-
PDF
- Split View
-
Views
-
Cite
Cite
Alexandra Silva, Morphometric variation among sardine (Sardina pilchardus) populations from the northeastern Atlantic and the western Mediterranean, ICES Journal of Marine Science, Volume 60, Issue 6, 2003, Pages 1352–1360, https://doi.org/10.1016/S1054-3139(03)00141-3
- Share Icon Share
Abstract
During the past decade, regional changes in the dynamics of the Atlanto-Iberian stock of sardine, and its exploitation by Portuguese and Spanish purse-seine fisheries, have increased the uncertainties in estimated trends of spawning biomass, stock abundance, and fishing mortality. Together with recent evidence for lack of discontinuities in the distribution of sardine eggs at the edges of the stock area, this casts doubts on the hypothesis that the stock is a panmictic, closed population. Sardine morphometric data (truss variables and landmark data) from 14 samples spanning the northeastern Atlantic and the western Mediterranean were analysed by multivariate and geometric methods. The analyses explored the homogeneity of sardine shape within the area studied, as well as its relation to that of adjacent and distant populations (Azores and northwestern Mediterranean). Principal components analysis on size-corrected truss variables and cluster analysis of mean fish shape using landmark data indicate that the shape of sardine off southern Iberia and Morocco is distinct from the shape of sardine in the rest of the area. The two groups of sardine are significantly separated by discriminant analysis, and their validity was confirmed by large percentages of correct classifications of test fish (87 and 86% of fish from the test sample were correctly classified into each group, respectively). There was also some evidence that fish from the western Mediterranean and the Azores form a separate morphometric group. These results question both the homogeneity within the Atlanto-Iberian sardine stock and the validity of its current boundaries.
Introduction
The sardine (Sardina pilchardus, Walbaum, 1792) is a clupeoid whose distribution in the northeastern Atlantic extends from the southern Celtic Sea and North Sea to Mauritania and Senegal, with residual populations also off the Azores, Madeira, and the Canary Islands (Parrish et al., 1989; Figure 1). It is also found throughout most of the Mediterranean Sea, although the degree of mixing between Mediterranean and Atlantic populations is unknown. Abundance is greatest in the coastal waters of Morocco, where annual purse-seine catches exceeded 400 000 t throughout the 1990s (FAO, 2001), but the species is also abundant in the Atlantic waters of the Iberian Peninsula, where it is the main target of the purse-seine fleets of Portugal and Spain. Total sardine catches in those countries peaked at around 250 000 t in the mid-1960s, but have declined during the past 15 years, and are currently at some 100 000 t (ICES, 2003). Sardine catches north of the Iberian Peninsula are comparatively small, although a dedicated fishery with annual catches of some 15 000–20 000 t existed in French waters up to the mid-1960s (ICES, 1979). Sardine fisheries also exist throughout the Mediterranean, but they have traditionally contributed less than 20% of the total catch there (Andreu, 1969).
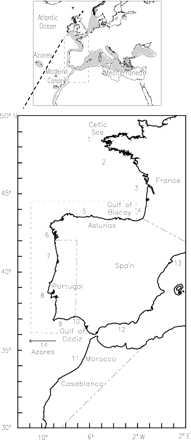
Locations of sampling sites (numbers) and delimitation of the stock area of Atlanto-Iberian sardine (dashed line). The map on the top shows the area of distribution of the species.
Attempts to differentiate sardine populations date from the 1920s (for reviews, see Andreu, 1969; Parrish et al., 1989), with most studies using univariate analyses of meristic characters (counts of vertebrae and gill rakers) or morphometric variables (e.g. the ratio of head to total length: the cephalic index). Differences in mean vertebral counts and the cephalic index were studied from restricted geographic areas and/or periods and led to various definitions of sardine races or geographical groups. However, failure to demonstrate persistent and significant phenotypic differences within a wide geographic area prevented consensus on the structure of sardine populations within its area of distribution. In the most thorough and geographically extensive study to date, the allometric relationship between the number of gillrakers and sardine length was considered the most appropriate index to differentiate populations. That analysis could only distinguish between sardine from the Atlantic continental shelf and those in the Mediterranean, and around Madeira and the Canary Islands (Andreu, 1969). Within the Atlantic, the most widely accepted subdivision is based on the early vertebral count study of Fage (1920), and it considers the existence of four sardine groups: the septentrional Atlantic group, distributed from the North Sea (57°N) to the Cantabrian coast of Spain (43°N), the Iberian or meridional Atlantic group, distributed off the Spanish and Portuguese coasts (from 43°N to 36°N), the Moroccan group, distributed from Cap Spartel (36°N) to Cap Juby (28°N), and the Saharian group, distributed from Cap Juby to Levrier Bay (21°N; Parrish et al., 1989).
For management purposes, sardine in European Atlantic waters have always been considered to belong to a single stock, although the geographical limits have changed over time. Exploratory sardine assessment began in 1978, using biological, fisheries, and survey data from France, Spain, and Portugal (ICES, 1978). At that time the stock was delimited within ICES Divisions VIII (Bay of Biscay and Cantabrian Sea) and IXa (western and southern Iberia). However, the biological criteria available for stock delimitation were not considered adequate and emphasis was placed on the need to clarify the relationships between populations within the assumed stock area (ICES, 1978). The northern border of the stock was redefined in 1980 (ICES, 1980), giving rise to what is currently known as the Atlanto-Iberian stock of sardine, delimited by the France/Spain border in the north, and by the Strait of Gibraltar in the south (ICES Divisions VIIIc and IXa). Routine assessment of the Atlanto-Iberian stock of sardine has been conducted annually since 1982 under the auspices of the International Council of the Exploration of the Sea (e.g. ICES, 2003).
Throughout the 1980s, the biological and fisheries data did not raise serious concerns about the area of delimitation or the biological homogeneity of the Atlanto-Iberian stock. However, recently there has been growing evidence of distinct regional changes in sardine distribution within the known stock area, increasing uncertainties in the estimates of stock size and in identification of trends in fishing mortality and abundance over time (ICES, 2000). This, together with recent observations indicating lack of discontinuities in the distribution of sardine eggs at the edges of the known stock area (ICES, 2000; Stratoudakis et al., 2003), casts new doubts on the hypothesis that the Atlanto-Iberian stock of sardine is a panmictic, closed population. The issue was addressed in a multivariate morphometric analysis of sardine samples from the area (ICES, 2000) that showed that head dimension increases from north to south. There is a latitudinal gradient from small head and large body size of sardine in the north (inner Bay of Biscay) to a large head and small body size in the south (southern Portuguese waters, and the Gulf of Cadiz). It was not possible to identify geographical areas with distinct sardine morphotypes in the study, and the need to increase the size and geographical range of samples was acknowledged (ICES, 2000).
In this paper, I examine the morphometric variability of sardine sampled from a broad geographic range (from the Celtic Sea to Morocco, and from the western Mediterranean to the Azores). The results are derived from a large number of fish per sample, and state-of-the-art methods of morphometric data collection (digital images) and analysis (combining multivariate and geometric morphometrics; Marcus et al., 1996; Cadrin and Friedland, 1999; Cadrin, 2000). The analysis explores the homogeneity of sardine shape within the Atlanto-Iberian stock area, its relation with adjacent areas to the north (France), south (Morocco), and west (western Mediterranean), and makes comparisons with a distant population (Azores). Finally, distinctive characters for discrimination among sardine morphotypes are identified, and the output of traditional multivariate analysis of morphometric data is compared with that obtained from recent geometric methods. In geometric methods, the analysis takes into account the geometry of configurations, providing additional information on shape differences that is not available from multivariate methods (Rohlf and Marcus, 1993). Therefore, combination of multivariate and geometric methods is expected to increase the chances of detecting the small morphometric differences that are anticipated at an intraspecific level.
Material and methods
A total of 14 samples of sardine (Table 1) was collected between December 1999 and May 2000 within an area between the Celtic Sea and the Moroccan coast, and from the western Mediterranean to the Azores (Figure 1). All samples were collected during research surveys, apart from those off Morocco and the Azores, which were obtained from commercial vessels at the port of landing. In those cases, samples were collected about 6 h after capture, but were kept in a good condition. Samples were frozen soon after collection and defrosted for laboratory analysis, which took place about 2 months later to ensure that all fish were analysed following a similar period of freezing. Digital photographs were taken with a Nikon Coolpix 950 (image resolution: 28.3 pixels cm−1) on the right side of each fish, and 15 landmarks were defined and recorded as two-dimensional coordinates (Figure 2). Landmarks were selected to provide a homogeneous coverage of the whole shape, but their homology and clarity in each fish were also taken into account. Ten of these landmarks (1–10) were used to calculate body distances on a truss network (Strauss and Bookstein, 1982), and 13 (1–10, 13–15) were used to describe body shape for geometric analysis (Rohlf and Slice, 1990). Data were examined using bivariate scatter plots of truss variables against fish total length, and plots of superimposed landmark configurations within each sample. From the total number of fish collected (1344), some were excluded from further analysis owing to incomplete (96) or clearly outlying measurements (49 in the truss analysis, 72 in the geometric analysis). The multivariate approach was applied to 1199 of the fish analysed, and the geometric method to 1176 fish; final sample sizes ranged from 53 to 103 fish (Table 1).
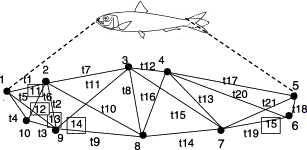
Location of anatomic landmarks and design of the truss network used in sardine morphometric analysis. Distances used in multivariate methods (t1–t21) were defined by landmarks 1–10 (truss network) and 11 and 12 (eye diameter). Landmarks 13–15 (indicated by squares) were only included in the geometric analysis.
Summary information of sardine samples (date, location, geographic area, and number of fish, n) used in morphometric analysis. Exact location is unknown for samples 11 and 14, which were obtained from commercial vessels. n1 and n2 indicate sample size for multivariate and geometric approaches, respectively.
Sample . | Date . | Latitude . | Longitude . | Country – area . | n1 . | n2 . |
---|---|---|---|---|---|---|
1 | 20 March 2000 | 48°30.0′N | 05°26.0′W | France – Celtic Sea | 70 | 67 |
2 | 11 May 2000 | 46°51.0′N | 04°56.7′W | France – NW coast | 78 | 77 |
3 | 22 April 2000 | 45°09.5′N | 02°02.8′W | France – SW coast | 98 | 96 |
4 | 10 April 2000 | 43°25.3′N | 01°44.5′W | Spain – Bay of Biscay | 72 | 77 |
5 | 6 April 2000 | 43°40.3′N | 06°30.1′W | Spain – Asturias | 87 | 84 |
6 | 25 March 2000 | 42°21.9′N | 08°47.7′W | Spain – S Galicia | 72 | 67 |
7 | 15 March 2000 | 40°59.8′N | 08°42.8′W | Portugal – NW coast | 99 | 96 |
8 | 21 March 2000 | 38°34.7′N | 09°18.4′W | Portugal – SW coast | 87 | 85 |
9 | 29 March 2000 | 37°05.2′N | 08°30.5′W | Portugal – S Algarve | 94 | 94 |
10 | 6 March 2000 | 36°33.3′N | 06°23.6′W | Spain – Gulf of Cadiz | 96 | 95 |
11 | January 2000 | – | – | Morocco – Casablanca | 97 | 93 |
12 | December 1999 | 36°41.0′N | 03°00.0′W | Spain – SW Mediterranean Sea | 103 | 100 |
13 | December 1999 | 40°41.0′N | 00°30.0′E | Spain – NW Mediterranean Sea | 53 | 53 |
14 | May 2000 | – | – | Portugal – Azores | 93 | 92 |
Total | 1199 | 1176 |
Sample . | Date . | Latitude . | Longitude . | Country – area . | n1 . | n2 . |
---|---|---|---|---|---|---|
1 | 20 March 2000 | 48°30.0′N | 05°26.0′W | France – Celtic Sea | 70 | 67 |
2 | 11 May 2000 | 46°51.0′N | 04°56.7′W | France – NW coast | 78 | 77 |
3 | 22 April 2000 | 45°09.5′N | 02°02.8′W | France – SW coast | 98 | 96 |
4 | 10 April 2000 | 43°25.3′N | 01°44.5′W | Spain – Bay of Biscay | 72 | 77 |
5 | 6 April 2000 | 43°40.3′N | 06°30.1′W | Spain – Asturias | 87 | 84 |
6 | 25 March 2000 | 42°21.9′N | 08°47.7′W | Spain – S Galicia | 72 | 67 |
7 | 15 March 2000 | 40°59.8′N | 08°42.8′W | Portugal – NW coast | 99 | 96 |
8 | 21 March 2000 | 38°34.7′N | 09°18.4′W | Portugal – SW coast | 87 | 85 |
9 | 29 March 2000 | 37°05.2′N | 08°30.5′W | Portugal – S Algarve | 94 | 94 |
10 | 6 March 2000 | 36°33.3′N | 06°23.6′W | Spain – Gulf of Cadiz | 96 | 95 |
11 | January 2000 | – | – | Morocco – Casablanca | 97 | 93 |
12 | December 1999 | 36°41.0′N | 03°00.0′W | Spain – SW Mediterranean Sea | 103 | 100 |
13 | December 1999 | 40°41.0′N | 00°30.0′E | Spain – NW Mediterranean Sea | 53 | 53 |
14 | May 2000 | – | – | Portugal – Azores | 93 | 92 |
Total | 1199 | 1176 |
Summary information of sardine samples (date, location, geographic area, and number of fish, n) used in morphometric analysis. Exact location is unknown for samples 11 and 14, which were obtained from commercial vessels. n1 and n2 indicate sample size for multivariate and geometric approaches, respectively.
Sample . | Date . | Latitude . | Longitude . | Country – area . | n1 . | n2 . |
---|---|---|---|---|---|---|
1 | 20 March 2000 | 48°30.0′N | 05°26.0′W | France – Celtic Sea | 70 | 67 |
2 | 11 May 2000 | 46°51.0′N | 04°56.7′W | France – NW coast | 78 | 77 |
3 | 22 April 2000 | 45°09.5′N | 02°02.8′W | France – SW coast | 98 | 96 |
4 | 10 April 2000 | 43°25.3′N | 01°44.5′W | Spain – Bay of Biscay | 72 | 77 |
5 | 6 April 2000 | 43°40.3′N | 06°30.1′W | Spain – Asturias | 87 | 84 |
6 | 25 March 2000 | 42°21.9′N | 08°47.7′W | Spain – S Galicia | 72 | 67 |
7 | 15 March 2000 | 40°59.8′N | 08°42.8′W | Portugal – NW coast | 99 | 96 |
8 | 21 March 2000 | 38°34.7′N | 09°18.4′W | Portugal – SW coast | 87 | 85 |
9 | 29 March 2000 | 37°05.2′N | 08°30.5′W | Portugal – S Algarve | 94 | 94 |
10 | 6 March 2000 | 36°33.3′N | 06°23.6′W | Spain – Gulf of Cadiz | 96 | 95 |
11 | January 2000 | – | – | Morocco – Casablanca | 97 | 93 |
12 | December 1999 | 36°41.0′N | 03°00.0′W | Spain – SW Mediterranean Sea | 103 | 100 |
13 | December 1999 | 40°41.0′N | 00°30.0′E | Spain – NW Mediterranean Sea | 53 | 53 |
14 | May 2000 | – | – | Portugal – Azores | 93 | 92 |
Total | 1199 | 1176 |
Sample . | Date . | Latitude . | Longitude . | Country – area . | n1 . | n2 . |
---|---|---|---|---|---|---|
1 | 20 March 2000 | 48°30.0′N | 05°26.0′W | France – Celtic Sea | 70 | 67 |
2 | 11 May 2000 | 46°51.0′N | 04°56.7′W | France – NW coast | 78 | 77 |
3 | 22 April 2000 | 45°09.5′N | 02°02.8′W | France – SW coast | 98 | 96 |
4 | 10 April 2000 | 43°25.3′N | 01°44.5′W | Spain – Bay of Biscay | 72 | 77 |
5 | 6 April 2000 | 43°40.3′N | 06°30.1′W | Spain – Asturias | 87 | 84 |
6 | 25 March 2000 | 42°21.9′N | 08°47.7′W | Spain – S Galicia | 72 | 67 |
7 | 15 March 2000 | 40°59.8′N | 08°42.8′W | Portugal – NW coast | 99 | 96 |
8 | 21 March 2000 | 38°34.7′N | 09°18.4′W | Portugal – SW coast | 87 | 85 |
9 | 29 March 2000 | 37°05.2′N | 08°30.5′W | Portugal – S Algarve | 94 | 94 |
10 | 6 March 2000 | 36°33.3′N | 06°23.6′W | Spain – Gulf of Cadiz | 96 | 95 |
11 | January 2000 | – | – | Morocco – Casablanca | 97 | 93 |
12 | December 1999 | 36°41.0′N | 03°00.0′W | Spain – SW Mediterranean Sea | 103 | 100 |
13 | December 1999 | 40°41.0′N | 00°30.0′E | Spain – NW Mediterranean Sea | 53 | 53 |
14 | May 2000 | – | – | Portugal – Azores | 93 | 92 |
Total | 1199 | 1176 |
Total length and biological parameters (sex, stage of sexual maturity, fat content, and stomach weight) were recorded for each sardine photographed. Macroscopic maturity was classified according to a six-stage scale (adapted from Pinto, 1957; Pinto and Andreu, 1957): juvenile/immature (1), maturing (2), pre-spawning (3), spawning (4), post-spawning (5), and recovering (6). Fat content (quantity of fat in the abdominal cavity) was visually evaluated on an ordinal scale from 1 (no fat) to 4 (abdominal cavity full of fat; Furnestin, 1945). Stomach weight refers to the wet weight of the whole stomach, including the stomach wall. Otoliths were extracted from each fish and the age was subsequently estimated using standard methods and criteria (ICES, 1997). Preliminary analyses of the impact of biological characteristics on morphometric variables showed that the maturity stage considerably affected abdominal volume, introducing confounding effects in the comparison of samples containing fish of different spawning condition. Abdominal volume affected the vertical position of landmark 8, and therefore of two derived truss variables that correspond to nearly vertical distances (variables t8 and t16; Figure 2). Both the landmark and the truss variables associated with the abdomen were excluded from further analysis.
In all, 19 truss variables (t1–t7, t9–t15, t17–t21) and the eye diameter were analysed by multivariate methods, and the coordinates of 12 landmarks (1–7, 9, 10, 13–15) were used in a Procrustes analysis (Rohlf and Marcus, 1993). Truss variables (log-transformed) were corrected for size using the Burnaby method (Burnaby, 1966; Rohlf and Bookstein, 1987). This method requires that variables be log-transformed, and it assumes that the first eigenvector of the within-group covariance matrix of log-morphometric variables is a multivariate index of the size of fish. The effect of size is corrected by projecting the truss variables on the sub-space orthogonal to the space spanned by the size vector. The existence of a size vector common to all samples was tested by comparing the angle between the first eigenvector of the covariance matrix of each sample with the common size vector in the data set. Bootstrap 95% confidence limits (Manly, 1997) indicated that the angle between the common size vector and the individual size vectors was not significant for any of the samples, suggesting that a common size transformation would standardize all samples. After size correction, the correlation between truss variables and fish total length decreased considerably, confirming that the influence of size was effectively eliminated. In the geometric method, the correction for size was incorporated in the process of superposition of configurations (Rohlf and Slice, 1990), by dividing the coordinates of each landmark by the centroid size for each fish (the sum of squared distances between all landmarks and the centroid of the configuration).
Principal components analysis was applied to size-corrected truss variables to outline groups of samples and to identify influential variables (Johnson and Wichern, 1998). Principal components were extracted from the covariance matrix. Mean values (centroids) and 95% asymptotic confidence limits of the scores of individual sardine on the first two principal components were computed for each sample. In the geometric analysis, the landmark data determining the configuration of individuals in each sample were superimposed by generalized orthogonal Procrustes analysis (Rohlf and Slice, 1990). Configurations were centred, scaled, and rotated in order to minimize the sum-of-squared distances (Procrustes distances) between homologous landmarks of all individuals. Mean shapes (consensus configurations) were calculated for each sample as arithmetic means of optimally superimposed configurations. The matrix of Procrustes distances among mean shapes was analysed by hierarchical clustering using complete linkage (Johnson and Wichern, 1998). This algorithm is recommended for data that are anticipated to show a cline of poorly separated clusters (Kaufman and Rousseeuw, 1990).
Two main groups of samples were suggested by the morphometric analyses, and these groups were discriminated using Fisher's linear discriminant analysis of truss variables (Johnson and Wichern, 1998). Differences among the group means in the discriminant space were tested using the Mahalanobis distance, and the consistency of the groups was evaluated by computing the misclassification rates of new individuals (Johnson and Wichern, 1998). A total of 150 sardine (100 from the larger group and 50 from the smaller group) was randomly withdrawn before the discriminant analysis to establish a sample for validation, and the remaining fish (training sample) were used to estimate the discriminant functions. The scores of individual fish and the corresponding Euclidean distances from the group centroids were calculated for the validation sample. Each sardine was assigned to the group with the nearest centroid, and the percentage of individual fish from one group correctly classified was computed. All calculations were carried out with the software S-Plus 2000 for Windows (Statistical Sciences, Inc.).
Results
Table 2 summarizes the biological characteristics of the 14 sardine samples used in the analysis. Total length in the pooled samples ranged between 12.0 and 24.7 cm (mean 17.9 cm), corresponding to ages of 1–10 years (mean 2.3 years). Length and age distributions generally overlapped considerably among samples. Sardine in the Mediterranean and Celtic Sea samples were overall the smallest (mean lengths around 15 cm). The largest sardine were caught close to the boundaries of the Atlanto-Iberian stock area, with a mean length of 22.1 cm in Asturias, 20.3 cm in Algarve (south Portugal), and 22.0 cm off northwestern France. Sampling dates were intended to follow the south-to-north progress of the spawning season. Samples were mainly spawning fish, as indicated by both advanced maturity and low fat content, except off the Azores, where the biological data suggest a resting condition, and from the Celtic Sea, where fish were possibly in a pre-spawning state.
Summary of biological characteristics of sardine in each sample. The percentage of fish spawning corresponds to fish in pre-spawning and spawning condition (maturity stages 3, 4, and 6). Sex ratio is the percentage of females, and s.d. is the standard deviation.
Sample . | Mean length (cm) and (range) . | Mean age in years and (range) . | Sex ratio (%) . | Percentage of fish spawning . | Mean stomach weight/body weight (s.d.) . | Dominant fat stage (%) . |
---|---|---|---|---|---|---|
1 | 15.6 (12.0–18.7) | 1.0 (1–2) | 57.1 | 22 | 4.6 (1.2) | 1 (98) |
2 | 22.1 (19.3–24.7) | 4.3 (2–8) | 51.3 | 100 | 6.0 (1.0) | 1 (78) |
3 | 16.3 (14.0–19.0) | 1.1 (1–2) | 42.9 | 66 | 6.3 (1.2) | 1 (78) |
4 | 17.8 (15.8–20.2) | 1.5 (1–3) | 45.2 | 100 | 5.1 (0.7) | 1 (99) |
5 | 22.1 (20.2–24.7) | 4.4 (2–8) | 44.8 | 100 | 6.2 (1.0) | 1 (100) |
6 | 17.2 (15.0–22.8) | 1.4 (1–3) | 30.6 | 100 | 5.2 (0.7) | 1 (68) |
7 | 19.1 (16.6–22.1) | 2.7 (1–6) | 68.7 | 100 | 8.1 (0.9) | 1 (100) |
8 | 17.3 (15.0–19.9) | 1.5 (1–4) | 43.2 | 67 | 7.0 (1.1) | 1 (74) |
9 | 20.3 (18.0–22.4) | 5.8 (2–10) | 38.3 | 96 | 5.9 (0.9) | 1 (96) |
10 | 17.2 (14.5–20.7) | 2.3 (1–6) | 43.0 | 54 | 4.3 (0.9) | 1 (57) |
11 | 16.7 (12.0–18.5) | 2.0 (1–4) | 49.5 | 98 | 5.5 (0.4) | 1 (74) |
12 | 15.3 (13.3–20.6) | 1.2 (1–3) | 25.2 | 100 | 4.0 (1.1) | 2 (71) |
13 | 15.6 (13.5–19.4) | 1.5 (1–3) | 60.4 | 51 | 3.5 (0.6) | 1 (79) |
14 | 16.7 (13.7–18.3) | 1.1 (1–2) | 61.3 | 0 | 6.3 (0.9) | 4 (70) |
Total/mean | 17.9 (12.0–24.7) | 2.3 (1–10) | 46.8 | 76.6 | 5.7 | 1 |
Sample . | Mean length (cm) and (range) . | Mean age in years and (range) . | Sex ratio (%) . | Percentage of fish spawning . | Mean stomach weight/body weight (s.d.) . | Dominant fat stage (%) . |
---|---|---|---|---|---|---|
1 | 15.6 (12.0–18.7) | 1.0 (1–2) | 57.1 | 22 | 4.6 (1.2) | 1 (98) |
2 | 22.1 (19.3–24.7) | 4.3 (2–8) | 51.3 | 100 | 6.0 (1.0) | 1 (78) |
3 | 16.3 (14.0–19.0) | 1.1 (1–2) | 42.9 | 66 | 6.3 (1.2) | 1 (78) |
4 | 17.8 (15.8–20.2) | 1.5 (1–3) | 45.2 | 100 | 5.1 (0.7) | 1 (99) |
5 | 22.1 (20.2–24.7) | 4.4 (2–8) | 44.8 | 100 | 6.2 (1.0) | 1 (100) |
6 | 17.2 (15.0–22.8) | 1.4 (1–3) | 30.6 | 100 | 5.2 (0.7) | 1 (68) |
7 | 19.1 (16.6–22.1) | 2.7 (1–6) | 68.7 | 100 | 8.1 (0.9) | 1 (100) |
8 | 17.3 (15.0–19.9) | 1.5 (1–4) | 43.2 | 67 | 7.0 (1.1) | 1 (74) |
9 | 20.3 (18.0–22.4) | 5.8 (2–10) | 38.3 | 96 | 5.9 (0.9) | 1 (96) |
10 | 17.2 (14.5–20.7) | 2.3 (1–6) | 43.0 | 54 | 4.3 (0.9) | 1 (57) |
11 | 16.7 (12.0–18.5) | 2.0 (1–4) | 49.5 | 98 | 5.5 (0.4) | 1 (74) |
12 | 15.3 (13.3–20.6) | 1.2 (1–3) | 25.2 | 100 | 4.0 (1.1) | 2 (71) |
13 | 15.6 (13.5–19.4) | 1.5 (1–3) | 60.4 | 51 | 3.5 (0.6) | 1 (79) |
14 | 16.7 (13.7–18.3) | 1.1 (1–2) | 61.3 | 0 | 6.3 (0.9) | 4 (70) |
Total/mean | 17.9 (12.0–24.7) | 2.3 (1–10) | 46.8 | 76.6 | 5.7 | 1 |
Summary of biological characteristics of sardine in each sample. The percentage of fish spawning corresponds to fish in pre-spawning and spawning condition (maturity stages 3, 4, and 6). Sex ratio is the percentage of females, and s.d. is the standard deviation.
Sample . | Mean length (cm) and (range) . | Mean age in years and (range) . | Sex ratio (%) . | Percentage of fish spawning . | Mean stomach weight/body weight (s.d.) . | Dominant fat stage (%) . |
---|---|---|---|---|---|---|
1 | 15.6 (12.0–18.7) | 1.0 (1–2) | 57.1 | 22 | 4.6 (1.2) | 1 (98) |
2 | 22.1 (19.3–24.7) | 4.3 (2–8) | 51.3 | 100 | 6.0 (1.0) | 1 (78) |
3 | 16.3 (14.0–19.0) | 1.1 (1–2) | 42.9 | 66 | 6.3 (1.2) | 1 (78) |
4 | 17.8 (15.8–20.2) | 1.5 (1–3) | 45.2 | 100 | 5.1 (0.7) | 1 (99) |
5 | 22.1 (20.2–24.7) | 4.4 (2–8) | 44.8 | 100 | 6.2 (1.0) | 1 (100) |
6 | 17.2 (15.0–22.8) | 1.4 (1–3) | 30.6 | 100 | 5.2 (0.7) | 1 (68) |
7 | 19.1 (16.6–22.1) | 2.7 (1–6) | 68.7 | 100 | 8.1 (0.9) | 1 (100) |
8 | 17.3 (15.0–19.9) | 1.5 (1–4) | 43.2 | 67 | 7.0 (1.1) | 1 (74) |
9 | 20.3 (18.0–22.4) | 5.8 (2–10) | 38.3 | 96 | 5.9 (0.9) | 1 (96) |
10 | 17.2 (14.5–20.7) | 2.3 (1–6) | 43.0 | 54 | 4.3 (0.9) | 1 (57) |
11 | 16.7 (12.0–18.5) | 2.0 (1–4) | 49.5 | 98 | 5.5 (0.4) | 1 (74) |
12 | 15.3 (13.3–20.6) | 1.2 (1–3) | 25.2 | 100 | 4.0 (1.1) | 2 (71) |
13 | 15.6 (13.5–19.4) | 1.5 (1–3) | 60.4 | 51 | 3.5 (0.6) | 1 (79) |
14 | 16.7 (13.7–18.3) | 1.1 (1–2) | 61.3 | 0 | 6.3 (0.9) | 4 (70) |
Total/mean | 17.9 (12.0–24.7) | 2.3 (1–10) | 46.8 | 76.6 | 5.7 | 1 |
Sample . | Mean length (cm) and (range) . | Mean age in years and (range) . | Sex ratio (%) . | Percentage of fish spawning . | Mean stomach weight/body weight (s.d.) . | Dominant fat stage (%) . |
---|---|---|---|---|---|---|
1 | 15.6 (12.0–18.7) | 1.0 (1–2) | 57.1 | 22 | 4.6 (1.2) | 1 (98) |
2 | 22.1 (19.3–24.7) | 4.3 (2–8) | 51.3 | 100 | 6.0 (1.0) | 1 (78) |
3 | 16.3 (14.0–19.0) | 1.1 (1–2) | 42.9 | 66 | 6.3 (1.2) | 1 (78) |
4 | 17.8 (15.8–20.2) | 1.5 (1–3) | 45.2 | 100 | 5.1 (0.7) | 1 (99) |
5 | 22.1 (20.2–24.7) | 4.4 (2–8) | 44.8 | 100 | 6.2 (1.0) | 1 (100) |
6 | 17.2 (15.0–22.8) | 1.4 (1–3) | 30.6 | 100 | 5.2 (0.7) | 1 (68) |
7 | 19.1 (16.6–22.1) | 2.7 (1–6) | 68.7 | 100 | 8.1 (0.9) | 1 (100) |
8 | 17.3 (15.0–19.9) | 1.5 (1–4) | 43.2 | 67 | 7.0 (1.1) | 1 (74) |
9 | 20.3 (18.0–22.4) | 5.8 (2–10) | 38.3 | 96 | 5.9 (0.9) | 1 (96) |
10 | 17.2 (14.5–20.7) | 2.3 (1–6) | 43.0 | 54 | 4.3 (0.9) | 1 (57) |
11 | 16.7 (12.0–18.5) | 2.0 (1–4) | 49.5 | 98 | 5.5 (0.4) | 1 (74) |
12 | 15.3 (13.3–20.6) | 1.2 (1–3) | 25.2 | 100 | 4.0 (1.1) | 2 (71) |
13 | 15.6 (13.5–19.4) | 1.5 (1–3) | 60.4 | 51 | 3.5 (0.6) | 1 (79) |
14 | 16.7 (13.7–18.3) | 1.1 (1–2) | 61.3 | 0 | 6.3 (0.9) | 4 (70) |
Total/mean | 17.9 (12.0–24.7) | 2.3 (1–10) | 46.8 | 76.6 | 5.7 | 1 |
The principal components analysis of size-corrected truss variables showed that the first two principal components (PC) account for 50.2% of the total variance (33.1% for PC1, 17.1% for PC2). The first PC is essentially a contrast between the dimensions of the fish head (t1–t6 and ED), and of the fish body (t7, t9–t15, t17–t21; Figure 3a). A group of three samples, from southern Portuguese waters, the Gulf of Cadiz, and Morocco (samples 9–11, respectively), segregates from the remaining samples on this PC (Figure 3b), the first group having a larger head and smaller body dimensions than the latter. PC2 is dominated by variables t12 and t13, indicating differences in the length of the dorsal fin base. There is more overlap among samples on this PC, however, fish from sample 12 (southwestern Mediterranean) showing slightly larger mean values of dorsal base length.
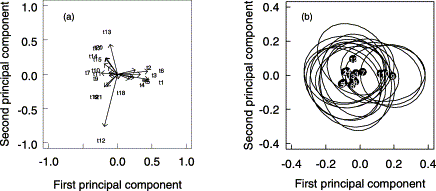
Main results of the principal components analysis on size-corrected truss variables. Vectors indicate (a) the loadings and (b) centroids (black dots) and 95% confidence ellipses of the scores for each of the samples on the first two principal components.
The cluster analysis of distances among mean sardine shape of each sample supports the two-group structure highlighted by the principal components analysis (Figure 4), but in this case there is some additional evidence that samples from the Mediterranean and the Azores (samples 12–14) form a separate cluster from the French coast–northern Iberia group (samples 1–8). Figure 5 shows the average sardine for each of the three groups of samples, French coast–northern Iberia (samples 1–8), southern Iberia–Morocco (samples 9–11), and Mediterranean–Azores (samples 12–14), and the configuration of the overall mean fish. The most evident difference between the mean shape of sardine from the southern Iberia–Morocco cluster and sardine from the other two groups is the larger absolute head dimension and the higher proportion of head to overall body size. On the other hand, sardine from the French coast–northern Iberia group have a more slender body and a more posterior insertion of the dorsal fin, and these differences are larger than the ones when comparing sardine from the Mediterranean–Azores group with sardine from southern Iberia.
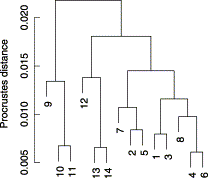
Dendrogram resulting from cluster analysis of Procrustes distances among sardine mean shape for each of the samples.
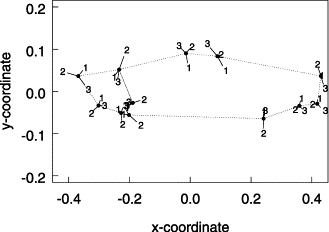
Landmark coordinates of the average individuals from the three groups of samples suggested by cluster analysis of Procrustes distances among sample means. The black dots are the coordinates of the overall mean individual. Segments correspond to the difference between the mean individuals of each group and the overall mean individual. The length of the segments was enlarged six times to show the differences among landmark positions. Fish head is on the left and the dorsal side on top of the plot. Group 1: French coast–northern Iberia (samples 1–8), Group 2: southern Iberia–Morocco (samples 9–11), Group 3: Mediterranean–Azores (samples 12–14).
The discriminant function based on the two main groups, northern Atlantic–Mediterranean and southern Iberia–Morocco, suggested by both multivariate and geometric analyses, has a discriminant correlation of 0.77, indicating that a considerable proportion of total variability in the discriminant space corresponds to within-group variability (41.5%; Table 3). However, the Mahalanobis distance between the two group means (8.06) is significant at 1% level (Figure 6), and the high percentage of correct classification of new fish (87% for the northern Atlantic–Mediterranean group, 86% for the southern Iberia–Morocco group) provides support to the differences between the groups, and highlights their homogeneity (Table 4). The pattern of correlation of the original variables with the discriminant function is similar to the pattern of loadings on the first PC, indicating that the dimensions of the head and the eye diameter explain most of the morphometric differences between sardine from the northern Atlantic–Mediterranean and those from southern Iberia–Morocco.
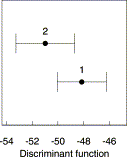
Plots of the centroids of the groups of sardine in the discriminant function. The error bars correspond to two standard deviations of the group observations on the discriminant function. Group 1: North Atlantic–Mediterranean (samples 1–8 and 12–14), Group 2: southern Iberia–Morocco (samples 9–11).
Coefficients of correlation of the morphometric variables (body distances on a truss network) with the discriminant function. The corresponding discriminant capacity is shown at the bottom of the table.
Morphometric variable . | Discriminant function . |
---|---|
t1 | −0.61 |
t2 | −0.71 |
t3 | −0.44 |
t4 | −0.45 |
t5 | −0.60 |
t6 | −0.76 |
t7 | 0.51 |
t9 | 0.26 |
t10 | 0.30 |
t11 | 0.26 |
t12 | 0.36 |
t13 | 0.19 |
t14 | 0.49 |
t15 | 0.51 |
t17 | 0.57 |
t18 | −0.19 |
t19 | 0.52 |
t20 | 0.59 |
t21 | 0.40 |
Eye diameter | −0.61 |
Discriminant capacity (%) | 58.5 |
Morphometric variable . | Discriminant function . |
---|---|
t1 | −0.61 |
t2 | −0.71 |
t3 | −0.44 |
t4 | −0.45 |
t5 | −0.60 |
t6 | −0.76 |
t7 | 0.51 |
t9 | 0.26 |
t10 | 0.30 |
t11 | 0.26 |
t12 | 0.36 |
t13 | 0.19 |
t14 | 0.49 |
t15 | 0.51 |
t17 | 0.57 |
t18 | −0.19 |
t19 | 0.52 |
t20 | 0.59 |
t21 | 0.40 |
Eye diameter | −0.61 |
Discriminant capacity (%) | 58.5 |
Coefficients of correlation of the morphometric variables (body distances on a truss network) with the discriminant function. The corresponding discriminant capacity is shown at the bottom of the table.
Morphometric variable . | Discriminant function . |
---|---|
t1 | −0.61 |
t2 | −0.71 |
t3 | −0.44 |
t4 | −0.45 |
t5 | −0.60 |
t6 | −0.76 |
t7 | 0.51 |
t9 | 0.26 |
t10 | 0.30 |
t11 | 0.26 |
t12 | 0.36 |
t13 | 0.19 |
t14 | 0.49 |
t15 | 0.51 |
t17 | 0.57 |
t18 | −0.19 |
t19 | 0.52 |
t20 | 0.59 |
t21 | 0.40 |
Eye diameter | −0.61 |
Discriminant capacity (%) | 58.5 |
Morphometric variable . | Discriminant function . |
---|---|
t1 | −0.61 |
t2 | −0.71 |
t3 | −0.44 |
t4 | −0.45 |
t5 | −0.60 |
t6 | −0.76 |
t7 | 0.51 |
t9 | 0.26 |
t10 | 0.30 |
t11 | 0.26 |
t12 | 0.36 |
t13 | 0.19 |
t14 | 0.49 |
t15 | 0.51 |
t17 | 0.57 |
t18 | −0.19 |
t19 | 0.52 |
t20 | 0.59 |
t21 | 0.40 |
Eye diameter | −0.61 |
Discriminant capacity (%) | 58.5 |
Percentage of new individuals re-allocated in each group in validation of the discriminant function. Group North Atlantic–Mediterranean: samples 1–8 and 12–14; Group southern Iberia–Morocco: samples 9–11. Classification was for 100 sardine from the first group and 50 sardine from the second group.
. | Re-allocation group . | |
---|---|---|
Original group . | North Atlantic–Mediterranean . | Southern Iberia–Morocco . |
North Atlantic–Mediterranean | 87% | 13% |
Southern Iberia–Morocco | 14% | 86% |
. | Re-allocation group . | |
---|---|---|
Original group . | North Atlantic–Mediterranean . | Southern Iberia–Morocco . |
North Atlantic–Mediterranean | 87% | 13% |
Southern Iberia–Morocco | 14% | 86% |
Percentage of new individuals re-allocated in each group in validation of the discriminant function. Group North Atlantic–Mediterranean: samples 1–8 and 12–14; Group southern Iberia–Morocco: samples 9–11. Classification was for 100 sardine from the first group and 50 sardine from the second group.
. | Re-allocation group . | |
---|---|---|
Original group . | North Atlantic–Mediterranean . | Southern Iberia–Morocco . |
North Atlantic–Mediterranean | 87% | 13% |
Southern Iberia–Morocco | 14% | 86% |
. | Re-allocation group . | |
---|---|---|
Original group . | North Atlantic–Mediterranean . | Southern Iberia–Morocco . |
North Atlantic–Mediterranean | 87% | 13% |
Southern Iberia–Morocco | 14% | 86% |
Discussion
The distance and landmark morphometric data of sardine used in this study led to the identification of two morphological types, with geographic coherence within the northeastern Atlantic and the Mediterranean. Both morphometric methods indicated that sardine from southern Iberia (southern Portugal, Gulf of Cadiz) and northern Morocco had a morphotype distinct from the remaining area. Discrimination of the two morphotypes was confirmed statistically by the significant difference between group centroids and by the high percentage of correct classification (>85%) of new fish. Sardine from the northern Atlantic and from the Mediterranean area had a smaller head, eye diameter, and head-to-body ratio than those from southern Iberia and northern Morocco (Figures 4 and 6). These differences reinforce the results of a recent multivariate study of sardine morphometry in Iberian waters that suggested a southerly increasing latitudinal gradient in the absolute and relative size of the head (ICES, 2000). Unlike observed here, that study provided no evidence of a discontinuity in sardine shape along the study area. This lack of agreement is not fully understood, but the smaller sample size and less precise morphometric measurements used by ICES (2000) possibly decreased the chance of detecting differences among samples that have considerable variability and overlap. Earlier univariate analyses on the cephalic index (Andreu, 1969; Fréon and Stéquert, 1982) also indicated an increase in the head-to-body ratio from north to south within the Atlantic.
Apart from the identification of two morphotypes based on head-to-body ratios, there is also some evidence for the identification of distinct morphological groups, mainly on the basis of the position of the dorsal fin (Figure 4). This character separates sardine from northern Iberia and the Mediterranean, consistent with earlier studies that have identified phenotypic differences between sardine in Mediterranean and Atlantic European waters (Parrish et al., 1989). It should also be noted that the sample from the Azores is grouped in the same morphotype as sardine from the Mediterranean. This is a counter-intuitive association if one takes into account the geographic relationship between the areas, but it has some support from the few existing studies that have analysed samples from the Azores. The local population had closer affinities with the Mediterranean than with the Atlantic populations in terms of cephalic index and growth rate of gillrakers (Ramalho, 1929; Andreu, 1969).
However, the separation of the Mediterranean–Azores group from sardine from northern Iberia was only depicted by the geometric analysis, so the result should be viewed with caution. The discrepancy between the results from the traditional multivariate and geometric methods regarding this separation may be explained partly by allometric differences between fish from the two groups, because a Procrustes analysis only corrects isometric size (the Mediterranean–Azores group has globally smaller sardine; Klingenberg, 1996). This is partly supported by the similarity between the pattern of sample clustering based on Procrustes distances, and on the analysis of truss distances corrected only for isometric size.
Morphometric features may also vary among cohorts, because the environment at the time of spawning and juvenile development changes between years (Meng and Stocker, 1984; Austin et al., 1999). The samples analysed in this study had different age compositions and were therefore represented by different cohorts. However, in this case the cohort composition does not seem to have affected the pattern of similarity among samples. In fact, the morphometric groups formed in the analyses are largely independent of the cohorts represented in their component samples.
Overall, the population structure suggested here increases doubts on the correct definition of the Atlanto-Iberian sardine stock, both regarding current boundaries and the homogeneity within the stock area. The lack of a boundary in the Bay of Biscay indicated by sardine morphology is compatible with the continuous distribution of sardine eggs and adults along the Cantabrian and French coasts (Anon., 2001). The situation is different for another small pelagic fish (Engraulis encrasicolus), for which populations in the Bay of Biscay segregate from populations in adjacent waters of Cantabria, possibly because oceanographic processes favour retention of eggs and larvae (Junquera and Perez-Gandáras, 1993). The discontinuity between sardine populations across the Strait of Gibraltar supports that feature as the eastern limit of the stock, but the link between sardine populations from the Gulf of Cadiz and northern Morocco implied here highlights the need to investigate the southern stock boundary further.
It is now commonly accepted that morphological variation has both environmental and genetic components, but that stable differences in shape among groups of fish may reveal different growth, mortality, or reproductive rates that are relevant for the definition of stocks (Swain and Foote, 1999; Cadrin, 2000). Here I used advanced image-processing techniques, the combination of two recent morphometric approaches (multivariate and geometric), and appropriate analytical methods to uncover differences in sardine from a wide geographic area. However, the temporal stability of morphometric groups depicted here has still to be confirmed, and samples from the 2003 spawning season will be analysed with that goal, focusing on areas of transition between the currently defined morphological groups. Comparison of the morphological variability in spawning and feeding seasons will provide additional insight into the temporal stability of the groups, and possibly into seasonal movements between areas. Finally, integrating morphometric information with the output of other phenotypic approaches, such as analysis of life history properties or otolith chemistry, and with genetic data, is essential for efficient stock identification (Begg and Waldman, 1999; Cadrin, 2000). Revision of the current stock definition should follow only if the morphological groups shown here prove to be persistent and coherent with other phenotypic and genetic structures of sardine populations.
I thank P. Carrera (IEO), J. Massé (IFREMER), D. Reis (Azores University–DOP), R. Abad (IEO), H. Mesfioui (INRH), and A. Morais (IPIMAR) for collaboration in the collection of samples (mainly as part of the EU Study PELASSES 080/99), and D. Morais, M. Garção, and A. Jorge for assistance in the laboratory. I thank particularly Y. Stratoudakis for his constant encouragement during the analysis of data and preparation of the manuscript. J. P. Granadeiro gave valuable assistance in S-Plus programming, and J. Cadima, C. D. Darby, M. A. Dias, and A. Murta provided helpful comments on drafts of the manuscript. Finally, Dominique Ponton and an anonymous reviewer made valuable suggestions on the submitted manuscript. The work was supported mainly by the project PELAGICOS (financed by the Portuguese Ministry of Science and Higher Education and affiliated to SPACC), and, in its final stages, by the EU project SARDYN (Q5RS-2002-000818).