-
PDF
- Split View
-
Views
-
Cite
Cite
Giacomo Rozzi, Francesco P Lo Muzio, Camilla Sandrini, Stefano Rossi, Lorenzo Fassina, Giuseppe Faggian, Michele Miragoli, Giovanni Battista Luciani, Real-time video kinematic evaluation of the in situ beating right ventricle after pulmonary valve replacement in patients with tetralogy of Fallot: a pilot study, Interactive CardioVascular and Thoracic Surgery, Volume 29, Issue 4, October 2019, Pages 625–631, https://doi.org/10.1093/icvts/ivz120
- Share Icon Share
Abstract
The timing for pulmonary valve replacement (PVR) after tetralogy of Fallot repair is controversial, due to limitations in estimating right ventricular dysfunction and recovery. Intraoperative imaging could add prognostic information, but transoesophageal echocardiography is unsuitable for exploring right heart function. Right ventricular function after PVR was investigated in real time using a novel video-based contactless kinematic evaluation technology (Vi.Ki.E.), which calculates cardiac fatigue and energy consumption.
Six consecutive patients undergoing PVR at 13.8 ± 2.6 years (range 6.9–19.8) after the repair of tetralogy of Fallot were enrolled. Mean right ventricular end-diastolic and end-systolic volume at magnetic resonance imaging were 115.6 ± 16.2 ml/m2 and 61.5 ± 14.6 ml/m2, respectively. Vi.Ki.E. uses a fast-resolution camera placed 45 cm above the open chest, recording cardiac kinematics before and after PVR. An algorithm defines cardiac parameters, such as energy, fatigue, maximum contraction velocity and tissue displacement.
There were no perioperative complications, with patients discharged in satisfactory clinical conditions after 7 ± 2 days (range 5–9). Vi.Ki.E. parameters describing right ventricular dysfunction decreased significantly after surgery: energy consumption by 45% [271 125 ± 9422 (mm/s)2 vs 149 202 ± 11 980 (mm/s)2, P = 0.0001], cardiac fatigue by 12% (292 671 ± 29 369 mm/s2 vs 258 755 ± 42 750 mm/s2, P = 0.01), contraction velocity by 54% (3412 ± 749 mm/s vs 1579 ± 400 mm/s, P = 0.0007) and displacement by 23% (27 ± 4 mm vs 21 ± 4 mm, P = 0.01). Patients undergoing PVR at lower end-diastolic volumes, had greater functional recovery of Vi.Ki.E. parameters.
Intraoperative Vi.Ki.E shows immediate recovery of right ventricular mechanics after PVR with less cardiac fatigue and energy consumption, providing novel insights that may have a prognostic relevance for functional recovery.
INTRODUCTION
Pulmonary regurgitation (PR) is the most common sequela late after repair of tetralogy of Fallot (ToF) [1]. The resulting chronic right ventricle (RV) volume overload leads to varying degrees of ventricular dilatation and dysfunction, which are associated with arrhythmias, cardiac failure and premature death [1]. While pulmonary valve replacement (PVR) has been shown to be effective in resolving PR [2], late results after surgery have not been uniformly associated with clinical improvement [3]. Although to some clinicians, this observation would suggest that the current indications for PVR may be excessively conservative, leading to intervention when irreversible RV dysfunction has ensued [4], others object that a prognosis without PVR may be better in select patient cohorts, due to the additional morbidity associated with PVR surgery [5]. Therefore, the indication and timing for PVR remain a matter of debate.
The current international guidelines for PVR after ToF repair focus on RV functional deterioration, even in asymptomatic patients [6].
However, there is mounting evidence that load-dependent and load-independent indices of RV function assessed by cardiac magnetic resonance (CMR), may be instrumental in guiding indication and timing for PVR [7]. Accordingly, both RV end-diastolic volume (EDV) and RV end-systolic volume (ESV), including ejection fraction, are currently utilized to assess the correct timing of PVR [8]. However, controversy remains on threshold values due to often unpredictable outcomes after PVR. This has stimulated a quest for alternative clinical and experimental methods to investigate the RV function, in patients with chronic PR after ToF repair [9–11]. Theoretically, a technology able to evaluate RV performance during PVR may track functional changes, and ultimately aid in determining the best timing for PVR, in the future. We recently described an original method to elaborate high temporal and spatial resolution parameters of ventricular function in real time during open-chest surgery, using video kinematic recordings [12]. Briefly, this innovative technique was validated in our previous work [12], in which its algorithm efficiency and quality were evaluated by comparing the data obtained from: the cardiac electro-mechanical coupling model; the pendulum harmonic motion; the spatial and temporal resolution and longitudinal assessment of the kinematic parameters and the contraction force by placing a known mass onto the epicardium of a beating rat heart. This pilot study aims to assess safety and efficacy of intraoperative kinematic evaluation to describe acute RV functional changes in patients undergoing PVR late after ToF repair.
MATERIALS AND METHODS
The study was approved by the Institutional Review Board (# 847CESC Protocol # 13371), and all patients signed an informed consent. Selection criteria for the study were (i) PR after transannular patch repair of ToF with pulmonary stenosis; (ii) symptomatic patients with severe PR or asymptomatic patients with evidence of progressive severe RV dilatation or dysfunction [6]; (iii) PVR using stented bioprostheses; and (iv) feasibility of PVR normothermic cardiopulmonary bypass on the beating heart. The latter criterion was introduced to eliminate the influence of intraoperative myocardial ischaemia on postoperative cardiac kinematics. Furthermore, this criterion was also adopted to closely replicate potential advantages of transcatheter PVR, when no additional myocardial ischaemia was involved. Between November 2016 and February 2018, 6 consecutive patients were enrolled. All patients underwent preoperative CMR and transthoracic echocardiographic evaluation. Demographic and laboratory variables are reported in Table 1.
. | N = 6 . | Mean . | Median . | Range . |
---|---|---|---|---|
Gender (male/female) | 3/3 | |||
BSA (m2) | 1.3 ± 0.2 | 1.4 | 0.7–1.7 | |
Age at PVR (years) | 13.0 ± 2.3 | 12.2 | 6.9–20.1 | |
Age at ToF repair (months) | 3.0 ± 0.6 | 2 | 1–5 | |
Time to PVR (years) | 13.8 ± 2.6 | 12.5 | 6.9–19.8 | |
NYHA class II–III | 4/6 | |||
SR, complete RBBB (EKG) | 4/6 | |||
QRS duration (ms) | 156.5 ± 8.7 | 162 | 128–174 | |
Severe PR (ECHO) | 5/6 | |||
Preoperative CMR | ||||
RVEDVi (ml/m2) | 115.6 ± 16.2 | 108.5 | 62.5–183.0 | |
RVESVi (ml/m2) | 61.5 ± 14.6 | 53.7 | 12.5–104.0 | |
RVEF (%) | 55.1 ± 5.5 | 53.8 | 42.0–79.0 | |
PR fraction (%) | 49.0 ± 6.9 | 49.0 | 22.0–73.0 | |
LVEF (%) | 56.0 ± 1.7 | 54.5 | 51.0–62.0 |
. | N = 6 . | Mean . | Median . | Range . |
---|---|---|---|---|
Gender (male/female) | 3/3 | |||
BSA (m2) | 1.3 ± 0.2 | 1.4 | 0.7–1.7 | |
Age at PVR (years) | 13.0 ± 2.3 | 12.2 | 6.9–20.1 | |
Age at ToF repair (months) | 3.0 ± 0.6 | 2 | 1–5 | |
Time to PVR (years) | 13.8 ± 2.6 | 12.5 | 6.9–19.8 | |
NYHA class II–III | 4/6 | |||
SR, complete RBBB (EKG) | 4/6 | |||
QRS duration (ms) | 156.5 ± 8.7 | 162 | 128–174 | |
Severe PR (ECHO) | 5/6 | |||
Preoperative CMR | ||||
RVEDVi (ml/m2) | 115.6 ± 16.2 | 108.5 | 62.5–183.0 | |
RVESVi (ml/m2) | 61.5 ± 14.6 | 53.7 | 12.5–104.0 | |
RVEF (%) | 55.1 ± 5.5 | 53.8 | 42.0–79.0 | |
PR fraction (%) | 49.0 ± 6.9 | 49.0 | 22.0–73.0 | |
LVEF (%) | 56.0 ± 1.7 | 54.5 | 51.0–62.0 |
BSA: body surface area; CMR: cardiac magnetic resonance; LVEF: left ventricular ejection fraction; NYHA: New York Heart Association; PR: pulmonary regurgitation; PVR: pulmonary valve replacement; RBBB: right bundle branch block; RVEDVi: right ventricular end-diastolic volume index; RVEF: right ventricular ejection fraction; RVESVi: right ventricular end-systolic volume index; SR: sinus rhythm; ToF: tetralogy of Fallot.
. | N = 6 . | Mean . | Median . | Range . |
---|---|---|---|---|
Gender (male/female) | 3/3 | |||
BSA (m2) | 1.3 ± 0.2 | 1.4 | 0.7–1.7 | |
Age at PVR (years) | 13.0 ± 2.3 | 12.2 | 6.9–20.1 | |
Age at ToF repair (months) | 3.0 ± 0.6 | 2 | 1–5 | |
Time to PVR (years) | 13.8 ± 2.6 | 12.5 | 6.9–19.8 | |
NYHA class II–III | 4/6 | |||
SR, complete RBBB (EKG) | 4/6 | |||
QRS duration (ms) | 156.5 ± 8.7 | 162 | 128–174 | |
Severe PR (ECHO) | 5/6 | |||
Preoperative CMR | ||||
RVEDVi (ml/m2) | 115.6 ± 16.2 | 108.5 | 62.5–183.0 | |
RVESVi (ml/m2) | 61.5 ± 14.6 | 53.7 | 12.5–104.0 | |
RVEF (%) | 55.1 ± 5.5 | 53.8 | 42.0–79.0 | |
PR fraction (%) | 49.0 ± 6.9 | 49.0 | 22.0–73.0 | |
LVEF (%) | 56.0 ± 1.7 | 54.5 | 51.0–62.0 |
. | N = 6 . | Mean . | Median . | Range . |
---|---|---|---|---|
Gender (male/female) | 3/3 | |||
BSA (m2) | 1.3 ± 0.2 | 1.4 | 0.7–1.7 | |
Age at PVR (years) | 13.0 ± 2.3 | 12.2 | 6.9–20.1 | |
Age at ToF repair (months) | 3.0 ± 0.6 | 2 | 1–5 | |
Time to PVR (years) | 13.8 ± 2.6 | 12.5 | 6.9–19.8 | |
NYHA class II–III | 4/6 | |||
SR, complete RBBB (EKG) | 4/6 | |||
QRS duration (ms) | 156.5 ± 8.7 | 162 | 128–174 | |
Severe PR (ECHO) | 5/6 | |||
Preoperative CMR | ||||
RVEDVi (ml/m2) | 115.6 ± 16.2 | 108.5 | 62.5–183.0 | |
RVESVi (ml/m2) | 61.5 ± 14.6 | 53.7 | 12.5–104.0 | |
RVEF (%) | 55.1 ± 5.5 | 53.8 | 42.0–79.0 | |
PR fraction (%) | 49.0 ± 6.9 | 49.0 | 22.0–73.0 | |
LVEF (%) | 56.0 ± 1.7 | 54.5 | 51.0–62.0 |
BSA: body surface area; CMR: cardiac magnetic resonance; LVEF: left ventricular ejection fraction; NYHA: New York Heart Association; PR: pulmonary regurgitation; PVR: pulmonary valve replacement; RBBB: right bundle branch block; RVEDVi: right ventricular end-diastolic volume index; RVEF: right ventricular ejection fraction; RVESVi: right ventricular end-systolic volume index; SR: sinus rhythm; ToF: tetralogy of Fallot.
Surgical methods
All PVR procedures were performed by 1 surgeon (G.B.L.). Patients underwent PVR via repeat median sternotomy, using aortic and bicaval cannulation and under normothermic (36°C) cardiopulmonary bypass on the beating heart. Video kinematic recordings were focused on the apical trabecular component of the RV, in line with previous evidence showing this portion as the one bearing most of the chronic volume overload [13] (Fig. 1A). All patients underwent postoperative transthoracic echocardiographic evaluation.
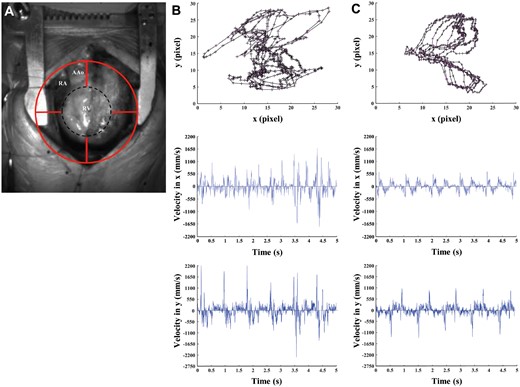
Video kinematic results obtained pre- and post-pulmonary valve replacement (PVR). (A) Marker position (red circle) on the patient’s heart (RV) during open-chest surgery. (B) Upper panel, marker trajectories (black lines with ticks) and acceleration (pink arrows) pre-PVR. Middle panel, video cardiogram in x-axis pre-PVR. Lower panel, video cardiogram in y-axis pre-PVR. (C) Same as (B) but post-PVR. AAo: ascending aorta; Black dashed circle: effective area of analysis; RA: right atrium; RV: right ventricle.
Experimental protocol
For each patient, a high-speed camera (Basler acA1300-200um USB 3.0 with the ON Semiconductor PYTHON 1300 CMOS sensor, Ahrensburg, Germany, equipped with Edmund Optics 6 mm compact fixed focal length lens, Barrington, NJ, USA) was placed approximately 0.4 m above the heart during ToF surgery and 4 videos of 5 s at 200 FPS with a resolution of 1280 × 1024 and a quality of 10p were recorded at 2 time points. Compared to the previous study [12], this camera and the lenses were specifically chosen for human hearts with the intention of increasing the field of view and assessing the RV kinematics globally. The first recording (pre-PVR) was after chest opening, the second one (post-PVR) around 30 min after weaning from cardiopulmonary bypass exit. In the post-PVR recording, great care was taken to reproduce the same haemodynamic conditions observed in the pre-PVR, including central venous pressure, systolic arterial blood pressure, heart rate and identical ventilator settings. No patient required inotropic support and only one required intravenous antiarrhythmic therapy, as specified below (case #3). The videos were acquired on a high-performance PC (Intel core i7-6700HQ 3.5 GHz, 16GB DDR4 2133 MHz SDRAM, 1TB HDD 7200 RPM With 128GB SSD PCIE ×4) by using an USB 3.0 cable through the camera’s own software (Pylon 5 Camera Software Suite 5.0.5 for Microsoft Windows, Ahrensburg, Germany).
Video kinematic evaluation of right ventricle function
The videos were evaluated by using the Video Spot Tracker software (VST, CISMM, Computer Integrated Systems for Microscopy and Manipulation, UNC Chapel Hill, NC, USA), allowing a virtual marker to be placed on the first frame of the video, which follows the light spots on the epicardial surface, tracking the spatial–temporal coordinates x, y and t of that marker in each frame (Fig. 1A and Video 1). Afterwards, a custom-made algorithm [14] implemented with MATLAB Programming Language (The MathWorks, Inc., Natick, MA, USA) analyses these coordinates, evaluates video cardiograms (ViCGs) and presents the following physical quantities:
Displacement: estimates instantaneous movement of the cardiac tissue;
Contraction velocity: estimates instantaneous velocity of the cardiac tissue;
Force: estimates cardiac fatigue (instantaneous acceleration);
Energy: estimates the kinetic energy during cardiac cycle (it follows the classical and Hamiltonian mechanics for kinetic energy ½ mv2) [12].
Intraoperative video recording of cardiac kinematics of the right ventricular outflow tract. A virtual target (red) allows to focus the virtual marker. The virtual marker (yellow) on the first frame of the video follows the light spots on the epicardial surface, tracking the spatial–temporal coordinates x, y and t of that marker in each frame.
The algorithm presents the raw data in pixels and we reported these in SI-accepted unit (m) to provide useful information to surgeons. In the laboratory, we reconstructed a transformation pixel-to-mm curve (r2 = 0.9977) by placing the camera at different heights, from 0.05 to 0.6 m with 0.05 m step, above a millimetre paper (Supplementary Material, Table).
Of note, all scientists involved with experimental data acquisition and interpretation were blinded to preoperative and postoperative clinical and laboratory data.
Statistical analysis
Data are presented as mean ± SEM. The normality of the data was assessed with the Kolmogorov–Smirnov test. The Student’s t-test with Welch’s correction and cumulative distribution with the Kolmogorov–Smirnov test were performed. A value of P<0.05 was considered significant. The programme GraphPad v.6 (GraphPad Software, Inc., La Jolla, CA, USA) was used for analysis and results.
RESULTS
Clinical outcome
All patients underwent surgical PVR on the beating heart: 4 patients received a 23-mm, 1 patient a 21-mm and 1 patient a 25-mm stented bioprosthesis. There were no perioperative complications, except for 1 patient experiencing reversible subclinical hepatic dysfunction. Patients were discharged after a mean of 7.0 ± 2.0 days (5–9 days) of hospitalization. Transthoracic echocardiogram prior to discharge documented the recovery of normal RV dimensions in 5 out of 6 patients (83%), preserved (>50%) right ventricular ejection fraction (RVEF) in 3 out of 6 patients and mildly reduced (>40%) RVEF in 3 out of 6 patients. On Doppler examination there was absence of PR in all patients and a mean peak trans-prosthetic pulmonary valve gradient of 16.5 ± 7.1 mmHg. During a mean follow-up of 8.5 ± 4.5 months (2–15 months), all patients were in New York Heart Association (NYHA) I, except 1 patient in NYHA II. No patient experienced adverse cardiovascular events and all were in regular sinus rhythm, free from oral anticoagulation. The follow-up echocardiogram was similar to that taken before discharge, with a mean peak trans-prosthetic pulmonary valve gradient of 14.5 ± 7.9 mmHg and preserved RVEF in 5 out of 6 patients.
Intraoperative video kinematic evaluation of right ventricle function in patients with tetralogy of Fallot
The qualitative and quantitative results of the 6 patients were extremely homogeneous, except for 1 patient (#3), who exhibited counterintuitive experimental outcomes. Intraoperatively, this patient showed atrial ectopic tachycardia after PVR, which was initially refractory to antiarrhythmic therapy and eventually subsided 6 h after surgery.
From a qualitative point of view, trajectories and ViCGs differed between pre- and post-PVR condition in all patients (Fig. 1). In detail, all trajectories showed irregular and chaotic patterns (Fig. 1B, upper panel) prior to PVR. The same occurred for ViCGs, where systolic and diastolic phases were difficult to differentiate because of background noise in both x and y axes (Fig. 1B, middle and lower panels). On the contrary, trajectories showed regular, overlapped and well-preserved patterns after PVR (Fig. 1C, upper panel). The ViCGs displayed both systolic and diastolic phases and we identified less noise in both x and y axes (Fig. 1C, middle and lower panels). Moreover, marker displacement in trajectories and ViCGs amplitude decreased after PVR when compared to before PVR.
From a quantitative point of view, all kinematic parameters significantly decreased (Fig. 2A) after PVR when compared to before PVR. On an average, displacement decreased by 23%, maximum contraction velocity by 54%, force by 12% and energy by 45% (Fig. 2A). Regarding the mechanical performances of individual patients, we noted (Fig. 2B) that all patients showed a decreasing trend for all parameters, except for patient #3 who exhibited an increasing trend. Prior to PVR, patient #6 showed the highest values for each parameter and the greatest percentage decrease after PVR. In particular, patient #6 values after PVR were greater than the rest of the study population, and, even though these exhibited the greatest decrease, the final parameter values remained 2-fold higher than pre-PVR values from the rest of the study population.
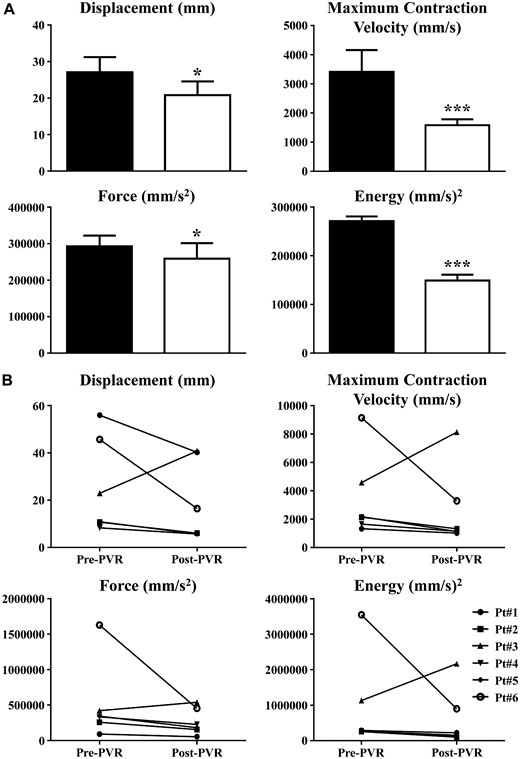
Kinematic parameters in tetralogy of Fallot patients. (A) Pre-PVR (black columns) was recorded at the chest opening before aortic cannulation. Post-PVR (white columns) was recorded after the surgery and protamine sulphate infusion. Data are shown as mean ± SEM. *P < 0.05 versus pre-PVR, ***P < 0.001 versus pre-PVR. (B) Visual representation of video kinematic evaluation parameters, trends for each patient pre- and post-PVR. PVR: pulmonary valve replacement; SEM: standard error of the mean.
To suggest possible clinical correlations, the acquired RV kinematics parameters were compared with CMR, the gold standard RV imaging technique. Thus, we plotted RVEDV index (RVEDVi) versus energy (Fig. 3, upper panel) and force, a surrogate for cardiac fatigue (Fig. 3, lower panel), because these kinematic parameters exhibit the strongest clinical implications. In both graphs, patients undergoing surgery with an RVEDV below 129 ml/m2 showed a consistent decrease in parameter values after PVR, in line with recovery of minimal contraction energy and cardiac fatigue. Patient #6, who underwent PVR with an RVEDVi of 183 ml/m2 (a value currently representing standard indication for surgery) also displayed lower values after PVR with the greatest decrease by far. However, after PVR, energy and force (cardiac fatigue) values in this patient remained higher than the corresponding parameters prior to PVR in the remainder of the study population.
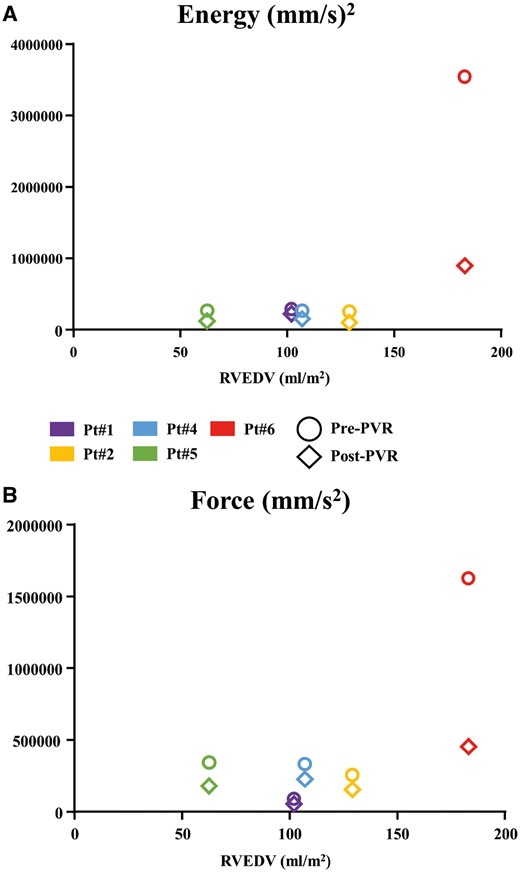
Correlation between cardiac magnetic resonance and right ventricle kinematic parameters. (A) Graph displaying the relation between RVEDV and energy. Colours represent different patients (see legend), circle is pre-PVR and diamond is post-PVR. (B) Same as (A) but for force. PVR: pulmonary valve replacement; RVEDV: right ventricular end-diastolic volume.
DISCUSSION
Although PVR leads to prompt resolution of PR occuring after ToF repair [2], clinical improvement after PVR is not always predictable [3, 5, 7], leading to controversy over indications and timing for surgery.
Therefore, in addition to clinical observations using meta-analyses [2], propensity matched [5] and prospective [4] studies, efforts have been made to devise experimental models [9–11] able to unravel pathophysiology of the RV following PVR.
In this pilot study, a novel method to describe real time in vivo cardiac kinematics was experimentally applied to offer insight into acute changes of RV properties associated with resolution of volume overload by surgical PVR. This technique was previously applied, in patients undergoing surgical myocardial revascularization [12].
The original experimental approach is a video-based and contactless technique, which can describe precise cardiac kinematic information during open-chest procedures. This technology is versatile, as it may be applied for both basic and clinical research, in conjunction with standard monitoring procedures, without interfering with open-chest cardiac surgery. In patients undergoing surgical myocardial revascularization [12], this technique was able to detect fine contraction of the ischaemic/reperfused cardiac tissue areas, which are poorly explored by conventional transoesophageal echocardiography. In this study, we focused our attention on RV pathophysiology.
The results of the current experimental study, demonstrate that in patients with previously operated ToF, RV preload reduction by PVR allows: (i) decrease of contraction force (cardiac fatigue) by 12%; (ii) decrease of energy by 45%; and (iii) decrease of maximum contraction velocity and displacement (instantaneous velocity and instantaneous movement of the cardiac tissue, respectively), because the reduction of force and energy leads to less RV epicardial movement and velocity. One plausible and expected mechanism that could explain this trend is related to the Frank–Starling law: a decrease in RV preload generates a decrease in myocardium stretch (less tension development) and, consequently, a decrease in actin–myosin crossbridge activity leading to less cardiac force and kinetic energy [15].
The pilot study herein provides, for the first time, evidence that PVR may afford immediate recovery of RV functional properties in children and young adults late after ToF repair. Therefore, this work allows one to take the recent findings by Heng et al. [4] on very early (on average 10 days) RV volume recovery at CMR in adults undergoing PVR for chronic PI after ToF on step further. It is noteworthy that when applying standard CMR criteria for PVR, early and late mortality are not negligible and the rate of adverse cardiovascular events (death, VT) does not correlate with either EDV or ESV indices [4, 5]. This suggests that current RV threshold volumes at preoperative CMR will not reliably predict clinical outcome, whereas decline in systolic function (RVEF) may [4, 5, 8]. Surprisingly, the same observations have been made even when using more proactive criteria to refer for PVR [5]. The latter finding may lead one to consider conservative management preferable rather than PVR in patients with PR after repaired ToF. Alternatively, it can be hypothesized that even the use of proactive CMR criteria to refer ToF patients for PVR may be too restrictive to favourably influence late prognosis.
Indeed, evidence by Frigiola et al. [16] suggests that PVR, when performed in children and adolescents (younger than 18 years old) and with more aggressive CMR indications (RVEDVi <150 ml/m2), may be associated with negligible early and late mortality. Furthermore, their surgical referral policy may allow normalization of RV volumes, improvement in biventricular function and normalization of VE/VCO2, a parameter of exercise capacity, which best reflects everyday requirements [16].
Our patient population differs in many ways from most large clinical series on PVR late after ToF repair [2–5, 7, 8]. First and foremost, the average age at primary ToF repair was in the first 3 months of life, representing current standard timing for infant repair, and the average age at PVR was in the early teenage years. On the other hand, most published series report on patients in the third and fourth decade of life undergoing PVR 20–30 years after orginally having had ToF repair at a preschool or school age. Therefore, exposure to the consequences of chronic RV overload had significantly shorter duration in our series. Furthermore, the underlying diagnosis in this study was restricted to ToF with pulmonary stenosis, whereas prior series encompassed a variety of cardiac defects, associated with ToF, including pulmonary atresia. The latter often imply more complex surgery, repair using a period of myocardial ischaemia and the use of extra-cardiac conduits, all factors that may adversely affect RV function. In addition, average RV volumes at preoperative CMR in our series were well below even proactive criteria for PVR in older ToF patients, although closer to the ones reported by Frigiola et al. [16] on PVR in adolescent ToF patients. Finally, and most importantly, unlike most other series, we selected only patients who could undergo beating heart PVR on normothermic cardiopulmonary bypass. Therefore, the additional influence of RV dysfunction due to myocardial ischaemia was eliminated in our study. It is noteworthy, that the series with clinical and CMR variables similar to ours also resorted to routine beating heart PVR [16]. The feasibility of beating heart PVR is important, as prior studies have surmised that failure of PVR to affect mid-term clinical outcome might also be due to the additional surgical trauma incurred during the procedure [2, 3, 5], thus advocating wider use of transcatheter PVR. Based on this hypothesis, we are witnessing a trend towards recommending the lowering of CMR thresholds for PVR when transcatheter intervention is possible [17].
This study suggests a correlation between RV volumes at CMR and contraction force, index of cardiac fatigue, and energy even in patients with preoperative RVEDVi lower than current ‘proactive’ recommendations for surgery (115 vs 152 ml/m2). This observation is in line with prior experimental findings using cardiac catheterization and CMR, where a rapid decline in RV power and efficiency are seen at critical values of 139 ml/m2 RVEDV and 75 ml/m2 RVESV index [18].
Limitations
As a pilot study, the current work is limited by the small cohort size. We focused our attention on young patients with ToF undergoing PVR in a single centre. Moreover, our observations were restricted to the intraoperative acute phase of RV changes following PVR. Both circumstances hamper the ability of this study to generate experimental indices, which may reflect its prognostic clinical value. A 6-month postoperative CMR is currently underway to ascertain whether video kinematic parameters may predict late RV remodelling.
A technical limitation of video kinematic evaluation is related to the use of a 2-dimensional camera (for which depth is obviously missing) in acquiring the 3-dimensional cardiac phenomenon. Nevertheless, the algorithm is already implemented for the 3-dimensional motion. Employing a second camera in the operating room will be the next upgrade.
Finally, although video kinematic evaluation may display limited acute prognostic ability in ToF patients undergoing elective PVR, as the perioperative course of these patients is generally uncomplicated, this novel technology is currently being tested in other operative settings, far more commonly exposed to acute perioperative RV failure, such as staged palliation of single RVs (i.e. aortic or mitral atresia) and LVAD implantation in cardiomyopathies.
CONCLUSIONS
Video kinematic analysis is a safe, contactless and valid intraoperative technique, which demonstrates prompt improvement of RV functional properties through the reduction of cardiac fatigue and kinetic energy in select ToF patients after PVR.
Funding
This study was supported by the Institutional funds of the University of Verona and of the University of Parma [FFBR-MIUR-2017 to M.M.].
Conflict of interest: none declared.
REFERENCES
Author notes
Giacomo Rozzi and Francesco P. LoMuzio authors contributed equally to this work.
MicheleMiragoli and Giovanni Battista Luciani authors jointly supervised this work.
- magnetic resonance imaging
- tetralogy of fallot
- ventricular end-systolic volume
- right ventricle
- fatigue
- diastole
- energy metabolism
- intraoperative care
- surgical procedures, operative
- heart
- patient prognosis
- surgery specialty
- chest
- kinematics
- replacement of pulmonary valve
- repair of tetralogy of fallot