-
PDF
- Split View
-
Views
-
Cite
Cite
Paola Aguiari, Michele Fiorese, Laura Iop, Gino Gerosa, Andrea Bagno, Mechanical testing of pericardium for manufacturing prosthetic heart valves, Interactive CardioVascular and Thoracic Surgery, Volume 22, Issue 1, January 2016, Pages 72–84, https://doi.org/10.1093/icvts/ivv282
- Share Icon Share
Abstract
Mammalian pericardia are currently used for the production of percutaneous prosthetic heart valves. The characteristics of biological tissues largely influence the durability of prosthetic devices used in the percutaneous approach and in traditional surgery, too. This paper reviews methodologies employed to assess and compare mechanical properties of pericardial patches from different mammalian species in order to identify the biomaterials adequate for manufacturing prosthetic heart valves.
INTRODUCTION
Transcatheter aortic valve replacement (TAVR) allows replacing the diseased valve off-pump on a beating heart. The prosthetic valve is delivered via transfemoral, transapical, subclavian and direct aortic access, through a low invasive surgical procedure that has been implemented for high-risk patients since 2002 [1, 2]. So far, the design of percutaneous implantable prosthetic heart valves has become an increasingly important area of investigation in parallel with expanding indications for TAVR [3].
Due to its excellent biological and mechanical properties, pericardium is harvested from mammalian species for constructing bioprosthetic heart valves and for other surgical purposes (i.e. cardiac patches, staple line reinforcement, vascular stents in cardiovascular and lung surgery) [4]. At present, the only TAVR devices that are FDA-approved and CE-marked are produced with bovine and porcine pericardial tissues mounted either within a cobalt–chromium balloon-expandable stent (Edwards SAPIEN, Edwards Lifesciences, Inc., Irvine, CA, USA) [5] or a self-expandable nitinol stent (Medtronic CoreValve, Medtronic, Inc., MN, USA) [6].
Biological tissues are often used after chemical fixation. Glutaraldehyde (GA) is a typical fixation agent known to modify pericardium properties: it mainly reacts with the ε-amino groups of lysyl and hydroxylysyl residues cross-linking collagen molecules. Cross-linking extension depends on GA concentration, temperature and pH. GA allows tissue fixation/stabilization, also reducing antigenicity in heterologous tissues to prevent immune rejection [7]. Other treatments for tissue stabilization have been more recently proposed and they will be briefly presented herewith.
All bioprostheses, including percutaneous heart valves (PHVs), are still suffering from some drawbacks due to the GA treatment: it triggers structural damages in the extracellular matrix (ECM) and increases the risk of calcific degeneration [8, 9], affecting tissue durability. Furthermore, the cytotoxicity and poor biocompatibility of GA are well documented [10]. As a consequence, the research for innovative PHVs is geared towards the development of more compatible biomaterials. To this aim, pericardial tissues are assessed to understand their physiological function and guide their use as heterograft biomaterials [11, 12].
Currently, the mechanical characterization of biological tissues does not follow univocal procedures. Several methods and procedures have been used to assess the mechanical behaviour of pericardial samples harvested from different mammalian species either untreated, chemically treated or decellularized (Table 1).
Synoptic view of major procedures used for the mechanical assessment of pericardial samples
Authors . | Year . | Specie(s) . | Preconditioning . | Testing . |
---|---|---|---|---|
Hildebrandt et al. | 1969 | Canine (native) | Cyclic loading | Uniaxial and biaxial loading |
Lee and Boughnerl | 1981 | Canine | Cyclic loading | Stress relaxation, creep |
Wiegner et al. | 1981 | Canine (native) | Cyclic loading | Stress relaxation, creep, fracture |
Reece et al. | 1982 | Bovine (native, GA-treated) | Cyclic loading | Stress relaxation, creep |
Radjeman et al. | 1985 | Bovine | – | Uniaxial tensile loading |
Lee and Boughner | 1985 | Human and canine | Cyclic loading | Stress relaxation, creep, fracture |
Trowbridge et al. | 1985 | Bovine (GA-treated) | Cyclic loading | Uniaxial tensile loading, stress relaxation |
Lee et al. | 1987 | Human and canine | Cyclic loading | Stress relaxation, creep |
Cohn et al. | 1987 | Human, bovine and canine | – | Uniaxial loading |
Crofts and Trowbridge | 1988 | Bovine (native, GA-treated) | Cyclic loading | Uniaxial tensile loading, stress relaxation, creep |
Lee et al. | 1989 | Bovine | – | Uniaxial loading |
Naimark et al. | 1992 | Bovine, canine, porcine, ovine | Cyclic loading | Stress–strain response, stress relaxation, and forced vibration |
Zioupos et al. | 1992 | Bovine | – | Inflation |
Courtman et al. | 1994 | Bovine | Cyclic loading | Uniaxial loading |
Pasquino et al. | 1994 | Bovine | – | Uniaxial loading |
Duncan and Boughner | 1998 | Bovine (GA-treated) | Cyclic loading | Stress–strain, stress relaxation, creep |
Langdon et al. | 1999 | Bovine (GA-treated) | Cyclic loading | Biaxial tensile loading |
Sung et al. | 1999 | Porcine aortic valve (fixed with a naturally occurring cross-linking agent) | Cyclic loading | Stress relaxation + extension to fracture |
Garcia Páez et al. | 2001 | Ostrich (GA-treated and sutured) | – | Hydraulic simulator |
Garcia Páez et al. | 2001 | Bovine (GA-treated) | – | Hydraulic simulator |
Garcia Páez et al. | 2001 | Porcine (GA-treated) | – | Hydraulic simulator |
Chang et al. | 2002 | Bovine (fixed with a naturally occurring cross-linking agent) | – | Uniaxial tensile loading |
Garcia Páez et al. | 2002 | Bovine (GA-treated) | – | Uniaxial and biaxial tensile loading |
Garcia Páez et al. | 2002 | Porcine(GA-treated) | – | Uniaxial and biaxial tensile loading to rupture |
Garcia Páez et al. | 2003 | Pig, calf, ostrich (GA-treated and Gore-Tex sutured) | – | Uniaxial tensile loading |
Sun et al. | 2004 | Bovine (GA-treated) | 10 cycles | Uniaxial and biaxial tensile cyclic loading |
Mavrilas et al. | 2005 | Bovine (native and degraded) | Manual preloading | Uniaxial tensile cyclic loading |
Liao et al. | 2005 | Bovine (native and GA-treated) | Prestretching | Biaxial tensile loading |
Mirnajafi et al. | 2005 | Bovine (native and GA treated) | – | Flexural loading |
Mirsadraee et al. | 2006 | Human (native and decellularized) | Loading–unloading cycles | Uniaxial tensile loading |
Martin-Maestro et al. | 2006 | Bovine and ostrich (GA treated) | Loading–unloading cycles | Uniaxial tensile loading |
Garcia Páez et al. | 2007 | Calf (GA-treated, polypropylene sutured and glued) | – | Uniaxial tensile loading |
Oswal et al | 2007 | Bovine (native, decellularized, GA-treated) | Preloading of 0.02 N | Uniaxial tensile loading |
Tedder et al. | 2009 | Porcine (native, GA-treated and PGG-treated) | 10 cycles | Cyclic biaxial tensile loading |
SánchezArévalo et al. | 2010 | Bovine (lyophilized and GA-treated) | 10 cycles | Cyclic biaxial tensile loading |
Mendoza-Novelo et al. | 2011 | Bovine (fresh and decellularized) | – | Uniaxial loading, stress relaxation |
Pagoulatou et al. | 2012 | Bovine (fresh and decellularized) | – | Uniaxial loading |
Arbeiter et al. | 2012 | Porcine (native and GA-treated) | – | Uniaxial tensile loading with different strain rates |
Gauvin et al. | 2012 | Bovine and porcine (GA-treated) | 3 cycles | Cyclic biaxial tensile loading |
Yamashita et al. | 2012 | Human (GA-treated) | – | Uniaxial loading |
Gauvin et al. | 2012 | Bovine and porcine (GA-treated) | 3 cycles | Cyclic biaxial tensile loading |
Chen et al. | 2012 | Bovine and donkey (GA-treated) | – | Uniaxial tensile loading |
Vinci et al. | 2013 | Human (decellularized and cryopreserved) | Loading–unloading cycles | Uniaxial tensile loading |
Bai et al. | 2013 | Bovine | – | Uniaxial tensile loading |
Hülsmann et al. | 2013 | Bovine (fresh, decellularized and GA-treated) | – | Uniaxial tensile loading |
Authors . | Year . | Specie(s) . | Preconditioning . | Testing . |
---|---|---|---|---|
Hildebrandt et al. | 1969 | Canine (native) | Cyclic loading | Uniaxial and biaxial loading |
Lee and Boughnerl | 1981 | Canine | Cyclic loading | Stress relaxation, creep |
Wiegner et al. | 1981 | Canine (native) | Cyclic loading | Stress relaxation, creep, fracture |
Reece et al. | 1982 | Bovine (native, GA-treated) | Cyclic loading | Stress relaxation, creep |
Radjeman et al. | 1985 | Bovine | – | Uniaxial tensile loading |
Lee and Boughner | 1985 | Human and canine | Cyclic loading | Stress relaxation, creep, fracture |
Trowbridge et al. | 1985 | Bovine (GA-treated) | Cyclic loading | Uniaxial tensile loading, stress relaxation |
Lee et al. | 1987 | Human and canine | Cyclic loading | Stress relaxation, creep |
Cohn et al. | 1987 | Human, bovine and canine | – | Uniaxial loading |
Crofts and Trowbridge | 1988 | Bovine (native, GA-treated) | Cyclic loading | Uniaxial tensile loading, stress relaxation, creep |
Lee et al. | 1989 | Bovine | – | Uniaxial loading |
Naimark et al. | 1992 | Bovine, canine, porcine, ovine | Cyclic loading | Stress–strain response, stress relaxation, and forced vibration |
Zioupos et al. | 1992 | Bovine | – | Inflation |
Courtman et al. | 1994 | Bovine | Cyclic loading | Uniaxial loading |
Pasquino et al. | 1994 | Bovine | – | Uniaxial loading |
Duncan and Boughner | 1998 | Bovine (GA-treated) | Cyclic loading | Stress–strain, stress relaxation, creep |
Langdon et al. | 1999 | Bovine (GA-treated) | Cyclic loading | Biaxial tensile loading |
Sung et al. | 1999 | Porcine aortic valve (fixed with a naturally occurring cross-linking agent) | Cyclic loading | Stress relaxation + extension to fracture |
Garcia Páez et al. | 2001 | Ostrich (GA-treated and sutured) | – | Hydraulic simulator |
Garcia Páez et al. | 2001 | Bovine (GA-treated) | – | Hydraulic simulator |
Garcia Páez et al. | 2001 | Porcine (GA-treated) | – | Hydraulic simulator |
Chang et al. | 2002 | Bovine (fixed with a naturally occurring cross-linking agent) | – | Uniaxial tensile loading |
Garcia Páez et al. | 2002 | Bovine (GA-treated) | – | Uniaxial and biaxial tensile loading |
Garcia Páez et al. | 2002 | Porcine(GA-treated) | – | Uniaxial and biaxial tensile loading to rupture |
Garcia Páez et al. | 2003 | Pig, calf, ostrich (GA-treated and Gore-Tex sutured) | – | Uniaxial tensile loading |
Sun et al. | 2004 | Bovine (GA-treated) | 10 cycles | Uniaxial and biaxial tensile cyclic loading |
Mavrilas et al. | 2005 | Bovine (native and degraded) | Manual preloading | Uniaxial tensile cyclic loading |
Liao et al. | 2005 | Bovine (native and GA-treated) | Prestretching | Biaxial tensile loading |
Mirnajafi et al. | 2005 | Bovine (native and GA treated) | – | Flexural loading |
Mirsadraee et al. | 2006 | Human (native and decellularized) | Loading–unloading cycles | Uniaxial tensile loading |
Martin-Maestro et al. | 2006 | Bovine and ostrich (GA treated) | Loading–unloading cycles | Uniaxial tensile loading |
Garcia Páez et al. | 2007 | Calf (GA-treated, polypropylene sutured and glued) | – | Uniaxial tensile loading |
Oswal et al | 2007 | Bovine (native, decellularized, GA-treated) | Preloading of 0.02 N | Uniaxial tensile loading |
Tedder et al. | 2009 | Porcine (native, GA-treated and PGG-treated) | 10 cycles | Cyclic biaxial tensile loading |
SánchezArévalo et al. | 2010 | Bovine (lyophilized and GA-treated) | 10 cycles | Cyclic biaxial tensile loading |
Mendoza-Novelo et al. | 2011 | Bovine (fresh and decellularized) | – | Uniaxial loading, stress relaxation |
Pagoulatou et al. | 2012 | Bovine (fresh and decellularized) | – | Uniaxial loading |
Arbeiter et al. | 2012 | Porcine (native and GA-treated) | – | Uniaxial tensile loading with different strain rates |
Gauvin et al. | 2012 | Bovine and porcine (GA-treated) | 3 cycles | Cyclic biaxial tensile loading |
Yamashita et al. | 2012 | Human (GA-treated) | – | Uniaxial loading |
Gauvin et al. | 2012 | Bovine and porcine (GA-treated) | 3 cycles | Cyclic biaxial tensile loading |
Chen et al. | 2012 | Bovine and donkey (GA-treated) | – | Uniaxial tensile loading |
Vinci et al. | 2013 | Human (decellularized and cryopreserved) | Loading–unloading cycles | Uniaxial tensile loading |
Bai et al. | 2013 | Bovine | – | Uniaxial tensile loading |
Hülsmann et al. | 2013 | Bovine (fresh, decellularized and GA-treated) | – | Uniaxial tensile loading |
GA: glutaraldehyde.
Synoptic view of major procedures used for the mechanical assessment of pericardial samples
Authors . | Year . | Specie(s) . | Preconditioning . | Testing . |
---|---|---|---|---|
Hildebrandt et al. | 1969 | Canine (native) | Cyclic loading | Uniaxial and biaxial loading |
Lee and Boughnerl | 1981 | Canine | Cyclic loading | Stress relaxation, creep |
Wiegner et al. | 1981 | Canine (native) | Cyclic loading | Stress relaxation, creep, fracture |
Reece et al. | 1982 | Bovine (native, GA-treated) | Cyclic loading | Stress relaxation, creep |
Radjeman et al. | 1985 | Bovine | – | Uniaxial tensile loading |
Lee and Boughner | 1985 | Human and canine | Cyclic loading | Stress relaxation, creep, fracture |
Trowbridge et al. | 1985 | Bovine (GA-treated) | Cyclic loading | Uniaxial tensile loading, stress relaxation |
Lee et al. | 1987 | Human and canine | Cyclic loading | Stress relaxation, creep |
Cohn et al. | 1987 | Human, bovine and canine | – | Uniaxial loading |
Crofts and Trowbridge | 1988 | Bovine (native, GA-treated) | Cyclic loading | Uniaxial tensile loading, stress relaxation, creep |
Lee et al. | 1989 | Bovine | – | Uniaxial loading |
Naimark et al. | 1992 | Bovine, canine, porcine, ovine | Cyclic loading | Stress–strain response, stress relaxation, and forced vibration |
Zioupos et al. | 1992 | Bovine | – | Inflation |
Courtman et al. | 1994 | Bovine | Cyclic loading | Uniaxial loading |
Pasquino et al. | 1994 | Bovine | – | Uniaxial loading |
Duncan and Boughner | 1998 | Bovine (GA-treated) | Cyclic loading | Stress–strain, stress relaxation, creep |
Langdon et al. | 1999 | Bovine (GA-treated) | Cyclic loading | Biaxial tensile loading |
Sung et al. | 1999 | Porcine aortic valve (fixed with a naturally occurring cross-linking agent) | Cyclic loading | Stress relaxation + extension to fracture |
Garcia Páez et al. | 2001 | Ostrich (GA-treated and sutured) | – | Hydraulic simulator |
Garcia Páez et al. | 2001 | Bovine (GA-treated) | – | Hydraulic simulator |
Garcia Páez et al. | 2001 | Porcine (GA-treated) | – | Hydraulic simulator |
Chang et al. | 2002 | Bovine (fixed with a naturally occurring cross-linking agent) | – | Uniaxial tensile loading |
Garcia Páez et al. | 2002 | Bovine (GA-treated) | – | Uniaxial and biaxial tensile loading |
Garcia Páez et al. | 2002 | Porcine(GA-treated) | – | Uniaxial and biaxial tensile loading to rupture |
Garcia Páez et al. | 2003 | Pig, calf, ostrich (GA-treated and Gore-Tex sutured) | – | Uniaxial tensile loading |
Sun et al. | 2004 | Bovine (GA-treated) | 10 cycles | Uniaxial and biaxial tensile cyclic loading |
Mavrilas et al. | 2005 | Bovine (native and degraded) | Manual preloading | Uniaxial tensile cyclic loading |
Liao et al. | 2005 | Bovine (native and GA-treated) | Prestretching | Biaxial tensile loading |
Mirnajafi et al. | 2005 | Bovine (native and GA treated) | – | Flexural loading |
Mirsadraee et al. | 2006 | Human (native and decellularized) | Loading–unloading cycles | Uniaxial tensile loading |
Martin-Maestro et al. | 2006 | Bovine and ostrich (GA treated) | Loading–unloading cycles | Uniaxial tensile loading |
Garcia Páez et al. | 2007 | Calf (GA-treated, polypropylene sutured and glued) | – | Uniaxial tensile loading |
Oswal et al | 2007 | Bovine (native, decellularized, GA-treated) | Preloading of 0.02 N | Uniaxial tensile loading |
Tedder et al. | 2009 | Porcine (native, GA-treated and PGG-treated) | 10 cycles | Cyclic biaxial tensile loading |
SánchezArévalo et al. | 2010 | Bovine (lyophilized and GA-treated) | 10 cycles | Cyclic biaxial tensile loading |
Mendoza-Novelo et al. | 2011 | Bovine (fresh and decellularized) | – | Uniaxial loading, stress relaxation |
Pagoulatou et al. | 2012 | Bovine (fresh and decellularized) | – | Uniaxial loading |
Arbeiter et al. | 2012 | Porcine (native and GA-treated) | – | Uniaxial tensile loading with different strain rates |
Gauvin et al. | 2012 | Bovine and porcine (GA-treated) | 3 cycles | Cyclic biaxial tensile loading |
Yamashita et al. | 2012 | Human (GA-treated) | – | Uniaxial loading |
Gauvin et al. | 2012 | Bovine and porcine (GA-treated) | 3 cycles | Cyclic biaxial tensile loading |
Chen et al. | 2012 | Bovine and donkey (GA-treated) | – | Uniaxial tensile loading |
Vinci et al. | 2013 | Human (decellularized and cryopreserved) | Loading–unloading cycles | Uniaxial tensile loading |
Bai et al. | 2013 | Bovine | – | Uniaxial tensile loading |
Hülsmann et al. | 2013 | Bovine (fresh, decellularized and GA-treated) | – | Uniaxial tensile loading |
Authors . | Year . | Specie(s) . | Preconditioning . | Testing . |
---|---|---|---|---|
Hildebrandt et al. | 1969 | Canine (native) | Cyclic loading | Uniaxial and biaxial loading |
Lee and Boughnerl | 1981 | Canine | Cyclic loading | Stress relaxation, creep |
Wiegner et al. | 1981 | Canine (native) | Cyclic loading | Stress relaxation, creep, fracture |
Reece et al. | 1982 | Bovine (native, GA-treated) | Cyclic loading | Stress relaxation, creep |
Radjeman et al. | 1985 | Bovine | – | Uniaxial tensile loading |
Lee and Boughner | 1985 | Human and canine | Cyclic loading | Stress relaxation, creep, fracture |
Trowbridge et al. | 1985 | Bovine (GA-treated) | Cyclic loading | Uniaxial tensile loading, stress relaxation |
Lee et al. | 1987 | Human and canine | Cyclic loading | Stress relaxation, creep |
Cohn et al. | 1987 | Human, bovine and canine | – | Uniaxial loading |
Crofts and Trowbridge | 1988 | Bovine (native, GA-treated) | Cyclic loading | Uniaxial tensile loading, stress relaxation, creep |
Lee et al. | 1989 | Bovine | – | Uniaxial loading |
Naimark et al. | 1992 | Bovine, canine, porcine, ovine | Cyclic loading | Stress–strain response, stress relaxation, and forced vibration |
Zioupos et al. | 1992 | Bovine | – | Inflation |
Courtman et al. | 1994 | Bovine | Cyclic loading | Uniaxial loading |
Pasquino et al. | 1994 | Bovine | – | Uniaxial loading |
Duncan and Boughner | 1998 | Bovine (GA-treated) | Cyclic loading | Stress–strain, stress relaxation, creep |
Langdon et al. | 1999 | Bovine (GA-treated) | Cyclic loading | Biaxial tensile loading |
Sung et al. | 1999 | Porcine aortic valve (fixed with a naturally occurring cross-linking agent) | Cyclic loading | Stress relaxation + extension to fracture |
Garcia Páez et al. | 2001 | Ostrich (GA-treated and sutured) | – | Hydraulic simulator |
Garcia Páez et al. | 2001 | Bovine (GA-treated) | – | Hydraulic simulator |
Garcia Páez et al. | 2001 | Porcine (GA-treated) | – | Hydraulic simulator |
Chang et al. | 2002 | Bovine (fixed with a naturally occurring cross-linking agent) | – | Uniaxial tensile loading |
Garcia Páez et al. | 2002 | Bovine (GA-treated) | – | Uniaxial and biaxial tensile loading |
Garcia Páez et al. | 2002 | Porcine(GA-treated) | – | Uniaxial and biaxial tensile loading to rupture |
Garcia Páez et al. | 2003 | Pig, calf, ostrich (GA-treated and Gore-Tex sutured) | – | Uniaxial tensile loading |
Sun et al. | 2004 | Bovine (GA-treated) | 10 cycles | Uniaxial and biaxial tensile cyclic loading |
Mavrilas et al. | 2005 | Bovine (native and degraded) | Manual preloading | Uniaxial tensile cyclic loading |
Liao et al. | 2005 | Bovine (native and GA-treated) | Prestretching | Biaxial tensile loading |
Mirnajafi et al. | 2005 | Bovine (native and GA treated) | – | Flexural loading |
Mirsadraee et al. | 2006 | Human (native and decellularized) | Loading–unloading cycles | Uniaxial tensile loading |
Martin-Maestro et al. | 2006 | Bovine and ostrich (GA treated) | Loading–unloading cycles | Uniaxial tensile loading |
Garcia Páez et al. | 2007 | Calf (GA-treated, polypropylene sutured and glued) | – | Uniaxial tensile loading |
Oswal et al | 2007 | Bovine (native, decellularized, GA-treated) | Preloading of 0.02 N | Uniaxial tensile loading |
Tedder et al. | 2009 | Porcine (native, GA-treated and PGG-treated) | 10 cycles | Cyclic biaxial tensile loading |
SánchezArévalo et al. | 2010 | Bovine (lyophilized and GA-treated) | 10 cycles | Cyclic biaxial tensile loading |
Mendoza-Novelo et al. | 2011 | Bovine (fresh and decellularized) | – | Uniaxial loading, stress relaxation |
Pagoulatou et al. | 2012 | Bovine (fresh and decellularized) | – | Uniaxial loading |
Arbeiter et al. | 2012 | Porcine (native and GA-treated) | – | Uniaxial tensile loading with different strain rates |
Gauvin et al. | 2012 | Bovine and porcine (GA-treated) | 3 cycles | Cyclic biaxial tensile loading |
Yamashita et al. | 2012 | Human (GA-treated) | – | Uniaxial loading |
Gauvin et al. | 2012 | Bovine and porcine (GA-treated) | 3 cycles | Cyclic biaxial tensile loading |
Chen et al. | 2012 | Bovine and donkey (GA-treated) | – | Uniaxial tensile loading |
Vinci et al. | 2013 | Human (decellularized and cryopreserved) | Loading–unloading cycles | Uniaxial tensile loading |
Bai et al. | 2013 | Bovine | – | Uniaxial tensile loading |
Hülsmann et al. | 2013 | Bovine (fresh, decellularized and GA-treated) | – | Uniaxial tensile loading |
GA: glutaraldehyde.
This paper reviews the biomechanical assessment of pericardial tissues to identify optimal candidate materials for manufacturing percutaneous prosthetic heart valves. A preliminary distinction will be done between pericardia from humans and animals, and between fresh (native), chemically treated and decellularized tissues.
HUMAN PERICARDIUM
Major data on the mechanical characterization of human pericardium are presented in Table 2.
Authors . | Year . | Pericardium . | Preconditioning . | Testing . | Modulus . | UTS . | Strain at fracture (%) . |
---|---|---|---|---|---|---|---|
Lee and Boughner | 1985 | Native | Cyclic loading | Stress relaxation, creep, fracture | 20.4 ± 1.9 MN/m2 | 2.51 ± 0.21 MN/m2 | 34.9 ± 1.1 |
Lee et al. | 1987 | Native | Cyclic loading (∼10 times) | Stress relaxation, creep, fracture | – | – | – |
Cohn et al. | 1987 | Native | – | Uniaxial loading | 49.62 ± 3.22 MPa | 7.62 ± 0.96 MPa | – |
Vincentelli et al. | 1998 | Native | – | Uniaxial loading | 0.32 ± 0.15 MPa | 22.17 ± 8.30 MPa | 16.3 ± 0.73 |
GA-treated 5 min | 0.35 ± 0.09 MPa | – | – | ||||
GA-treated 10 min | 0.32 ± 0.09 MPa | 38.46 ± 11.75 MPa | 21.85 ± 0.75 | ||||
GA-treated 30 min | 0.36 ± 0.10 MPa | – | 20.12 ± 1.04 | ||||
GA-treated 60 min | – | – | 18.87 ± 0.86 | ||||
GA-treated 6 months | 0.26 ± 0.06 MPa | – | 30.62 ± 2.54 | ||||
Mirsadraee et al. | 2006 | Native transverse | Loading–unloading cycles | Uniaxial loading | 3.58 E−02 MPaa | 6.4 MPa | 33.4 |
Decell. transverse | 3.88 E−02 MPaa | 7.7 MPa | 34.9 | ||||
Native parallel | 5.40 E−02 MPaa | 9.1 MPa | 28.7 | ||||
Decell. parallel | 4.63 E−02 MPaa | 9.9 MPa | 34.7 | ||||
Ozolinis et al. | 2008 | Native | – | Uniaxial loading | 98.67 ± 20.75 MPa | 3.14 ± 0.70 MPa | 0.119 ± 0.037 |
GA-treated | 50.25 ± 16.04 MPa | 6.20 ± 1.47 MPa | 0.098 ± 0.015 | ||||
Yamashita et al. | 2012 | GA-treated | – | Uniaxial loading | 50.8 MPa | 10.03 MPa | – |
Vinci et al. | 2013 | Native | Loading–unloading cycles at 15% maximum strain | Uniaxial loading | – | – | – |
Decellularized | |||||||
Decellularized and cryopreserved |
Authors . | Year . | Pericardium . | Preconditioning . | Testing . | Modulus . | UTS . | Strain at fracture (%) . |
---|---|---|---|---|---|---|---|
Lee and Boughner | 1985 | Native | Cyclic loading | Stress relaxation, creep, fracture | 20.4 ± 1.9 MN/m2 | 2.51 ± 0.21 MN/m2 | 34.9 ± 1.1 |
Lee et al. | 1987 | Native | Cyclic loading (∼10 times) | Stress relaxation, creep, fracture | – | – | – |
Cohn et al. | 1987 | Native | – | Uniaxial loading | 49.62 ± 3.22 MPa | 7.62 ± 0.96 MPa | – |
Vincentelli et al. | 1998 | Native | – | Uniaxial loading | 0.32 ± 0.15 MPa | 22.17 ± 8.30 MPa | 16.3 ± 0.73 |
GA-treated 5 min | 0.35 ± 0.09 MPa | – | – | ||||
GA-treated 10 min | 0.32 ± 0.09 MPa | 38.46 ± 11.75 MPa | 21.85 ± 0.75 | ||||
GA-treated 30 min | 0.36 ± 0.10 MPa | – | 20.12 ± 1.04 | ||||
GA-treated 60 min | – | – | 18.87 ± 0.86 | ||||
GA-treated 6 months | 0.26 ± 0.06 MPa | – | 30.62 ± 2.54 | ||||
Mirsadraee et al. | 2006 | Native transverse | Loading–unloading cycles | Uniaxial loading | 3.58 E−02 MPaa | 6.4 MPa | 33.4 |
Decell. transverse | 3.88 E−02 MPaa | 7.7 MPa | 34.9 | ||||
Native parallel | 5.40 E−02 MPaa | 9.1 MPa | 28.7 | ||||
Decell. parallel | 4.63 E−02 MPaa | 9.9 MPa | 34.7 | ||||
Ozolinis et al. | 2008 | Native | – | Uniaxial loading | 98.67 ± 20.75 MPa | 3.14 ± 0.70 MPa | 0.119 ± 0.037 |
GA-treated | 50.25 ± 16.04 MPa | 6.20 ± 1.47 MPa | 0.098 ± 0.015 | ||||
Yamashita et al. | 2012 | GA-treated | – | Uniaxial loading | 50.8 MPa | 10.03 MPa | – |
Vinci et al. | 2013 | Native | Loading–unloading cycles at 15% maximum strain | Uniaxial loading | – | – | – |
Decellularized | |||||||
Decellularized and cryopreserved |
aCollagen phase slope.
GA: glutaraldehyde; UTS: ultimate tensile strength.
Authors . | Year . | Pericardium . | Preconditioning . | Testing . | Modulus . | UTS . | Strain at fracture (%) . |
---|---|---|---|---|---|---|---|
Lee and Boughner | 1985 | Native | Cyclic loading | Stress relaxation, creep, fracture | 20.4 ± 1.9 MN/m2 | 2.51 ± 0.21 MN/m2 | 34.9 ± 1.1 |
Lee et al. | 1987 | Native | Cyclic loading (∼10 times) | Stress relaxation, creep, fracture | – | – | – |
Cohn et al. | 1987 | Native | – | Uniaxial loading | 49.62 ± 3.22 MPa | 7.62 ± 0.96 MPa | – |
Vincentelli et al. | 1998 | Native | – | Uniaxial loading | 0.32 ± 0.15 MPa | 22.17 ± 8.30 MPa | 16.3 ± 0.73 |
GA-treated 5 min | 0.35 ± 0.09 MPa | – | – | ||||
GA-treated 10 min | 0.32 ± 0.09 MPa | 38.46 ± 11.75 MPa | 21.85 ± 0.75 | ||||
GA-treated 30 min | 0.36 ± 0.10 MPa | – | 20.12 ± 1.04 | ||||
GA-treated 60 min | – | – | 18.87 ± 0.86 | ||||
GA-treated 6 months | 0.26 ± 0.06 MPa | – | 30.62 ± 2.54 | ||||
Mirsadraee et al. | 2006 | Native transverse | Loading–unloading cycles | Uniaxial loading | 3.58 E−02 MPaa | 6.4 MPa | 33.4 |
Decell. transverse | 3.88 E−02 MPaa | 7.7 MPa | 34.9 | ||||
Native parallel | 5.40 E−02 MPaa | 9.1 MPa | 28.7 | ||||
Decell. parallel | 4.63 E−02 MPaa | 9.9 MPa | 34.7 | ||||
Ozolinis et al. | 2008 | Native | – | Uniaxial loading | 98.67 ± 20.75 MPa | 3.14 ± 0.70 MPa | 0.119 ± 0.037 |
GA-treated | 50.25 ± 16.04 MPa | 6.20 ± 1.47 MPa | 0.098 ± 0.015 | ||||
Yamashita et al. | 2012 | GA-treated | – | Uniaxial loading | 50.8 MPa | 10.03 MPa | – |
Vinci et al. | 2013 | Native | Loading–unloading cycles at 15% maximum strain | Uniaxial loading | – | – | – |
Decellularized | |||||||
Decellularized and cryopreserved |
Authors . | Year . | Pericardium . | Preconditioning . | Testing . | Modulus . | UTS . | Strain at fracture (%) . |
---|---|---|---|---|---|---|---|
Lee and Boughner | 1985 | Native | Cyclic loading | Stress relaxation, creep, fracture | 20.4 ± 1.9 MN/m2 | 2.51 ± 0.21 MN/m2 | 34.9 ± 1.1 |
Lee et al. | 1987 | Native | Cyclic loading (∼10 times) | Stress relaxation, creep, fracture | – | – | – |
Cohn et al. | 1987 | Native | – | Uniaxial loading | 49.62 ± 3.22 MPa | 7.62 ± 0.96 MPa | – |
Vincentelli et al. | 1998 | Native | – | Uniaxial loading | 0.32 ± 0.15 MPa | 22.17 ± 8.30 MPa | 16.3 ± 0.73 |
GA-treated 5 min | 0.35 ± 0.09 MPa | – | – | ||||
GA-treated 10 min | 0.32 ± 0.09 MPa | 38.46 ± 11.75 MPa | 21.85 ± 0.75 | ||||
GA-treated 30 min | 0.36 ± 0.10 MPa | – | 20.12 ± 1.04 | ||||
GA-treated 60 min | – | – | 18.87 ± 0.86 | ||||
GA-treated 6 months | 0.26 ± 0.06 MPa | – | 30.62 ± 2.54 | ||||
Mirsadraee et al. | 2006 | Native transverse | Loading–unloading cycles | Uniaxial loading | 3.58 E−02 MPaa | 6.4 MPa | 33.4 |
Decell. transverse | 3.88 E−02 MPaa | 7.7 MPa | 34.9 | ||||
Native parallel | 5.40 E−02 MPaa | 9.1 MPa | 28.7 | ||||
Decell. parallel | 4.63 E−02 MPaa | 9.9 MPa | 34.7 | ||||
Ozolinis et al. | 2008 | Native | – | Uniaxial loading | 98.67 ± 20.75 MPa | 3.14 ± 0.70 MPa | 0.119 ± 0.037 |
GA-treated | 50.25 ± 16.04 MPa | 6.20 ± 1.47 MPa | 0.098 ± 0.015 | ||||
Yamashita et al. | 2012 | GA-treated | – | Uniaxial loading | 50.8 MPa | 10.03 MPa | – |
Vinci et al. | 2013 | Native | Loading–unloading cycles at 15% maximum strain | Uniaxial loading | – | – | – |
Decellularized | |||||||
Decellularized and cryopreserved |
aCollagen phase slope.
GA: glutaraldehyde; UTS: ultimate tensile strength.
Human pericardium was mechanically assessed and compared with canine pericardium in 1985 [13]. Surgical samples of human pericardium were preconditioned by cyclic loading. After preconditioning, each tissue strip was tested for stress relaxation and creep. Finally, each sample was extended until fracture. Authors concluded that the mechanical behaviour of human pericardium is at least qualitatively similar to that of canine pericardium. In particular, stiffness seems identical; lower stiffness per unit thickness of the human tissue at predicted physiological stresses is compensated by greater wall thickness (human pericardium is ≈ 7.3 times thicker than canine). This effect is also shown from data on fracture strength and stiffness. However, human pericardium displayed a greater viscous response, as it arises from doubled cyclic hysteresis losses, and greater stress relaxation and creep.
Two years later, the behaviour of human pericardium subjected to biaxial isotropic loading was assessed [14]. Preconditioning was performed by cyclic loading. The effects of stretching rate were examined by varying the loading frequency from 0.001 to 0.1 Hz. Thereafter, pericardial samples were subjected to relaxation and creep tests. Authors concluded that human pericardium is viscoelastic: hysteresis was present during cyclic loading tests and relaxation was significant. As for canine pericardium, creep was not significant. The tension–stretch relationship during cyclic loading was insensitive to the loading frequency. Furthermore, canine pericardium is not isotropic, whereas human pericardium is, due to the presence of more layers of variously oriented collagen fibres.
Human pericardium has been evaluated as candidate material for the production of scaffolds for tissue engineering applications. Autologous human pericardium is commonly used for the reconstruction of heart valve leaflet avoiding valve replacement, especially in young patients [15]. Nonetheless, the use of human pericardium for allogenic transplantation requires specific treatments ensuring the elimination of all immunological issues. Unfortunately, these treatments are currently based on GA fixation.
Autologous pericardium was used to reconstruct diseased mitral [16, 17] and aortic valves [18]. The latter paper represents the first and unique attempt to characterize GA-treated human pericardium. GA-treated human pericardium and aortic valve leaflets with various degrees of calcification, collected from patients who underwent aortic valve surgery, were compared. Ultimate tensile strength (UTS) values measured on GA-treated human pericardium, and on non-calcified, calcified and decalcified leaflets were 10, 2.8, 1.0 and 0.8 MPa, respectively. Thus, GA-treated autologous pericardium has sufficient tensile strength for use in aortic valve reconstruction.
The effect of GA on human pericardium was investigated by Vincentelli et al. [19]. They exposed pericardial samples harvested from 25 patients to 0.625% GA for increasing times (5, 10, 30, 60 min and 6 months); strain was significantly greater in treated tissue than in the untreated one, while stiffness decreased with the duration of GA immersion. It was stated that brief immersion in 0.625% GA allows improving the durability of human pericardium.
The lack of a sufficient number of donors restricts the extensive use of any human tissue. This reason probably limited the number of papers on the characterization of human pericardium when compared with the huge number of studies on animal tissues, particularly bovine and porcine.
ANIMAL PERICARDIUM
Among mammalian species, bovine and porcine pericardia have been more frequently characterized and are currently used for the construction of PHVs [5, 6]; dog, sheep and donkey have been also taken into consideration as alternative sources. Surprisingly, there is a lack of biochemical, structural and mechanical characterization of equine pericardium despite the presence of equine pericardial valves: the stentless bioprosthesis 3-F valve from Medtronic [20] and the Cribier et al.'s percutaneous valve [21].
Bovine and porcine pericardium
Bovine pericardium is mechanically characterized as hardly non-linear, anisotropic, multilaminate composite pliable material, which is usually subjected to large deformations during its physiological function as implant material. Histologically, it is made of a network of collagenous and elastic fibres embedded in an amorphous matrix. Collagen type I accounts for 90% of total protein content [22], while the matrix also contains proteoglycans and glycosaminoglycans (GAGs) engaging fibres, cells and interstitial fluid [23].
Major data on the mechanical characterization of bovine pericardium are presented in Table 3.
Authors . | Year . | Pericardium . | Preconditioning . | Testing . | Modulus . | UTS . | Strain at fracture (%) . |
---|---|---|---|---|---|---|---|
Reece et al. | 1982 | GA-treated | – | Uniaxial loading | – | – | – |
Radjeman et al. | 1985 | Native anterior | – | Uniaxial loading | 1.25 ± 0.09 (109 dyne/cm2)a | 13.70 ± 0.95 (109 dyne/cm2) | 16.13 ± 1.16 |
Native posterior | 1.07 ± 0.06 (109 dyne/cm2)a | 12.85 ± 1.26 (109 dyne/cm2) | 15.34 ± 0.47 | ||||
Trowbridge et al. | 1985 | GA-treated | Cyclic loading | Uniaxial loading, stress relaxation | – | – | – |
Cohn et al. | 1987 | Native | – | Uniaxial loading | 53.13 ± 3.74 MPa | 8.91 ± 0.75 MPa | – |
Crofts and Trowbridge | 1988 | Native | – | Uniaxial loading, stress relaxation | – | 8.77 ± 0.77 N/mm2b | 27.17 ± 1.39b |
GA-treated | 10.442 ± 0.96 N/mm2b | 46.05 ± 1.54b | |||||
Naimark et al. | 1992 | Native | Cyclic loading | Stress–strain response, stress relaxation and forced vibration | 30 ± 4 MPa at 0.1 Hz | – | – |
31 ± 4 MPa at 1 Hz | – | – | |||||
29 ± 4 MPa at 10 Hz | – | – | |||||
Zioupos et al. | 1992 | Native circumfer. | – | Inflation | 46 MPac | 18 MPa | – |
Native root-to-apex | 14 MPac | 2.5 MPa | – | ||||
Courtman et al. | 1994 | Native | – | Uniaxial loading | – | 2.25 kN/m | 30.1 kN/m |
TX100 | Uniaxial loading | – | 2.1 kN/m | 28.7 kN/m | |||
Pasquino et al. | 1994 | GA + GLTA | – | Uniaxial loading | – | 16.8 ± 3.8 N/mm2d | 50.4 + 10.4d |
TX100/SDS + GA + GLTA | 13.3 ± 1.5 N/mm2d | 45.1 ± 3.6d | |||||
TX100/SDS + GA | 12.1 ± 2.8 N/mm2d | 42.3 ± 5.4d | |||||
GA | 12.8 ± 4.8 N/mm2d | 38.6 ± 9.9d | |||||
Fresh | 9.1 ± 5.4 N/mm2d | 21.9 ± 6.1d | |||||
Duncan and Boughner | 1998 | Native | Cyclic loading | Uniaxial loading, stress relaxation | 17.0 ± 1.7 MPae | – | – |
GA-treated (static) | 11.9 ± 4.3 MPae | – | – | ||||
GA-treated (dynamic) | 18.0 ± 4.9 MPae | – | – | ||||
Langdon et al. | 1999 | GA-treated | Cyclic loading | Biaxial loading | 250 ± 100 MPaf | – | – |
Garcia Páez et al. | 2001 | GA-treated right ventricle | Cyclic loading | Hydraulic simulator | – | 61.18 ± 12.65 MPag right | |
– | 60.50 ± 13.39 MPag left | – | |||||
Garcia Páez et al. | 2002 | GA-treated right ventricle | – | Hydraulic simulator | – | 49.93 ± 12.01 MPah | – |
GA-treated left ventricle | 71.26 ± 34.47 MPah | – | |||||
Chang et al. | 2002 | GA-treated and fixed with a naturally occurring cross-linking agent | – | Hydraulic simulator, uniaxial loading | – | – | – |
Garcia Páez et al. | 2003 | GA-treated (Gore-Tex sutured) | – | Uniaxial loading | – | 7.16 ± 2.97 MPai | – |
6.48 ± 2.34 MPaj | |||||||
11.49 ± 4.64 MPak | |||||||
Sun et al. | 2004 | GA-treated | 10 cycles | Uniaxial and biaxial tensile cyclic loading | – | – | – |
Liao et al. | 2005 | Bovine (native and GA-treated) | – | Biaxial loading | – | – | – |
Mirnajafi et al. | 2005 | Bovine (native and GA-treated) | Prestretching | Flexural loading | – | – | – |
Mavrilas et al. | 2005 | Native | Manual preloading | Cyclic uniaxial loading | 14 → 7 MPal | – | – |
Degraded | 10 → 5 MPal | – | – | ||||
Maestro et al. | 2006 | GA-treated | – | Uniaxial loading | – | 11.5 ± 4.6 MPa | – |
Oswal et al. | 2007 | Native | – | Cyclic uniaxial loading | 0.099 ± 0.038 GPa | 13.862 ± 6.655 MPa | 36.228 ± 9.018 |
Decellularized | 0.092 ± 0.037 GPa | 14.3424 ± 4.437 MPa | 31.769 ± 18.950 | ||||
0.05% GA-treated | 0.033 ± 0.022 GPa | 8.134 ± 5.019 MPa | 52.293 ± 6.106 | ||||
Decell./0.05% GA | 0.033 ± 0.021 GPa | 7.107 ± 3.437 MPa | 52.218 ± 9.661 | ||||
0.5% GA-treated | 0.077 ± 0.023 GPa | 9.007 ± 4.466 MPa | 47.985 ± 2.689 | ||||
Decell./0.5% GA | 0.049 ± 0.029 GPa | 6.151 ± 3.103 MPa | 42.340 ± 10.913 | ||||
Garcia Páez et al | 2007 | Calf (GA-treated, polypropylene sutured and glued) | – | Uniaxial loading | – | 34.36 ± 12.40 MPam | – |
27.54 ± 10.89 MPan | |||||||
21.28 ± 6.52 MPao | |||||||
19.65 ± 5.26 MPap | |||||||
20.21 ± 6.25 MPaq | |||||||
16.95 ± 8.52 MPar | |||||||
19.29 ± 5.25 MPas | |||||||
15.80 ± 4.23 MPat | |||||||
Sánchez-Arévalo et al. | 2010 | GA-treated 0° | – | Uniaxial loading | 105 MPa | – | – |
GA-treated 45° | 70 MPa | – | – | ||||
GA-treated 90° | 63 MPa | – | – | ||||
Mendoza-Novelo et al. | 2011 | Native | – | Uniaxial loading, stress relaxation | 169.1 MPau | 28 MPau | 28u |
102.9 MPav | 18 MPav | 20v | |||||
Decell ATE | 157.7 MPau | 21 MPau | 25u | ||||
92.9 MPav | 12.5 MPav | 21v | |||||
Decell RAS + ATE | 126.9 MPau | 18 MPau | 28u | ||||
94.4 MPav | 12 MPav | 22v | |||||
Decell Triton X-100 | 139.6 MPau | 17 MPau | 26u | ||||
92.9 MPav | 12 MPav | 23v | |||||
Pagoulatou et al. | 2012 | Fresh | Manual preloading, 10 preconditioning cycles | Uniaxial loading, cyclic uniaxial loading | 48.12 ± 10.19 MPav | – | – |
41.63 ± 14.65 MPau | |||||||
Triton X-100 | 52.67 ± 7.59 MPav | – | – | ||||
44.65 ± 19.73 MPau | |||||||
Trypsin/EDTA | 36.82 ± 3.23 MPav | – | – | ||||
20.96 ± 8.17 MPau | |||||||
Gauvin et al. | 2012 | GA-treated | Cyclic loading | Uniaxial loading | ∼190 MPa | ∼25 MPa | ∼25 |
Chen et al. | 2012 | Acellular GA-treated | – | Uniaxial loading | 21.67 ± 11.69 MPa | 15.05 ± 4.50 (kN/m) m2 | – |
Bai et al. | 2013 | Native | – | Uniaxial loading | 27 MPa | 25 MPa | 59 |
Triton X-100 | 27 MPa | 25 MPa | 58 | ||||
GA-treated | 21 MPa | 29 MPa | 60 | ||||
Hülsmann et al. | 2013 | Native | – | Uniaxial loading | 27 MPa | 7 MPa | 25 |
GA-treated | 10 MPa | 6 MPa | 50 | ||||
Decell SDS/DCA | 12 MPa | 7 MPa | 60 | ||||
Decell SDS/DCA + GA-treated | 5 MPa | 4 MPa | 70 | ||||
Decell Trypsin/EDTA | 15 MPa | 6 MPa | 45 |
Authors . | Year . | Pericardium . | Preconditioning . | Testing . | Modulus . | UTS . | Strain at fracture (%) . |
---|---|---|---|---|---|---|---|
Reece et al. | 1982 | GA-treated | – | Uniaxial loading | – | – | – |
Radjeman et al. | 1985 | Native anterior | – | Uniaxial loading | 1.25 ± 0.09 (109 dyne/cm2)a | 13.70 ± 0.95 (109 dyne/cm2) | 16.13 ± 1.16 |
Native posterior | 1.07 ± 0.06 (109 dyne/cm2)a | 12.85 ± 1.26 (109 dyne/cm2) | 15.34 ± 0.47 | ||||
Trowbridge et al. | 1985 | GA-treated | Cyclic loading | Uniaxial loading, stress relaxation | – | – | – |
Cohn et al. | 1987 | Native | – | Uniaxial loading | 53.13 ± 3.74 MPa | 8.91 ± 0.75 MPa | – |
Crofts and Trowbridge | 1988 | Native | – | Uniaxial loading, stress relaxation | – | 8.77 ± 0.77 N/mm2b | 27.17 ± 1.39b |
GA-treated | 10.442 ± 0.96 N/mm2b | 46.05 ± 1.54b | |||||
Naimark et al. | 1992 | Native | Cyclic loading | Stress–strain response, stress relaxation and forced vibration | 30 ± 4 MPa at 0.1 Hz | – | – |
31 ± 4 MPa at 1 Hz | – | – | |||||
29 ± 4 MPa at 10 Hz | – | – | |||||
Zioupos et al. | 1992 | Native circumfer. | – | Inflation | 46 MPac | 18 MPa | – |
Native root-to-apex | 14 MPac | 2.5 MPa | – | ||||
Courtman et al. | 1994 | Native | – | Uniaxial loading | – | 2.25 kN/m | 30.1 kN/m |
TX100 | Uniaxial loading | – | 2.1 kN/m | 28.7 kN/m | |||
Pasquino et al. | 1994 | GA + GLTA | – | Uniaxial loading | – | 16.8 ± 3.8 N/mm2d | 50.4 + 10.4d |
TX100/SDS + GA + GLTA | 13.3 ± 1.5 N/mm2d | 45.1 ± 3.6d | |||||
TX100/SDS + GA | 12.1 ± 2.8 N/mm2d | 42.3 ± 5.4d | |||||
GA | 12.8 ± 4.8 N/mm2d | 38.6 ± 9.9d | |||||
Fresh | 9.1 ± 5.4 N/mm2d | 21.9 ± 6.1d | |||||
Duncan and Boughner | 1998 | Native | Cyclic loading | Uniaxial loading, stress relaxation | 17.0 ± 1.7 MPae | – | – |
GA-treated (static) | 11.9 ± 4.3 MPae | – | – | ||||
GA-treated (dynamic) | 18.0 ± 4.9 MPae | – | – | ||||
Langdon et al. | 1999 | GA-treated | Cyclic loading | Biaxial loading | 250 ± 100 MPaf | – | – |
Garcia Páez et al. | 2001 | GA-treated right ventricle | Cyclic loading | Hydraulic simulator | – | 61.18 ± 12.65 MPag right | |
– | 60.50 ± 13.39 MPag left | – | |||||
Garcia Páez et al. | 2002 | GA-treated right ventricle | – | Hydraulic simulator | – | 49.93 ± 12.01 MPah | – |
GA-treated left ventricle | 71.26 ± 34.47 MPah | – | |||||
Chang et al. | 2002 | GA-treated and fixed with a naturally occurring cross-linking agent | – | Hydraulic simulator, uniaxial loading | – | – | – |
Garcia Páez et al. | 2003 | GA-treated (Gore-Tex sutured) | – | Uniaxial loading | – | 7.16 ± 2.97 MPai | – |
6.48 ± 2.34 MPaj | |||||||
11.49 ± 4.64 MPak | |||||||
Sun et al. | 2004 | GA-treated | 10 cycles | Uniaxial and biaxial tensile cyclic loading | – | – | – |
Liao et al. | 2005 | Bovine (native and GA-treated) | – | Biaxial loading | – | – | – |
Mirnajafi et al. | 2005 | Bovine (native and GA-treated) | Prestretching | Flexural loading | – | – | – |
Mavrilas et al. | 2005 | Native | Manual preloading | Cyclic uniaxial loading | 14 → 7 MPal | – | – |
Degraded | 10 → 5 MPal | – | – | ||||
Maestro et al. | 2006 | GA-treated | – | Uniaxial loading | – | 11.5 ± 4.6 MPa | – |
Oswal et al. | 2007 | Native | – | Cyclic uniaxial loading | 0.099 ± 0.038 GPa | 13.862 ± 6.655 MPa | 36.228 ± 9.018 |
Decellularized | 0.092 ± 0.037 GPa | 14.3424 ± 4.437 MPa | 31.769 ± 18.950 | ||||
0.05% GA-treated | 0.033 ± 0.022 GPa | 8.134 ± 5.019 MPa | 52.293 ± 6.106 | ||||
Decell./0.05% GA | 0.033 ± 0.021 GPa | 7.107 ± 3.437 MPa | 52.218 ± 9.661 | ||||
0.5% GA-treated | 0.077 ± 0.023 GPa | 9.007 ± 4.466 MPa | 47.985 ± 2.689 | ||||
Decell./0.5% GA | 0.049 ± 0.029 GPa | 6.151 ± 3.103 MPa | 42.340 ± 10.913 | ||||
Garcia Páez et al | 2007 | Calf (GA-treated, polypropylene sutured and glued) | – | Uniaxial loading | – | 34.36 ± 12.40 MPam | – |
27.54 ± 10.89 MPan | |||||||
21.28 ± 6.52 MPao | |||||||
19.65 ± 5.26 MPap | |||||||
20.21 ± 6.25 MPaq | |||||||
16.95 ± 8.52 MPar | |||||||
19.29 ± 5.25 MPas | |||||||
15.80 ± 4.23 MPat | |||||||
Sánchez-Arévalo et al. | 2010 | GA-treated 0° | – | Uniaxial loading | 105 MPa | – | – |
GA-treated 45° | 70 MPa | – | – | ||||
GA-treated 90° | 63 MPa | – | – | ||||
Mendoza-Novelo et al. | 2011 | Native | – | Uniaxial loading, stress relaxation | 169.1 MPau | 28 MPau | 28u |
102.9 MPav | 18 MPav | 20v | |||||
Decell ATE | 157.7 MPau | 21 MPau | 25u | ||||
92.9 MPav | 12.5 MPav | 21v | |||||
Decell RAS + ATE | 126.9 MPau | 18 MPau | 28u | ||||
94.4 MPav | 12 MPav | 22v | |||||
Decell Triton X-100 | 139.6 MPau | 17 MPau | 26u | ||||
92.9 MPav | 12 MPav | 23v | |||||
Pagoulatou et al. | 2012 | Fresh | Manual preloading, 10 preconditioning cycles | Uniaxial loading, cyclic uniaxial loading | 48.12 ± 10.19 MPav | – | – |
41.63 ± 14.65 MPau | |||||||
Triton X-100 | 52.67 ± 7.59 MPav | – | – | ||||
44.65 ± 19.73 MPau | |||||||
Trypsin/EDTA | 36.82 ± 3.23 MPav | – | – | ||||
20.96 ± 8.17 MPau | |||||||
Gauvin et al. | 2012 | GA-treated | Cyclic loading | Uniaxial loading | ∼190 MPa | ∼25 MPa | ∼25 |
Chen et al. | 2012 | Acellular GA-treated | – | Uniaxial loading | 21.67 ± 11.69 MPa | 15.05 ± 4.50 (kN/m) m2 | – |
Bai et al. | 2013 | Native | – | Uniaxial loading | 27 MPa | 25 MPa | 59 |
Triton X-100 | 27 MPa | 25 MPa | 58 | ||||
GA-treated | 21 MPa | 29 MPa | 60 | ||||
Hülsmann et al. | 2013 | Native | – | Uniaxial loading | 27 MPa | 7 MPa | 25 |
GA-treated | 10 MPa | 6 MPa | 50 | ||||
Decell SDS/DCA | 12 MPa | 7 MPa | 60 | ||||
Decell SDS/DCA + GA-treated | 5 MPa | 4 MPa | 70 | ||||
Decell Trypsin/EDTA | 15 MPa | 6 MPa | 45 |
aFinal modulus.
bAveraged over five different orientations.
cTerminal tensile modulus.
dMeasured within 1 week after the process.
eAt 30 mm/s.
fHighest value measured by authors.
gBest result.
hBiaxial stepwise.
iLeft 45°.
jRight 90°.
kCentre.
lCollagen modulus calculated under middle to high-frequency cyclic loadings.
mLongitudinally cut control samples.
nTransversely cut control samples.
oSamples cut longitudinally and sutured.
pSamples cut transversely and sutured.
qLongitudinal samples sutured and glued with Glubran.
rTransverse samples sutured and glued with Glubran.
sLongitudinal samples sutured and glued with Loctite.
tTransverse samples sutured and glued with Loctite.
uApex to base.
vTransverse samples.
GA: glutaraldehyde; UTS: ultimate tensile strength; Triton-X100: t-octylphenoxypolyethoxyethanol; SDS: sodium dodecyl sulphate; ATE: tridecyl alcohol ethoxylate; EDTA: ethylenediaminetetraacetic acid; RAS: reversible alkaline swelling; GLTA: glutamic acid; DCA: deoxycholic acid.
Authors . | Year . | Pericardium . | Preconditioning . | Testing . | Modulus . | UTS . | Strain at fracture (%) . |
---|---|---|---|---|---|---|---|
Reece et al. | 1982 | GA-treated | – | Uniaxial loading | – | – | – |
Radjeman et al. | 1985 | Native anterior | – | Uniaxial loading | 1.25 ± 0.09 (109 dyne/cm2)a | 13.70 ± 0.95 (109 dyne/cm2) | 16.13 ± 1.16 |
Native posterior | 1.07 ± 0.06 (109 dyne/cm2)a | 12.85 ± 1.26 (109 dyne/cm2) | 15.34 ± 0.47 | ||||
Trowbridge et al. | 1985 | GA-treated | Cyclic loading | Uniaxial loading, stress relaxation | – | – | – |
Cohn et al. | 1987 | Native | – | Uniaxial loading | 53.13 ± 3.74 MPa | 8.91 ± 0.75 MPa | – |
Crofts and Trowbridge | 1988 | Native | – | Uniaxial loading, stress relaxation | – | 8.77 ± 0.77 N/mm2b | 27.17 ± 1.39b |
GA-treated | 10.442 ± 0.96 N/mm2b | 46.05 ± 1.54b | |||||
Naimark et al. | 1992 | Native | Cyclic loading | Stress–strain response, stress relaxation and forced vibration | 30 ± 4 MPa at 0.1 Hz | – | – |
31 ± 4 MPa at 1 Hz | – | – | |||||
29 ± 4 MPa at 10 Hz | – | – | |||||
Zioupos et al. | 1992 | Native circumfer. | – | Inflation | 46 MPac | 18 MPa | – |
Native root-to-apex | 14 MPac | 2.5 MPa | – | ||||
Courtman et al. | 1994 | Native | – | Uniaxial loading | – | 2.25 kN/m | 30.1 kN/m |
TX100 | Uniaxial loading | – | 2.1 kN/m | 28.7 kN/m | |||
Pasquino et al. | 1994 | GA + GLTA | – | Uniaxial loading | – | 16.8 ± 3.8 N/mm2d | 50.4 + 10.4d |
TX100/SDS + GA + GLTA | 13.3 ± 1.5 N/mm2d | 45.1 ± 3.6d | |||||
TX100/SDS + GA | 12.1 ± 2.8 N/mm2d | 42.3 ± 5.4d | |||||
GA | 12.8 ± 4.8 N/mm2d | 38.6 ± 9.9d | |||||
Fresh | 9.1 ± 5.4 N/mm2d | 21.9 ± 6.1d | |||||
Duncan and Boughner | 1998 | Native | Cyclic loading | Uniaxial loading, stress relaxation | 17.0 ± 1.7 MPae | – | – |
GA-treated (static) | 11.9 ± 4.3 MPae | – | – | ||||
GA-treated (dynamic) | 18.0 ± 4.9 MPae | – | – | ||||
Langdon et al. | 1999 | GA-treated | Cyclic loading | Biaxial loading | 250 ± 100 MPaf | – | – |
Garcia Páez et al. | 2001 | GA-treated right ventricle | Cyclic loading | Hydraulic simulator | – | 61.18 ± 12.65 MPag right | |
– | 60.50 ± 13.39 MPag left | – | |||||
Garcia Páez et al. | 2002 | GA-treated right ventricle | – | Hydraulic simulator | – | 49.93 ± 12.01 MPah | – |
GA-treated left ventricle | 71.26 ± 34.47 MPah | – | |||||
Chang et al. | 2002 | GA-treated and fixed with a naturally occurring cross-linking agent | – | Hydraulic simulator, uniaxial loading | – | – | – |
Garcia Páez et al. | 2003 | GA-treated (Gore-Tex sutured) | – | Uniaxial loading | – | 7.16 ± 2.97 MPai | – |
6.48 ± 2.34 MPaj | |||||||
11.49 ± 4.64 MPak | |||||||
Sun et al. | 2004 | GA-treated | 10 cycles | Uniaxial and biaxial tensile cyclic loading | – | – | – |
Liao et al. | 2005 | Bovine (native and GA-treated) | – | Biaxial loading | – | – | – |
Mirnajafi et al. | 2005 | Bovine (native and GA-treated) | Prestretching | Flexural loading | – | – | – |
Mavrilas et al. | 2005 | Native | Manual preloading | Cyclic uniaxial loading | 14 → 7 MPal | – | – |
Degraded | 10 → 5 MPal | – | – | ||||
Maestro et al. | 2006 | GA-treated | – | Uniaxial loading | – | 11.5 ± 4.6 MPa | – |
Oswal et al. | 2007 | Native | – | Cyclic uniaxial loading | 0.099 ± 0.038 GPa | 13.862 ± 6.655 MPa | 36.228 ± 9.018 |
Decellularized | 0.092 ± 0.037 GPa | 14.3424 ± 4.437 MPa | 31.769 ± 18.950 | ||||
0.05% GA-treated | 0.033 ± 0.022 GPa | 8.134 ± 5.019 MPa | 52.293 ± 6.106 | ||||
Decell./0.05% GA | 0.033 ± 0.021 GPa | 7.107 ± 3.437 MPa | 52.218 ± 9.661 | ||||
0.5% GA-treated | 0.077 ± 0.023 GPa | 9.007 ± 4.466 MPa | 47.985 ± 2.689 | ||||
Decell./0.5% GA | 0.049 ± 0.029 GPa | 6.151 ± 3.103 MPa | 42.340 ± 10.913 | ||||
Garcia Páez et al | 2007 | Calf (GA-treated, polypropylene sutured and glued) | – | Uniaxial loading | – | 34.36 ± 12.40 MPam | – |
27.54 ± 10.89 MPan | |||||||
21.28 ± 6.52 MPao | |||||||
19.65 ± 5.26 MPap | |||||||
20.21 ± 6.25 MPaq | |||||||
16.95 ± 8.52 MPar | |||||||
19.29 ± 5.25 MPas | |||||||
15.80 ± 4.23 MPat | |||||||
Sánchez-Arévalo et al. | 2010 | GA-treated 0° | – | Uniaxial loading | 105 MPa | – | – |
GA-treated 45° | 70 MPa | – | – | ||||
GA-treated 90° | 63 MPa | – | – | ||||
Mendoza-Novelo et al. | 2011 | Native | – | Uniaxial loading, stress relaxation | 169.1 MPau | 28 MPau | 28u |
102.9 MPav | 18 MPav | 20v | |||||
Decell ATE | 157.7 MPau | 21 MPau | 25u | ||||
92.9 MPav | 12.5 MPav | 21v | |||||
Decell RAS + ATE | 126.9 MPau | 18 MPau | 28u | ||||
94.4 MPav | 12 MPav | 22v | |||||
Decell Triton X-100 | 139.6 MPau | 17 MPau | 26u | ||||
92.9 MPav | 12 MPav | 23v | |||||
Pagoulatou et al. | 2012 | Fresh | Manual preloading, 10 preconditioning cycles | Uniaxial loading, cyclic uniaxial loading | 48.12 ± 10.19 MPav | – | – |
41.63 ± 14.65 MPau | |||||||
Triton X-100 | 52.67 ± 7.59 MPav | – | – | ||||
44.65 ± 19.73 MPau | |||||||
Trypsin/EDTA | 36.82 ± 3.23 MPav | – | – | ||||
20.96 ± 8.17 MPau | |||||||
Gauvin et al. | 2012 | GA-treated | Cyclic loading | Uniaxial loading | ∼190 MPa | ∼25 MPa | ∼25 |
Chen et al. | 2012 | Acellular GA-treated | – | Uniaxial loading | 21.67 ± 11.69 MPa | 15.05 ± 4.50 (kN/m) m2 | – |
Bai et al. | 2013 | Native | – | Uniaxial loading | 27 MPa | 25 MPa | 59 |
Triton X-100 | 27 MPa | 25 MPa | 58 | ||||
GA-treated | 21 MPa | 29 MPa | 60 | ||||
Hülsmann et al. | 2013 | Native | – | Uniaxial loading | 27 MPa | 7 MPa | 25 |
GA-treated | 10 MPa | 6 MPa | 50 | ||||
Decell SDS/DCA | 12 MPa | 7 MPa | 60 | ||||
Decell SDS/DCA + GA-treated | 5 MPa | 4 MPa | 70 | ||||
Decell Trypsin/EDTA | 15 MPa | 6 MPa | 45 |
Authors . | Year . | Pericardium . | Preconditioning . | Testing . | Modulus . | UTS . | Strain at fracture (%) . |
---|---|---|---|---|---|---|---|
Reece et al. | 1982 | GA-treated | – | Uniaxial loading | – | – | – |
Radjeman et al. | 1985 | Native anterior | – | Uniaxial loading | 1.25 ± 0.09 (109 dyne/cm2)a | 13.70 ± 0.95 (109 dyne/cm2) | 16.13 ± 1.16 |
Native posterior | 1.07 ± 0.06 (109 dyne/cm2)a | 12.85 ± 1.26 (109 dyne/cm2) | 15.34 ± 0.47 | ||||
Trowbridge et al. | 1985 | GA-treated | Cyclic loading | Uniaxial loading, stress relaxation | – | – | – |
Cohn et al. | 1987 | Native | – | Uniaxial loading | 53.13 ± 3.74 MPa | 8.91 ± 0.75 MPa | – |
Crofts and Trowbridge | 1988 | Native | – | Uniaxial loading, stress relaxation | – | 8.77 ± 0.77 N/mm2b | 27.17 ± 1.39b |
GA-treated | 10.442 ± 0.96 N/mm2b | 46.05 ± 1.54b | |||||
Naimark et al. | 1992 | Native | Cyclic loading | Stress–strain response, stress relaxation and forced vibration | 30 ± 4 MPa at 0.1 Hz | – | – |
31 ± 4 MPa at 1 Hz | – | – | |||||
29 ± 4 MPa at 10 Hz | – | – | |||||
Zioupos et al. | 1992 | Native circumfer. | – | Inflation | 46 MPac | 18 MPa | – |
Native root-to-apex | 14 MPac | 2.5 MPa | – | ||||
Courtman et al. | 1994 | Native | – | Uniaxial loading | – | 2.25 kN/m | 30.1 kN/m |
TX100 | Uniaxial loading | – | 2.1 kN/m | 28.7 kN/m | |||
Pasquino et al. | 1994 | GA + GLTA | – | Uniaxial loading | – | 16.8 ± 3.8 N/mm2d | 50.4 + 10.4d |
TX100/SDS + GA + GLTA | 13.3 ± 1.5 N/mm2d | 45.1 ± 3.6d | |||||
TX100/SDS + GA | 12.1 ± 2.8 N/mm2d | 42.3 ± 5.4d | |||||
GA | 12.8 ± 4.8 N/mm2d | 38.6 ± 9.9d | |||||
Fresh | 9.1 ± 5.4 N/mm2d | 21.9 ± 6.1d | |||||
Duncan and Boughner | 1998 | Native | Cyclic loading | Uniaxial loading, stress relaxation | 17.0 ± 1.7 MPae | – | – |
GA-treated (static) | 11.9 ± 4.3 MPae | – | – | ||||
GA-treated (dynamic) | 18.0 ± 4.9 MPae | – | – | ||||
Langdon et al. | 1999 | GA-treated | Cyclic loading | Biaxial loading | 250 ± 100 MPaf | – | – |
Garcia Páez et al. | 2001 | GA-treated right ventricle | Cyclic loading | Hydraulic simulator | – | 61.18 ± 12.65 MPag right | |
– | 60.50 ± 13.39 MPag left | – | |||||
Garcia Páez et al. | 2002 | GA-treated right ventricle | – | Hydraulic simulator | – | 49.93 ± 12.01 MPah | – |
GA-treated left ventricle | 71.26 ± 34.47 MPah | – | |||||
Chang et al. | 2002 | GA-treated and fixed with a naturally occurring cross-linking agent | – | Hydraulic simulator, uniaxial loading | – | – | – |
Garcia Páez et al. | 2003 | GA-treated (Gore-Tex sutured) | – | Uniaxial loading | – | 7.16 ± 2.97 MPai | – |
6.48 ± 2.34 MPaj | |||||||
11.49 ± 4.64 MPak | |||||||
Sun et al. | 2004 | GA-treated | 10 cycles | Uniaxial and biaxial tensile cyclic loading | – | – | – |
Liao et al. | 2005 | Bovine (native and GA-treated) | – | Biaxial loading | – | – | – |
Mirnajafi et al. | 2005 | Bovine (native and GA-treated) | Prestretching | Flexural loading | – | – | – |
Mavrilas et al. | 2005 | Native | Manual preloading | Cyclic uniaxial loading | 14 → 7 MPal | – | – |
Degraded | 10 → 5 MPal | – | – | ||||
Maestro et al. | 2006 | GA-treated | – | Uniaxial loading | – | 11.5 ± 4.6 MPa | – |
Oswal et al. | 2007 | Native | – | Cyclic uniaxial loading | 0.099 ± 0.038 GPa | 13.862 ± 6.655 MPa | 36.228 ± 9.018 |
Decellularized | 0.092 ± 0.037 GPa | 14.3424 ± 4.437 MPa | 31.769 ± 18.950 | ||||
0.05% GA-treated | 0.033 ± 0.022 GPa | 8.134 ± 5.019 MPa | 52.293 ± 6.106 | ||||
Decell./0.05% GA | 0.033 ± 0.021 GPa | 7.107 ± 3.437 MPa | 52.218 ± 9.661 | ||||
0.5% GA-treated | 0.077 ± 0.023 GPa | 9.007 ± 4.466 MPa | 47.985 ± 2.689 | ||||
Decell./0.5% GA | 0.049 ± 0.029 GPa | 6.151 ± 3.103 MPa | 42.340 ± 10.913 | ||||
Garcia Páez et al | 2007 | Calf (GA-treated, polypropylene sutured and glued) | – | Uniaxial loading | – | 34.36 ± 12.40 MPam | – |
27.54 ± 10.89 MPan | |||||||
21.28 ± 6.52 MPao | |||||||
19.65 ± 5.26 MPap | |||||||
20.21 ± 6.25 MPaq | |||||||
16.95 ± 8.52 MPar | |||||||
19.29 ± 5.25 MPas | |||||||
15.80 ± 4.23 MPat | |||||||
Sánchez-Arévalo et al. | 2010 | GA-treated 0° | – | Uniaxial loading | 105 MPa | – | – |
GA-treated 45° | 70 MPa | – | – | ||||
GA-treated 90° | 63 MPa | – | – | ||||
Mendoza-Novelo et al. | 2011 | Native | – | Uniaxial loading, stress relaxation | 169.1 MPau | 28 MPau | 28u |
102.9 MPav | 18 MPav | 20v | |||||
Decell ATE | 157.7 MPau | 21 MPau | 25u | ||||
92.9 MPav | 12.5 MPav | 21v | |||||
Decell RAS + ATE | 126.9 MPau | 18 MPau | 28u | ||||
94.4 MPav | 12 MPav | 22v | |||||
Decell Triton X-100 | 139.6 MPau | 17 MPau | 26u | ||||
92.9 MPav | 12 MPav | 23v | |||||
Pagoulatou et al. | 2012 | Fresh | Manual preloading, 10 preconditioning cycles | Uniaxial loading, cyclic uniaxial loading | 48.12 ± 10.19 MPav | – | – |
41.63 ± 14.65 MPau | |||||||
Triton X-100 | 52.67 ± 7.59 MPav | – | – | ||||
44.65 ± 19.73 MPau | |||||||
Trypsin/EDTA | 36.82 ± 3.23 MPav | – | – | ||||
20.96 ± 8.17 MPau | |||||||
Gauvin et al. | 2012 | GA-treated | Cyclic loading | Uniaxial loading | ∼190 MPa | ∼25 MPa | ∼25 |
Chen et al. | 2012 | Acellular GA-treated | – | Uniaxial loading | 21.67 ± 11.69 MPa | 15.05 ± 4.50 (kN/m) m2 | – |
Bai et al. | 2013 | Native | – | Uniaxial loading | 27 MPa | 25 MPa | 59 |
Triton X-100 | 27 MPa | 25 MPa | 58 | ||||
GA-treated | 21 MPa | 29 MPa | 60 | ||||
Hülsmann et al. | 2013 | Native | – | Uniaxial loading | 27 MPa | 7 MPa | 25 |
GA-treated | 10 MPa | 6 MPa | 50 | ||||
Decell SDS/DCA | 12 MPa | 7 MPa | 60 | ||||
Decell SDS/DCA + GA-treated | 5 MPa | 4 MPa | 70 | ||||
Decell Trypsin/EDTA | 15 MPa | 6 MPa | 45 |
aFinal modulus.
bAveraged over five different orientations.
cTerminal tensile modulus.
dMeasured within 1 week after the process.
eAt 30 mm/s.
fHighest value measured by authors.
gBest result.
hBiaxial stepwise.
iLeft 45°.
jRight 90°.
kCentre.
lCollagen modulus calculated under middle to high-frequency cyclic loadings.
mLongitudinally cut control samples.
nTransversely cut control samples.
oSamples cut longitudinally and sutured.
pSamples cut transversely and sutured.
qLongitudinal samples sutured and glued with Glubran.
rTransverse samples sutured and glued with Glubran.
sLongitudinal samples sutured and glued with Loctite.
tTransverse samples sutured and glued with Loctite.
uApex to base.
vTransverse samples.
GA: glutaraldehyde; UTS: ultimate tensile strength; Triton-X100: t-octylphenoxypolyethoxyethanol; SDS: sodium dodecyl sulphate; ATE: tridecyl alcohol ethoxylate; EDTA: ethylenediaminetetraacetic acid; RAS: reversible alkaline swelling; GLTA: glutamic acid; DCA: deoxycholic acid.
The first complete characterization of bovine pericardium was proposed in 1985 [24]. The typical J-shaped stress–strain curve that pericardial tissues present in response to uniaxial tensile stress was observed . Anisotropy of bovine pericardium was ascertained: the tissue is more extensible when stretched along the vertical direction and less extensible along the horizontal direction (strain at transition: 5.45 ± 0.26% horizontal and 6.96 ± 0.20% vertical). Viscoelastic properties were also checked. Moreover, the strain at rupture was found to be independent of the strain rates and stretching direction; the stress at rupture was largest when stretched in the horizontal (circumferential) direction (13.45 ± 0.87 × 107 dyn/cm2) and least in the vertical direction (7.21 ± 0.31 × 107 dyn/cm2). Stresses at rupture also increased with the strain rate (13.5 ± 0.87 × 107 dyn/cm2 at 666.7%/min; 11.38 ± 0.38 dyn/cm2 at 66.7%/min; 8.44 ± 0.55 dyn/cm2 at 6.7%/min).
Comparing human, canine and bovine pericardia, Cohn et al. proposed that the J-shaped stress–strain curve represents a common basic mechanical response for all the tissues analysed. The considered mechanical parameters showed that canine pericardium is the stiffest and the strongest (E2 in MPa: human 49.62 ± 3.22, canine 223.80 ± 18.25 and bovine 53.13 ± 3.74; UTS in MPa: human 7.62 ± 0.96, canine 23.35 ± 2.10 and bovine 8.91 ± 0.75). Moreover, the specific collagen contribution to mechanical behaviour was assessed through selective collagenase digestion. The mechanical response under high stresses is maintained by collagen fibres, whereas elastin is responsible for the initial low modulus [25]. Eventually, the anisotropy described by Radjeman was denied.
The anisotropy of fresh bovine pericardium represents a controversial issue: it was also argued by Zioupos et al. [26]. The optical anisotropy of both native and chemically treated pericardium was investigated by polarized light microscopy. Significant directional differences were found: UTS was greater along the circumferential direction with a mean value of 18 MPa compared with the root-to-apex value of 2.5 MPa (P < 0.01). Failure occurred abruptly for the circumferential strips; the root-to-apex strip failure appeared as an irregular progressive fall in load. A specific apparatus for the inflation test was proposed.
The group of Michael Sacks deeply investigated the arrangement of collagen fibres in bovine pericardium through the small-angle light-scattering technique, combined with biaxial mechanical testing. Relationships between collagen network architecture and isotropy were assessed [27]. Authors gave evidence of collagen fibres’ preferential orientation along the circumferential direction in the anterior part of the pericardial sac. To address the choice of the best area as the source of tissue, a cartography of fibres’ prevalent orientation combined with their thickness distribution was proposed [28, 29]. A large area with uniform characteristics was identified close to the left ventricle.
Mavrilas et al. [4] argued on the contribution of GAGs (hyaluronan, chondroitine sulphate and dermatan sulphate) to the mechanical behaviour of bovine pericardium. By cyclic uniaxial tensile testing after enzymatic degradation, authors concluded that interfibrillar GAGs are involved in transmitting loads at molecular and microstructural levels.
Bovine and porcine pericardial tissues are currently preferred for their availability and traceability. Bovine pericardium has been intensively investigated due to its optimal mechanical features, while porcine pericardium has been explored mainly due to its lower thickness. In a comparative study to highlight their potential advantages, bovine and porcine pericardial patches were morphologically, biologically and mechanically compared [30]. Specimens were subjected to uniaxial tensile load after preconditioning (three cyclic loading sequences to 10% of failure strain). UTS values showed that calf pericardium displays mechanical properties significantly higher than porcine (25 vs 12 MPa). Similar results were seen in the elastic modulus. On the other hand, no significant difference was found with regard to the strain at rupture.
Major data on the mechanical characterization of porcine pericardium are presented in Table 4.
Authors . | Year . | Pericardium . | Preconditioning . | Testing . | Modulus . | UTS . | Strain at fracture (%) . |
---|---|---|---|---|---|---|---|
Naimark et al. | 1992 | Native | Cyclic loading | Stress–strain response, stress relaxation, and forced vibration | 41 ± 3 MPa at 0.1 Hz | – | – |
46 ± 4 MPa at 1 Hz | – | – | |||||
43 ± 4 MPa at 10 Hz | – | – | |||||
Garcia Páez et al. | 2001 | GA-treated | – | Hydraulic simulator | – | 43.29–63.01 MPa | – |
Chloroform/methanol | – | 28.93–42.30 MPa | – | ||||
SDS | – | 13.49–19.06 MPa | – | ||||
Garcia Páez et al. | 2001 | GA-treated (different regions, different zones) | – | Hydraulic simulator | – | 64.38 ± 17.59 MPaa | |
Garcia Páez et al. | 2002 | GA-treated right ventricle | – | Uniaxial and biaxial loading | – | 61.15 ± 23.31 MPab | – |
GA-treated left ventricle | – | 67.12 ± 37.46 MPab | – | ||||
Garcia Páez et al. | 2003 | GA-treated (Gore-Tex sutured) | – | Uniaxial loading | – | 10.46 ± 2.70 MPac | – |
– | 9.85 ± 3.09 MPad | – | |||||
– | 14.00 ± 3.50 MPae | – | |||||
Tedder et al. | 2011 | Native | Cyclic loading | Biaxial loading | – | – | – |
GA-treated | |||||||
PGG-treated | |||||||
Gauvin et al. | 2012 | GA-treated | Cyclic loading | Uniaxial loading | ∼100 MPa | ∼12 MPa | ∼24 |
Arbeiter et al. | 2012 | Native | – | Uniaxial loading | 133 ± 15 MPa | 9.4 ± 1.7 MPa | 20 ± 1.1 |
GA-treated | 47 ± 9 MPa | 9.5 ± 1.9 MPa | 49.5 ± 1.7 |
Authors . | Year . | Pericardium . | Preconditioning . | Testing . | Modulus . | UTS . | Strain at fracture (%) . |
---|---|---|---|---|---|---|---|
Naimark et al. | 1992 | Native | Cyclic loading | Stress–strain response, stress relaxation, and forced vibration | 41 ± 3 MPa at 0.1 Hz | – | – |
46 ± 4 MPa at 1 Hz | – | – | |||||
43 ± 4 MPa at 10 Hz | – | – | |||||
Garcia Páez et al. | 2001 | GA-treated | – | Hydraulic simulator | – | 43.29–63.01 MPa | – |
Chloroform/methanol | – | 28.93–42.30 MPa | – | ||||
SDS | – | 13.49–19.06 MPa | – | ||||
Garcia Páez et al. | 2001 | GA-treated (different regions, different zones) | – | Hydraulic simulator | – | 64.38 ± 17.59 MPaa | |
Garcia Páez et al. | 2002 | GA-treated right ventricle | – | Uniaxial and biaxial loading | – | 61.15 ± 23.31 MPab | – |
GA-treated left ventricle | – | 67.12 ± 37.46 MPab | – | ||||
Garcia Páez et al. | 2003 | GA-treated (Gore-Tex sutured) | – | Uniaxial loading | – | 10.46 ± 2.70 MPac | – |
– | 9.85 ± 3.09 MPad | – | |||||
– | 14.00 ± 3.50 MPae | – | |||||
Tedder et al. | 2011 | Native | Cyclic loading | Biaxial loading | – | – | – |
GA-treated | |||||||
PGG-treated | |||||||
Gauvin et al. | 2012 | GA-treated | Cyclic loading | Uniaxial loading | ∼100 MPa | ∼12 MPa | ∼24 |
Arbeiter et al. | 2012 | Native | – | Uniaxial loading | 133 ± 15 MPa | 9.4 ± 1.7 MPa | 20 ± 1.1 |
GA-treated | 47 ± 9 MPa | 9.5 ± 1.9 MPa | 49.5 ± 1.7 |
aHighest result.
bBiaxial stepwise.
cLeft 45°.
dRight 90°.
eCentre.
GA: glutaraldehyde; UTS: ultimate tensile strength; SDS: sodium dodecyl sulphate; PGG: penta-galloyl glucose.
Authors . | Year . | Pericardium . | Preconditioning . | Testing . | Modulus . | UTS . | Strain at fracture (%) . |
---|---|---|---|---|---|---|---|
Naimark et al. | 1992 | Native | Cyclic loading | Stress–strain response, stress relaxation, and forced vibration | 41 ± 3 MPa at 0.1 Hz | – | – |
46 ± 4 MPa at 1 Hz | – | – | |||||
43 ± 4 MPa at 10 Hz | – | – | |||||
Garcia Páez et al. | 2001 | GA-treated | – | Hydraulic simulator | – | 43.29–63.01 MPa | – |
Chloroform/methanol | – | 28.93–42.30 MPa | – | ||||
SDS | – | 13.49–19.06 MPa | – | ||||
Garcia Páez et al. | 2001 | GA-treated (different regions, different zones) | – | Hydraulic simulator | – | 64.38 ± 17.59 MPaa | |
Garcia Páez et al. | 2002 | GA-treated right ventricle | – | Uniaxial and biaxial loading | – | 61.15 ± 23.31 MPab | – |
GA-treated left ventricle | – | 67.12 ± 37.46 MPab | – | ||||
Garcia Páez et al. | 2003 | GA-treated (Gore-Tex sutured) | – | Uniaxial loading | – | 10.46 ± 2.70 MPac | – |
– | 9.85 ± 3.09 MPad | – | |||||
– | 14.00 ± 3.50 MPae | – | |||||
Tedder et al. | 2011 | Native | Cyclic loading | Biaxial loading | – | – | – |
GA-treated | |||||||
PGG-treated | |||||||
Gauvin et al. | 2012 | GA-treated | Cyclic loading | Uniaxial loading | ∼100 MPa | ∼12 MPa | ∼24 |
Arbeiter et al. | 2012 | Native | – | Uniaxial loading | 133 ± 15 MPa | 9.4 ± 1.7 MPa | 20 ± 1.1 |
GA-treated | 47 ± 9 MPa | 9.5 ± 1.9 MPa | 49.5 ± 1.7 |
Authors . | Year . | Pericardium . | Preconditioning . | Testing . | Modulus . | UTS . | Strain at fracture (%) . |
---|---|---|---|---|---|---|---|
Naimark et al. | 1992 | Native | Cyclic loading | Stress–strain response, stress relaxation, and forced vibration | 41 ± 3 MPa at 0.1 Hz | – | – |
46 ± 4 MPa at 1 Hz | – | – | |||||
43 ± 4 MPa at 10 Hz | – | – | |||||
Garcia Páez et al. | 2001 | GA-treated | – | Hydraulic simulator | – | 43.29–63.01 MPa | – |
Chloroform/methanol | – | 28.93–42.30 MPa | – | ||||
SDS | – | 13.49–19.06 MPa | – | ||||
Garcia Páez et al. | 2001 | GA-treated (different regions, different zones) | – | Hydraulic simulator | – | 64.38 ± 17.59 MPaa | |
Garcia Páez et al. | 2002 | GA-treated right ventricle | – | Uniaxial and biaxial loading | – | 61.15 ± 23.31 MPab | – |
GA-treated left ventricle | – | 67.12 ± 37.46 MPab | – | ||||
Garcia Páez et al. | 2003 | GA-treated (Gore-Tex sutured) | – | Uniaxial loading | – | 10.46 ± 2.70 MPac | – |
– | 9.85 ± 3.09 MPad | – | |||||
– | 14.00 ± 3.50 MPae | – | |||||
Tedder et al. | 2011 | Native | Cyclic loading | Biaxial loading | – | – | – |
GA-treated | |||||||
PGG-treated | |||||||
Gauvin et al. | 2012 | GA-treated | Cyclic loading | Uniaxial loading | ∼100 MPa | ∼12 MPa | ∼24 |
Arbeiter et al. | 2012 | Native | – | Uniaxial loading | 133 ± 15 MPa | 9.4 ± 1.7 MPa | 20 ± 1.1 |
GA-treated | 47 ± 9 MPa | 9.5 ± 1.9 MPa | 49.5 ± 1.7 |
aHighest result.
bBiaxial stepwise.
cLeft 45°.
dRight 90°.
eCentre.
GA: glutaraldehyde; UTS: ultimate tensile strength; SDS: sodium dodecyl sulphate; PGG: penta-galloyl glucose.
Biochemical and structural characterization of porcine pericardium was proposed by Braga-Vilela et al. [31]. They found the presence of a network of relatively fine collagen fibres in multidirectionally oriented layers. Collagen in porcine pericardium constitutes almost 50% of the matrix.
Fresh and treated porcine pericardia were compared with aortic valves harvested from domestic pigs, and mechanically assessed by uniaxial tensile tests [32]. Results showed a different behaviour of native pericardium with respect to aortic valvular leaflets. The fixation process was shown to influence the properties of native pericardium, which is stiffer than valvular tissue. By fixing native pericardium with GA, its mechanical properties shifted to a more elastic behaviour.
An interesting paper by Naimark et al. [33] was aimed at investigating differences among pericardia from calves, dogs, pigs and sheep. Thinner canine and porcine pericardia were found to be significantly stiffer than thicker bovine and ovine tissues, but equivalently viscoelastic. Differences were justified on the basis of different levels of Type III collagen combined with a different degree of cross-linking.
GA-TREATED BOVINE AND PORCINE PERICARDIUM
In 1982, GA-treated bovine pericardium was investigated by means of uniaxial stress/strain tests [34]. Significant alterations of mechanical properties due to GA were observed. Authors concluded that a valve made from such a tissue may be stenotic and possibly prone to early failure.
A very interesting paper was published by Trowbridge et al. in 1985 [35]: it discussed the way soft tissues are mechanically tested and modelled, and clarified both the terminology and analytical inconsistencies of earlier studies. Effects of cyclic preconditioning and of different positions of samples from different pericardia were considered. Authors stated that ‘GA fixed pericardium can be modelled, to a good approximation, as an isotropic compressible elastic material that undergoes a transition to a second elastic material governed by a potential energy function of different magnitude but the same functional form as that associated with the initial elastic material. There is no experimental evidence of anisotropy’. Isotropy of the GA-treated bovine pericardium was also underlined by Lee et al. [36], while Zioupos et al. [26] denied that the treatment modifies the anisotropy of native tissue.
Further non-destructive and destructive uniaxial loading tests were performed on native and GA-treated bovine pericardium by Crofts and Trowbridge [37], who proposed that chemical modification could increase tensile strength: variations in tensile strength and strain at fracture are of an anatomical nature and are due to elastin content. GA fixation increases the percentage strain at fracture (UTS: 27.17 ± 1.39% native vs 46.05 ± 1.54% GA-fixed).
A hydraulic simulator reproducing the mechanical behaviour of pericardial membranes under continuous flow was proposed to assess GA-treated calf pericardium [38]. Pericardial patches were exposed to increasing pressure (from 0 to 16 atm). The upper zones of calf pericardium, corresponding to either the right or left ventricle, showed best mean results at rupture (60 MPa). The expected stress for an elongation of 30% was 1.12 MPa. By combining morphological criteria with mechanical behaviour, excellent mathematical fits were implemented to predict the tensile strength.
The mechanical behaviour of calf, pig and ostrich pericardia was compared by Garcia Páez et al. [39]. They stated that collagen quality and quantity determine the mechanical resistance of different types of pericardium. From the same group, uniaxial and biaxial tensile tests on GA-treated bovine and porcine pericardia were performed to investigate the mechanical impact of repeated or stepwise increments in loading [38, 40, 41]. Alternatively, GA-treated bovine pericardium was tested under cyclic load: deterioration of collagen begins immediately, whereas fibre straightening and reorientation dominates changes in the mechanical behaviour after billions of cycles [42].
The relation between collagen fibres’ preferred orientation and the resulting flexural properties of native and GA-treated bovine pericardium was studied [43]: flexural properties are dominated by inter-fibre cross-links rather than by the stiffness of collagen fibres themselves.
Implementing the fixation process influences the properties of native pericardium towards the biomechanical behaviour of aortic valvular leaflet tissue. Langdon et al. [44] investigated the effect of biaxial constraint during GA cross-linking on equibiaxial mechanical properties of bovine pericardium. Authors found that several mechanical properties can be significantly modulated by applying stress (or strain) during cross-linking. Mean strains and tensile moduli were calculated under 20 and 150 kPa biaxial stresses for both base-to-apex and circumferential specimens. The highest strain (20.8 ± 1.4%) was gained by fresh circumferential samples under 150 kPa. The highest modulus was reached after 200 g initial load by base-to-apex samples under both 20 and 150 kPa.
Other authors proposed dynamic fixation protocols and mechanically characterized treated tissues [45]. It was hypothesized that elastin modulates ‘the biomechanical properties by limiting the degree of reorientation of the collagen geometry under both low and high strains when collagen fibres start to align in an extended configuration’. GA fixation of elastin fibres resulted in a loss of elastic recovery and reduced modulus: this effect explains the lower extensibilities of high-pressure fixed pericardial tissue compared with fresh or non-pressure fixed samples.
Besides its effects on mechanical behaviour, GA makes pericardial tissue prone to cytotoxicity and calcification. Therefore, alternative fixation procedures were recommended [46–50].
In the study of Chang et al. [47], no significant change in the mechanical behaviour of cellular and acellular tissues was caused by treatments with GA and genipin. The latter is a naturally occurring cross-linking agent significantly less cytotoxic than GA. Decellularized porcine pericardium was treated with penta-galloyl glucose (PGG), a collagen-binding polyphenol, and tested for biodegradation, biaxial mechanical properties and in vivo biocompatibility [51]. PGG-treated pericardium exhibited excellent biaxial mechanical properties, did not calcify in vivo and supported infiltration by host fibroblasts.
DECELLULARIZED PERICARDIUM
After GA treatments, structural valve deterioration occur [52]. Recently, it has been found that GA is not so efficient in removing animal-specific antigens as was previously believed [53, 54]. This evidence confirms the need of alternative reagents/procedures for the chemical treatment of biological tissues. The employment of decellularization procedures has been explored, that is, the selective elimination of all cellular components and nucleic residues that could evoke immunological reaction, while maintaining optimal ECM composition and structure. Major decellularization procedures are based on t-octylphenoxypolyethoxyethanol (Triton-X100), sodium dodecyl sulphate (SDS) and combinations of SDS, Triton-X100 and deoxycholic acid.
The first attempt to decellularize pericardium was made by Courtman et al. in 1994 [55]. The procedure was based on a combination of hypotonic buffer, Triton-X100 and nucleases, and provided a scaffold with few cell remnants, normal collagen and elastin structures, and an increased thickness of the decellularized tissue compared with the fresh one. Authors observed the presence of ‘open spaces’ in the matrix due to the removal of cells and selective extraction of elastin and other proteins. Nevertheless, the mechanical characterization of the decellularized tissue confirmed the persistence of the same properties of fresh pericardium, with the exception of a slightly increased stress relaxation (fracture tension: 2.25 ± 0.36 vs 2.10 ± 0.49 kN/m; strain at fracture: 30.1 ± 1.0 vs 28.7 ± 1.1%; remaining stress: 64.9 ± 1.7 vs 59.9 ± 2.1%, for native and decellularized tissue, respectively). This outcome was lately confirmed by Bai et al. [56] who investigated the feasibility of the use of decellularized bovine pericardium for guided bone regeneration. The proposed decellularization procedure was further employed by many other groups to evaluate the biocompatibility of acellular bovine pericardia fixed with genipin [47] or to describe scaffold biocompatibility [57].
In his pioneering paper, Courtman et al. [55] described the use of SDS resulting in a detrimental effect on tissue architecture. Nonetheless, the application of a decellularization procedure based on a low concentration of SDS (0.1%) and nucleases both on human [55] and bovine [45, 58] pericardia caused the production of acellular scaffolds with well-preserved ultrastructure and biomechanical features. Transitional stress, average transitional strain, average UTS and average failure strain showed no significant difference between native human and decellularized samples: a significant decrease in the elastic phase slope was observed in samples cut parallel to the visualized collagen bundles [58].
Vinci et al. [59] investigated the mechanical compliance and immunological compatibility of SDS decellularized cryopreserved human pericardium. The decellularization was applied on pericardial tissue from cadavers allowing the complete removal of cells without affecting ECM structure and preserving its mechanical properties and immunological tolerance.
The effects of different GA concentrations on fresh and decellularized pericardia were compared: the highest UTS value (16.205 ± 4.169 MPa) pertained to the fresh pericardium after 0.05% GA treatment; the highest failure strain (90.79 ± 9.471%) to the decellularized pericardium after treatment with 0.5% GA; and the highest slope of the collagen phase (0.099 ± 0.038 GPa) to the fresh and untreated pericardium [45].
The mechanical features of bovine pericardium decellularized with a dimethyl sulfoxide based procedure and treated with GA have been compared with those of conventional pericardium. The latter demonstrated a rupture tension greater than the decellularized pericardium; other mechanical parameters were found equal. This suggests the possible utilization of decellularized pericardium as a valued alternative to current biomaterials [60].
Some papers compared different decellularization methods. In [61] three decellularization protocols were studied, based on: (i) a non-ionic detergent, tridecyl alcohol ethoxylate (ATE); (ii) an alkaline swelling treatment and ATE; (iii) the Courtman's method: hypotonic buffer, Triton-X100 and nucleases [55]. All methods had similar effects in terms of decellularization effectiveness; they caused no statistical difference in tensile strength and strain at rupture in the treated tissues. Stress relaxation was reduced after decellularization.
A procedure based on the combination of SDS, TRITOX-X100 and deoxycholic acid was proposed by Pagoulatou et al. [49]. Pericardial samples with different orientations and commercial products were compared. The decellularized pericardial tissues maintained original mechanical strength. On the other hand, trypsin and ethylenediaminetetraacetic acid (EDTA) did not preserve original mechanical behaviour.
Few attempts to decellularize porcine pericardium have been made. In [48], the effects of chemical treatments (GA for tissue cross-linking, chloroform/methanol for lipid extraction to avoid tissue mineralization/calcification and SDS as a decellularizing agent) on porcine pericardium were studied: the mean breaking strength was significantly higher in the samples treated with GA (between 43.29 and 63.01 MPa) when compared with those treated with chloroform/methanol (29.92–42.30 MPa) or with SDS (13.49–19.06 MPa). Interestingly, a significant loss of breaking strength in tissue samples treated with SDS was detected, depending on the region of the pericardium considered.
Bovine pericardium decellularized with methods based on SDS-deoxycholic acid or trypsin/EDTA showed similar elastic moduli of GA-treated bovine pericardium [62]. This is a further confirmation of the above-described biomechanical features of decellularized pericardium: this outcome supports the utilization of bovine decellularized pericardium as biomaterial for heart valve engineering.
OTHER MAMMALIAN SPECIES
The mechanical behaviour of pericardia from mammalian species other than pig, calf and humans was investigated, too. Major data on the mechanical characterization of ostrich, dog and donkey pericardium are presented in Tables 5–7.
Authors . | Year . | Pericardium . | Preconditioning . | Testing . | Modulus . | UTS . | Strain at fracture . |
---|---|---|---|---|---|---|---|
García Páez et al. | 2001 | GA-treated and sutured and different zones | – | Hydraulic simulator | – | Lefta 56.84 ± 8.97 MPa | – |
Righta 58.68 ± 11.69 MPa | |||||||
Centrea 55.92 ± 22.69 MPa | |||||||
Leftb 22.52 ± 5.21 MPa | |||||||
Rightb 23.66 ± 4.85 MPa | |||||||
Centreb 25.36 ± 6.17 MPa | |||||||
Leftc 18.38 ± 3.94 MPa | |||||||
Rightc 16.95 ± 2.21 MPa | |||||||
Centrec 20.96 ± 11.59 MPa | |||||||
Leftd 14.54 ± 5.58 MPa | |||||||
Rightd 13.84 ± 2.62 MPa | |||||||
Centred 16.47 ± 3.87 MPa | |||||||
Lefte 17.77 ± 6.87 MPa | |||||||
Righte 18.36 ± 5.90 MPa | |||||||
Centree 16.16 ± 4.40 MPa | |||||||
García Páez et al. | 2003 | GA-treated (Gore-Tex sutured) | – | Uniaxial loading | – | 21.81 ± 2.21 MPaf | – |
– | 20.81 ± 5.84 MPag | – | |||||
– | 32.42 ± 7.50 MPah | – | |||||
Martin-Maestro et al. | 2006 | GA-treated | – | Uniaxial loading | – | 32.4 ± 7.5 MPa | – |
Authors . | Year . | Pericardium . | Preconditioning . | Testing . | Modulus . | UTS . | Strain at fracture . |
---|---|---|---|---|---|---|---|
García Páez et al. | 2001 | GA-treated and sutured and different zones | – | Hydraulic simulator | – | Lefta 56.84 ± 8.97 MPa | – |
Righta 58.68 ± 11.69 MPa | |||||||
Centrea 55.92 ± 22.69 MPa | |||||||
Leftb 22.52 ± 5.21 MPa | |||||||
Rightb 23.66 ± 4.85 MPa | |||||||
Centreb 25.36 ± 6.17 MPa | |||||||
Leftc 18.38 ± 3.94 MPa | |||||||
Rightc 16.95 ± 2.21 MPa | |||||||
Centrec 20.96 ± 11.59 MPa | |||||||
Leftd 14.54 ± 5.58 MPa | |||||||
Rightd 13.84 ± 2.62 MPa | |||||||
Centred 16.47 ± 3.87 MPa | |||||||
Lefte 17.77 ± 6.87 MPa | |||||||
Righte 18.36 ± 5.90 MPa | |||||||
Centree 16.16 ± 4.40 MPa | |||||||
García Páez et al. | 2003 | GA-treated (Gore-Tex sutured) | – | Uniaxial loading | – | 21.81 ± 2.21 MPaf | – |
– | 20.81 ± 5.84 MPag | – | |||||
– | 32.42 ± 7.50 MPah | – | |||||
Martin-Maestro et al. | 2006 | GA-treated | – | Uniaxial loading | – | 32.4 ± 7.5 MPa | – |
aNon-sutured pericardium.
bPericardium sutured with silk.
cPericardium sutured with Prolene.
dPericardium sutured with nylon.
ePericardium sutured with Gore-Tex.
fLeft 45°.
gRight 90°.
hCentre.
GA: glutaraldehyde; UTS: ultimate tensile strength.
Authors . | Year . | Pericardium . | Preconditioning . | Testing . | Modulus . | UTS . | Strain at fracture . |
---|---|---|---|---|---|---|---|
García Páez et al. | 2001 | GA-treated and sutured and different zones | – | Hydraulic simulator | – | Lefta 56.84 ± 8.97 MPa | – |
Righta 58.68 ± 11.69 MPa | |||||||
Centrea 55.92 ± 22.69 MPa | |||||||
Leftb 22.52 ± 5.21 MPa | |||||||
Rightb 23.66 ± 4.85 MPa | |||||||
Centreb 25.36 ± 6.17 MPa | |||||||
Leftc 18.38 ± 3.94 MPa | |||||||
Rightc 16.95 ± 2.21 MPa | |||||||
Centrec 20.96 ± 11.59 MPa | |||||||
Leftd 14.54 ± 5.58 MPa | |||||||
Rightd 13.84 ± 2.62 MPa | |||||||
Centred 16.47 ± 3.87 MPa | |||||||
Lefte 17.77 ± 6.87 MPa | |||||||
Righte 18.36 ± 5.90 MPa | |||||||
Centree 16.16 ± 4.40 MPa | |||||||
García Páez et al. | 2003 | GA-treated (Gore-Tex sutured) | – | Uniaxial loading | – | 21.81 ± 2.21 MPaf | – |
– | 20.81 ± 5.84 MPag | – | |||||
– | 32.42 ± 7.50 MPah | – | |||||
Martin-Maestro et al. | 2006 | GA-treated | – | Uniaxial loading | – | 32.4 ± 7.5 MPa | – |
Authors . | Year . | Pericardium . | Preconditioning . | Testing . | Modulus . | UTS . | Strain at fracture . |
---|---|---|---|---|---|---|---|
García Páez et al. | 2001 | GA-treated and sutured and different zones | – | Hydraulic simulator | – | Lefta 56.84 ± 8.97 MPa | – |
Righta 58.68 ± 11.69 MPa | |||||||
Centrea 55.92 ± 22.69 MPa | |||||||
Leftb 22.52 ± 5.21 MPa | |||||||
Rightb 23.66 ± 4.85 MPa | |||||||
Centreb 25.36 ± 6.17 MPa | |||||||
Leftc 18.38 ± 3.94 MPa | |||||||
Rightc 16.95 ± 2.21 MPa | |||||||
Centrec 20.96 ± 11.59 MPa | |||||||
Leftd 14.54 ± 5.58 MPa | |||||||
Rightd 13.84 ± 2.62 MPa | |||||||
Centred 16.47 ± 3.87 MPa | |||||||
Lefte 17.77 ± 6.87 MPa | |||||||
Righte 18.36 ± 5.90 MPa | |||||||
Centree 16.16 ± 4.40 MPa | |||||||
García Páez et al. | 2003 | GA-treated (Gore-Tex sutured) | – | Uniaxial loading | – | 21.81 ± 2.21 MPaf | – |
– | 20.81 ± 5.84 MPag | – | |||||
– | 32.42 ± 7.50 MPah | – | |||||
Martin-Maestro et al. | 2006 | GA-treated | – | Uniaxial loading | – | 32.4 ± 7.5 MPa | – |
aNon-sutured pericardium.
bPericardium sutured with silk.
cPericardium sutured with Prolene.
dPericardium sutured with nylon.
ePericardium sutured with Gore-Tex.
fLeft 45°.
gRight 90°.
hCentre.
GA: glutaraldehyde; UTS: ultimate tensile strength.
Authors . | Year . | Pericardium . | Preconditioning . | Testing . | Modulus . | UTS . | Strain at fracture (%) . |
---|---|---|---|---|---|---|---|
Hildebrandt et al. | 1969 | Native strips and sheets | Cyclic loading | Stress relaxation, creep, fracture | – | – | – |
Wiegner et al. | 1981 | Native | Cyclic loading (10 stretch/release cycles) | Stress relaxation, creep, fracture | 14.5 ± 3.2 g/mm2a | – | – |
53.8 ± 12.9 g/mm2b | |||||||
210 ± 31 g/mm2c | |||||||
383 ± 41 g/mm2d | |||||||
Lee and Boughner | 1981 | Vertical stripse | Cyclic loading (from 0 to 16 or 500 g) | Stress relaxation, creep, strain rate, fracture | 235 ± 21 (107 dynes/cm2) | 21.4 ± 1.4 (107 dynes/cm2) | 22.0 ± 1.9 |
Horizontal stripse | 168 ± 5 (107 dynes/cm2) | 14.8 ± 1.2 (107 dynes/cm2) | 22.8 ± 1.4 | ||||
Lee and Boughner | 1985 | Native | Cyclic loading (10 mm/min between 0 load and a maximum load of 40 or 500 g) | Stress relaxation, creep, fracture | 198 ± 20 MN/m2 | 18.4 ± 1.5 MN/m2 | 21.4 ± 1.9 |
Lee et al. | 1987 | Native | Cyclic isotropic loading (∼10 times) | Stress relaxation, creep, fracture | – | – | – |
Cohn et al. | 1987 | Native | – | Uniaxial loading | 223.8 MPa | 23.352 MPa | – |
Naimark et al. | 1992 | Native | Cyclic loading | Stress–strain response, stress relaxation, and forced vibration | 29 ± 2 MPa at 0.1 Hz | – | – |
32 ± 2 MPa at 1 Hz | – | – | |||||
34 ± 2 MPa at 10 Hz | – | – |
Authors . | Year . | Pericardium . | Preconditioning . | Testing . | Modulus . | UTS . | Strain at fracture (%) . |
---|---|---|---|---|---|---|---|
Hildebrandt et al. | 1969 | Native strips and sheets | Cyclic loading | Stress relaxation, creep, fracture | – | – | – |
Wiegner et al. | 1981 | Native | Cyclic loading (10 stretch/release cycles) | Stress relaxation, creep, fracture | 14.5 ± 3.2 g/mm2a | – | – |
53.8 ± 12.9 g/mm2b | |||||||
210 ± 31 g/mm2c | |||||||
383 ± 41 g/mm2d | |||||||
Lee and Boughner | 1981 | Vertical stripse | Cyclic loading (from 0 to 16 or 500 g) | Stress relaxation, creep, strain rate, fracture | 235 ± 21 (107 dynes/cm2) | 21.4 ± 1.4 (107 dynes/cm2) | 22.0 ± 1.9 |
Horizontal stripse | 168 ± 5 (107 dynes/cm2) | 14.8 ± 1.2 (107 dynes/cm2) | 22.8 ± 1.4 | ||||
Lee and Boughner | 1985 | Native | Cyclic loading (10 mm/min between 0 load and a maximum load of 40 or 500 g) | Stress relaxation, creep, fracture | 198 ± 20 MN/m2 | 18.4 ± 1.5 MN/m2 | 21.4 ± 1.9 |
Lee et al. | 1987 | Native | Cyclic isotropic loading (∼10 times) | Stress relaxation, creep, fracture | – | – | – |
Cohn et al. | 1987 | Native | – | Uniaxial loading | 223.8 MPa | 23.352 MPa | – |
Naimark et al. | 1992 | Native | Cyclic loading | Stress–strain response, stress relaxation, and forced vibration | 29 ± 2 MPa at 0.1 Hz | – | – |
32 ± 2 MPa at 1 Hz | – | – | |||||
34 ± 2 MPa at 10 Hz | – | – |
aDuring stretching at 0.3 g/mm2.
bDuring stretching at 1 g/mm2.
cDuring stretching at 3 g/mm2.
dDuring stretching at 5 g/mm2.
eTested at 37°C in Hanks' solution.
UTS: ultimate tensile strength.
Authors . | Year . | Pericardium . | Preconditioning . | Testing . | Modulus . | UTS . | Strain at fracture (%) . |
---|---|---|---|---|---|---|---|
Hildebrandt et al. | 1969 | Native strips and sheets | Cyclic loading | Stress relaxation, creep, fracture | – | – | – |
Wiegner et al. | 1981 | Native | Cyclic loading (10 stretch/release cycles) | Stress relaxation, creep, fracture | 14.5 ± 3.2 g/mm2a | – | – |
53.8 ± 12.9 g/mm2b | |||||||
210 ± 31 g/mm2c | |||||||
383 ± 41 g/mm2d | |||||||
Lee and Boughner | 1981 | Vertical stripse | Cyclic loading (from 0 to 16 or 500 g) | Stress relaxation, creep, strain rate, fracture | 235 ± 21 (107 dynes/cm2) | 21.4 ± 1.4 (107 dynes/cm2) | 22.0 ± 1.9 |
Horizontal stripse | 168 ± 5 (107 dynes/cm2) | 14.8 ± 1.2 (107 dynes/cm2) | 22.8 ± 1.4 | ||||
Lee and Boughner | 1985 | Native | Cyclic loading (10 mm/min between 0 load and a maximum load of 40 or 500 g) | Stress relaxation, creep, fracture | 198 ± 20 MN/m2 | 18.4 ± 1.5 MN/m2 | 21.4 ± 1.9 |
Lee et al. | 1987 | Native | Cyclic isotropic loading (∼10 times) | Stress relaxation, creep, fracture | – | – | – |
Cohn et al. | 1987 | Native | – | Uniaxial loading | 223.8 MPa | 23.352 MPa | – |
Naimark et al. | 1992 | Native | Cyclic loading | Stress–strain response, stress relaxation, and forced vibration | 29 ± 2 MPa at 0.1 Hz | – | – |
32 ± 2 MPa at 1 Hz | – | – | |||||
34 ± 2 MPa at 10 Hz | – | – |
Authors . | Year . | Pericardium . | Preconditioning . | Testing . | Modulus . | UTS . | Strain at fracture (%) . |
---|---|---|---|---|---|---|---|
Hildebrandt et al. | 1969 | Native strips and sheets | Cyclic loading | Stress relaxation, creep, fracture | – | – | – |
Wiegner et al. | 1981 | Native | Cyclic loading (10 stretch/release cycles) | Stress relaxation, creep, fracture | 14.5 ± 3.2 g/mm2a | – | – |
53.8 ± 12.9 g/mm2b | |||||||
210 ± 31 g/mm2c | |||||||
383 ± 41 g/mm2d | |||||||
Lee and Boughner | 1981 | Vertical stripse | Cyclic loading (from 0 to 16 or 500 g) | Stress relaxation, creep, strain rate, fracture | 235 ± 21 (107 dynes/cm2) | 21.4 ± 1.4 (107 dynes/cm2) | 22.0 ± 1.9 |
Horizontal stripse | 168 ± 5 (107 dynes/cm2) | 14.8 ± 1.2 (107 dynes/cm2) | 22.8 ± 1.4 | ||||
Lee and Boughner | 1985 | Native | Cyclic loading (10 mm/min between 0 load and a maximum load of 40 or 500 g) | Stress relaxation, creep, fracture | 198 ± 20 MN/m2 | 18.4 ± 1.5 MN/m2 | 21.4 ± 1.9 |
Lee et al. | 1987 | Native | Cyclic isotropic loading (∼10 times) | Stress relaxation, creep, fracture | – | – | – |
Cohn et al. | 1987 | Native | – | Uniaxial loading | 223.8 MPa | 23.352 MPa | – |
Naimark et al. | 1992 | Native | Cyclic loading | Stress–strain response, stress relaxation, and forced vibration | 29 ± 2 MPa at 0.1 Hz | – | – |
32 ± 2 MPa at 1 Hz | – | – | |||||
34 ± 2 MPa at 10 Hz | – | – |
aDuring stretching at 0.3 g/mm2.
bDuring stretching at 1 g/mm2.
cDuring stretching at 3 g/mm2.
dDuring stretching at 5 g/mm2.
eTested at 37°C in Hanks' solution.
UTS: ultimate tensile strength.
Authors . | Year . | Pericardium . | Preconditioning . | Testing . | Modulus . | UTS . | Strain at fracture . |
---|---|---|---|---|---|---|---|
Chen et al. | 2013 | Decell GA-treated | – | Uniaxial loading | 81.67 ± 20.41 MPa | 21.64 ± 7.02 kN/m | – |
Authors . | Year . | Pericardium . | Preconditioning . | Testing . | Modulus . | UTS . | Strain at fracture . |
---|---|---|---|---|---|---|---|
Chen et al. | 2013 | Decell GA-treated | – | Uniaxial loading | 81.67 ± 20.41 MPa | 21.64 ± 7.02 kN/m | – |
GA: glutaraldehyde; UTS: ultimate tensile strength.
Authors . | Year . | Pericardium . | Preconditioning . | Testing . | Modulus . | UTS . | Strain at fracture . |
---|---|---|---|---|---|---|---|
Chen et al. | 2013 | Decell GA-treated | – | Uniaxial loading | 81.67 ± 20.41 MPa | 21.64 ± 7.02 kN/m | – |
Authors . | Year . | Pericardium . | Preconditioning . | Testing . | Modulus . | UTS . | Strain at fracture . |
---|---|---|---|---|---|---|---|
Chen et al. | 2013 | Decell GA-treated | – | Uniaxial loading | 81.67 ± 20.41 MPa | 21.64 ± 7.02 kN/m | – |
GA: glutaraldehyde; UTS: ultimate tensile strength.
Canine pericardium was mechanically assessed and compared with human pericardium as it has been previously reported [13, 14]. Dog pericardium was previously investigated by Hildebrandt et al. in 1969 [63]. They developed a method for analysing both uniaxial and uniform biaxial strain data obtained from nearly isotropic tissues. A further attempt to correlate mechanical and structural properties of canine pericardium was published in 1981 by Wiegner et al. [64]. They measured stiffness, stress relaxation and creep in samples from the anterior surface of 14 canine pericardia, assessing the viscoelastic properties within the physiological range of stresses and relating mechanical behaviour to fibre direction.
Lee and Boughner [65] investigated the mechanical behaviour of canine pericardium in different environments, giving evidence for time-dependent accommodation, absence of plasticity, and roles for collagen and elastin. Mechanical properties of isolated canine pericardium were tested at 37°C in Hanks' solution, and compared with similar tests at room temperature, both in Hanks' solution and kept moist with saline. They asserted that slowly applied loads produce an ‘accommodation effect’ as fibres rearrange through the viscous ground substance, but without any plasticity. This accommodation is produced by shifts in the stress–strain curve due to cyclic loading, stress relaxation and creep. Lee and Boughner argued that ‘Although the pericardium is clearly subject to cyclic loading and unloading during diastole and systole, respectively, the details of this regimen in vivo have not been studied’. This sentence raises doubts on the effects of preconditioning applied under not physiological conditions.
Ostrich pericardium was studied for the construction of bioprosthetic cardiac valve leaflets [48]: treated with GA, it showed excellent resistance to rupture in biaxial testing, withstanding stresses of up to 100 MPa, and never lower than 30 MPa.
In 2006, the group of García Páez and Jorge-Herrero [66] performed a further comparative analysis of GA-preserved ostrich pericardium. It was found that it is slightly thinner and has higher water content than bovine pericardium (70 ± 2 vs 62 ± 2%). Additionally, ostrich pericardium presents a 1.6-fold lower elastin content and a lower percentage of collagen in reference to the total protein content (68 ± 2 vs 76 ± 2%). Authors found that ostrich pericardium shows better mechanical properties, with higher tensile stress at rupture, than calf pericardium (32.4 ± 7.5 vs 11.5 ± 4.6). From the same research group, a comparison of the mechanical behaviour of biological tissues (pig, calf and ostrich pericardium sutured with Gore-Tex) had been previously investigated by uniaxial tensile testing [39]. The influence of polypropylene sutures and adhesives on GA-treated calf pericardium was investigated [67]. Several orientations and treatments were assessed. Interestingly, authors identified the so-called G point in the stress versus strain curves of the samples subjected to stepwise increases in loads. It is an inflection point that occurs at a load just beyond the final step, and was not observed in the samples subjected directly to rupture. Authors were not able to provide a precise explanation for this phenomenon.
Donkey pericardium was proposed as an alternative material for prosthetic heart valves [68]. The exploitation of this mammalian species is justified by restriction of bovine import in China. The characteristics of GA-treated acellular bovine and donkey pericardium were compared using histological analysis and electronic microscopy, shrinkage temperature and mechanical properties, as well as determining calcium and phosphorus content at 4 and 8 weeks after subcutaneous implantation. Despite the reduced thickness, mechanical testing showed no significant difference in maximum load and tear strength, and the tensile strength of donkey pericardium was higher than bovine. Therefore, donkey pericardium should be considered when choosing materials for bioprosthetic heart valves, also for its anticalcification effect.
Actually, equine pericardium has been exploited for the production of biological prosthetic heart valves. The experience of implantation of the 3-F aortic bioprosthesis (3F Therapeutics, Lake Forest, CA, USA) has been presented in the literature [69]: it is a stentless biological heart valve fabricated from three equal leaflets of equine pericardium, assembled in a tubular shape and implanted in the native aortic root to replace the patient's diseased aortic leaflets [59].
CONCLUSIONS
The future of heart valve surgery will be based on tissue-engineered heart valves (TEHVs) [70]. Tissue engineering is the only approach characterized by the unique potential for the creation of autologous tissues, with longer durability and fewer side effects. Although there is still a long way to go, TEHVs will definitely revolutionize cardiac surgery of the future [71].
As it clearly appears from the proposed literature review, the mechanical characterization of pericardial tissues as sources for the production of any prosthetic device lacks standardized protocols. Over the years, some authors characterized tissue samples by uniaxial and/or biaxial loading under static or dynamic conditions, at different loading rates, with or without preconditioning; stress relaxation, creep and flexural loading were also used to assess the behaviour of pericardia belonging to different animal species and differently treated. As a matter of fact, experimental data are hardly comparable and sometimes significant discordances arise. Thus, it is not possible to identify standard criteria to guide the selection of biological tissues for manufacturing prosthetic heart valves.
In spite of the above-mentioned limitations, we tried to compare mechanical data belonging to bovine (native, GA-treated, decellularized, and GA-treated plus decellularized) and porcine pericardium (native and GA-treated). Data had been originally obtained under different experimental conditions and expressed in different units: we converted the elastic modulus and the UTS into MPa and considered the strain at fracture (in %) (Fig. 1). With regard to the elastic modulus (Fig. 1A), native and GA-treated tissues exhibit quite similar behaviours: chemical fixation seems responsible for higher values, but differences are not that striking. Decellularization with different protocols by itself does not modify the performances of bovine pericardium, but GA fixation decreases the elastic modulus.
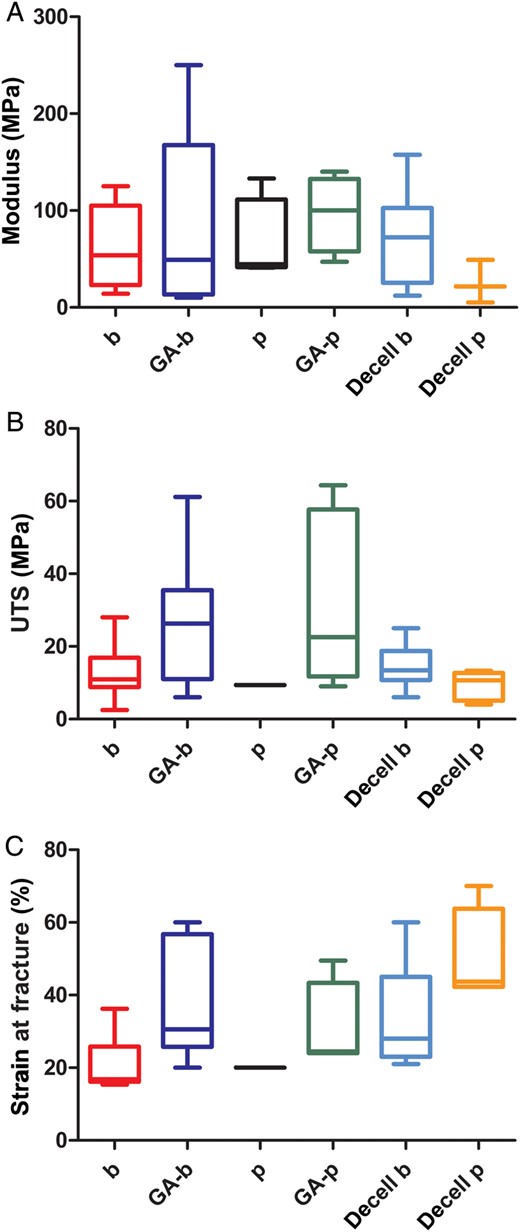
Comparison of the mechanical properties of: native bovine (b), GA-b, decell b pericardium; native porcine (p), GA-p, decell p pericardium. (A) Elastic modulus (MPa); (B) UTS (MPa); (C) strain at fracture (percent). GA-b: GA-treated bovine; decell b: decellularized bovine; GA-p: GA-treated porcine; decell p: decellularized porcine; UTS: ultimate tensile strength.
Similarly, UTS and strain at fracture values are slightly increased for treated pericardia (Fig. 1B and C), but without significant differences compared with those of native tissues. Interestingly, after GA fixation the resistance to rupture of bovine pericardium is markedly improved.
However, due to differences of experimental protocols, tools and conditions applied, the bulk of data available on the mechanical characterization of pericardial samples does not address the real contribution of GA treatment, decellularization and utilization of different animal species. The urgent need for standardized procedures and treatments to properly guide the choice of one tissue or another is demonstrated: it is mandatory in order to identify the ‘optimal’ biomaterial for TEHV construction.
Funding
The present work has been granted by the Padova Heart Project, CaRiPaRo Foundation (Padova, Italy).
Conflict of interest: none declared.