowledge rarely been collected in adult cohorts; it may be particularly prone to recall bias as many participants may be unaware of their mother’s lifestyle-related exposures during pregnancy. In the study by Reese et al.,31 the prediction of maternal smoking based on measures in blood of newborns was very accurate in external data (AUC∼0.9), but no data were presented relative to the prediction of maternal smoking in adults. The prediction of maternal smoking by the three other scores, evaluated in Richmond et al.,33 appeared somewhat less accurate (AUC∼0.7), presumably due to a longer time since exposure (mean age of 30 years). Participants in our study had a median age of 61 years, which is a long time for these signatures measured in blood to be altered. Although we adjusted for past history and intensity of smoking and methylation patterns due to adult smoking, we did not have information on several other environmental and occupational exposures to chemicals that increase the risk of bladder/urothelial cancer (e.g. polycyclic aromatic hydrocarbons and aromatic amines).54 Although the currently known genes at which these exposures modify DNA methylation do not appear to substantially overlap with those included in maternal smoking scores,55 we cannot rule out that confounding by environmental/occupational exposures played some role in explaining our findings, e.g. if participants exposed to maternal smoking were more frequently exposed to occupational risk factors; adjustment for educational attainment and socio-economic status might have provided some control for such confounding. Another limitation of our study is that we did not include a validation set to replicate our findings; to our knowledge few studies with similar design are available. We performed a relatively large number of (correlated) tests in our analysis; although we did not explicitly correct for multiple testing, the P-values observed for association with urothelial cancer risk in Model 2 were, for four of the five maternal smoking methylation scores, lower than the Bonferroni correction corresponding to eight cancer types (P = 6 x 10−3). Our study included different types of blood samples and blood was collected at two time points (baseline, 1990–94, for the vast majority of samples and follow-up, 2003–07, for a subset of the urothelial cancer study), but these factors did not appear to have introduced heterogeneity in the observed associations, consistent with the relative stability of DNA methylation with blood-storage duration and conditions observed in other studies.56,57 Finally, because the cohort was recruited at adult age (median: 60 years), all deaths and cancer events occurring before baseline were not accounted for; this selection bias possibly resulted in an attenuation of our effect estimates.58
The methylation score based on 15 CpGs showed a negative association with risk of B-cell lymphoma. This finding should be considered with caution due to the weaker evidence and association, and less consistent associations across scores. It is interesting to note however that several types of lymphoma have been positively (i.e. in opposite direction) associated with adverse environmental and occupational exposures59 as well as possibly smoking.60 Similarly, we are cautious not to over-interpret the negative associations between two smoking scores for maternal smoking and risk of lung cancer. Nevertheless, we speculate that as individuals exposed to maternal smoking in pregnancy have increased risk of asthma, allergies and reduced lung function,61 they might inhale tobacco smoke less strongly or avoid any passive smoking or exposure to irritants, which could result in a reduced risk of lung cancer. Adjustment for personal smoking history and methylation scores might also result in some over-adjustment (by a mediating variable) given the potential association between prenatal exposure to maternal smoking and smoking in adulthood. Lung cancer cases and their controls were matched on smoking history but residual differences existed in their smoking pack-years and age at starting, which might explain the OR change after more comprehensive adjustment for smoking.
Although our study does not allow disentangling whether DNA methylation played a mediating role in the observed associations or acted merely as a proxy,35,62,63 cancer-related biological pathways have been identified as among the most enriched for differentially methylated genes with respect to maternal smoking.64 Additionally, several metabolites of tobacco-smoke carcinogens can be detected in the urine of newborns whose mothers smoked during pregnancy and may exert an effect through contact with the developing bladder membrane.65,66 Studies of amniotic fluid of smoking mothers have also found that it was characterized by the presence of tobacco carcinogens67 and amniocyte chromosomal instability,68 which are thought to affect trophoblastic and foetal cells biological functions.69,70 Finally, our study may provide a molecular marker of urothelial cancer that could be useful to better predict or stratify individual risk. It also potentially uncovers a novel mechanism by which environmental exposures may cause urothelial cancer in adulthood, i.e. prenatally.
Conclusion
We used methylation scores to study the association between maternal smoking and risk for eight cancer types. We observed a strong positive association with risk of urothelial cancer, which was robust to adjustment by questionnaire-collected smoking variables and methylation scores for smoking in adulthood. Our study demonstrates the potential for using DNA methylation to investigate the impact of early-life, unmeasured exposures on the risk of disease in adulthood.
Ethics approval
Study participants provided informed consent in accordance with the Declaration of Helsinki and the study was approved by Cancer Council Victoria’s Human Research Ethics Committee.
Supplementary data
Supplementary data are available at IJE online.
Funding
This work was supported by the Australian National Health and Medical Research Council (NHMRC) grant 1164455. MCCS cohort recruitment was funded by VicHealth and Cancer Council Victoria. The MCCS was further supported by Australian NHMRC grants 209057, 251553 and 504711 and by infrastructure provided by Cancer Council Victoria. The nested case–control methylation studies were supported by the NHMRC grants 1011618, 1026892, 1027505, 1050198, 1043616 and 1074383. M.C.S. is an NHMRC Senior Research Fellow (1061177).
Acknowledgements
Cases were ascertained through the Victorian Cancer Registry (VCR) and the Australian Cancer Database (Australian Institute of Health and Welfare).
Data availability
The data and code that support the findings of this study are available from the corresponding author upon reasonable request.
Conflict of interest
None declared.
References
Victorian Cancer Registry.