-
PDF
- Split View
-
Views
-
Cite
Cite
Richard J. Bell, David E. Richardson, Jonathan A. Hare, Patrick D. Lynch, Paula S. Fratantoni, Disentangling the effects of climate, abundance, and size on the distribution of marine fish: an example based on four stocks from the Northeast US shelf, ICES Journal of Marine Science, Volume 72, Issue 5, May/June 2015, Pages 1311–1322, https://doi.org/10.1093/icesjms/fsu217
- Share Icon Share
Abstract
Climate change and fishing can have major impacts on the distribution of natural marine resources. Climate change alters the distribution of suitable habitat, forcing organisms to shift their range or attempt to survive under suboptimal conditions. Fishing reduces the abundance of marine populations and truncates their age structure leading to range contractions or shifts. Along the east coast of the United States, there have been major changes in fish populations due to the impacts of fishing and subsequent regulations, as well as changes in the climate. Black sea bass, scup, summer flounder, and winter flounder are important commercial and recreational species, which utilize inshore and offshore waters on the northeast shelf. We examined the distributions of the four species with the Northeast Fisheries Science Center trawl surveys to determine if the along-shelf centres of biomass had changed over time and if the changes were attributed to changes in temperature or fishing pressure through changes in abundance and length structure. Black sea bass, scup, and summer flounder exhibited significant poleward shifts in distributions in at least one season while the Southern New England/Mid-Atlantic Bight stock of winter flounder did not shift. Generalized additive modelling indicated that the changes in the centres of biomass for black sea bass and scup in spring were related to climate, while the change in the distribution of summer flounder was largely attributed to a decrease in fishing pressure and an expansion of the length–age structure. While the changes in ocean temperatures will have major impacts on the distribution of marine taxa, the effects of fishing can be of equivalent magnitude and on a more immediate time scale. It is important for management to take all factors into consideration when developing regulations for natural marine resources.
Introduction
Increasing global temperatures can have major impacts on marine organisms, including shifts in distributions and changes in abundance (Walther et al., 2002; Hare et al., 2010; Pinsky et al., 2013. Recent studies have shown that with the poleward shift in ocean temperature isotherms (Burrows et al., 2011) there has been a subsequent shift both poleward and to greater depths in marine taxa (Pinsky et al., 2013; Poloczanska et al., 2013). Organisms increase their overall survival and fecundity by occupying, to the extent possible, their optimal habitat (Anderson et al., 2013). Individuals within their optimal habitat maximize their overall fitness and minimize their mortality risks. Climate change, however, can affect certain aspects of an organism's habitat. The physical structure or photoperiod may remain constant at a given location or latitude, but other habitat components such as temperature, salinity, dissolved oxygen, and advection may be altered (Anderson et al., 2013). Shifts in distribution are then the result of individuals attempting to remain within the best conditions possible, through migration or declines in abundance in suboptimal environments, while expanding in better suited environments, if they exist.
In addition to climate-mediated changes in distribution, range shifts may also be caused by simple changes in overall abundance. When populations are low, they occupy the highest quality habitat available. As the population density increases, individuals move out into formerly inferior habitat where intraspecific competition is lower (MacCall, 1990; Quinn and Deriso, 1999). Habitat quality is rarely isotropic, so the areal expansion of the population along a habitat gradient with increasing abundance could result in a shift in distribution.
Changes in abundance due to increases in mortality can also affect distribution through changes in the length–age structure of a population. Within certain species, such as Pacific hake, there is a tendency for individuals to be distributed by size, with larger members at higher latitudes (Nelson and Dark, 1985; Smith et al., 1992). Fishing preferentially removes the largest and oldest fish (Fenberg and Kaustuv, 2008). For stocks segregated by size, fishing pressure could remove the larger individuals, which, if located at higher latitudes, would result in a truncation of the species range and a distribution centred at a lower latitude.
Along the Northeast Shelf of the United States there have been major shifts in the distribution of marine taxa (Nye et al., 2009; Lucey and Nye, 2010; Howell and Auster, 2012; Pinsky and Fogarty, 2012). Changes in the species assemblages due to the shifts can have major impacts on ecosystem goods and services. The distribution shifts are particularly important because the catch quota for a number of stocks and therefore peoples livelihoods, are allocated based on the species' distributions in previous decades (ASMFC, 2004). Understanding the mechanisms which regulate distribution must be taken into account as part of any potential change to the quota allocation system.
On the Northeast shelf there is evidence of warming water temperatures (Nixon et al., 2004; Friedland and Hare, 2007; Belkin, 2009) and large changes in the abundance of fish species due to fishing and recovery (Fogarty and Murawski, 1998; Terceiro, 2012b). Many of the studies on species distribution shifts have implicated increasing temperature as a major driver. However, changes in size and age structure (Radlinski et al., 2013) and changes in abundance (Hare et al., 2010) have also been implicated in distribution shifts in the ecosystem.
We examined four species in the Southern New England/Mid-Atlantic Bight (SNE/MAB) region of the Northeast shelf in detail to determine if there had been shifts in their distributions and if so, could one of the three hypotheses account for the changes: (1) Climate change: Species shift to remain within their optimal habitat. (2) Abundance effects: Species shift owing to changes in abundance and the area of habitat occupied. (3) Size effects: Species shift because changes in the length structure of the population changes the latitudinal range occupied.
Black sea bass (Centropristis striata), scup (Stenotomus chrysops), summer flounder (Paralichthys dentatus), and winter flounder (Pseudopleuronectes americanus) are important commercial and recreational species along the east coast of the United States that have varied in abundance over the last 40 years. All four species utilize nearshore habitat during both early development and adult stages and their latitudinal ranges are believed to be influenced by their upper or lower thermal tolerance in the SNE/MAB (Collette and Klein-MacPhee, 2002). All four have also experienced heavy fishing pressure at times over the last 40 years. We examined the distributions of the four species to see if their along-shelf centres of biomass had changed over time and if the changes were attributed to changes in the physical environment or fishing pressure through changes in abundance and length structure.
Methods
We determined the centre of biomass for each species and tested if there had been a significant change in distribution over the time series. The three hypotheses were examined by fitting a generalized additive model to the centre of biomass data with three independent variables related to the hypotheses: (1) water temperature (climate change), (2) abundance (abundance effects), and (3) length (size effects).
As an additional test of Hypothesis 2 (abundance effects), the difference between the 75th and 25th percentiles of each species' range was assessed for expansion or contraction over time and a relationship with abundance. As an additional test of Hypothesis 3 (size effects), the empirical along-shelf distributions of different length classes were calculated for each species to further examine the effect of size on distribution.
Along-shelf centre of biomass
We calculated the seasonal centre of biomass for the four species based on the stratified-weighted mean biomass from the Northeast Fisheries Science Center (NEFSC) spring and fall bottom trawl surveys. The methods were similar to Nye et al. (2009) except for three factors. (1) Biomass was used instead of ln(biomass + 1). (2) Biomass was estimated from the length of the fish in one centimetre size bins based on the equation w = aLb, where the parameters (a and b) were obtained from the NOAA fisheries age–length–weight website (NEFSC Fisheries Biology Program, 2013) (Table 1). (3) To account for the stratified random sampling design, each tow was weighted by stratum area/num of tows (num of tows = number of tows within a stratum each sampling period) so that differences in the annual-seasonal allocation of stations to each stratum would not affect the centre of biomass. Because the east coast of the United States runs in a northeast direction and not directly north-south, the centre of biomass was measured as changes along the continental shelf (Figure 1). Following Nye et al. (2009), the distance along the shelf was calculated for each tow at the 100 m depth contour.
Variables for the length-to-weight conversions for each species w = aLb, and the YOY length thresholds.
Species . | a . | b . | YOY (cm) . |
---|---|---|---|
Black Sea Bass | 1.598691e−05 | 2.91 | <15 |
Scup | 1.410615e−05 | 3.19 | <10 |
Summer flounder | 7.478006e−06 | 3.18 | <30 |
Winter flounder | 1.36979e−05 | 2.97 | <16.5 |
Species . | a . | b . | YOY (cm) . |
---|---|---|---|
Black Sea Bass | 1.598691e−05 | 2.91 | <15 |
Scup | 1.410615e−05 | 3.19 | <10 |
Summer flounder | 7.478006e−06 | 3.18 | <30 |
Winter flounder | 1.36979e−05 | 2.97 | <16.5 |
Variables for the length-to-weight conversions for each species w = aLb, and the YOY length thresholds.
Species . | a . | b . | YOY (cm) . |
---|---|---|---|
Black Sea Bass | 1.598691e−05 | 2.91 | <15 |
Scup | 1.410615e−05 | 3.19 | <10 |
Summer flounder | 7.478006e−06 | 3.18 | <30 |
Winter flounder | 1.36979e−05 | 2.97 | <16.5 |
Species . | a . | b . | YOY (cm) . |
---|---|---|---|
Black Sea Bass | 1.598691e−05 | 2.91 | <15 |
Scup | 1.410615e−05 | 3.19 | <10 |
Summer flounder | 7.478006e−06 | 3.18 | <30 |
Winter flounder | 1.36979e−05 | 2.97 | <16.5 |

The position of the along-shelf metric for measuring the centre of biomass on the east coast of North America. The individual survey strata are in light grey.
Sampling strata for the NEFSC trawl survey have changed over the years (Sosebee and Cadrin, 2006). While some strata have been continuously sampled since 1963, sampling began on the more inshore areas in 1972. Sampling of the two most inshore depth zones ceased in 2009. We restricted our analysis to the years 1972–2008 to be able to include all depth strata in all years.
Black sea bass, scup, and summer flounder are considered one continuous stock from Cape Hatteras north to the Canadian border (NEFSC, 2012; Shepherd, 2012; Terceiro, 2012a, b). The majority of the individuals are in the SNE/MAB region with some fish on Georges Bank. Relatively few individuals are caught north of Cape Cod (Gulf of Maine). For example, over the entire time series, only five black sea bass were recorded in the Gulf of Maine in spring. In the Fall, roughly 2% of black sea bass catches were in the Gulf of Maine. We restricted the analysis to strata in the SNE/MAB and Georges Bank region. Winter flounder is divided into three stocks (NEFSC, 2011). We focused only on species in SNE/MAB and therefore only included the SNE/MAB stock of winter flounder. All winter flounder individuals south and west of the Great South Channel were included in the analysis of distribution. None of the winter flounder on Georges Bank or in the Gulf of Maine were included.
The young-of-the-year (YOY) of each species were not fully accessible to the fall trawl survey and were excluded from analyses. All four species utilize nearshore areas as nursery grounds and all except winter flounder move offshore at the end of their first summer (Collette and Klein-MacPhee, 2002). YOY in more northerly latitudes move offshore into the survey area earlier than those further south, while the southern area is sampled before the northern area. The northern YOY are then accessible to the survey while the southern portion is not accessible. This may not be true for all species in all years, but to create a consistent dataset, all individuals below the YOY length threshold in autumn were excluded. The YOY length thresholds were taken from the NOAA fisheries age–length–weight website (NEFSC Fisheries Biology Program, 2013; Table 1). All size classes were included in spring analyses.
In the lag−1 autoregressive structure, the residuals in year t are equal to the residuals in year t − 1, times the autoregressive parameter (ϕ) plus a normally distributed error term (vt). The autocorrelation decays if the absolute value of ϕ is <1. We examined trends along the full time series from 1972 to 2008 for all species.
Generalized additive models
Generalized additive modelling (GAM) was used to examine potential factors driving changes in the along-shelf centres of biomass. GAMs are extensions of linear models in which the dependent variable is the sum of smooth functions of the independent variables (Wood, 2006). Our analyses were conducted with the freeware software R with the GAM package (mgcv) (Wood, 2006). We examined the change in distribution relative to the three hypotheses, as implemented by the change in the mean length of the population, temperature, and abundance.
The temperature term corresponds to the inner-shelf, surface temperature anomaly averaged over the 12-week period before the central date of spring and fall trawl surveys. Both temperature anomaly time series extend from 1977 to 2008 with some missing years. Temperature observations were extracted from a 35-year archive of hydrographic observations collected by the Northeast Fishery Science Center (NEFSC). An area-weighted average temperature was calculated from observations in the inner-shelf region of the SNE/MAB, roughly the area inshore of the 50 m isobath. This region encompasses the majority of the range of the four stocks and integrates the cumulative seasonal temperature effect that could drive changes in species distribution. Anomalies were calculated relative to a reference annual cycle, estimated by applying a basic harmonic curve-fitting technique to the full archive of historical temperature observations falling within the same inner-shelf region (Mountain and Holzwarth, 1989). Anomaly time series represent the difference between the time series of regional mean temperatures and corresponding reference temperatures predicted by the reference annual cycle for the same time of year. The mean date of the spring trawl survey over all years was Week 13 of the year (the second week of April) and the mean date of the fall trawl survey over all years was Week 40 of the year (the second week of October). The anomalies were averaged within two 12-week windows, corresponding to the survey timing, to produce spring and fall temperature anomaly time series used for the temperature term in the GAMs. The spring temperature term is the average of the temperature anomalies from Weeks 2 through 13 (early Jan to early April) of each year, while the fall temperature term is the average of the anomalies from Weeks 29 through 40 (early July to early October).
The general location of fish with regard to temperature is typically a response to the cumulative impacts of temperature over an entire season. A fish's location is the integral of the temperature over the entire season and not just the temperature at the specific time and place that the fish is caught. The seasonally averaged temperature smooths over the daily fluctuations in temperature to provide a general picture of the conditions potentially driving distribution. Twelve weeks (3 months) were selected as the length of the season.
Abundance effects
We examined if changes in distribution were due to changes in abundance, which resulted in the expansion or contraction of a species range (Hypothesis 2). The frequency histogram of the along-shelf position of all individuals of a species each season per year was expressed as a cumulative density function. The difference between the 75th percentile of the along-shelf location cumulative density function and 25th percentile was calculated to determine the annual distance occupied. A linear regression was fit to the annual distance occupied, accounting for autocorrelation in the residuals as indicated above, to determine if there was a significant expansion or contraction of each species' range. The annual distance occupied was then regressed against the stratified-weighted abundance, to determine if the total occupied range expanded and contracted in relations to the number of individuals.
Size effects
The along-shelf distributions of different sized individuals of each stock were examined, to determine if different length classes occupied different along-shelf ranges (Hypothesis 3). We divided the survey data into length classes based on the length distribution of each species. The annual along-shelf centre of biomass for each length class was determined, as well as the along-shelf position of the 25, 50, and 75th percentile in the same manner as described in the abundance effects section above. The overall mean position was the mean of the annual along-shelf centre of abundance for each percentile. The proportion of total stratified mean abundance in each size class, each year was also calculated. The YOY data from the fall survey were included in the calculations for demonstration purposes.
Results
Along-shelf centre of biomass
The changes in the annual centre of biomass varied among species and seasons (Figure 2). Black sea bass and scup had significant northerly trends in spring that were not present in autumn (Figure 3). Summer flounder had a significant northward trend in the fall, but no change in distribution in spring. The pattern was similar between the two seasons however, with the distribution further north in the 1970s and 1980s, shifting south in the early 1990s then moving poleward into the 2000s. The SNE/MAB stock of winter flounder varied over time, but showed no change in distribution over the time series during either season.
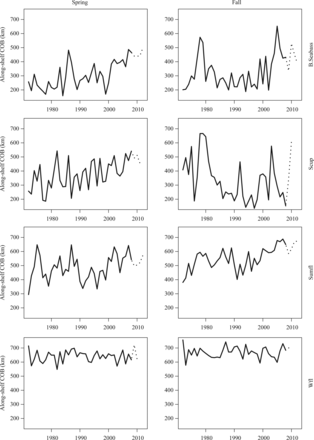
The along-shelf centre of biomass for each species in each season. The solid line covers the period 1972–2008. The dotted line is data from a different survey vessel starting in 2009 that was not used in the analyses (B.Seabass—black sea bass; Scup—scup; Sumfl—summer flounder; Wfl—winter flounder).
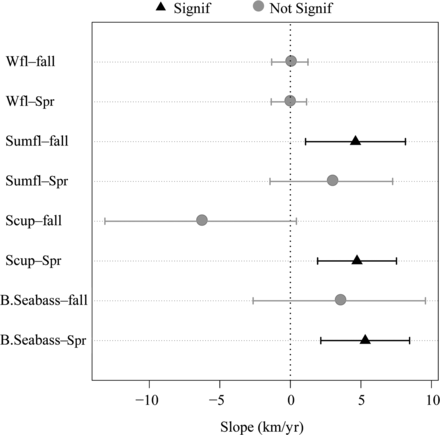
The slope in kilometres per year of the annual along-shelf centre of biomass for each species in each season with 95% confidence intervals. Slopes with a p-value of <0.05 were considered significant (B.Seabass—black sea bass; Scup—scup; Sumfl—summer flounder; Wfl—winter flounder).
Generalized additive models
The GAMs fit to the changes in the centre of biomass produced a range of results (Table 2 and Figure 4). Over the course of the time series the spring and fall temperatures increased (Figure 5), while abundance and mean length varied (see Supplemental material). Different seasons and species had different significant parameters and the total amount of deviance explained varied considerably. The along-shelf centre of biomass for black sea bass and scup in spring had a significant positive relationship with temperature (Table 2). The other covariates, the length structure, and the total population did not exhibit a relationship for these two species. The GAMs explicitly modelled the relationship between the interannual change in mean spring temperature and the interannual change in the centre of biomass. The temperature varied annually, but increased over the time series, indicating that the long-term distribution shifts of black sea bass and scup in spring were likely related to the long-term warming trend on the shelf. The two species presumably shifted to remain in their preferred thermal habitat, which moved north. The GAMs explain 34 and 29% of the total deviance indicating that there are additional factors affecting the changes in distribution. The GAMs suggest Hypothesis 1 (climate change) as an important mechanism for distribution shifts in these two species in spring. In the fall, the temperature along the Northeast shelf is within the suitable range for black sea bass and scup (Collette and Klein-MacPhee, 2002) and the physical environment was not significantly related to the centre of biomass. The mean length was the only significant term for both species with the centre of biomass further north when larger fish made up a greater proportion of total abundance (Supplementary material, Figures SA6 and SA10).
The estimated degrees of freedom for each species and season from the generalized additive models.
Species . | M.lgth . | Temp . | N . | Dev. exp. . |
---|---|---|---|---|
B.Seabass—Spr | 1 | 1.0951* | 1 | 0.34 |
B.Seabass—Fall | 1* | 1.4143 | 1 | 0.46 |
Scup—Spr | 1 | 1* | 1 | 0.29 |
Scup—fall | 2.6869* | 1 | 4.8375 | 0.90 |
Sumfl—Spr | 2.9585* | 1.2554 | 1 | 0.62 |
Sumfl—Fall | 2.1219* | 1.6673 | 1 | 0.82 |
Wfl—Spr | 3.9728 | 1 | 1.1559 | 0.38 |
Wfl—Fall | 1 | 1 | 1 | 0.03 |
Species . | M.lgth . | Temp . | N . | Dev. exp. . |
---|---|---|---|---|
B.Seabass—Spr | 1 | 1.0951* | 1 | 0.34 |
B.Seabass—Fall | 1* | 1.4143 | 1 | 0.46 |
Scup—Spr | 1 | 1* | 1 | 0.29 |
Scup—fall | 2.6869* | 1 | 4.8375 | 0.90 |
Sumfl—Spr | 2.9585* | 1.2554 | 1 | 0.62 |
Sumfl—Fall | 2.1219* | 1.6673 | 1 | 0.82 |
Wfl—Spr | 3.9728 | 1 | 1.1559 | 0.38 |
Wfl—Fall | 1 | 1 | 1 | 0.03 |
The proportion of the deviance explained (Dev. Exp.) is for the models with all three terms included. The asterisk denotes significant terms.
B.Seabass, black sea bass; Scup, scup; Sumfl, summer flounder; Wfl, winter flounder.
The estimated degrees of freedom for each species and season from the generalized additive models.
Species . | M.lgth . | Temp . | N . | Dev. exp. . |
---|---|---|---|---|
B.Seabass—Spr | 1 | 1.0951* | 1 | 0.34 |
B.Seabass—Fall | 1* | 1.4143 | 1 | 0.46 |
Scup—Spr | 1 | 1* | 1 | 0.29 |
Scup—fall | 2.6869* | 1 | 4.8375 | 0.90 |
Sumfl—Spr | 2.9585* | 1.2554 | 1 | 0.62 |
Sumfl—Fall | 2.1219* | 1.6673 | 1 | 0.82 |
Wfl—Spr | 3.9728 | 1 | 1.1559 | 0.38 |
Wfl—Fall | 1 | 1 | 1 | 0.03 |
Species . | M.lgth . | Temp . | N . | Dev. exp. . |
---|---|---|---|---|
B.Seabass—Spr | 1 | 1.0951* | 1 | 0.34 |
B.Seabass—Fall | 1* | 1.4143 | 1 | 0.46 |
Scup—Spr | 1 | 1* | 1 | 0.29 |
Scup—fall | 2.6869* | 1 | 4.8375 | 0.90 |
Sumfl—Spr | 2.9585* | 1.2554 | 1 | 0.62 |
Sumfl—Fall | 2.1219* | 1.6673 | 1 | 0.82 |
Wfl—Spr | 3.9728 | 1 | 1.1559 | 0.38 |
Wfl—Fall | 1 | 1 | 1 | 0.03 |
The proportion of the deviance explained (Dev. Exp.) is for the models with all three terms included. The asterisk denotes significant terms.
B.Seabass, black sea bass; Scup, scup; Sumfl, summer flounder; Wfl, winter flounder.
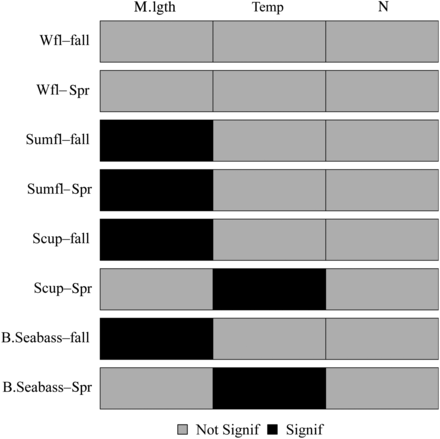
The significance of each term in the generalized additive model for the dependent variable along-shelf centre of biomass for each species in each season. Terms with a p-value of <0.05 were considered significant (B.Seabass—black sea bass; Scup—scup; Sumfl—summer flounder; Wfl—winter flounder).
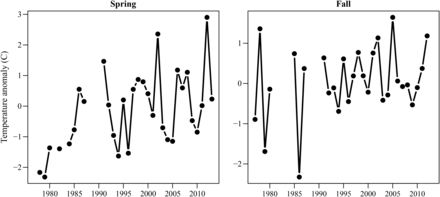
The interannual changes in the along-shelf centre of biomass for summer flounder in both seasons showed a significant relationship with the interannual changes in mean length, but not with temperature or abundance. Initial GAM runs with the default number of knots for summer flounder in the fall, resulted in significant terms for both mean length and temperature. The temperature response curve overfit the data and was not ecologically plausible, however (Supplementary material, Figure SA15). Reducing the number of initial knots produced a plausible response curve that did not overfit the data, but the temperature term was no longer significant. This was not an issue for any of the other GAMs.
Larger summer flounder occupy habitat further north and as the proportion of larger fish in the population has increased the stock has shifted north (Supplementary material, Figures SA12, SA13, and SA14). Temperature was not a significant term in the model suggesting it had little influence on the change in distribution (Table 2). The shift is particularly dramatic since the early 1990s when the population was severely depleted and the length structure truncated (Supplementary material, Figure SA11). The results indicate that a change in the length–age structure (Hypothesis 3—size effects) is the main driver of interannual shifts in summer flounder distribution.
The centre of biomass of winter flounder varied without a trend and none of the terms in the GAMs were significant. There was little support for any of the hypotheses concerning winter flounder. Abundance was not significant in any of the GAMs for any species. The analyses provided no evidence for changes in abundance impacting the interannual changes in the centre of biomass (Hypothesis 2—abundance effects).
Abundance effects
None of the species exhibited a significant change in the along-shelf distance occupied (Table 3). The annual range occupied varied, but the difference between the 75th and 25th percentile of the along-shelf position did not expand or contract consistently over the course of the time series (Supplementary material, Figures SA1 and SA2). As an example, the spring trawl survey data indicate that black sea bass is moving north, but the total occupied range does not exhibit a trend (Figure 6).
The slope of the difference between the 75th and 25th percentile of the along-shelf centre of biomass for each species over time.
Species . | Slope . | p-value . |
---|---|---|
B.Seabass—Spr | 1.73 | 0.21 |
B.Seabass—Fall | 2.11 | 0.41 |
Scup—Spr | −3.11 | 0.16 |
Scup—Fall | −2.61 | 0.27 |
Sumfl—Spr | −0.17 | 0.92 |
Sumfl—Fall | −0.37 | 0.73 |
Wfl—Spr | 1.11 | 0.10 |
Wfl—Fall | −1.86 | 0.11 |
Species . | Slope . | p-value . |
---|---|---|
B.Seabass—Spr | 1.73 | 0.21 |
B.Seabass—Fall | 2.11 | 0.41 |
Scup—Spr | −3.11 | 0.16 |
Scup—Fall | −2.61 | 0.27 |
Sumfl—Spr | −0.17 | 0.92 |
Sumfl—Fall | −0.37 | 0.73 |
Wfl—Spr | 1.11 | 0.10 |
Wfl—Fall | −1.86 | 0.11 |
B.Seabass, black sea bass; Scup, scup; Sumfl, summer flounder; Wfl, winter flounder.
The slope of the difference between the 75th and 25th percentile of the along-shelf centre of biomass for each species over time.
Species . | Slope . | p-value . |
---|---|---|
B.Seabass—Spr | 1.73 | 0.21 |
B.Seabass—Fall | 2.11 | 0.41 |
Scup—Spr | −3.11 | 0.16 |
Scup—Fall | −2.61 | 0.27 |
Sumfl—Spr | −0.17 | 0.92 |
Sumfl—Fall | −0.37 | 0.73 |
Wfl—Spr | 1.11 | 0.10 |
Wfl—Fall | −1.86 | 0.11 |
Species . | Slope . | p-value . |
---|---|---|
B.Seabass—Spr | 1.73 | 0.21 |
B.Seabass—Fall | 2.11 | 0.41 |
Scup—Spr | −3.11 | 0.16 |
Scup—Fall | −2.61 | 0.27 |
Sumfl—Spr | −0.17 | 0.92 |
Sumfl—Fall | −0.37 | 0.73 |
Wfl—Spr | 1.11 | 0.10 |
Wfl—Fall | −1.86 | 0.11 |
B.Seabass, black sea bass; Scup, scup; Sumfl, summer flounder; Wfl, winter flounder.
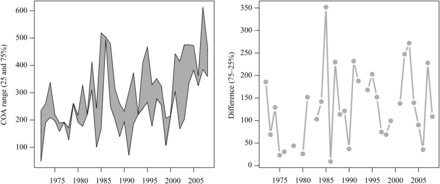
(a) The 25th and 75th percentile of the along-shelf centre of abundance for black sea bass in the spring. (b) The difference between the 75th and 25th percentile of the along-shelf centre of abundance as a measure of range expansion–contraction.
The annual occupied range was not significantly related to the stratified-weighted abundance except for summer flounder in spring (Table 4). In general, at the scales examined, the occupied range was not a function of abundance suggesting that for the four species, changes in population were not driving changes in distribution.
The slope of the difference between the 75th and 25th percentile of the along-shelf centre of biomass for each species and abundance.
Species . | N . | p-value . | R2 . |
---|---|---|---|
B.Seabass—Spr | 9.99E−06 | 0.916 | |
B.Seabass—Fall | −1.43E−04 | 0.196 | |
Scup—Spr | −6.49E−06 | 0.454 | |
Scup—Fall | −5.92E−06 | 0.067 | |
Sumfl—Spr | 4.39E−04 | 0.035 | 0.1211 |
Sumfl—Fall | −8.26E−05 | 0.567 | |
Wfl—Spr | −4.51E−05 | 0.173 | |
Wfl—Fall | −7.88E−05 | 0.327 |
Species . | N . | p-value . | R2 . |
---|---|---|---|
B.Seabass—Spr | 9.99E−06 | 0.916 | |
B.Seabass—Fall | −1.43E−04 | 0.196 | |
Scup—Spr | −6.49E−06 | 0.454 | |
Scup—Fall | −5.92E−06 | 0.067 | |
Sumfl—Spr | 4.39E−04 | 0.035 | 0.1211 |
Sumfl—Fall | −8.26E−05 | 0.567 | |
Wfl—Spr | −4.51E−05 | 0.173 | |
Wfl—Fall | −7.88E−05 | 0.327 |
B.Seabass, black sea bass; Scup, scup; Sumfl, summer flounder; Wfl, winter flounder.
The slope of the difference between the 75th and 25th percentile of the along-shelf centre of biomass for each species and abundance.
Species . | N . | p-value . | R2 . |
---|---|---|---|
B.Seabass—Spr | 9.99E−06 | 0.916 | |
B.Seabass—Fall | −1.43E−04 | 0.196 | |
Scup—Spr | −6.49E−06 | 0.454 | |
Scup—Fall | −5.92E−06 | 0.067 | |
Sumfl—Spr | 4.39E−04 | 0.035 | 0.1211 |
Sumfl—Fall | −8.26E−05 | 0.567 | |
Wfl—Spr | −4.51E−05 | 0.173 | |
Wfl—Fall | −7.88E−05 | 0.327 |
Species . | N . | p-value . | R2 . |
---|---|---|---|
B.Seabass—Spr | 9.99E−06 | 0.916 | |
B.Seabass—Fall | −1.43E−04 | 0.196 | |
Scup—Spr | −6.49E−06 | 0.454 | |
Scup—Fall | −5.92E−06 | 0.067 | |
Sumfl—Spr | 4.39E−04 | 0.035 | 0.1211 |
Sumfl—Fall | −8.26E−05 | 0.567 | |
Wfl—Spr | −4.51E−05 | 0.173 | |
Wfl—Fall | −7.88E−05 | 0.327 |
B.Seabass, black sea bass; Scup, scup; Sumfl, summer flounder; Wfl, winter flounder.
Size effects
Among the four fish species, larger individuals were generally found further north, or there was no pattern between size and distribution (Figure 7; Supplemental material). In spring, scup and summer flounder segregated by size with the largest fish caught the furthest north. Black sea bass and winter flounder exhibited relatively little size segregation, with all sizes distributed relatively similarly along the shelf. The size distribution of summer flounder and winter flounder in the fall, was similar to that in spring. Smaller summer flounder were further south while larger individuals were further north (Figure 7a) and the different size classes of winter flounder were generally centred ∼550–650 km.
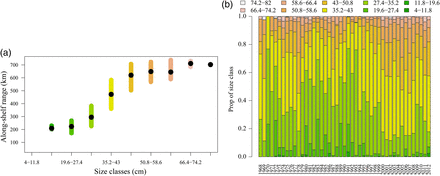
The along-shelf 25–75% range of each of the length classes (a) of summer flounder over the years 1972–2008 and the proportion of each length class each year (b) in the fall.
As indicated in Methods, the YOY of black sea bass and scup (the two smallest size classes) were predominately collected in the north (Supplementary material, Figures SA4 and SA8). In many years, the YOY were not encountered in the southern stations, because they had not yet moved offshore when the fall trawl survey occurred and were therefore not represented in the data. The next largest size class were caught in the most southerly locations. The individuals larger than the YOY size classes exhibited a relatively smooth distributional pattern.
Summer flounder and winter flounder did not exhibit the same strong YOY distribution pattern as black sea bass and scup. Summer flounder, however, move offshore around the same time in autumn as black sea bass and scup and thus could also be incompletely sampled. There is the potential that the YOY data for all species could be inadequate, therefore, both as a precaution and to be consistent, all YOY data in fall were removed. The removal of the YOY data did not alter any of the major conclusions of the study. Overall, there was evidence for size effects for summer flounder (both seasons) and black sea bass and scup (fall) (Figure 4). The SNE/MAB stock of winter flounder exhibited weak to no evidence for increasing size with latitude.
The proportion of different size classes constituting total abundance was relatively similar between the two seasons for summer flounder and winter flounder, but not for black sea bass and scup. The proportion of larger summer flounder varied during the time series and was lowest in the late 1980s—early 1990s. The proportion of larger fish then increased during the late 1990s and 2000s in both seasons (Figure 7b; Supplementary material, Figure SA12c). The proportion of different size classes of winter flounder varied over the time series with a slight increase in larger fish during the 2000s that was similar in both seasons. The proportions of different size classes for black sea bass and scup were different between the two seasons with substantially more juveniles present in autumn(fish ∼<15 cm). The fall trawl survey catches a large proportion of age-0 and age-1 fish moving offshore as the temperature declines, but the majority of these fish are caught in the more northerly areas of the stock range.
Discussion
Changes in environmental conditions and changes in fishing mortality are important mechanisms which affect the distribution of marine taxa (Engelhard et al., 2011). It is clear from the analyses that all species cannot be assumed to move poleward with increasing temperatures and that all range shifts cannot simply be attributed to climate change. Of the four species examined in detail, the distribution of black sea bass and scup had a significant relationship with temperature, summer flounder centre of biomass was significantly related to the length structure and thus to fishing mortality and the distribution of winter flounder did not shift.
Increasing temperatures due to climate change (Hypothesis 1) have shifted the thermal habitat of many natural marine resources further north. Thermal habitat is often limiting during winter, when temperature is considered one of the major constraints on survival (Hurst, 2007). Winter conditions reduce the suitable habitat and limit the ranges of fish species. Along the northeast shelf, the northern range of many species such as croaker is limited by winter temperatures (Hare and Able, 2007). Their ranges contract during cooler periods and expand during warmer periods.
During winter, black sea bass and scup are typically concentrated on the shelf in water >7°C (Neville and Talbot, 1964; Drohan et al., 2007; Moser and Shepherd, 2009). Colder winters typically restrict the available thermal habitat, as was evident in the early part of the 1900s, when severe winters enabled good catches with limited effort for the winter fishery (Neville and Talbot, 1964). Despite the thermal constraints, the two species are moving north and have shifted their spring centre of biomass by 150–200 km over the past four decades. The generalized additive models attribute the shifts to changes in temperature, which has increased on the shelf (Friedland and Hare, 2007; Belkin, 2009). The 7°C isotherm has shifted further north enabling the two species to survive at higher latitudes and occupy new habitat. The full population of black sea bass and scup, from YOY to adults, is offshore in March and April when the spring trawl survey occurs making this survey a good sample of the two stocks distributions.
During the warmer months, the lower thermal constraints are reduced and other factors, such as the magnitude of seasonal movement, are typically more important in regulating a species range. Larger fish can generally migrate further than smaller individuals and move further from their offshore, overwintering grounds. The fall trawl survey, however, does not provide a good sample of the full population for black sea bass and scup, as it largely catches age-0 and age-1 individuals (NEFSC, 2012; Shepherd, 2012; Terceiro, 2012a). Due to the biased catch, fall, along-shelf centre of biomass does not provide a good measure of the distribution for the entire population, but largely reflects the proportion of juveniles. Black sea bass and scup are spatially segregated by length in fall with larger individuals further north. The centre of biomass is further south when the number of juveniles is high and further north when the number of juveniles is low. This is reflected in the significant mean length term in the fall generalized additive models.
While temperature appears to be the main driver affecting changes in the centre of biomass for black sea bass, fishing may also have a limited role. Along the east coast of the United States, the recreational fishing sector accounts for up to 51% of the total allowable catch and regulations vary by state (ASMFC, 2014). Minimum size limits tend to increase moving north, while bag limits tend to decrease (ASMFC, 2014). The potential variable fishing pressure could also contribute to the shift in distribution. Temperature is still considered the main driver, however, because fishing could not account for the recent emergence of a black sea bass fishery in the Gulf of Maine where they were once rare visitors.
The summer flounder stock declined in the 1980s under intense fishing pressure, but rebounded in the late 1990s as management regulations came into effect. The stock is currently above the management target for biomass (Bmsy) (Terceiro, 2012b). Summer flounder are spatially distributed by size, with larger individuals further north. As the population declined with fishing, the larger, older individuals were depleted, and the proportion of smaller fish increased constituting the bulk of the summer flounder stock. Smaller fish live at lower latitudes and the centre of biomass shifted south. Fishing regulations in the 1990s reduced mortality leading to an increase in the population and an expansion of the length–age structure. The proportion of larger fish (>43 cm) is currently as high or higher than it has been during the entire time series. The larger, older individuals repopulated areas in which they historically resided and as their numbers increased, they pulled the centre of biomass north.
Temperature is not an important driver for summer flounder in this analysis, which corroborates with previous findings that it is not currently a major driver affecting recruitment (Bell et al., 2014). Laboratory studies suggest that summer flounder recruitment should vary inversely with winter temperatures because larvae overwinter in nearshore areas and experience mortality at temperatures <2–4°C (Malloy and Targett, 1991, 1994; Szedlmayer et al., 1992). Despite large changes in summer flounder abundance over the last three decades, recruitment has been relatively stable and exhibited no relationship with winter temperatures (Bell et al., 2014). Changes in the abundance of summer flounder, as well as the size structure and distribution, have been largely governed by changes in the mortality of older age classes through fishing and not by changes in the natural mortality due to temperature during the early life stages.
Fishing was the primary driver of changes in distribution with the level of fishing mortality determining the proportion of different size classes in the population. This implicates Hypothesis 3—size effects, because it explains how the effects of fishing would impact the distribution. Summer flounder were not moving to remain in shifting optimal habitat, but the different size classes experienced different levels of mortality, which altered the abundance of different ages, and subsequently shifted the centre of biomass north or south.
Temperature could still be important, however. The different size classes of summer flounder may still be following suitable habitat and shifting their distribution north with the increase in temperature, but the current impact of fishing is far greater, overwhelming any temperature signal. Potential effects of climate change may only be noticeable after the population equilibrates with the fishing mortality and a stable age structure is achieved. Future changes in fishing mortality with different management regulations, however, have the potential to quickly alter the length distribution of the population and shift the distribution independent of any temperature increase.
The SNE/MAB stock of winter flounder exhibited no change in the along-shelf centre of biomass over the time series. Previous work has suggested that the range of winter flounder has shifted north, but included the SNE/MAB and Georges Bank stocks as one unit (Nye et al., 2009). Partitioning the species at the Great South Channel agrees with the current understanding of the stock structure (NEFSC, 2011), but may impose a historical boundary that is superseded by the habitat needs of the population. Winter flounder is a cold water species which is potentially susceptible to increases in temperature (Rose, 2005). There may be movement across the Great South Channel onto Georges Bank or a decline in the SNE/MAB stock and an increase in the Georges Bank stock. Within the SNE/MAB region however, there is no evidence of a shift north or a decline in the occupied along-shelf distance.
SNE/MAB winter flounder abundance has declined since the 1980s with a slight increase in the 2000s (NEFSC, 2011). Their range however has not shifted or contracted suggesting that winter flounder still occupy the same area, but their total density within that area is greatly reduced. The lack of a range shift may be due to their life history. Adult winter flounder exhibits a moderate-to-high level of homing and spawn each winter in their natal estuary (Collette and Klein-MacPhee, 2002). The productivity of the stock has declined with warming conditions leading to lower recruitment (Bell et al., 2014). Because they show site fidelity, it appears that individuals are not shifting north with their suitable habitat, but return to their natal estuary where conditions are suboptimal. Their total fitness is reduced potentially leading to a continued decline in abundance, but a stable range, contrary to what might be expected with a population decline. The abundance and not the distribution would exhibit a relationship with climate change, which explains the lack of significant terms and low deviance explained in the generalized additive models. The reduced number of individuals occupying the same range could also lead to extremely low densities within individual estuaries and the potential for inbreeding which has already been documented in New York (O'Leary et al., 2013).
The along-shelf centre of biomass for three of the four species has shifted north during recent decades. While many studies have found a relationship between the environment, typically temperature, and distribution shifts (Perry et al., 2005; Nye et al., 2009; Pinsky et al., 2013), a more species-specific analysis indicated that multiple factors regulate changes in distribution. The working hypotheses provided mechanisms to understand how different factors, such as shifting habitat or anthropogenic depletions, impact species distribution.
Climate change alters the physical environment in which an individual lives and has a direct influence on its overall fitness and reproductive output. As conditions change around the globe, the optimal habitat for many species has shifted poleward and will continue to shift. Species must either migrate to remain within suitable habitat or suffer the consequences. The exact mechanism enabling the shift is not always clear and is different for different types of organisms. For large migratory species, individuals may have the ability to move with changing conditions and remain within suitable habitat. For demersal species, however, such as those along the Northeast shelf, lower recruitment success and a decline in nursery conditions at the southern extent of their range and better recruitment and higher-quality nursery conditions in the northern extent of their range are considered to be important mechanisms, resulting in distribution shifts (Rijnsdorp et al., 2009). For species which exhibit spawning site fidelity such as winter flounder or salmon, shifting conditions could be particularly challenging. As suitable conditions move past a species historical range, species may not be able to alter their behaviour or adapt quickly enough to shift with it, leading to major declines in abundance.
Fishing can have major impacts on the distribution of marine organisms by removing individuals, reducing biodiversity, altering predation and competition and changing bottom habitat (Rijnsdorp et al., 2009). Fishing also alters the age/length structure, which can have serious impacts for species which segregate by size. As is clear from summer flounder, high fishing mortality rapidly removed the largest members of the population and shifted the centre of biomass south. The reduction in fishing pressure and recovery of the age structure then shifted the centre of biomass north ∼250 km in just under two decades.
Understanding these regulating factors enables management to develop policies on how range shifts affect stocks and how catch quota allocations can be implemented across state and national boundaries. For transboundary, size segregated species such as summer flounder and Pacific hake, these factors can be major issues. Changes in fishing pressure due to management decisions could rapidly shift species across state or national lines. These considerations will only become more important for stocks with catch allocations divided across political boundaries. Many natural marine resources will shift poleward or decline in the coming decades (Walther et al., 2002; Burrows et al., 2011; Poloczanska et al., 2013); however, the impacts of fishing and other factors can have major and more immediate impacts on distribution. Accounting for all these drivers is essential to proper management because they affect the sustainability of fish stocks and directly impact livelihoods.
Supplementary data
Supplementary material available at the ICESJMS online version of the manuscript.
Acknowledgements
We thank the hard working men and women of the Northeast Fisheries Science Center who battled the conditions to collect the survey data.pt. The manuscript was improved by three reviewers. This work was funded under a National Research Council Fellowship and requested by the Atlantic States Marine Fisheries Commission.
References
Author notes
Handling editor: Mary Hunsicker