-
PDF
- Split View
-
Views
-
Cite
Cite
Geret DePiper, Sarah Gaichas, Brandon Muffley, Greg Ardini, Jeffrey Brust, Jessica Coakley, Kiley Dancy, G Warren Elliott, Dustin C Leaning, Douglas Lipton, Jason McNamee, Charles Perretti, Kirby Rootes-Murdy, Michael J Wilberg, Learning by doing: collaborative conceptual modelling as a path forward in ecosystem-based management, ICES Journal of Marine Science, Volume 78, Issue 4, August 2021, Pages 1217–1228, https://doi.org/10.1093/icesjms/fsab054
- Share Icon Share
Abstract
Managers, stakeholders, and scientists recognize the need for collaborative, transparent, integrated approaches to complex resource management issues, and frameworks to address these complex issues are developing. Through the course of 2019, the Mid-Atlantic Fishery Management Council developed a conceptual model of ecosystem linkages and risks for summer flounder, a species of recreational and commercial fisheries importance. The proximal aim of the model was to develop a list of integrated management questions that could be refined and addressed through a future quantitative management strategy evaluation. As such, this conceptual model served as a scoping tool. However, the true value of the conceptual model lays elsewhere: familiarizing resource managers historically focused on single-species management with the potential utility of an ecosystem approach to management. This paper details the goals and development of the conceptual model and situates this process in the broader context of best practices for collaborative open science and scientific reproducibility. Further, it highlights a successful path by which the shift towards ecosystem-based management can be actuated.
Introduction
The science underpinning ecosystem-based management (EBM) is well developed, as numerous surveys of the literature can attest (e.g. Long et al., 2015; Borja et al., 2016; Link and Browman, 2017). Despite this, and with some notable exceptions (e.g. Smith et al., 2017; Zador et al., 2017; Ramírez-Monsalve et al., 2021), there are very few examples in which ecosystem science has been explicitly used as the basis for a management decision, particularly at the level of a large marine ecosystem (LME). Research has identified a number of issues inhibiting the management uptake of EBM science, including the state of transdisciplinary science, capacity constraints, limits of statutory authority, and lack of appropriate scoping for management objectives and stakeholder concerns (Dickey-Collas, 2014; Harvey et al., 2017; Link and Browman, 2017; Piet et al., 2020).
EBM involves management of multiple human activities in changing environments, requiring interdisciplinary collaborations (Levin et al., 2016b; DePiper et al., 2017; Suryanarayanan et al., 2018). Open, transparent, reproducible science is critically important in support of EBM, both so scientists can work together across disciplines and to improve trust when science contributes to difficult decisions regarding public health or natural resources (Elliott and Resnik, 2019). Open science is a philosophy of free access to research results, data sets, and detailed methods (including computer code) to facilitate more transparent, rapid, and effective scientific progress (National Academies of Sciences, 2018).
In this article, we highlight work scoping ecosystem considerations in support of federal fisheries management in the US Mid-Atlantic. We pay particular attention to the role that conceptual modelling and the open science concepts of research efficiency, transparency, and reproducibility played in developing an understanding of the system shared between scientists, managers, and stakeholders. This manuscript is not the first to assess the role conceptual modelling and open science can play in supporting EBM (e.g. Harvey et al., 2016; Tai and Robinson, 2018; Borja et al., 2019; Broszeit et al., 2019; Powers and Hampton, 2019; Beck et al., 2020; Bastille et al., 2021). However, we add to the existing literature by exploring the manner in which these processes and concepts facilitated management uptake, as one of the few instances in which ecosystem science has been explicitly requested, and used, by managers to guide strategic decision-making at the level of an LME. Scientists, managers, and stakeholders need to learn how to concurrently develop, understand, and utilize ecosystem science. We believe conceptual modelling exercises, such as those described in this manuscript, present an important path by which the transition towards EBM can be actuated in a management context.
Before delving into the specifics of the case study, some background is warranted to better understand managers’ motivation for requesting the development of a conceptual model. Both fisheries and ecosystem management have been described as “wicked problems” where the problem definition varies across diverse stakeholders and optimal solutions do not exist (Rittel and Webber, 1973; Jentoft and Chuenpagdee, 2009; DeFries and Nagendra, 2017). Nevertheless, or perhaps because fisheries problems are already wicked, the Mid-Atlantic Fishery Management Council (Council) has been developing capacity for ecosystem science since 2006, with a formal management process adopted in 2016. This process began as an outshoot of a stakeholder visioning project which identified increased stakeholder engagement, scientific, and management transparency, and the importance of ecosystem processes as core focus areas. A description of this visioning process, as well as the path by which ecosystem science will be integrated into the Council management process, can be found in Muffley et al. (2021). Ultimately, the Council adopted an approach modelled off of NOAA’s Integrated Ecosystem Assessment process (Levin et al., 2008, 2009), whose steps include identifying management objectives, developing indicators to track system performance against those objectives, assessing system risk to achieving objectives, and testing the performance of strategies that aim to address the highest risks to system performance. The current manuscript focuses on the work conducted in transitioning from an ecosystem-level risk assessment, as described in Gaichas et al. (2018), to a Management Strategy Evaluation (MSE; Smith, 1994; Punt et al., 2016) aimed at mitigating these interacting system-level risks for the highest priority component(s) of the system.
The risk assessment tracked 11 ecological and 14 social risk elements applied across all Council-managed species. Each risk element was explicitly linked to management objectives and assessed using at least one indicator. Risk ranking criteria were developed for each element, with most mapping to status levels of low, low-moderate, moderate-high, and high risk. The risk elements, indicators, and criteria were all developed by the Council with input from stakeholders and scientists. The final risk assessment was delivered to and adopted by the Council in December 2017 and is updated on an annual basis.
The results of the risk assessment led the Council to focus on summer flounder (Paralichthys dentatus) for additional analysis, due to the fact that its fisheries faced the highest number of moderate-high and high risks and the substantial value of both the commercial and recreational fisheries. Table 1 presents the risk elements which were identified as either moderate-high or high for summer flounder in the ecosystem risk assessment, as detailed in Gaichas et al. (2018) and discussed below. Summer flounder (also known as fluke) is opportunistic piscivorous ambush predators whose range stretches from the Atlantic Coast of Florida, US through Nova Scotia, Canada, although they are most prevalent between the US states of North Carolina and Massachusetts (Collette and Klein-Macphee, 2002; Link et al., 2002). Their estuarine dependence means summer flounder population dynamics are likely impacted by the poor estuarine condition within their range (Able, 2005; EPA, 2012). In the United States, summer flounder is jointly managed between the Council and the Atlantic States Marine Fisheries Commission, as fishing occurs in both federal and state waters. At the time of the 2017 risk assessment, summer flounder was estimated to be in an overfishing status, although a more recent stock assessment updated the status to no overfishing occurring (NEFSC, 2019). Despite this change in status, the summer flounder population trend is downward with lower than average recent recruitment and concern for the health of this species remains.
Moderate high and high ranking risk elements for Summer Flounder from the Council’s 2017 ecosystem-level risk assessment
Risk element . | Risk score . | Risk definition . |
---|---|---|
F status | High | Fishing mortality greater than Fmsy. Indicates risk to achieving Optimal Yield. |
Distributional shift | Moderate high | High potential for distributional shifts, which can increase the risk of ineffective spatial catch allocation and risk to achieving of Optimal Yield. |
Estuarine habitat | High | Summer Flounder is categorized as an estuarine dependent species, and the estuarine condition is poor which increases the risk to achieving Optimal Yield. |
Management control | Moderate higha | Routine, but small to moderate overages in recreational catch, which indicates some lack of control in measuring and monitoring catch and thus increased risk of not attaining Optimal Yield. |
Technical interactions | Moderate higha | Commercial technical interactions with species managed outside of the Council, including protected species, can lead to accountability measures being triggered for other species and impacting the prosecution of the summer flounder fishery. |
Regulatory complexity | Higha | Recreational regulations of high complexity and frequent changes. Commercial regulations of moderate-high complexity with occasional changes. This complexity can lead to non-compliance and spill over effects into other fisheries. |
Discards | Higha | Discards, particularly regulatory discards, are a major concern for Council stakeholders and impact attainment of Optimal Yield as biological and economic waste. Regular but managed discards occur in the summer flounder recreational fishery. |
Allocation | Higha | Recent and ongoing Council discussion suggests concerns about the risk of not achieving Optimal Yield due to inefficient allocations both within and between the commercial and recreational fisheries. |
Commercial revenue | Moderate high | Significant long-term decreases in revenue, proxying for profits, indicates risk of not maximizing commercial value from the fishery. |
Recreational value | High | Significant recent decreases in angler effort, proxying for recreational welfare, indicates risk to maximizing recreational from the fishery. |
Commercial shoreside support | Moderate high | Significant recent decreases in the number of seafood wholesalers, packaging, and markets within Mid-Atlantic states impacts fishermen’s access to existing shoreside support infrastructure. |
Fleet diversity | Moderate high | Significant recent decreases in fleet diversity suggests changes in access to fishery resources. |
Seafood production | Higha | Significant recent decrease in commercial seafood production and long-term decrease in recreational seafood landings decreases the ability to optimize seafood production, which is identified as a benefit in the Magnuson-Stevens Act, the primary law governing marine fisheries management in U.S. federal waters (16U.S.C. 1801-1891d). |
Risk element . | Risk score . | Risk definition . |
---|---|---|
F status | High | Fishing mortality greater than Fmsy. Indicates risk to achieving Optimal Yield. |
Distributional shift | Moderate high | High potential for distributional shifts, which can increase the risk of ineffective spatial catch allocation and risk to achieving of Optimal Yield. |
Estuarine habitat | High | Summer Flounder is categorized as an estuarine dependent species, and the estuarine condition is poor which increases the risk to achieving Optimal Yield. |
Management control | Moderate higha | Routine, but small to moderate overages in recreational catch, which indicates some lack of control in measuring and monitoring catch and thus increased risk of not attaining Optimal Yield. |
Technical interactions | Moderate higha | Commercial technical interactions with species managed outside of the Council, including protected species, can lead to accountability measures being triggered for other species and impacting the prosecution of the summer flounder fishery. |
Regulatory complexity | Higha | Recreational regulations of high complexity and frequent changes. Commercial regulations of moderate-high complexity with occasional changes. This complexity can lead to non-compliance and spill over effects into other fisheries. |
Discards | Higha | Discards, particularly regulatory discards, are a major concern for Council stakeholders and impact attainment of Optimal Yield as biological and economic waste. Regular but managed discards occur in the summer flounder recreational fishery. |
Allocation | Higha | Recent and ongoing Council discussion suggests concerns about the risk of not achieving Optimal Yield due to inefficient allocations both within and between the commercial and recreational fisheries. |
Commercial revenue | Moderate high | Significant long-term decreases in revenue, proxying for profits, indicates risk of not maximizing commercial value from the fishery. |
Recreational value | High | Significant recent decreases in angler effort, proxying for recreational welfare, indicates risk to maximizing recreational from the fishery. |
Commercial shoreside support | Moderate high | Significant recent decreases in the number of seafood wholesalers, packaging, and markets within Mid-Atlantic states impacts fishermen’s access to existing shoreside support infrastructure. |
Fleet diversity | Moderate high | Significant recent decreases in fleet diversity suggests changes in access to fishery resources. |
Seafood production | Higha | Significant recent decrease in commercial seafood production and long-term decrease in recreational seafood landings decreases the ability to optimize seafood production, which is identified as a benefit in the Magnuson-Stevens Act, the primary law governing marine fisheries management in U.S. federal waters (16U.S.C. 1801-1891d). |
Items scored separately for commercial and recreational sector. Only highest-valued risk of the two presented for brevity.
Moderate high and high ranking risk elements for Summer Flounder from the Council’s 2017 ecosystem-level risk assessment
Risk element . | Risk score . | Risk definition . |
---|---|---|
F status | High | Fishing mortality greater than Fmsy. Indicates risk to achieving Optimal Yield. |
Distributional shift | Moderate high | High potential for distributional shifts, which can increase the risk of ineffective spatial catch allocation and risk to achieving of Optimal Yield. |
Estuarine habitat | High | Summer Flounder is categorized as an estuarine dependent species, and the estuarine condition is poor which increases the risk to achieving Optimal Yield. |
Management control | Moderate higha | Routine, but small to moderate overages in recreational catch, which indicates some lack of control in measuring and monitoring catch and thus increased risk of not attaining Optimal Yield. |
Technical interactions | Moderate higha | Commercial technical interactions with species managed outside of the Council, including protected species, can lead to accountability measures being triggered for other species and impacting the prosecution of the summer flounder fishery. |
Regulatory complexity | Higha | Recreational regulations of high complexity and frequent changes. Commercial regulations of moderate-high complexity with occasional changes. This complexity can lead to non-compliance and spill over effects into other fisheries. |
Discards | Higha | Discards, particularly regulatory discards, are a major concern for Council stakeholders and impact attainment of Optimal Yield as biological and economic waste. Regular but managed discards occur in the summer flounder recreational fishery. |
Allocation | Higha | Recent and ongoing Council discussion suggests concerns about the risk of not achieving Optimal Yield due to inefficient allocations both within and between the commercial and recreational fisheries. |
Commercial revenue | Moderate high | Significant long-term decreases in revenue, proxying for profits, indicates risk of not maximizing commercial value from the fishery. |
Recreational value | High | Significant recent decreases in angler effort, proxying for recreational welfare, indicates risk to maximizing recreational from the fishery. |
Commercial shoreside support | Moderate high | Significant recent decreases in the number of seafood wholesalers, packaging, and markets within Mid-Atlantic states impacts fishermen’s access to existing shoreside support infrastructure. |
Fleet diversity | Moderate high | Significant recent decreases in fleet diversity suggests changes in access to fishery resources. |
Seafood production | Higha | Significant recent decrease in commercial seafood production and long-term decrease in recreational seafood landings decreases the ability to optimize seafood production, which is identified as a benefit in the Magnuson-Stevens Act, the primary law governing marine fisheries management in U.S. federal waters (16U.S.C. 1801-1891d). |
Risk element . | Risk score . | Risk definition . |
---|---|---|
F status | High | Fishing mortality greater than Fmsy. Indicates risk to achieving Optimal Yield. |
Distributional shift | Moderate high | High potential for distributional shifts, which can increase the risk of ineffective spatial catch allocation and risk to achieving of Optimal Yield. |
Estuarine habitat | High | Summer Flounder is categorized as an estuarine dependent species, and the estuarine condition is poor which increases the risk to achieving Optimal Yield. |
Management control | Moderate higha | Routine, but small to moderate overages in recreational catch, which indicates some lack of control in measuring and monitoring catch and thus increased risk of not attaining Optimal Yield. |
Technical interactions | Moderate higha | Commercial technical interactions with species managed outside of the Council, including protected species, can lead to accountability measures being triggered for other species and impacting the prosecution of the summer flounder fishery. |
Regulatory complexity | Higha | Recreational regulations of high complexity and frequent changes. Commercial regulations of moderate-high complexity with occasional changes. This complexity can lead to non-compliance and spill over effects into other fisheries. |
Discards | Higha | Discards, particularly regulatory discards, are a major concern for Council stakeholders and impact attainment of Optimal Yield as biological and economic waste. Regular but managed discards occur in the summer flounder recreational fishery. |
Allocation | Higha | Recent and ongoing Council discussion suggests concerns about the risk of not achieving Optimal Yield due to inefficient allocations both within and between the commercial and recreational fisheries. |
Commercial revenue | Moderate high | Significant long-term decreases in revenue, proxying for profits, indicates risk of not maximizing commercial value from the fishery. |
Recreational value | High | Significant recent decreases in angler effort, proxying for recreational welfare, indicates risk to maximizing recreational from the fishery. |
Commercial shoreside support | Moderate high | Significant recent decreases in the number of seafood wholesalers, packaging, and markets within Mid-Atlantic states impacts fishermen’s access to existing shoreside support infrastructure. |
Fleet diversity | Moderate high | Significant recent decreases in fleet diversity suggests changes in access to fishery resources. |
Seafood production | Higha | Significant recent decrease in commercial seafood production and long-term decrease in recreational seafood landings decreases the ability to optimize seafood production, which is identified as a benefit in the Magnuson-Stevens Act, the primary law governing marine fisheries management in U.S. federal waters (16U.S.C. 1801-1891d). |
Items scored separately for commercial and recreational sector. Only highest-valued risk of the two presented for brevity.
The fishery has substantial commercial (primarily bottom trawl) and recreational sectors, with 60% of the allowable landings currently allocated to the commercial sector and 40% allocated to the recreational sector based on historical harvest levels. However, updated recreational survey data suggest that the proportions of harvest by sector are actually reversed, which has, in part, led to discussion of revisiting these allocations. Each sector allocation is further subdivided by state, with 11 states receiving a percentage share of the coastwide commercial quota or recreational harvest limit based on historical harvest records. Since 2014, all states within predefined subregions of the summer flounder range have been required to implement consistent management measures. These historical splits are being called into question due to a well-documented northward shift of the summer flounder population (Pinsky et al., 2013; Bell et al., 2015; Kleisner et al., 2017; Perretti and Thorson, 2019), creating a disconnect between the management system, and where the bulk of the population is located.
The Mid-Atlantic commercial trawl fishery is multispecies in nature. Some of the species commonly caught with summer flounder, such as windowpane flounder managed by the neighbouring New England Fishery Management Council, are subject to accountability measures which would impact the commercial summer flounder fishery, if triggered. The summer flounder trawl fishery is also known to interact with small cetaceans protected under the Marine Mammal Protection Act (16 U.S.C. §§1361-1383 b, 1401-1406, 1411-1421 h) and the Atlantic Sturgeon and species of sea turtles listed under the Endangered Species Act (16 U.S.C. §§1531-1544), which could impact operation of the fishery through management decisions outside Council purview. Commercial regulations promulgated by the Council itself were qualified by Council staff as moderate-high in complexity and subject to occasional updates, which can impact fishermen’s ability to comply with existing regulations. In the Mid-Atlantic region, there has been a significant long-term decrease in the commercial fishery’s seafood production and resultant revenues (Summer Flounder commercial revenue and landings have been relatively flat recently). In addition, significant recent decreases in shoreside support businesses and a reduction in the diversity of the summer flounder fleet suggest potential frailty in the production chain (Gaichas et al., 2018). Shoreside support businesses include seafood merchant wholesalers, seafood product preparation and packaging, and seafood markets across all Mid-Atlantic states. Given the multispecies nature of the fishery and the relatively flat landings of summer flounder in recent years, this trend is likely driven by economic dynamics outside of the directed summer flounder fishery. Nevertheless, the decrease in the number of support businesses has implications for the summer flounder fishery.
The stakeholder engagement process within the EAFM Risk Assessment highlighted the following concerns for the recreational fishery. Recreational fishing in the Mid-Atlantic has seen significant recent decreases in effort, as proxy for welfare generated, and seafood produced. Regulatory discards have been of increasing concern to stakeholders in the summer flounder recreational fishery over the past few years, due to the biological and economic waste implicit in these activities and equity concerns across fishery segments. Equity concerns arise from each state implementing different fishery regulations with respect to recreational fishing, meaning the size and possession limits, and months the fishery is open, cam differs across these state lines. These recreational regulations are of high complexity and are frequently updated, which impacts the ability of fishermen to comply with existing laws. There are routine, but small to moderate, overages in recreational harvest targets, indicating some lack of management control in this sector.
The additional work highlighted in this manuscript aimed at better understanding the risks and challenges faced by the fishery, and transition towards an MSE to assess what management actions best mitigate those risks and address the challenges faced. The work reviewed in this manuscript is thus an intermediate product, facilitating management transition towards a quantitative MSE. Conceptual modelling (Heemskerk et al., 2003; Levin et al., 2016b) played a key role in this transition from risk assessment towards MSE, particularly given its ability to refine key management questions while identifying the principal ecosystem interactions of interest. As such, the Council requested the development of a conceptual model for summer flounder fishery–ecosystem interactions, along with an inventory of data availability and gaps, applicable models, the relative importance of risk elements, and example management questions which could be addressed with this knowledge base. Whereas most models are built to address specific questions, this model was built in order to identify questions for further development. Given this tasking, the conceptual model functioned very much as a scoping tool.
The need for this scoping after the completion of the risk assessment originates from two interlinked issues. First, no explicit weights were utilized in ranking management objectives during the risk assessment, leading to ambiguity in interpretation of the results. Although not unique to the Council, the inability to weight objectives has a substantial impact on the utility of the raw risk assessment results. Secondly, the day-to-day business of the Council focuses on single-species management issues. When they exist, management actions aimed at addressing systematic issues are usually circumscribed by law, e.g. omnibus actions to protect essential fish habitat or ending overfishing. As such, there are very few instances in which fishery managers in the United States develop operational ecosystem-level action plans, including objectives, from scratch. Therefore, the Council was “learning by doing” in implementing its EAFM policy guidance for the first time, and the conceptual model was the instrument by which ecosystem-level information was operationalized as specific and answerable management questions for the summer flounder fishery.
In what follows, we discuss how open science concepts and conceptual modelling facilitated the scoping of ecosystem issues for the Council. In doing so, we present one viable path available to navigate implementation issues that have stymied the management uptake of ecosystem science to date.
Methods
An interdisciplinary, and interagency, technical work group was convened over the course of 2019 to develop a conceptual model and document data and model availability for summer flounder. The group included economists, ecologists, population biologists, MSE specialists, and importantly, fishery managers, and management staff both at the state and federal levels. We outline the conceptual model and the process by which it was developed below. We also present examples to facilitate discussion. However, given the complexity of the undertaking, and that interactivity of both the conceptual model and documentation was key to the successful management utilization of the conceptual model, we also point the reader to the original html version, which can be found at https://nefsc.github.io/READ-SSB-DePiper_Summer_Flounder_Conceptual_Models/sfconsmod_riskfactors_subplots.html (last accessed March 19, 2021). Viewing this site will greatly enhance the reader’s understanding of the work undertaken. All of the code and documentation, and information used in developing the models can be found at https://github.com/NEFSC/READ-SSB-DePiper_Summer_Flounder_Conceptual_Models (last accessed March 19, 2021) or Gaichas and DePiper (2020).
Initially, the work group struggled with the development of the conceptual model. This first hurdle stemmed from the fact that scientists traditionally start with a question and build a model tailored to answer that specific question. The request to develop questions from a model thus reverses the research process in a manner that makes many scientists unsure of where to begin. To facilitate the modelling, the work group decided to start with the high-level risks which were identified from the risk assessment (i.e. the reasons that summer flounder was picked for further analysis by the Council in the first place), and build the model out from those ecosystem components.
The diagram editor software Dia (Alexander et al., 2011) was used to sketch out the conceptual model in real time. Dia was selected due to it being freeware, its ease of use, and most importantly the fact that the model itself can be imported into R (R Core Team, 2019), which greatly expands the possibilities in terms of visual representation and utilization of the information more generally. The work group met virtually four times in plenary over the course of 2019. The work group also met in two subgroups: one dedicated to developing components associated with the physical environment, and one dedicated to developing the human dimension aspects, with each subgroup meeting twice. Of the 17 work group members, 8 contributed to the Human Dimensions subgroup, 6 contributed to the Physical Environment submodel, and 3 contributed to both to facilitate communication and ensure consistency in the approaches employed. The work group iterated between plenary and subgroup meetings, with strategic decisions typically made in the plenary meetings and much of the modelling work conducted during the subgroup meetings. The plenary meetings were also used to identify relevant linkages between the submodels to ensure full system coupling.
In the spirit of open science, it was decided early on that all components to the conceptual model would be documented using a support table, similar to the approach employed in DePiper et al. (2017). This support table included an entry for the model element being described, a justification for its inclusion, an indicator for whether data for the model existed, a short description of existing data, data products, and models, including references and links where available, and whether any spatial considerations were important for the element. An example entry into this table is presented in Table 2, and full documentation can be found at Gaichas and DePiper (2020).
Example entry in the Summer Flounder Conceptual Model detailing a single allocation issuea
Model element . | Justification for inclusion . | Data to support (Y/N/M) . | Data—if yes, identify and list out all data sources (including temporal resolution and series length), analyses, model, Council project, etc. . | Spatial component (North/South; inshore/offshore; other—specify) . |
---|---|---|---|---|
Biomass distributional shifts (North/South) and Fluke SSB | Many of the current allocation discussions have focused on reallocation scenarios that are being driven, in part, due to distribution shifts or range expansion of biomass. As the summer flounder biomass changes over time due to climate and/or stock rebuilding, consideration of biomass distribution in any allocation scheme will be needed. Current commercial summer flounder amendment considered, although not selected, stock distribution changes for potential reallocation schemes. | Y | NEFSC trawl survey and other state and multistate (i.e. NEAMAP) survey information; commercial ammendment DEIS; 2018 benchmark stock assessment | North/South |
Model element . | Justification for inclusion . | Data to support (Y/N/M) . | Data—if yes, identify and list out all data sources (including temporal resolution and series length), analyses, model, Council project, etc. . | Spatial component (North/South; inshore/offshore; other—specify) . |
---|---|---|---|---|
Biomass distributional shifts (North/South) and Fluke SSB | Many of the current allocation discussions have focused on reallocation scenarios that are being driven, in part, due to distribution shifts or range expansion of biomass. As the summer flounder biomass changes over time due to climate and/or stock rebuilding, consideration of biomass distribution in any allocation scheme will be needed. Current commercial summer flounder amendment considered, although not selected, stock distribution changes for potential reallocation schemes. | Y | NEFSC trawl survey and other state and multistate (i.e. NEAMAP) survey information; commercial ammendment DEIS; 2018 benchmark stock assessment | North/South |
See https://nefsc.github.io/READ-SSB-DePiper_Summer_Flounder_Conceptual_Models/sfconsmod_riskfactors_subplots.html (last accessed March 19, 2021) for full support table.
Example entry in the Summer Flounder Conceptual Model detailing a single allocation issuea
Model element . | Justification for inclusion . | Data to support (Y/N/M) . | Data—if yes, identify and list out all data sources (including temporal resolution and series length), analyses, model, Council project, etc. . | Spatial component (North/South; inshore/offshore; other—specify) . |
---|---|---|---|---|
Biomass distributional shifts (North/South) and Fluke SSB | Many of the current allocation discussions have focused on reallocation scenarios that are being driven, in part, due to distribution shifts or range expansion of biomass. As the summer flounder biomass changes over time due to climate and/or stock rebuilding, consideration of biomass distribution in any allocation scheme will be needed. Current commercial summer flounder amendment considered, although not selected, stock distribution changes for potential reallocation schemes. | Y | NEFSC trawl survey and other state and multistate (i.e. NEAMAP) survey information; commercial ammendment DEIS; 2018 benchmark stock assessment | North/South |
Model element . | Justification for inclusion . | Data to support (Y/N/M) . | Data—if yes, identify and list out all data sources (including temporal resolution and series length), analyses, model, Council project, etc. . | Spatial component (North/South; inshore/offshore; other—specify) . |
---|---|---|---|---|
Biomass distributional shifts (North/South) and Fluke SSB | Many of the current allocation discussions have focused on reallocation scenarios that are being driven, in part, due to distribution shifts or range expansion of biomass. As the summer flounder biomass changes over time due to climate and/or stock rebuilding, consideration of biomass distribution in any allocation scheme will be needed. Current commercial summer flounder amendment considered, although not selected, stock distribution changes for potential reallocation schemes. | Y | NEFSC trawl survey and other state and multistate (i.e. NEAMAP) survey information; commercial ammendment DEIS; 2018 benchmark stock assessment | North/South |
See https://nefsc.github.io/READ-SSB-DePiper_Summer_Flounder_Conceptual_Models/sfconsmod_riskfactors_subplots.html (last accessed March 19, 2021) for full support table.
Although spatial structure can be directly incorporated into models, the decision to document this structure in the supporting documentation derived from the fact that the relevant scale (state waters, federal jurisdiction, full LME) and orientation (south–north vs. west–east) differed depending on the exact element under consideration. As an example, although the centre of summer flounder biomass is moving from south to north, there are seasonal inshore-offshore (west–east) migrations undertaken as well. For parsimony, then, the work group felt documentation in the support table would generate less confusion than coupling models of alternate scope/orientation in a single conceptual model.
Besides being useful for internal work group purposes, the documentation was important on a number of external fronts. First, reproducibility and transparency are key tenants of the scientific process which should extend to qualitative modelling exercises (see, e.g. Aguinis and Solarino, 2019 for a recent discussion). The work group adopted a number of additional best practices in open science, and these practices are detailed where appropriate in discussions to come. Secondly, this model was developed within the Council process, to be used in support of federal fishery management. As such, it is subject to National Oceanic and Atmospheric Administration information quality guidelines for “…ensuring and maximizing the quality, objectivity, utility, and integrity of information which it disseminates”(Mid-Atlantic Fishery Management Council, 2017). Of particular relevance is national guidance on interpreted products (which would include conceptual models), which indicates that data and information sources should be clearly identified/documented.
Work group members added to the conceptual model by identifying ecosystem elements that affect, or are affected by, the risk elements detailed in Table 1. Links between the risk elements themselves were also defined within the model. As a first pass, individuals were free to add any components they deemed relevant. However, only elements that could be explicitly justified for inclusion were kept in the final conceptual model. Of note is that justification for the inclusion of an element was not predicated on published works or data availability. For example, increased discussion during Council meetings sufficed to include management inequities and communication and outreach as important system components for issues of compliance. In addition, the Council envisioned a gap analysis as part of the utility for the conceptual model, by identifying ecosystem components that were deemed important but for which no data existed. The justification was thus merely a step added to ensure the working group was satisfied that inclusion was predicated on sound reasoning. In this way, the model and documentation tables contributed to both scientifically-relevant and socially-relevant transparency (Elliott and Resnik, 2019); the “Justification for inclusion” entry explained why each model element was linked to a particular risk category, which in turn linked to management objectives defined by the Council in the EAFM Risk Assessment.
Once a first iteration of the conceptual model and its corresponding gap analysis was drafted, the work group began developing the management questions which could be addressed given the current state of knowledge. The Council originally requested a list of 10 management questions. A subset of the technical work group drafted two straw man questions to seed discussion prior to the first meeting to develop draft management questions. The first straw man focused on the biological system: “What are the mechanisms driving summer flounder distributional shifts and what are the biological, management and socioeconomic implications of this change?” The second straw-man focused on the social system: “What are the most significant impediments to maximizing commercial profits and recreational participation in the summer flounder fisheries?” These two straw-men encapsulated issues consistently raised by stakeholders and scientists. Straw-men have played an important role throughout the Council’s transition towards EAFM (DePiper et al., 2017), as an example of what is being requested of managers and a place from which to begin discussion. Over a 2-h meeting, a total of nine draft management questions were developed by the technical work group, each of which was generally focused on a different risk element. These draft questions were reviewed and refined over another week’s time based on the technical work group’s expert opinion.
The draft conceptual model and management questions were then iterated between the technical work group and the Council’s Ecosystem and Ocean Planning Committee (EOP), a large subset of the Council membership, with meetings open to the public. The use of conceptual models as a visual communication tool (Heemskerk et al., 2003), to develop common understanding (e.g. Klinger et al., 2017), to identify research needs and priorities (e.g. Karp et al., 2019), to scope questions (Levin et al., 2016a), and to outline quantitative models (e.g. Francis et al., 2018) were all reviewed and connected with the “refine” step of the Mid-Atlantic Council’s EAFM process (Gaichas et al., 2016; Muffley et al., 2021). The review of conceptual models was also explicit about what they were not developed to do. In fisheries science, quantitative population dynamics models are routinely used to support tactical management advice, so it was important to emphasize that this conceptual model was not designed to provide quantitative advice. This introduction helped to ensure that EOP members understood exactly what had been developed for their use, and how best to use the information in transitioning towards an MSE.
Both model and management question refinement was iterative, as the EOP provided suggestions, which were then addressed by the technical work group. An example of this iterative process is illustrated by the integration of Offshore Wind as an ecosystem element. Other Ocean Uses was broadly covered in the original risk assessment, as detailed in Gaichas et al. (2018), and was scored as low-moderate in terms of risk to the summer flounder fishery. Nevertheless, the proposed footprint for offshore wind activities has continued to grow in the Northeast Atlantic US, to the extent that the EOP felt it critical to incorporate an Offshore Wind element into the conceptual model. The technical work group then identified 10 elements that could be directly impacted by offshore wind development, Estuarine Habitat, Offshore Habitat, Oceanographic Transport, Distribution Shifts, Allocation, Data Quality, Management Control, Spawning Stock Biomass, Recreational Fleet Dynamics, and Commercial Fleet Dynamics. Each linkage between these elements was documented in the support table. The EOP then reviewed the information and agreed to the manner by which Offshore Wind was incorporated into the conceptual model.
The development and refinement of management questions followed a similar pattern of iteration and collaboration. Of note is that some issues highlighted as important by managers and stakeholders were not included in the conceptual modelling exercise. For example, the effect of pollution, particularly pharmaceutical and micro-plastic pollution, on summer flounder was raised by a stakeholder during discussion with the EOP. However, the work group found little research assessing the impacts of these pollutants specifically on summer flounder, and this issue was wrapped up into a general consideration of uncertainty surrounding future stock productivity.
Model visualization
Given the size and complexity of the model, the technical work group decided to present both the full model, and simpler submodels to help in communicating it to managers and stakeholders. Given the role the conceptual model would play in developing ecosystem-level questions for MSE, and the risk assessment catalysing the endeavour, each submodel focuses on a high or moderate-high risk element. The technical team explicitly asked for guidance from the EOP on this structure, and suggested submodels could be delineated by ecological themes: Driver, Habitat, Fluke, Other Biota, Management, Benefits, Science, and Fishing Fleets. The EOP agreed with the focus on risk elements, however, due to risk assessment underpinning the conceptual modelling work. The submodels delineate all direct linkages to that risk element and between the elements linking to the risk element. Thus, individual linkages difficult to trace out through the full model become much easier to see in the submodels, and the exact manner of these impacts are described in the support table.
Given the large number of links in the full conceptual model, it was decided that an interactive visualization would provide some additional clarity for relationships represented in the conceptual model, as linkages between elements could more easily be traced, and even individually and dynamically labelled. This was an advantage over the static submodels where the smaller numbers of links were easily traced, but relationships between submodels could not easily be represented. The Council’s willingness to accept information in this (currently nonstandard) format took further advantage of the open science approach, and provided further benefits. For example, the documentation presented in Table 2 was also made interactive for clearer communication, with information again grouped by risk element to mirror the submodels. Thus, one could concurrently view a submodel and documentation filtered for the specific submodel.
Analysis and coding
For the technical work group, the open science approach meant that the most recent versions of the documentation tables were always accessible and editable as Google sheets. Key portions of the analysis relied on existing open-source software developed and shared by other researchers. The conceptual model from Dia was read into R using QPress (Wotherspoon et al., 2013), software for analysing qualitative network models. Both the static and interactive conceptual models were visualized using the R packages circlize (Gu et al., 2014; Gu, 2020) and chorddiag (Flor, 2020), allowing the team to focus on developing the model itself. In particular, the interactive conceptual model used the R package chorddiag based on javascript D3 libraries which would have been otherwise difficult to implement by the modelling team. The team used googledrive and readxl R packages (Wickham and Bryan, 2019; D’Agostino McGowan and Bryan, 2020) to automatically bring documentation tables into the full report with the model visualizations, eliminating cut-paste errors and permitting rapid updates as edits were made. Tables were formatted for easier reading using the R package kableExtra (Zhu, 2019). The report with the model and associated documentation tables were developed using the rmarkdown package in R (Xie et al., 2018; Allaire et al., 2019, 2020), open-source software that allows the user to integrate both text and analytical code in a single source document. The source document was converted to .html using the knitr package (Xie, 2014, 2015, 2019). All source code with full version controlled history is available in a public repository on GitHub (https://github.com/NEFSC/READ-SSB-DePiper_Summer_Flounder_Conceptual_Models, last accessed March 19, 2021), an open-source version control system coupled to an online platform that greatly facilitates team coding exercises. This allows the work group or any other researcher to reuse code and reproduce the analysis (Lowndes et al., 2017). Finally, the .html report for the Council was published to a permanent web address for free using GitHub Pages (https://pages.github.com/, last accessed March 19, 2021), so that anyone with the website link can peruse the interactive model and documentation.
The use of .html allowed changes to the conceptual model to be instantaneously represented in the final model. Additionally, the support table is published directly under the conceptual model, which greatly facilitates the interpretation of the visual model components while enhancing the model’s scientific reproducibility and transparency. To enhance socially-relevant transparency (Elliott and Resnik, 2019), the EOP suggested adding a table of definitions to the conceptual model documentation, to further facilitate interpretation, particularly for stakeholders who were not fully engaged during the development process. Given that the linkages were defined within the supporting table, the separate definition table focused on the risk elements. The table of definitions was developed efficiently using information from the Council’s risk assessment document, also produced in rmarkdown and archived on GitHub, and is published on the same page as the conceptual model, to ensure all relevant information is presented together. The definition table is sortable to help users navigate to the portions of interest, thanks to the R package DT (Xie et al., 2019).
Results
The full conceptual model included 57 elements with 138 linkages between them. A static representation of the full model can be found in Figure 1. There were a total of 16 submodels developed: the High Ecosystem Risk Elements, Summer Flounder Distributional Shift, Estuarine Habitat, Stock Biomass, Stock Assessment, Offshore Habitat, Allocation, Commercial Profits, Discards, Shoreside Support, Fleet Diversity, Management Control, Recreational Value, Regulatory Complexity, Seafood Production, and Technical Interactions. The Allocation submodel is presented in Figure 2, as an example. The utility of the submodel becomes apparent as, although the issues impacting allocation are difficult to trace in Figure 1, it becomes much easier to see that Data Quality, Summer Flounder Distributional Shifts, and Offshore Wind Development all impact Allocation, with Allocation in turn impacting Fishery Distributional Shifts, Offshore Wind impacting Data Quality, and Summer Flounder Distributional Shifts impacting Offshore Wind.
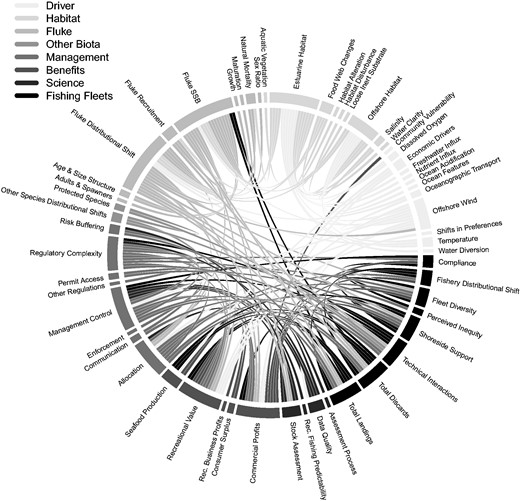
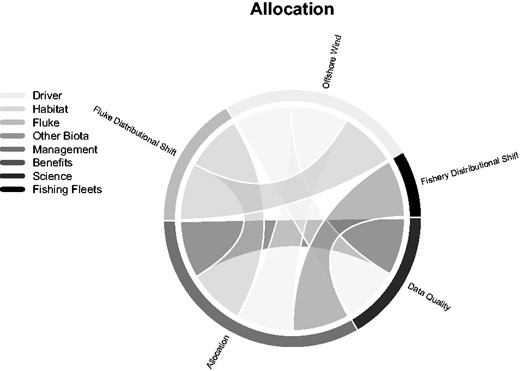
The conceptual model was used to identify seven highly relevant and important ecosystem-level management questions by the EOP. All seven questions are provided below to highlight the diversity and range of questions considered by the EOP. The top four were prioritized for detailed rationalization with respect to their management importance, and top three ultimately were presented to the full Council for consideration in December of 2019:
How does utilizing recreational data sources at scales that may be inappropriate for the data source [e.g. Marine Recreational Information Program (MRIP) data at the state/wave/mode level] affect management variability, uncertainty, and fishery performance? (MRIP is the US federal survey of recreational fishing, which uses in-person, mail, and telephone subsamples in 2-month waves to estimate population-level estimates of catch, harvest, and effort. Four modes of fishing are categorized: Shore, Private Vessel, Charter, and Head Boats. See https://www.fisheries.noaa.gov/topic/recreational-fishing-data, last accessed March 19, 2021, for further information.) Evaluate the impact of that variability and uncertainty and its use in the current conservation equivalency process on recreational fishery outcomes [Conservation equivalency was adopted in Framework 2 of the “Summer Flounder, Scup, Black Sea Bass Fishery Management Plan” (Mid-Atlantic Fishery Management Council, 2001) and allows states to implement differing recreational management strategies, so long as mortality is kept below target levels].
Rationale: The importance of the recreational summer flounder fishery, growing concerns about MRIP data and its use in management, and the potential application to other Council-managed fisheries were some of the reasons the EOP decided to make this question the top priority. However, it is important to note the focus of this question was not to conduct a review and evaluation of the MRIP but to understand the management implications of the current approaches and utilization of MRIP data within the recreational management process. While this question focuses on recreational data and management, the data quality element is linked to four high-risk factors contained in the conceptual model, and a full evaluation of this question could provide insight and guidance on a number of biological, environmental, social, economic, and management objectives.
What are the mechanisms driving summer flounder distribution shift and/or population range expansion? What are the biological, management, and socio-economic implications of these changes? Identify potential management and science strategies to help account for the impacts of these changes.
Rationale: The EOP noted the number of biological and management challenges the Council is already facing because of shifting species distributions. Evaluating this question has the potential to provide the Council with an increased understanding of what’s driving these population shifts, what those implications might be, and offer different tools and strategies to address these issues and meet its management objectives. Summer flounder distribution shift was identified as a high-risk factor through the EAFM Risk Assessment and is the most linked ecosystem element with 11 other high-risk factors, across all aspects of the summer flounder fishery conceptual model ecosystem, affected by summer flounder distribution shifts.
Evaluate the biological and economic benefits of minimizing discards and converting discards into landings in the recreational sector. Identify management strategies to effectively realize these benefits.
Rationale: Assessing the various management challenges to address and reduce regulatory discards, particularly within the recreational sector summer flounder fishery where 90% of the recreational catch is released, is a high priority for the Council. This issue is also raised frequently by stakeholders and Advisory Panel members (Advisory Panels are formal groups of stakeholders, primarily composed of fishermen and non-governmental organization representatives, who advise the Council on issues pertaining to fishery management. Each federal fishery management plan, including the joint “Summer Flounder, Scup, and Black Sea Bass” Plan, is supported by an Advisory Panel.) and the Council is considering management strategies that depart from the current management process in an effort to reduce discards. Given the Council’s consideration of addressing recreational summer flounder discards in the EAFM and in the traditional management process, this could present a unique opportunity to align these efforts. Summer Flounder Discards was identified as a high-risk factor through the EAFM risk assessment and is linked to seven additional high-risk factors across issues of Management, Summer Flounder Stock, Science, Fishing Fleets, and Benefits derived from the resource.
Are there alternative allocation schemes that would provide more flexibility in the commercial allocation strategy and allow fishermen to adapt to changing biological, economic, and social dynamics more effectively? Identify and evaluate potential fleet efficiencies, economic, and biological trade-offs and potential adjustments to baseline access to the summer flounder resource by the commercial sector through these alternate allocation schemes.
Rationale: The Council is currently considering allocation changes to four of its managed fisheries, including summer flounder. The EOP was interested in developing a question that considered allocation strategies for both the recreational and commercial sectors and evaluated minimum access scenarios (i.e. base or minimum levels of allocation) for each sector as well. However, the technical work group felt that minimum access scenarios for each fleet would be too variable and uncertain to define and, at this time, there was only enough information on the commercial sector to fully investigate allocation strategies. Since the question developed by the work group could not address all areas of interest to the EOP, the question was not considered as high a priority. However, some members of the EOP felt there was still value in considering this question and the potential outcomes due to recent Council actions to consider allocations changes to four Council-managed species. Allocation was identified as a high-risk factor through the EAFM risk assessment and is linked to 9 additional high-risk factors across issues of Management, Summer Flounder Stock, Fishing Fleets, Offshore Wind, and Benefits derived from the resource.
Although the issues are important to the Council, the questions below were considered by the EOP but were not identified as a priority and not listed in priority order.
Is the availability and quality of habitat a limiting factor for summer flounder stock productivity? Evaluate changes in critical habitat (i.e. quality, quantity, spatial extent, and overlap) across summer flounder life stages, identify habitat thresholds, and the implications for stock productivity. Develop potential management goals and strategies to address summer flounder habitat change and identify actionable outcomes for Council consideration.
What are the most influential elements that impact stock dynamics (i.e. recruitment, distribution, standing stock biomass, growth, etc.) and management decisions? Identify data gaps for those elements and develop a research planning process to address these gaps.
Offshore wind construction and operation is likely to impact the ecological and socio-economic environment for summer flounder and its fisheries. What are the key drivers of recreational and commercial fleet dynamics under different scenarios of opportunity and access level to offshore wind lease areas? Evaluate the changes to and potential trade-offs between sector fleet dynamics and evaluate the biological implications (e.g. spawning stock biomass, recruitment) of these fleet dynamic scenarios. Determine and evaluate fishery management options to address these sector-specific implications and trade-offs.
Discussion
Ecosystem analyses have been criticized as “too complex” for non-technical audiences to grasp or tolerate (Patrick and Link, 2015), but open science tools greatly facilitated communication in this instance. For presentation to the full Council, a dropdown menu of management questions was added to the full conceptual model using the open-source R package bsselect (Walker, 2016), which allowed toggling between the three top priority questions, focusing on one at a time. This alternate model representation can be found at https://nefsc.github.io/READ-SSB-DePiper_Summer_Flounder_Conceptual_Models/sfconsmod_final_2col.html (last accessed March 19, 2021). A subset of the technical work group was thus able to discuss how the conceptual model informed the development of the question itself, with a visual juxtaposition between the model and question. This discussion included tracing out the linkages between the ecosystem component which was the focus of each of the three questions and the remainder of the system in the interactive conceptual model. In this manner, the Council was provided a demonstration of the utility of the conceptual model that made the best use of the hour available in their full meeting schedule. The EAFM process knowledge developed through the collaborative modelling effort undertaken by the EOP and technical work group was thus transferred to the full Council and stakeholders present through these deep dives into a subset of questions.
The benefits of the approach outlined relate to the major challenges in implementing ecosystem science within a management system: the state of interdisciplinary/transdisciplinary science, capacity constraints, statutory limitations, and lack of appropriate scoping for management objectives and stakeholder concerns. O’Higgins et al. (2020) indicate the clear role learning by doing plays in building capacity to implement EBM. The work detailed here highlights how conceptual modelling and open science concepts can foster this learning. The transparency, reproducibility, and efficiency inherent in the concepts of open science decrease the costs of engaging in transdisciplinary ecosystem science, including scoping work, and helps address capacity constraints. Conceptual modelling allows scientists, managers, and stakeholders to collaboratively build a holistic view of the system; but one tailored to the management system being serviced by the science. This, in turn, helps address issues surrounding the state of transdisciplinary science and perceived statutory limitations.
In hindsight, more outreach could have been undertaken in the process. Presentations should have been recorded and made available to the public prior to meetings, and in conjunction with the conceptual model to facilitate communication beyond those who could attend meetings, as is more common in these current times of pandemic [see, e.g. Akers 2020 communication to the MAFMC (https://drive.google.com/file/d/1UF8hIyca9QcDuHQt0aK7wPLbcR6ywHeK/view, last accessed March 19, 2021)]. The Council’s timeline meant outreach to those individuals not directly involved in the development of the models was relatively passive, and relied on the standard Council communication channels. This outreach includes publishing upcoming meeting agendas and supporting documentation to the Council’s website no later than 1 week in advance of the respective meeting. More active outreach, including scheduling informal time for the technical work group to answer stakeholder questions regarding the conceptual model, would also likely have helped in better informing stakeholders of the process.
Even with the open science efficiencies, the complexity of the model made for a challenging quality assurance and quality control (qa/qc) process. In hindsight, a formal qa/qc step would have provided substantial benefits and streamlined what became a relatively drawn-out process spurred mainly by individual motivation. Engaging a lead qa/qc editor removed from the development process would likely have proven most effective, as familiarity with the model’s development coloured internal work group member’s understanding of the model as presented. A fresh set of eyes unbiased by the development process would have helped both in identifying missing links and ecosystem components and in transparent communication of the underlying concepts more broadly.
The Council ultimately selected question 3 “Evaluate the biological and economic benefits of minimizing discards and converting discards into landings in the recreational sector. Identify management strategies to effectively realize these benefits.” for development into an MSE, although there was substantial support for question 1 as well. The prevailing rationale for selecting question 3 was the clear focus on benefit generation, in that converting discards to landings will directly benefit recreational anglers. This is in contrast to questions 1 and 2, which although had vague distal implications for benefit provisioning were proximally focused on science and management performance issues. The issue of discarding was already being discussed within the Council, which provided an opportunity to align the traditional single-species approach with the newer EAFM approach, and the Council felt the topic was well disposed to assessment within an MSE. The conceptual model itself allowed the Council to understand the system linkages which should be considered when developing the MSE. This was in contrast to question 2, which seemed too nebulous to the Council for assessment within a single MSE, and concerns that keeping a focus on question 1 during stakeholder engagement and scoping would be difficult, given substantial stakeholder dissatisfaction with many facets of the MRIP.
A technical work group has been convened to facilitate the development of the MSE around this question, with substantial group overlap of experts from the conceptual modelling exercise. Final MSE results are expected to be presented to the Council in 2022.
Conclusion
This paper details the approach employed by the US Mid-Atlantic Fishery Management Council in transitioning from an ecosystem-level risk assessment towards a MSE. The approach focused on developing a conceptual model of key interactions between high-risk elements in a system, which was used to conduct an information gap analysis and scope potential management-relevant questions which could be answered through the implementation of an MSE. The MSE will be aimed at addressing the highest risks facing the summer flounder fishery, in support of the Council’s EAFM framework. We situate this work in best practices for open science, with an eye towards efficiency, transparency, and reproducibility. Indeed, the successful completion of this project within 1 year was due in large part to open science tools developed by other researchers, as well as the iteration of research products and ideas between scientists, managers, and stakeholders in the Council process. Although the implementation of open science concepts to support ecosystem science is not new (e.g. Tai and Robinson, 2018; Borja et al., 2019; Powers and Hampton, 2019; Beck et al., 2020), this case study highlights the role these concepts played in supporting fishery manager’s strategic ecosystem-level decision-making. The work outlined represents one path forward in transitioning towards EBM, as one of the few examples in which ecosystem science has directly supported strategic management decision-making at the national level. This approach acknowledges that there is a lack of experience in both the scientific and management context which has likely slowed the adoption of the EBM framework formally into management internationally.
In developing the conceptual model, managers and scientists created a shared understanding of the ecosystem linkages around summer flounder. The undertaking provided the Council with insight into how the information coming out of a risk assessment could be used to develop specific questions of interest to managers and stakeholders. It further provided insight into the engagement process that will prove valuable in developing the MSE to follow. As an example, broader dissemination of narrated presentations is envisioned as part of the MSE to enhance transparency and increase access points to the process for stakeholders. By voting to move forward with the MSE, the Council indicates a continued willingness to move towards EAFM, and an acknowledgement that system interactions play an important role in the success of management actions. As such, this exercise proved to be a successful example of learning by doing, which should ultimately lead to more robust strategic decision-making.
Data availability statement
All of the code and documentation, and information used in developing the models can be found at https://github.com/NEFSC/READ-SSB-DePiper_Summer_Flounder_Conceptual_Models or Gaichas and DePiper (2020).
References
DePiper, G., Gaichas, S., Muffley, B., Ardini, G., Brust, J., Coakley, J., Dancy, K., Elliott, G. W., Leaning, D. C., Lipton, D., McNamee, J., Perretti, C., Rootes-Murdy, K., and Wilberg, M. J. Learning by doing: Collaborative conceptual modelling as a path forward in ecosystem-based management. – ICES Journal of Marine Science, 00: 000–000.